Incoherence between systemic and skin tissue response to transfusion in volume-resuscitated patients withoutacute bleeding
Abstract
Alterations in skin blood flow (SBF) may help to detect occult hypoperfusion in critically ill patients after fluid resuscitation. In this study, SBF is globally unaltered by red blood cell transfusion (RBCT) in non-bleeding critically ill patients after initial resuscitation; however, 37.5% of patients showed a significant increase in SBF. No correlation between relative changes in SBF and systemic variables after RBCT was observed.
Dear Editor,
The primary objectives of resuscitation encompass the restoration of adequate flow and pressure, thereby ameliorating tissue perfusion and mitigating tissue hypoxia. Nevertheless, several studies have highlighted that despite the normalization of systemic variables following the initial resuscitative efforts, tissues may persist in a state of hypoperfusion [1]. Consequently, the monitoring of tissue perfusion emerges as a valuable tool for identifying patients who may derive benefit from therapies aimed at enhancing microvascular blood flow and oxygenation [2, 3], with red blood cell transfusions (RBCT) representing one potential intervention. Indeed, the decision to transfuse an anemic patient is still a challenge in the intensive care unit (ICU) and should always consider the risks of anemia, including failed weaning from mechanical ventilation [4] or an increased risk of poor outcome [5], as well as the potential harms associated with RBCT [6]. As such, tissue perfusion may be a specific trigger used to decide when to transfuse an ICU patient in a more personalized way. Because alterations in skin perfusion can occur early in patients with shock, even before hemodynamic variables are altered, and remain altered despite hemodynamic stabilization, the skin is considered a window for detecting alteration in tissue perfusion [2]. The methods of cutaneous blood flow monitoring have been mainly based on optical microscopy [7–9] and laser Doppler techniques [10]. Microscopic techniques, such as nailfold capillaroscopy (NVC), provide a direct measurement of cutaneous microcirculation [7–9, 11] and have found clinical applications in diseases affecting digital skin microcirculation [8, 9, 11]. Besides microscopic methods, the Skin Laser Doppler (SLD) technique is used to estimate the skin blood flow (SBF) [11].
In the context of septic patients, Sakr et al. have reported that RBCT could ameliorate microvascular blood flow, but only in those cases where it was impaired at baseline [12]. Furthermore, the alterations in microcirculation following RBCT have exhibited no significant correlation with systemic variables [12]. SLD technique has been increasingly used to assess microvascular flow in critically ill patients at the bedside [2]. This technique provides measurements of blood flow in a variable volume of tissue, which represents the blood flow in arterioles, venules, and capillaries, with the volume of the venous vessels being predominant [10]. Therefore, SLD does not describe exclusively the skin microcirculatory blood flow [10]. Nevertheless, alterations in SBF assessed by SLD have been associated with the severity of the underlying disease [2], have prognostic value [2], and respond to clinically relevant interventions in critically ill patients [3, 13]. However, no data are available on the correlation between changes in global hemodynamics and SLD after RBCT in non-bleeding critically ill patients after initial resuscitation.
We performed a secondary analysis of critically ill patients who received RBCT and who were included in a previous study [14]; for this study, we included those patients who had cardiac output (CO) monitored during RBCT. Skin blood flow (SBF) measurements, hemoglobin (Hb), cardiac index (CI), heart rate (HR), mean arterial pressure (MAP), arterial lactate, central venous pressure (CVP), the partial pressure of carbon dioxide in venous (PvCO2) and arterial (PaCO2) blood, as well as their difference (Pv-aCO2), central or mixed venous oxygen saturation (ScvO2 or SvO2) and arterial oxygen saturation (SaO2) were obtained before and after RBCT. Oxygen-derived variables were calculated using standard formulas. Organ driving pressure (DP) was calculated using the difference between MAP and CVP. Relative changes (Δ) for all variables were calculated using the formula: ((Post-transfusion value – Pre-transfusion value)/Pre-transfusion value)×100%. Finally, the relationships between relative changes in systemic and microcirculatory variables were assessed.
