Can we predict critical care mortality with non-conventional inflammatory markers in SARS-CoV-2 infected patients?
Abstract
BACKGROUND:
Severe COVID-19 disease is associated with multiple organ involvement,then failure and often fatal outcomes.In addition,inflammatory mechanisms and cytokine storms,documented in many COVID-19 patients,are responsible for the progression of the disease and high mortality rates.Inflammatory parameters,such as procalcitonin(PCT) and C-reactive protein(CRP), are widely used in clinical practice.
OBJECTIVE:
To evaluate the predictive power of non-conventional inflammatory markers regarding mortality risk.
METHODS:
In our prospective study 52 patients were followed for 5 days after admission to an intensive care unit immediately with severe SARS-CoV-2 infection.We compared leukocyte-,platelet antisedimentation rate (LAR, PAR),neutrophil lymphocyte ratio(NLR), CRP, PCT levels.
RESULTS:
In non-surviving(NSU) patients LAR remained largely constant from D1 to D4 with a statistically significant drop(p < 0.05) only seen on D5.The NSU group showed statistically significant(p < 0.05) elevated LAR medians on D4 and D5, compared to the SU group.NLR values were continually higher in the non-survivor group.The difference between the SU and NSU groups were statistically significant on every examined day.PAR, CRP and PCT levels didn’t show any significant differences between the SU and NSU groups.
CONCLUSIONS:
In conclusion, this study suggests that LAR and NLR are especially worthy of further investigation as prognostic markers.LAR might be of particular relevance as it is not routinely obtained in current clinical practice.It would seem beneficial to include LAR in data sets to train prognostic artificial intelligence.
1Introduction
So far in the 21st century, three major outbreaks of respiratory disease associated with novel coronaviruses have been reported: SARS-CoV-1, MERS-CoV, and the most recent SARS-CoV-2, provisionally called 2019-nCoV [1].
Severe COVID-19 disease is associated with multiple organ involvement, then failure, and often fatal outcomes [2]. Viral entry to the host cell is possible with the spike (S) protein receptor binding domain of SARS-CoV-2, which binds to the angiotensin-converting enzyme 2 (ACE-2) receptors of the host cells [3]. ACE-2 receptors are expressed in the whole body leading to direct injury of multiple organs [3]. In addition, inflammatory mechanisms, and cytokine storms, documented in many COVID-19 patients, are responsible for the progression of the disease and high mortality rates [4–6].
Inflammatory parameters, such as procalcitonin (PCT) and C-reactive protein (CRP), are widely used in clinical practice. In viral infections interferon pathways suppress procalcitonin production, resulting in less increase in concentration compared to bacterial infections [7]. Multiple studies during the H1N1 influenza pandemic suggest that an elevating procalcitonin level in influenza pneumonia is a sign of bacterial co-infection [8]. However, Gautam et al. found that respiratory viral infection can stimulate the production of procalcitonin even without a bacterial co-infection and procalcitonin elevation correlates with the severity of the infection [9]. Similarly, higher levels of C-reactive protein were associated with severe disease outcomes and can be used as a biomarker to predict complications in H1N1 influenza patients [10].
As the common diagnostic procedures do not always allow sufficient risk stratification, Loibl et al. already looked at the kinetic of different parameters in various patient groups. The goal was to find a marker, threshold or trend associated with poor outcome. They observed that the lack of early thrombocyte and leukocyte activation can be indicative of likely complications in patients with severe burns. The prospective observational study determined both LAR and PAR to be predictive of septic complications and poor outcome [11]. Rozanovic et al. also looked at these parameters in a prospective observational study focusing on massive trauma as well as burn injury. In this study CRP and PCT were observed in addition to LAR. It was demonstrated that a drop in LAR and CRP can be sign of the onset of sepsis [12].
The observations are based on the assumption that an immune response is provoked in the studied cases (trauma, burns, infection and sepsis). The first step of cellular response is the activation of neutrophil granulocytes as part of the innate immune system. The cytopathic effects of SARS-CoV-2 can especially be seen in type two pneumocytes when the lung is affected. This results in apoptosis and increased chemotaxis of neutrophils to the site of inflammation [13]. Their activation results in strong adherence and chemotaxis to the interstitial space. Furthermore, production of free radicals is intensified. Phagocytosis is increased and the cells absorb more water. As a consequence, the specific weight is reduced and can be measured. Previous studies have observed sedimentation in anticoagulated whole blood samples. They found up to 10% more leukocytes in the top half of the column after one hour. This can be explained by the upwards floatation described as antisedimentation [14, 15]. Their activation is associated with a further absorption of water and thus also further reduction in their specific weight. As a result, the antisedimentation rate can be seen to increase to 15% [14, 15]. This is the leukocyte antisedimentation rate (LAR) and can be measured via gravitational sedimentation in whole blood samples [15–17].
Thrombocytes also play an important role in the immune system. They can migrate to sites of injury or inflammation and interact with pathogens found there. Through their adhesive properties they can bind pathogens that are subsequently removed by granulocytes [18]. In SARS-CoV-2 infections the role of thrombocytes is often associated with the development of a hypercoagulative state and thromboembolic events [19, 20]. This is supported by high D-dimer being a risk factor for poor outcome [2, 21, 22]. The strong inflammatory response originating from the alveoli triggers a cascade of inflammatory thrombosis in pulmonary vessels and later systemic micro and macro vascular thrombosis. Cytokine storm, complement activation and endothelial inflammation can be seen to initiate thrombosis [2, 23, 24]. The activation of thrombocytes in SARS-CoV-2 can also be measured as thrombocyte antisedimentation rate (PAR).
Neutrophil-lymphocyte ratio (NLR) is also an informative biomarker in infectious diseases such as influenza. As a response of the innate immune system to inflammation, neutrophilia and lymphocytopenia are induced. In hospitalized patients with influenza infection, severe lymphopenia is a common finding and is an indicator of poor outcomes [25]. Han et al. found that NLR has a higher sensitivity in influenza virus infection than neutrophil or lymphocyte count and proved useful in differentiating viral and bacterial infection [26]. NLR was also associated with 7- and 28-day mortality in adult ICU patients, however, it seemed inferior compared to CRP and procalcitonin in sensitivity and specificity to predict the outcome [27].
Markers of inflammation have been effectively used in risk stratifying and as mortality predictors in patients with inflammatory diseases and various types of viral respiratory infections. Herein, we aimed to evaluate the prognostic value of these parameters in COVID-19 infection. We included C-reactive protein, procalcitonin, and hematological biomarkers such as leucocyte antisedimentation rate, platelet antisedimentation rate and neutrophil-leucocyte ratio.
2Patients and methods
2.1Ethics approval and consent
This study was conducted in accordance with the Helsinki declaration, Hungarian law and good clinical practice. Ethics approval was provided by the ethics committee of the University of Pécs (8581 –PTE 2021). Written consent was given by all participants or their next of kin. The study was conducted in a 27-bed intensive care unit (ICU) at the university of Pécs between October 2020 and March 2021. All patients were treated in accordance with Hungarian and international guidelines [28]. All consecutive patient were screened for eligibility.
2.2Inclusion / exclusion criteria
Included were adult Patients admitted to intensive care in critical condition due to a PCR confirmed SARS-CoV-2 infection. Exclusion criteria were inability to obtain consent, haematological malignancies and chronic immunosuppressive therapy. Patients with NYHA IV heart failure, liver cirrhosis or portal hypertension or long-term dependence on haemodialysis were also excluded. Ten healthy volunteers were recruited as control group, which was matched in gender and age.
2.3Location and design
The initial blood sample was taken on the day of admittance to the ICU (D1). Further samples were obtained daily for the duration of ICU treatment. In simple events the pro- and anti-inflammatory response usually reaches its peak after five days, when it stabilizes or begins to drop again [29, 30]. Thus the assumption was made, that five days of monitoring would be sufficient. The healthy control group was subjected to a single blood test. Control group members were not infected with SARS-CoV-2 before their sample was taken.
2.4Laboratory analysis
The samples of arterial blood were drawn in sedimentation tubes containing sodium acetate (5.2 ml Sodium citrate 0.105 M; Vacutainer, Becton Dickinson, Meylan, France). Following one hour of sedimentation the leukocyte count was determined in the upper (U) and lower (L) half of the sample. The equation LAR = 100 x (U-L)/(U + L) was used to calculate the % LAR value. This describes the number of leucocytes that have risen above the midline during one hour of sedimentation relative to the initial number of leucocytes [12, 14]. PAR was measured in the same way. The number of thrombocytes rising above the midline during one hour of sedimentation was calculated using PAR = 100 x (U-L)/(U + L) [31].
NLR was determined by dividing the number of circulating neutrophil granulocytes by the number of circulating lymphocytes. Both counts were obtained from routine daily lab reports.
2.5Patient’s therapy
For all patients in this study, we have used our department’s (University of Pécs, Clinical Center, Department of Anaesthesiology and Intensive Therapy) standard COVID-19 patient therapy protocol, whose main points are the following:
– Preventive dose LMWH (enoxaparin) for all patients was administered.
– Dexamethasone (12 mg) was administered for all patients since their admission to the ICU.
– Preventive antibiotic therapy wasn’t administered.
– Remdesivir (200 mg loading dose at day 0., 100 mg in the next 4 days) was administered for all patients.
– No biological therapy was administered in our patients.
– No platelet aggregation inhibitor was administered preventively.
– There were no vaccinated patients against SARS CoV-2 infection.
2.6Statistical analysis
Statistical Package for the Social Sciences (SPSS), Version 22.0 (IBM Corporation, USA) was used for analysis of the assembled data. Values were given as median and interquartile range (IQR), as no normal distribution could be shown using the Kolmogorov-Smirnov-Test. Kruskal-Wallis-Test and Mann-Whitney-U-Test were run to investigate interday tendencies. Significant trends were detected using the Jonckheere-Terpstra-Test. For all cases p < 0,05 was assumed for statistical significance.
3Results
3.1Demographic data
52 ICU patients with SARS-CoV-2 infections were included in this study. The median age was 67.5 years (60–75.5). Median age for the 14 surviving patients (SU) was 63 years (57.5–68.75). For the 38 patients who died on ICU (NS) the median age was 70 years (60–77.25). Lethality was 73% in this population, but it does not correlate with the whole COVID-19 lethality on our clinic. The sample was comprised of 33 male and 19 female patients. No significant difference in lethality was found for the two groups.
3.2Changes in LAR levels (Fig. 1)
Fig. 1
The kinetics of leukocyte antisedimentation rate (LAR) levels. White columns: surviving patients; Dark columns: non-surviving patients; Chessboard sampled column: Control group. *: p < 0.05 between the SU and the Control Group, ***: p < 0.001 between the SU and the Control Group, ##: p < 0.01 between the NSU and the Control Group, ###: p < 0.001 between the NSU and the Control Group. ∧: < 0.05 between the SU and the NSU Group, ∧∧: < 0.01 between the SU and the NSU Group. Data are given as median in a 25–75% interquartile range and 5–95% confidence interval.
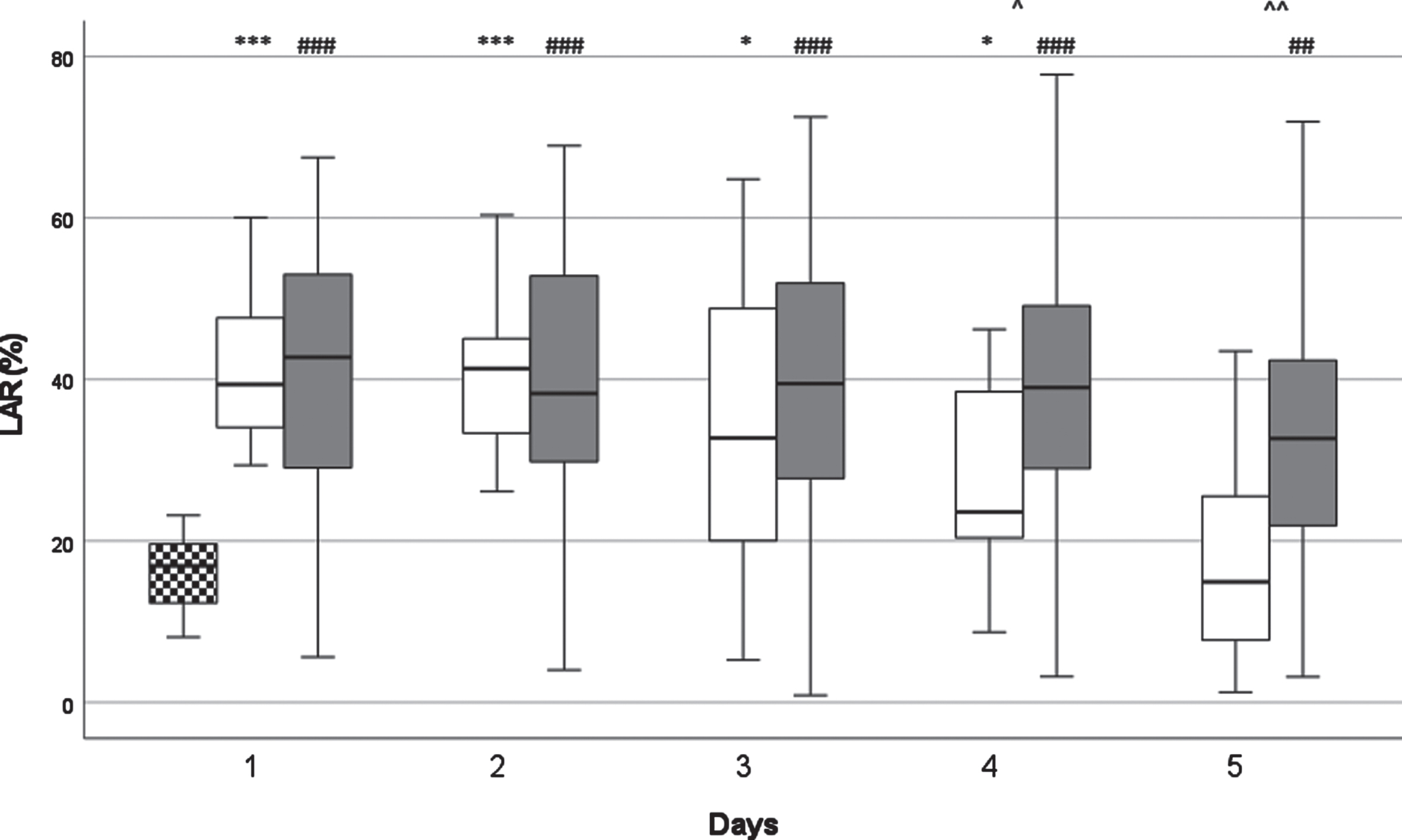
LAR decreased in both groups between D1 and D5. With the decrease being continuous in surviving (SU) patients and statistically significant on D4 (p < 0.05) and D5 (p < 0.01). In non-surviving (NSU) patients LAR remained largely constant from D1 to D4 with a statistically significant drop (p < 0.05) only seen on D5. The NSU group showed statistically significant (p < 0.05) elevated LAR medians on D4 and D5, compared to the SU group. There was a statistically significant and clear difference compared to the healthy control group on D1 and D2 (p < 0.001).