Out of the cohort comprising 175 critically ill patients, 48 individuals (27.4%) met the eligibility criteria for this study. The baseline clinical characteristics of the study population are presented in Table 1. Our study found that RBC transfusion had no straightforward effect on skin microvascular flow in a group of critically ill patients after resuscitation without acute bleeding despite the significant improvement in macrohemodynamic parameters, including oxygen delivery (DO2) and ScvO2 or SvO2, as depicted in Table 2. However, there was considerable interindividual variability; 37.5% of patients had a significant increase in SBF at basal temperature (SBFBT) after RBCT (SBF responders – Table 2). No significant correlation was observed between the changes in SBFBT and the changes in CI (Fig. 1A), blood pressure (Fig. 1B), arterial lactate (Fig. 1C), Δ Pv-aCO2 (Fig. 1D), or DP (ΔDP) (Fig. 1E) after RBCT. The substantial rise in DO2 was associated with a significant increase in oxygen consumption (VO2), but solely within the group of SBF responders (Table 2).
Table 1
Characteristic of the study population, according to SBF response to RBCT (responders vs. non-responders)
All (n = 48) | Responders (n = 18) | Non-Responders (n = 30) | P value | |
Age, years | 63.8±11.4 | 66.7±7.6 | 62.1±13.0 | 0.18 |
Male gender, n (%) | 33.0 (68.8) | 14.0 (77.8) | 19.0 (63.3) | 0.29 |
APACHE II score at ICU admission | 20.7±6.8 | 22.6±7.0 | 19.6±6.6 | 0.14 |
SOFA score at ICU admission | 9.6±2.8 | 9.3±2.6 | 9.8±2.9 | 0.56 |
Characteristics of measurements | ||||
Time in ICU before study inclusion (days) | 3.0 (0.5–17.0) | 3.5 (0.5–17.0) | 3.0 (0.5–16.0) | 0.43 |
Interference, n (%) | 2.0 (4.2) | 1.0 (5.6) | 1.0 (3.3) | 0.99 |
Characteristics on the study day, n (%) | ||||
Mechanical ventilation | 26.0 (54.2) | 11.0 (61.1) | 15.0 (50.0) | 0.45 |
Sedation | 18.0 (37.5) | 8.0 (44.4) | 10.0 (33.3) | 0.44 |
Norepinephrine dose (mcg/Kg/min) | 0.09 (0.01–1.73) | 0.10 (0.01–0.78) | 0.08 (0.01–1.73) | 0.98 |
During ICU stay, n (%) | ||||
28-day mortality | 9.0 (18.8) | 4.0 (22.2) | 5.0 (16.7) | 0.71 |
Note: Data are reported as mean (SD), median (IQRs), or count (%). Abbreviations: APACHE II, Acute Physiologic Assessment and Chronic Health Evaluation Scoring System II; ICU, Intensive Care Unit; SOFA, Sequential Organ Failure Assessment.
Table 2
Hemodynamic and skin tissue perfusion parameters before and after RBCT
All patients (n = 48) | SBF responders (n = 18) | SBF No-responders (n = 30) | |||||||
Baseline | 1-hour | p | Baseline | 1-hour | p | Baseline | 1-hour | p | |
Hb, g/dL | 7.6±0.8 | 9.0±1.0 | <0.01 | 7.6±0.8 | 8.8±1.1 | <0.01 | 7.7±0.8 | 9.0±0.9 | <0.01 |
MAP, mmHg | 81.9±14.8 | 83.6±15.4 | 0.35 | 82.0 (64.0–101.0) | 84.5 (47.0–111.0) | 0.18 | 76.5 (56.0–131.0) | 80.5 (67.0–129.0) | 0.30 |