3.3Changes in PAR levels (Fig. 2)
Fig. 2
The kinetics of platelet antisedimentation rate (PAR) levels.White columns: surviving patients; Dark columns: non-surviving patients; Chessboard sampled column: Control group.***: p < 0.001 between the SU and the Control Group, ##: p < 0.01 between the NSU and the Control Group, ###: p < 0.001 between the NSU and the Control Group. Data are given as median in a 25–75% interquartile range and 5–95% confidence interval.
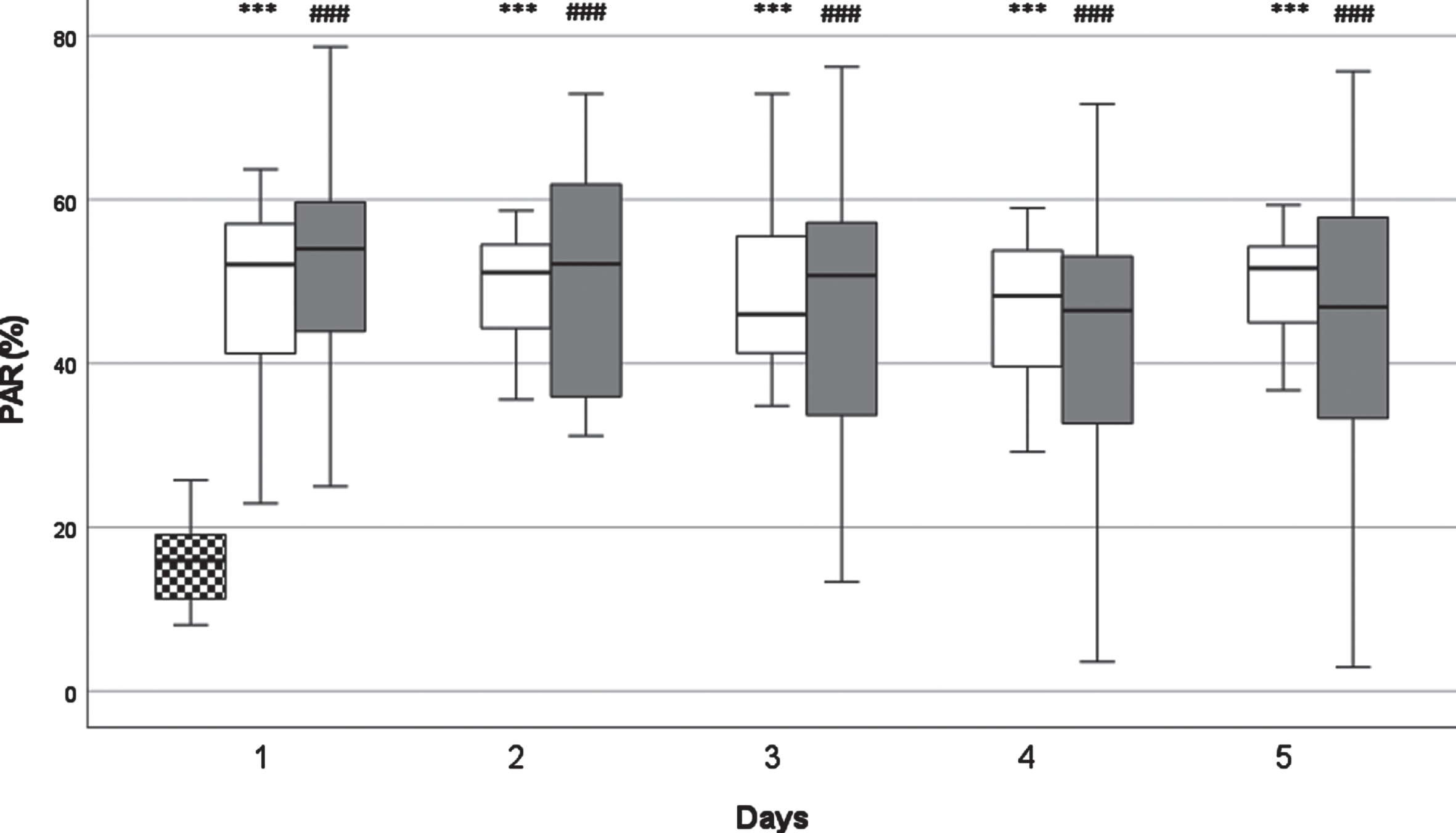
Median PAR values peaked on day one and showed a decreasing trend, but not reaching statistical significance. On D4 and D5 in SU group PAR levels showed an increasing tendency again, which was not registered in the NSU group. This decreasing trend was also characteristic for the non surviving group, except it did not start to increase on D4 and D5. An increase on day five could be observed, but was not statistically significant. In surviving patients there was a peak on day two. Overall, no statistically significant difference could be found between the two groups. There was statistically significant and clear difference compared to the healthy control group on every day in both groups (p < 0.001).
3.4Changes in NLR levels (Fig. 3)
Fig. 3
The kinetics of neutrophil-lymphocyte ratio (NLR) levels. White columns: surviving patients; Dark columns: non-surviving patients; Chessboard sampled column: Control group.***: p < 0.001 between the SU and the Control Group, ###: p < 0.001 between the NSU and the Control Group; ∧∧: < 0.01 between the SU and the NSU Group; ∧∧∧: < 0.001 between the SU and the NSU Group. Data are given as median in a 25–75% interquartile range and 5–95% confidence interval.
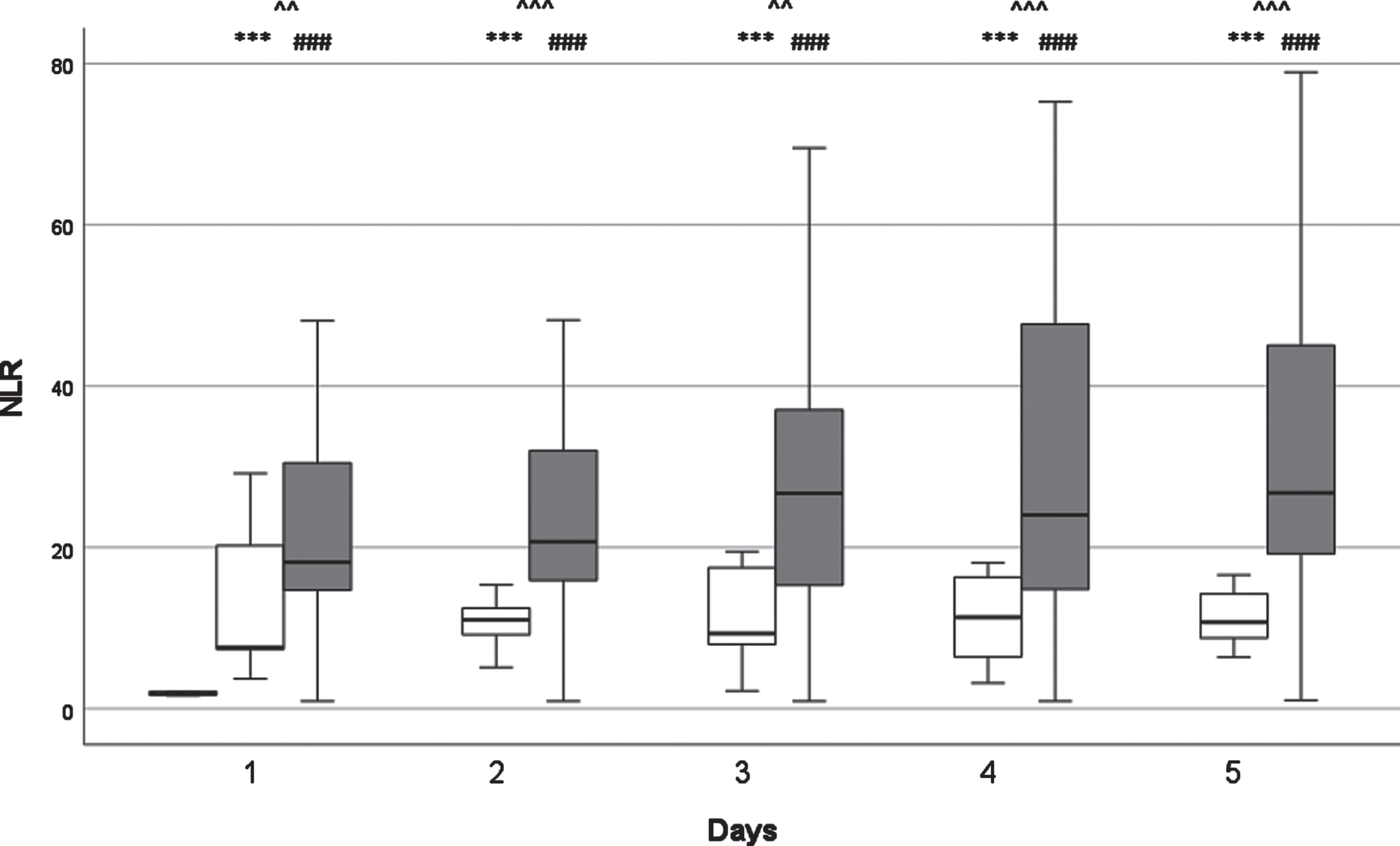
Thrombocyte numbers were within normal range at all times. No statistically significant differences to the control group were observed on day one.
NLR values were continuously higher in the non-survivor group. In the NSU group median NLR values rose until their peak at D3 before falling on the following days. The difference between the SU and NSU groups was statistically significant on every examined day (D1 and D3 p < 0.05; D2, D4 and D5 p < 0.001). There was statistically significant and clear difference compared to the healthy control group on every day in both groups (p < 0.001).
3.5Changes in CRP and PCT (Figs. 4 & 5)
Fig. 4
The kinetics of C-reactive protein (CRP) levels. White columns: surviving patients; Dark columns: non-surviving patients. Data are given as median in a 25–75% interquartile range and 5–95% confidence interval.
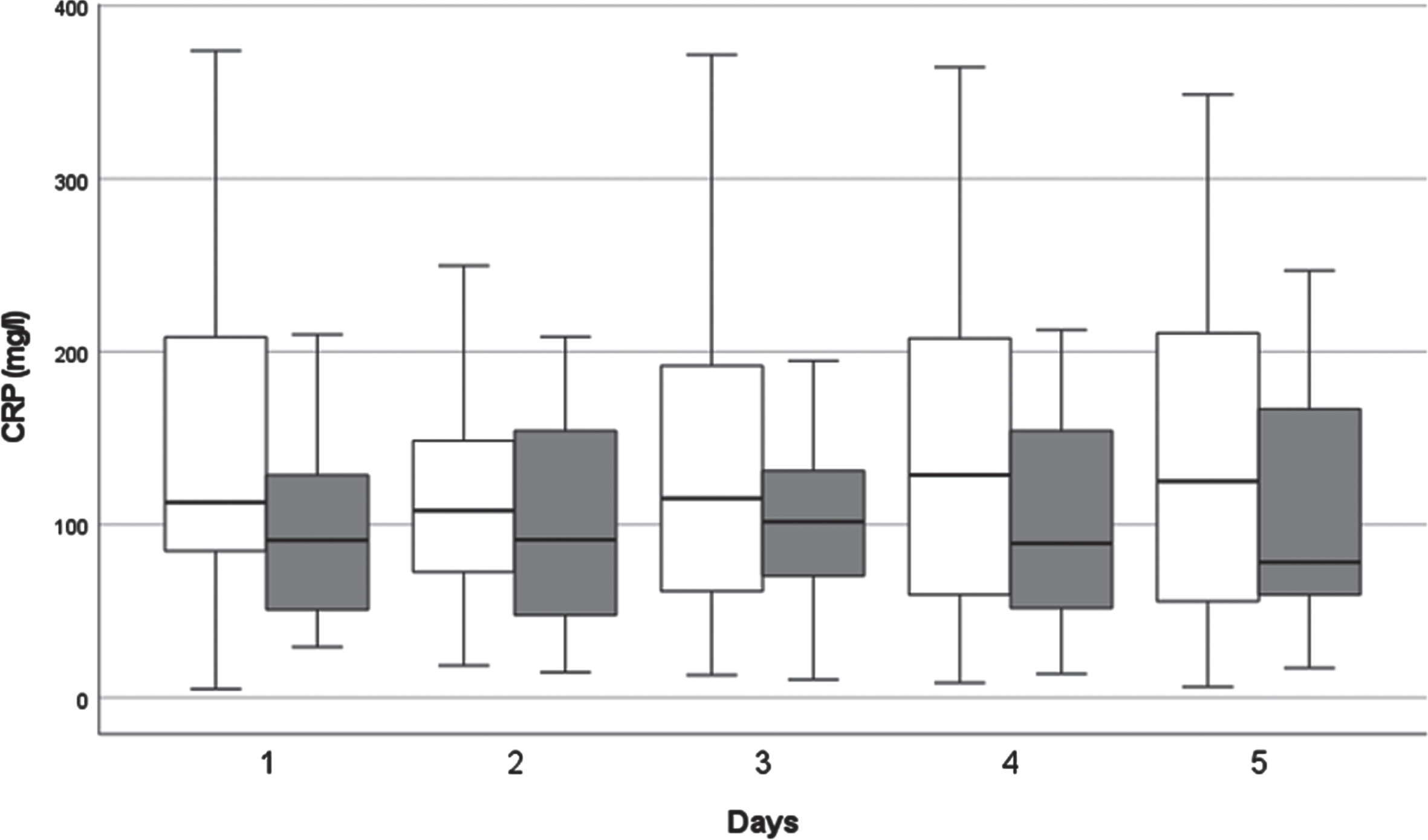
Fig. 5
The kinetics of procalcitonin (PCT) levels. White columns: surviving patients; Dark columns: non-surviving patients. ∧: < 0.05 between the SU and the NSU Group, Data are given as median in a 25–75% interquartile range and 5–95% confidence interval.