HR, bpm | 91.0±18.8 | 92.7±19.3 | 0.19 | 86.0 (72.0–126.0) | 84.0 (66.0–120.0) | 0.19 | 91.8±20.9 | 95.2±20.5 | 0.09 |
CVP, mmHg | 10.5±5.2 | 10.5±5.1 | 0.91 | 10.2±4.9 | 10.1±5.1 | 0.73 | 10.6±5.4 | 10.8±5.2 | 0.73 |
CI, L/min/m2 | 3.4±1.2 | 3.3±1.0 | 0.25 | 2.8 (1.7–6.1)* | 2.9 (1.8–5.5) | 0.55 | 3.6±1.2 | 3.5±1.0 | 0.28 |
DO2, ml/min/m2 | 345.6±117.8 | 392.5±121.9 | <0.01 | 296.3±87.8* | 341.9±92.8 | <0.01 | 375.1±124.7 | 422.9±128.4 | <0.01 |
VO2, ml/min/m2 | 116.5±41.9 | 122.1±38.5 | 0.23 | 107.5 (59.0–180.0) | 117.5 (54.0–198.0) | 0.048 | 119.2±48.8 | 123.0±42.8 | 0.58 |
O2ER, % | 35.9±12.2 | 33.0±11.0 | <0.01 | 39.7±10.6 | 36.6±10.1 | 0.02 | 33.7±12.8 | 30.9±11.0 | 0.05 |
Blood Lactate, mmol/L | 1.4 (0.7–5.6) | 1.7 (0.5–5.4) | 0.19 | 1.8 (0.7–5.1) | 1.8 (0.5–5.2) | 0.79 | 1.3 (0.7–5.6) | 1.5 (0.7–5.4) | 0.13 |
SaO2, % | 98.0 (91.8–99.9) | 97.7 (93.1–99.7) | 0.30 | 97.6 (91.8–99.2) | 97.8 (93.1–99.7) | 0.36 | 98.2±1.1 | 97.5±1.6 | 0.02 |
ScvO2 or SvO2, % | 63.3±12.4 | 66.0±11.2 | <0.01 | 58.9±10.3* | 62.6±10.5 | <0.01 | 65.9±12.9 | 68.0±11.3 | 0.11 |
Pv-aCO2, mmHg | 5.2±2.3 | 6.2±2.7 | 0.02 | 5.4±2.9 | 6.2±2.7 | 0.21 | 5.1±1.9 | 6.1±2.8 | 0.05 |
SBFBT, PU | 131.6±89.2 | 113.6±108.8 | 0.14 | 51.8 (4.3–279.3)* | 80.2 (9.8–561.6) | <0.01 | 161.6±81.7 | 101.8±76.4 | <0.01 |
SBF37, PU | 140.0 (11.2–444.6) | 122.2 (13.6–585.3) | 0.31 | 88.8 (11.2–285.9)* | 108.5 (15.1–585.3) | <0.01 | 178.4±86.5 | 123.5±73.4 | <0.01 |
ΔSBF/ΔT, PU/°C | 2.0 (–16.4–21.8) | 2.8 (–14.2–48.2) | 0.54 | 2.83 (–1.39–21.84) | 4.16 (–7.68–48.15) | 0.68 | 1.76 (–16.36–18.72) | 2.53 (–14.15–27.66) | 0.44 |
Note: Data are reported as mean (SD), or median (IQRs). Abbreviations: Hb, hemoglobin; MAP, mean arterial pressure; HR, heart rate; CVP, central venous pressure; CI, cardiac index; DO2, oxygen delivery; VO2, oxygen consumption; O2ER, oxygen extraction ratio; SaO2, arterial oxygen saturation; ScvO2 or SvO2, central or mixed venous oxygen saturation; Pv-aCO2, veno-arterial carbon dioxide difference; SBFBT, skin blood flow at basal temperature; SBF37, skin blood flow at 37°C; ΔSBF/ΔT= (SBF37- SBFBT/T37°C-T at baseline); PU, perfusion units; *p < 0.05 between SBF responders vs SBF non-responders.
Fig. 1
Correlation between the relative change in SBFBT and the relative change in systemic variables. Upper panel: correlation between the relative change in SBFBT and the relative change in CI (A), between the relative change in SBFBT and the relative change of MAP (B), and between the relative change in SBFBT and lactate (C) after RBCT. Lower panel: correlation between the relative change in SBFBT and the relative change in Pv-aCO2 (D), and the relative change in SBFBT and the relative change in DP (E) after RBCT.