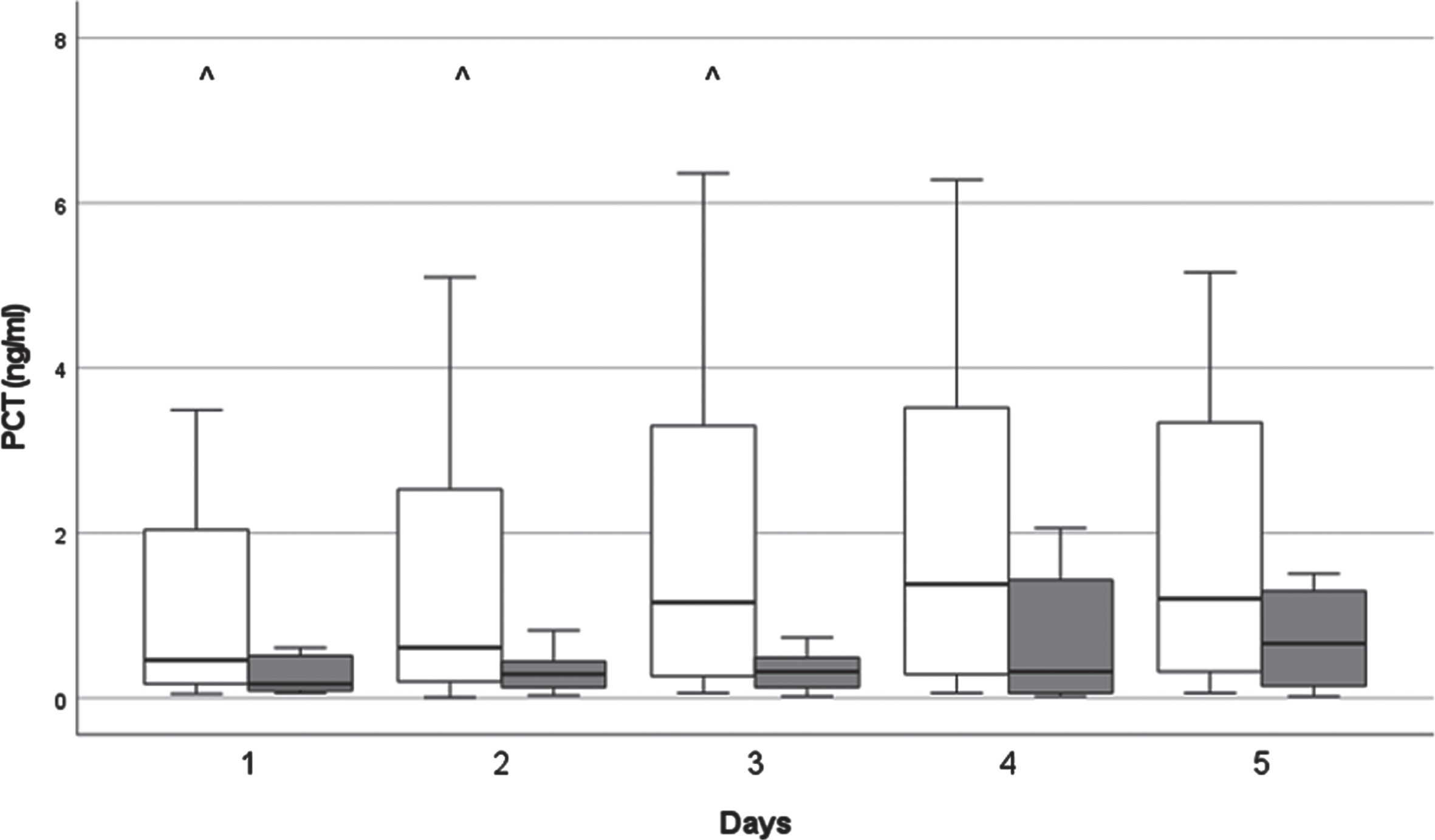
For both groups the CRP levels were elevated above the reference levels defined by the laboratory at all times.
PCT levels slightly rose above reference levels at D3 and after. In surviving patients, PCT level was significantly higher (p < 0.05) on D1-D3, than in non-survivor group.
4Discussion
LAR and PAR measurements are not routinely obtained in intensive care treatment. Thus, only a limited amount of literature exists regarding their kinetics. Previous studies have looked at LAR and PAR in various intensive care patients. However, to the best of our knowledge, this is the first study to focus specifically on these parameters in COVID-19 patients.
LAR has previously been described as an indicator for the activation of leukocytes [32–34]. A higher LAR percentage value has been shown to correspond with a higher number of activated leukocytes. In this study, we looked at LAR to investigate leukocyte activation in COVID-19 patients. Among patients who survived ICU treatment, an earlier decrease in LAR values could be observed (D4 in SU, D5 in NSU). Patients who survived showed significantly lower median LAR on days 4 and 5, compared to the NS group. These results suggest that a delayed drop in LAR and elevated leucocyte activation is associated with poor outcome.
Contrary to that, Loibl et al. showed LAR increase to be beneficial in patients with thermal injuries [32]. Rozanovic et al. also found an early increase in LAR to be associated with survival in patients after massive trauma and burn injury [12]. These studies suggested that reduced or delayed increase in LAR was associated with poor outcome as it demonstrated a less effective immune response.
Our previous study confirmed that increased LAR is primarily caused by reduced specific gravity of polymorphonuclear leukocytes [16]. This may be due to the degranulated state, as dense granules are the intracellular cell elements with the highest specific gravity. It can be assumed that the polymorphonuclear leukocytes of the NS group have reached a permanent degranulated state. In addition, it can also be assumed that the reduced specific gravity of these cells could have been caused by the significant water absorption and swelling accompanying their activation.
In addition to leukocyte activation, the number of circulating cells was also studied. We found elevated leukocyte counts in all COVID-19 patients. A significant difference to the control group was seen on D1. This suggests increased leukocytes to be a general aspect of SARS-CoV-2 infection. Furthermore, we observed significantly lower leukocyte levels on D2 and D5 in surviving patients. This indicates that more severe leukocytosis can be associated with less favourable outcomes. As early as January 2020 Zhou et al. investigated this in a retrospective cohort study with 191 patients in Wuhan. They were able to correlate elevated leukocyte levels with increased mortality [21]. Leukocytosis was also found in further studies, especially in patients that developed severe symptoms and needed intensive care treatment [2]. Leukocytosis can be an indicator of bacterial superinfection, that occurs due to the incurred lung damage [35].
In patients with SARS-CoV-2 infection, platelet count alterations are a common finding [36]. Low platelet count appears as a result of apoptosis, and their incorporation into microthrombi, partly due to hyperactivation. This hyperactivation of thrombocytes is induced by inflammatory factors and direct SARS-CoV-2 interactions as well [37]. PAR was included in this study as we assumed it to be a good indicator of thrombocyte activation. Rozanovic et al. have previously shown that high PAR is associated with survival in patients with severe burn injuries [32]. In the current study we found that PAR dropped from its peak one day earlier in the non-surviving patient group (D1 for NSU, D2 for SU). However, a significant difference could not be detected between the two groups.
PAR places focus on thrombocyte activation. In addition to that we also documented the number of thrombocytes in circulation. Thrombocyte levels increased continuously in surviving patients and remained above those of non-surviving patients throughout. These results suggest that reduced levels are indicative of poor outcome. They are also in line with previous studies that have shown thrombocyte levels to be of prognostic value [21].
In COVID-19 hemostasis is regularly affected. Patients have been shown to develop systemic hypercoagulopathy and resulting thromboembolic events [38]. An elevated D-dimer was found to be a common phenomenon and associated with elevated mortality. Higher incidence of deep vein thrombosis is observed in patients with a severe course of the disease. Hypercoagulopathy was also seen in previous infections with corona viruses SARS-CoV and MERS-CoV [38]. It appears as though the virus does not cause coagulopathy directly. Rather, coagulopathy is a result of the inflammatory response and endothelial damage.
Typically, severe COVID-19 cases show mild thrombocytopenia with elevated platelet consumption, thus increased compensatory neogenesis seems likely [38]. This suggests that a sudden drop in thrombocyte levels results in a poor thrombotic state. In this study we found no statistically significant trends in non-surviving patients. We did however see a drop after D3 following an initial increase. This is contrary to the surviving group which increased throughout. A poor thrombotic state likely results in more frequent macro and micro thromboembolic events [39]. Unfortunately, we have no data regarding thromboembolic complications in the studied patient groups.
SARS-CoV-2 infection also impacts the lympho-hematopoietic system in further ways. The changes in neutrophil granulocytes and lymphocytes can be described by the neutrophil-lymphocyte-ratio (NLR). We were able to demonstrate that NLR showed significantly higher values on D1, D4 and D5 for patients who did not survive.
These observations are in line with numerous previous studies, which identified high NLR as a marker for a severe course of COVID-19 and poor outcome [40, 41]. An increase in NLR can be explained by either an increase in circulating neutrophil granulocytes or reduced number of circulating lymphocytes. Neutrophil granulocytes migrate to the site of infection as part of the cellular response by the innate immune system. They are attracted by chemotactic substances produced by infected type two pneumocytes. Due to this, it can be assumed that strong manifestation in the lung goes along with elevated neutrophil levels. Significantly higher neutrophil counts are observed in COVID-19 patients on intensive care, compared to those on peripheral units [2]. Additionally, a reduced lymphocyte count was also identified as a negative prognostic marker [21]. Lymphocytes and their subgroups are the cellular effectors of the adaptive immune system. Both causes of NLR rise described above are associated with poor outcome and are primarily seen in patients developing ARDS [42].
In the pathophysiology of SARS-Cov-2 infection an aggressive inflammatory response takes place, where the severity of the disease depends not only on pathogenicity of the virus, but also on host response. In case of a dysfunctional immune response, the accumulation of immune cells, subsequent overproduction of cytokines presents, circulating in the organism and resulting in multi-organ damage [43]. Therefore, monitoring inflammatory markers may offer relevant information on disease prognosis.
CRP is an unspecific acute phase protein and functions as biomarker for inflammation, infection and tissue damage. It can already be elevated in early phases of SARS-CoV-2 infection [44]. In this study we found CRP to be elevated significantly above reference levels on day one. No significant trends or differences between the groups could be observed. Thus, we do not see CRP to be of prognostic value for COVID-19 patients based on this study. However, a systematic review of 61 studies reported an association between high levels of CRP and COVID-19 severity [45]. Nevertheless, some inconsistency in CRP cut-off values for risk stratification may be observed among different studies [46].
Another inflammatory marker examined in our study is procalcitonin (PCT). Typically, increased PCT can be observed in bacterial but not viral infections. It is consequently used to differentiate the cause of infection. An increase during the course of a viral infection can be indicative of bacterial superinfection. Serum PCT levels showed no prognostic value in this study. Neither trends nor differences between surviving and non-surviving groups were found. Similarly, Gupta et al. found no correlation between procalcitonin and mortality in COVID-19 patients admitted to intensive care units, explained by a better prevention of secondary infections [47].
The main limitation of this study was the low patient number. It is not known which of the comorbidities and chronic diseases they might have had impacted the outcome. Platelet aggregometry had not been performed in these patients, which would have been important related to the deeper PAR analysis.
In conclusion, this study suggests that LAR, leukocyte count and thrombocyte levels and NLR are especially worthy of further investigation as prognostic markers. LAR might be of particular relevance as it is not routinely obtained in current clinical practice. While LAR alone is likely not sufficient to guide individual patient treatment, it might play an important role as part of a prognostic tool looking at various parameters. It would seem beneficial to include LAR in data sets to train prognostic artificial intelligence.
Acknowledgments
We wish to thank for the cooperation of the University of Pécs provided by the following units: Department of Anesthesiology and Intensive Care, Department of Laboratory Medicine, Department of Public Health Medicine, Department of Neurology.
Our study was supported by “Thematic excellence program- NVA- 06” Establishment of the Center of Excellence in Defense Health at the University of Pécs Medical School.
Conflict of interest
The authors have read the journal’s policy and declare the following: no potential conflict of interest relevant to this article can be reported.