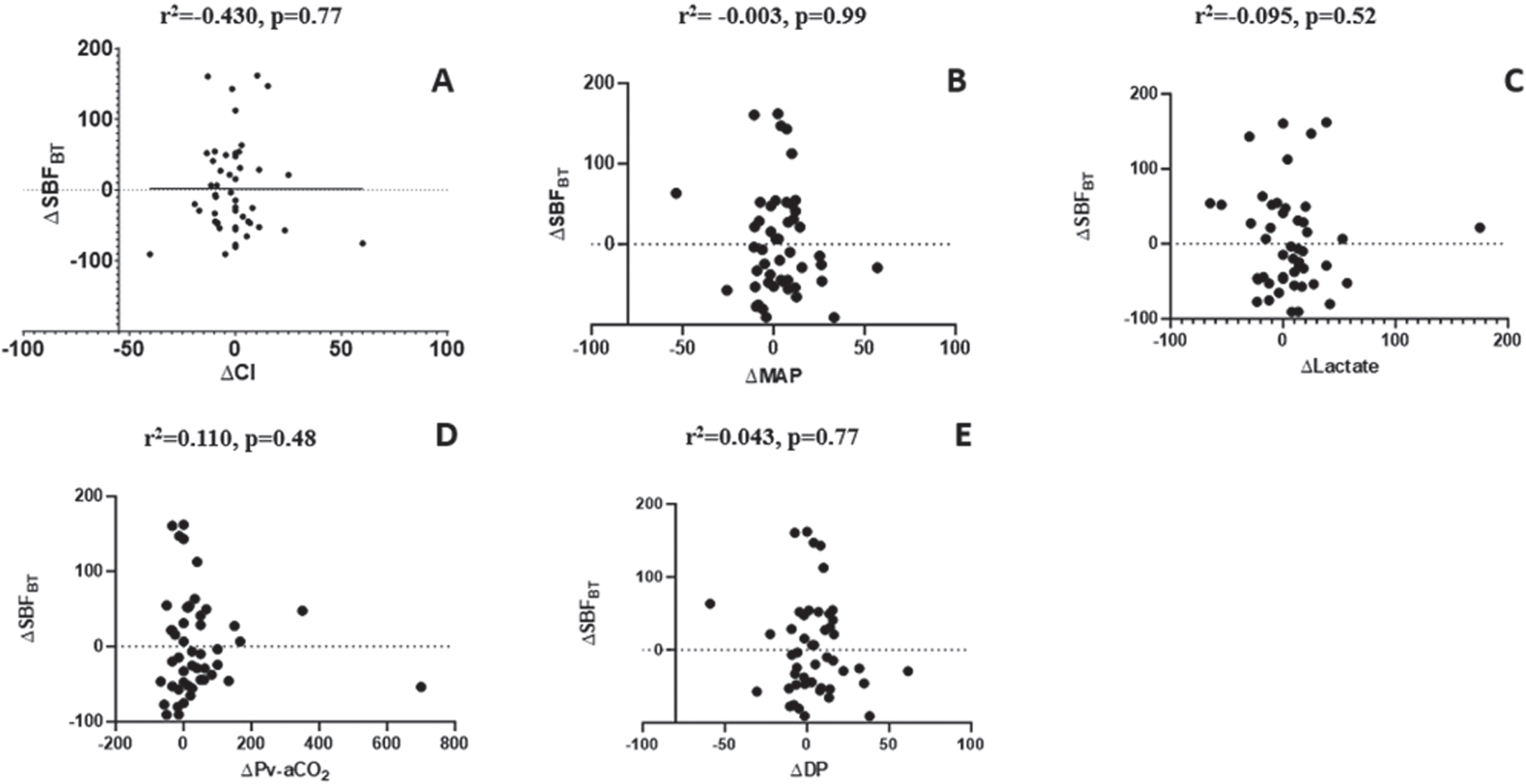
Subsequently, our analysis revealed an absence of a discernible correlation between modifications in skin tissue perfusion and shifts in systemic variables within the critically ill patient cohort following RBCT. Furthermore, it became apparent that the impact of RBCT on tissue perfusion exhibits considerable heterogeneity among this patient population and remains unpredictable based on presently available variables. This underscores the necessity for a more comprehensive patient evaluation, one that incorporates assessments of tissue perfusion, to guide the decision-making process regarding RBCT, in this clinical context. Indeed, it becomes evident that an increase in DO2 only yields a concurrent rise in VO2 among those individuals who exhibit a favorable response in terms of tissue perfusion, such as an improvement in microvascular flow. This observation suggests that cellular metabolism is contingent upon an adequate peripheral supply of oxygen. Skin tissue perfusion monitoring might help to individualize transfusion strategies in this setting.
Acknowledgments
The authors would like to thank Hassane Njimi for his help with the statistical analyses.
Author contributions
EC and FT conceived the study. EC selected the population and collected the data. EC and FT conducted the statistical analysis and wrote the first draft of the article. JC revised the text for intellectual content. All authors contributed to the article and approved the submitted version.
Availability of data and materials
Due to ethical restrictions, the datasets used and/or analyzed during the current study are available from the corresponding author upon reasonable request. Requests to access the datasets should be directed to Elaine Cavalcante dos Santos, .