References
[1] | Gorbalenya AE , Baker SC , Baric RS , de Groot RJ , Drosten C , Gulyaeva AA , et al. The species Severe acute respiratory syndrome-related coronavirus: classifying -nCoV and naming it SARS-CoV-2, Nat Microbiol (2020) ;5: :536–44. https://doi.org/10.1038/s41564-020-0695-z. |
[2] | Huang CL , Wang Y , Li X , Ren L , Zhao J Clinical features of patients infected with novel coronavirus in Wuhan, China, Lancet (2020) ;395: :497–506. |
[3] | Li ren S , Tang jian Z , Li han Z , Liu X Searching therapeutic strategy of new coronavirus pneumonia from angiotensin-converting enzyme the target of COVID-19 and SARS-CoV, Eur J Clin Microbiol Infect Dis (2020) ;39: :1021–6 https://doi.org/10.1007/s10096-020-03883-y. |
[4] | Ye Q , Wang B , Mao J The pathogenesis and treatment of the ‘Cytokine Storm’ in COVID-19, J Infect (2020) ;80: :607–13. https://doi.org/10.1016/j.jinf.2020.03.037. |
[5] | Zlojutro B , Jandric M , Momcicevic D , Dragic S , Kovacevic T , Djajic V , et al.Dynamic changes in coagulation, hematological and biochemical parameters as predictors of mortality in critically ill COVID-19 patients: A prospective observational study. Clin Hemorheol Microcirc. 2022:1-12. https://doi.org/10.3233/CH-221583. |
[6] | Binneboessel S , Bruno RR , Wernly B , Masyuk M , Flaatten H , Fjølner J , et al. Cytokine absorption in critically ill old COVID-19 patients with renal failure: A retrospective analysis of 503 intensive care unit patients. Clin Hemorheol Microcirc. 2022:1-9. https://doi.org/10.3233/CH-221579. |
[7] | Linscheid P , Seboek D , Nylen ES , Langer I , Schlatter M , Becker KL , et al. In Vitro and in Vivo Calcitonin I Gene Expression in Parenchymal Cells: A Novel Product of Human Adipose Tissue, Endocrinology (2003) ;144: :5578–84. https://doi.org/10.1210/en.2003-0854. |
[8] | Canavaggio P , Boutolleau D , Goulet H , Riou B , Hausfater P Procalcitonin for clinical decisions on influenza-like illness in emergency department during influenza a(H1N1)pandemic, Biomarkers (2018) ;23: :10–3. https://doi.org/10.1080/1354750X.2016.1276626. |
[9] | Gautam S , Cohen AJ , Stahl Y , Valda Toro P , Young GM , Datta R , et al. Severe respiratory viral infection induces procalcitonin in the absence of bacterial pneumonia, Thorax (2020) ;75: :974–81. https://doi.org/10.1136/thoraxjnl-2020-214896. |
[10] | Vasileva D , Badawi A C-reactive protein as a biomarker of severe H1N1 influenza, Inflamm Res (2019) ;68: :39–46. https://doi.org/10.1007/s00011-018-1188-x. |
[11] | Loibl C , Rozanovic M , Bogár L , Pankaczi A , Kovács P , Miseta A , et al. Lack of early platelet and leukocyte activation canindicate complications after major burn injury, Clin HemorheolMicrocirc (2021) ;77: :17–26. https://doi.org/10.3233/CH-190779. |
[12] | Rozanovic M , Csontos C , Bogár L , Szélig L , Bocskai T , Kovács P , et al. Can leukocyte antisedimentation rate (LAR)predict septic complications and critical care survival early inpolytrauma and burn victims? Clin Hemorheol Microcirc (2017) ;64: :875–85. https://doi.org/10.3233/CH-168024. |
[13] | Coperchini F , Chiovato L , Croce L , Magri F , Rotondi M The cytokine storm in COVID- An overview of the involvement of the chemokine/chemokine-receptor system, Cytokine Growth Factor Rev (2020) ;53: :25–32. https://doi.org/10.1016/j.cytogfr.2020.05.003. |
[14] | Bogar L , Tarsoly P , Jakso P Characteristics of light and heavy polymorphonuclear leukocytes, Clin Hemorheol Microcirc (2002) ;27: :149–53. |
[15] | Bogar L Sedimentation characteristics of leucocytes can predict bacteraemia in critical care patients, J Clin Pathol (2006) ;59: :523–5. https://doi.org/10.1136/jc2005.033035. |
[16] | Bogar L , Tarsoly P Gravity sedimentation of leukocytes is partially independent from erythrocyte sedimentation, Clin Hemorheol Microcirc (2006) ;34: :439–45. |
[17] | Bogar L , Molnar Z , Tarsoly P , Kenyeres P , Marton S Serum procalcitonin level and leukocyte antisedimentation rate as early predictors of respiratory dysfunction after oesophageal tumour resection, Crit Care (2006) ;10: :R110. https://doi.org/10.1186/cc4992. |
[18] | Gaertner F , Ahmad Z , Rosenberger G , Fan S , Nicolai L , Busch B , et al. Migrating Platelets Are Mechano-scavengers that Collect and Bundle Bacteria, Cell (2017) ;171: :1382–1382.e23. https://doi.org/10.1016/j.cell.2017.11.001. |
[19] | Rasyid A , Timan IS , Riyanto DL , Harris S , Kurniawan M , Mesiano T , et al. Coagulation and hemorheology profile of patient with stroke and COVID- A case series during second wave pandemic, Clin Hemorheol Microcirc (2022) ;82: :249–54. https://doi.org/10.3233/CH-221504. |
[20] | Xing Y , Yang W , Jin Y , Wang C , Guan X D-dimer daily continuous tendency predicts the short-term prognosis for COVID-19 independently: A retrospective study from Northeast China, Clin Hemorheol Microcirc (2021) ;79: :269–77. https://doi.org/10.3233/CH-201071. |
[21] | Zhou F , Yu T , Du R , Fan G , Liu Y , Liu Z , et al. Clinical course and risk factors for mortality of adult inpatients with COVID-19 in Wuhan, China: a retrospective cohort study, Lancet (2020) ;395: :1054–62. https://doi.org/10.1016/S0140-6736(20)30566-3. |
[22] | Tang N , Li D , Wang X , Sun Z Abnormal coagulation parameters are associated with poor prognosis in patients with novel coronavirus pneumonia, J Thromb Haemost7 (2020) ;18: :844–7. https://doi.org/10.1111/jth.14768. |
[23] | Varga Z , Flammer AJ , Steiger P , Haberecker M , Andermatt R , Zinkernagel AS , et al. Endothelial cell infection and endotheliitis in COVID-19, Lancet (2020) ;395: :1417–8. https://doi.org/10.1016/S0140-6736(20)30937-5. |