Conflict of interest
The authors declare that the research was conducted in the absence of any commercial or financial relationships that could be constructed as a potential conflict of interest.
Consent for publication
Not applicable.
Ethics approval and consent to participate
The studies involving humans were approved by the Erasme Hospital Ethical Committee. The studies were conducted following the local legislation and institutional requirements. The participants provided their written informed consent to participate in this study.
Funding
None.
References
[1] | De Backer D , Creteur J , Dubois MJ , Sakr Y , Koch M , Verdant C , et al. The effects of dobutamine on microcirculatory alterations in patients with septic shock are independent of its systemic effects. Critical Care Medicine. (2006) ;34: (2):403–8. |
[2] | Mongkolpun W , Orbegozo D , Cordeiro CPR , Franco CJCS , Vincent J-L , Creteur J . Alterations in skin blood flow at the fingertip are related to mortality in patients with circulatory shock. Critical Care Medicine. (2020) ;48: (4):443–50. |
[3] | Mongkolpun W , Gardette M , Orbegozo D , Vincent JL , Creteur J . An increase in skin blood flow induced by fluid challenge is associated with an increase in oxygen consumption in patients with circulatory shock. J Crit Care. (2022) ;69: :153984. |
[4] | Khamiees M , Raju P , DeGirolamo A , Amoateng-Adjepong Y , Manthous CA . Predictors of extubation outcome in patients who have successfully completed a spontaneous breathing trial. Chest. (2001) ;120: (4):1262–70. |
[5] | Erkens R , Duse D , Wernly B , Flaatten H , Fjølner J , Bruno RR , et al. Associations of anemia and blood transfusions with mortality in old, critically ill COVID-19 patients – Results from the prospective COVIP study. Clinical Hemorheology and Microcirculation. (2023) ;84: (3):309–20. |
[6] | Semple JW , Rebetz J , Kapur R . Transfusion associated circulatory overload and transfusion related acute lung injury. Blood. (2019) ;133: :1840–1853. |
[7] | Lopes KG , Farinatti P , Bottino DA , de Souza M , Maranhão P , Bouskela E , et al. Exercise with blood flow restriction improves muscle strength and mass while preserving the vascular and microvascular function and structure of older adults. Clinical Hemorheology and Microcirculation. (2022) ;82: (1):13–26. |
[8] | Mrowietz C , Sievers H , Pindur G , Hiebl B , Jung F . Cutaneous microcirculation in patients with peripheral arterial occlusive disease: Comparison of capillary blood circulation in the nail fold of finger and toe. Clinical Hemorheology and Microcirculation. (2020) ;76: (2):279–85. |
[9] | Park JW , Matschke K , Mrowietz C , Krüger-Genge A , Jung F . HELP-(Heparin-induced Extracorporeal LDL Precipitation)-apheresis in heart recipients with cardiac allograft vasculopathy and concomitant hypercholesterolemia: Influence of long-term treatment on the microcirculation. Clinical Hemorheology and Microcirculation. (2019) ;73: (1):19–27. |
[10] | Jung F , Leithäuser B , Landgraf H , Jünger M , Franzeck U , Pries A , et al. Laser Doppler flux measurement for the assessment of cutaneous microcirculatio–critical remarks. Clinical Hemorheology and Microcirculation. (2013) ;55: (4):411–6. |
[11] | Monfort JB , Chasset F , Barbaud A , Frances C , Senet P . Nailfold capillaroscopy findings in cutaneous lupus erythematosus patients with or without digital lesions and comparison with dermatomyositis patients: A prospective study. Lupus. (2021) ;30: (8):1207–13. |
[12] | Sakr Y , Chierego M , Piagnerelli M , Verdant C , Dubois MJ , Koch M , et al. Microvascular response to red blood cell transfusion in patients with severe sepsis. Critical Care Medicine. (2007) ;35: (7):1639–44. |
[13] | Mongkolpun W , Bakos P , Vincent JL , Creteur J . Monitoring skin blood flow to rapidly identify alterations in tissue perfusion during fluid removal using continuous veno-venous hemofiltration in patients with circulatory shock. Annals of Intensive Care. (2021) ;11: (1):59. |
[14] | Cavalcante Dos Santos E , Bakos P , Orbegozo D , Creteur J , Vincent JL , Taccone FS . Transfusion increased skin blood flow when initially low in volume-resuscitated patients without acute bleeding. Frontiers in Medicine. (2023) ;10: :1218462. |