[24] | Kalbhenn J , Pooth J-S , Trummer G , Kranzhöfer D , Schlagenhauf A , Zieger B Pervasive Platelet Secretion Defects in Patients with Severe Acute Respiratory Syndrome Coronavirus 2 (SARS-CoV-2), Cells (2023) ;12: :193. https://doi.org/10.3390/cells12010193. |
[25] | Lalueza A , Folgueira D , Díaz-Pedroche C , Hernández-Jiménez P , Ayuso B , Castillo C , et al. Severe lymphopenia in hospitalized patients with influenza virus infection as a marker of a poor outcome, Infect Dis (Auckl) (2019) ;51: :543–6. https://doi.org/10.1080/23744235.2019.1598572 |
[26] | Han Q , Wen X , Wang L , Han X , Shen Y , Cao J , et al. Role of hematological parameters in the diagnosis of influenza virus infection in patients with respiratory tract infection symptoms, J Clin Lab Anal (2020) ;34: :1–7. https://doi.org/10.1002/jcla.23191. |
[27] | Zhou T , Zheng N , Li X , Zhu D , Han Y Prognostic value of neutrophil- lymphocyte count ratio (NLCR) among adult ICU patients in comparison to APACHE II score and conventional inflammatory markers: a multi center retrospective cohort study, BMC Emerg Med (2021) ;21: :1–10. https://doi.org/10.1186/s12873-021-00418-2. |
[28] | Bobek I , Elek J , Gopcsa L , Lakatos B , Madurka IE , Biró K , et al. Handbook for Prevention and Therapy in identificated Coronavirus (SARS-CoV-2) infections (COVID-19), 2020. [original: évben azonosított új koronavírus (SARS-CoV-2) okozta fertőzések (COVID-19) megelőzésének és terápiájának kézikönyve] |
[29] | Lederer JA , Rodrick ML , Mannick JA The effects of injury on the adaptive immune response, Shock (1999) ;11: :153–9. https://doi.org/10.1097/00024382-199903000-00001. |
[30] | Pham TN , Cancio LC , Gibran NS , American Burn Association American Burn Association practice guidelines burn shock resuscitation, J Burn Care Res (2008) ;29: :257–66. https://doi.org/10.1097/BCR.0b013e31815f3876]. |
[31] | Ezer E , Schrick D , Tokés-Füzesi M , Szapary L , Bogar L , Molnar T A novel approach of platelet function test for predictionof attenuated response to clopidogrel, Clin Hemorheol Microcirc (2019) ;73: :359–69. https://doi.org/10.3233/CH-190580. |
[32] | Loibl C , Rozanovic M , Bogár L , Pankaczi A , Kovács P , Miseta A , et al. Lack of early platelet and leukocyte activation canindicate complications after major burn injury, Clin HemorheolMicrocirc (2021) ;77: :17–26. https://doi.org/10.3233/CH-190779. |
[33] | Karim SSA , Karim QA Omicron SARS-CoV-2 variant: a new chapter in the COVID-19 pandemic, Lancet (2021) ;398: :2126–8. https://doi.org/10.1016/S0140-6736(21)02758-6. |
[34] | Gómez CE , Perdiguero B , Esteban M Emerging SARS-CoV-2 Variants and Impact in Global Vaccination Programs against SARS-CoV-2/COVID-19, Vaccine (2021) ;9: :243. https://doi.org/10.3390/vaccines9030243. |
[35] | Chen N , Zhou M , Dong X , Qu J , Gong F , Han Y , et al. Epidemiological and clinical characteristics of 99 cases of novel coronavirus pneumonia in Wuhan, China: a descriptive study, Lancet (2020) ;395: :507–13. https://doi.org/10.1016/S0140-6736(20)30211-7. |
[36] | Behnood Bikdeli , Mahesh Madhavan V , David Jimenez , Taylor Chuich , Isaac Dreyfus , Elissa Driggin , Caroline Der Nigoghossian WA COVID-19 and Thrombotic or Thromboembolic Disease: Implications for Prevention, Antithrombotic Therapy, and Follow-Up. J Am Coll Cardiol. 2020:2950-73. https://doi.org/10.1016/j.jacc.2020.04.03. |
[37] | Rohlfing A-K , Rath D , Geisler T , Gawaz M Platelets and COVID-19, Hamostaseologie (2021) ;41: :379–85. https://doi.org/10.1055/a-1581-4355. |
[38] | Wool GD , Miller JL The Impact of COVID-19 Disease on Platelets and Coagulation, Pathobiology (2021) ;88: :15–27. https://doi.org/10.1159/000512007. |
[39] | Shaik A , Chen Q , Mar P , Kim H , Mejia P , Pacheco H , et al. Blood hyperviscosity in acute and recent COVID-19 infection, Clin Hemorheol Microcirc (2022) ;82: :149–55. https://doi.org/10.3233/CH-221429. |
[40] | Yang A-P , Liu J , Tao W , Li H The diagnostic and predictive role of NLR, d-NLR and PLR in COVID-19 patients, Int Immunopharmacol.04 (2020) ;84: :106504. https://doi.org/10.1016/j.intim2020.106504. |
[41] | Liu J , Liu Y , Xiang P , Pu L , Xiong H , Li C , et al. Neutrophil-to-lymphocyte ratio predicts critical illness patients with coronavirus disease in the early stage, J Transl Med (2020) ;18: :206. https://doi.org/10.1186/s12967-020-02374-0. |
[42] | Wu C , Chen X , Cai Y , Xia J , Zhou X , Xu S , et al. Risk Factors Associated With Acute Respiratory Distress Syndrome and Death in Patients With Coronavirus Disease Pneumonia in Wuhan, China, JAMA Intern Med (2020) ;180: :934. https://doi.org/10.1001/jamainternmed.2020.0994. |
[43] | Tay MZ , Poh CM , Rénia L , MacAry PA , Ng LFP The trinity ofCOVID- immunity, inflammation and intervention, Nat Rev Immunol (2020) ;20: :363–74. https://doi.org/10.1038/s41577-020-0311-8. |
[44] | Wang L C-reactive protein levels in the early stage of COVID-19, Médecine Mal Infect (2020) ;50: :332–4. https://doi.org/10.1016/j.medmal.2020.03.007. |
[45] | Ikeagwulonu RC , Ugwu NI , Ezeonu CT , Ikeagwulonu ZC , Uro-Chukwu HC , Asiegbu U V , et al. C-Reactive Protein and Covid-19 Severity: A Systematic Review, West Afr J Med (2021) ;38: :1011–23. |
[46] | Prasetya IB , Cucunawangsih , Lorens JO , Sungono V , El-Khobar KE , Wijaya RS Prognostic value of inflammatory markers in patients with COVID-19 in Indonesia, Clin Epidemiol Glob Heal (2021) ;11: :100803. https://doi.org/10.1016/j.cegh.2021.100803. |
[47] | Gupta D , Jain A , Chauhan M , Dewan S Retrospective Observational Analysis of Inflammatory Markers in COVID-19 Patients Admitted to Intensive Care Unit: As Early Predictors of Disease Severity, Indian J Crit Care Med (2022) ;26: :482–6. https://doi.org/10.5005/jp-journals-10071-24171. |