Multimodal ultrasound imaging with conventional B-mode, elastography, and parametric analysis of contrast-enhanced ultrasound (CEUS): A novel approach to assess small bowel manifestation in severe COVID-19 disease
Abstract
The aim was to describe the small bowel morphology with conventional B-mode and elastography and additionally to evaluate dynamic effects of COVID-19 associated small bowel microvascularization using CEUS with color coded perfusion parameters.
Thirteen patients with severe COVID-19 acute respiratory distress syndrome (ARDS) were investigated. 13 patients required intensive care treatment with mechanical ventilation. Five patients required extracorporeal membrane oxygenation (ECMO). Contrast-enhanced ultrasound (CEUS) was performed by an experienced investigator as a bolus injection of up to 2.4 ml sulfur hexafluoride microbubbles via a central venous catheter. In the parametric analysis of CEUS, the flare of microbubbles over time is visualized with colors. This is the first work using parametric analysis of CEUS to detect perfusion differences in the small bowel.
Parametric analysis of CEUS in the intestinal phase was carried out, using DICOM loops for 20 seconds. In 5 patients, parametric analysis revealed intraindividual differences in contrast agent behavior in the small bowel region. Analogous to the computed tomography (CT) images parametric analysis showed regions of simultaneous hyper- and hypoperfusion of the small intestine in a subgroup of patients. In 5 patients, the parametric image of transmural global contrast enhancement was visualized.
Our results using CEUS to investigate small bowel affection in COVID-19 suggest that in severe COVID-19 ARDS systemic inflammation and concomitant micro embolisms may lead to disruption of the epithelial barrier of the small intestine.
This is the first study using parametric analysis of CEUS to evaluate the extent of small bowel involvement in severe COVID-19 disease and to detect microemboli. In summary, we show that in COVID-19 the small bowel may also be an important interaction site. This is in line with the fact that enterocytes have been shown to a plenitude of angiotensin converting enzyme (ACE)-2 receptors as entry sites of the virus.
1Introduction
SARS-CoV-2 infections varies from asymptomatic to life-threatening phenotypes [1]. Many risk factors have been identified in the progression of COVID-19 infection into a severe and critical stage, including underlying comorbidities such as hypertension, diabetes, obesity, chronic lung diseases, heart, liver and kidney diseases and clinically apparent immunodeficiencies and local immunodeficiencies [2]. With growing evidence, SARS-CoV-2 infection is being conceptualized as a systemic disease [3, 4]. Current literature suggests that the SARS-CoV-2 virus can affect different organ systems and causes dysregulation of the lungs, kidneys, liver, biliary tract, pancreas and the gastrointestinal system [5]. Adults as well as up to 50% children display gastrointestinal (GI) symptoms, which include vomiting, diarrhea, nausea and abdominal pain [6]. Microbiome alterations and disruption of the epithelial intestinal barrier are mutually dependent on each other during severe COVID-19 disease [7, 8]. Microbiome alterations are also suspected to have a crucial contribution to the development of Long-Covid-syndrome [9, 10].
Pathophysiological mechanisms of COVID-19 infection involve immunological, vascular and prothrombotic factors [11–13]. Induction of a cytokine storm leads to endothelial damage and thrombosis, accompanied by remodeling of the vascular tissue and resulting hypoperfusion [14–16]. In the big picture, the complete vascular system is affected by dysregulation in the context of COVID-19 infection. In the pathophysiology of severe COVID-19 disease, a combination of hyper perfusion in the context of a cytokine storm and hypo perfusion in the context of micro emboli is also present in the small intestine.
In the study presented here, we investigate the impact of SARS-CoV-2 infection on the small bowel using state-of-the-art advanced ultrasound methods including parametric analysis. To date, no work has been published with the use of contrast-enhanced ultrasound to evaluate perfusion of the small intestine. With regard to intestinal diagnostics CEUS is of particular importance in case of diagnosis and follow-up of ulcerative colitis, Crohn’s disease and for the detection of permeability disorders in Graft versus Host disease (GVHD) [17–19].
Microbubbles are gas bubbles smaller than red blood cells. They are the basis of CEUS. A higher density of the gas sulfur hexafluoride causes them to remain in the bloodstream longer due to their slow diffusion through the capsule membrane and their lower solubility in the blood, which increases the stability of the microbubbles over a longer period of time. The capsule or shell of the microbubbles contributes to their stability and resonance properties [20–23]. Since CEUS can image micro vascularization to the capillary level using modern 2nd generation ultrasound contrast agents, it is also crucial for the assessment of pathologic organ alterations and -perfusion during the course of COVID-19 disease.
The parametric analysis of CEUS constitutes an advanced visual evaluation of intestinal perfusion. In parametric analysis of CEUS, the flooding of microbubbles over time is visualized with colors. The temporal, color-coded, enhancement behavior is generated by the software of the ultrasound machine and is therefore not subject to fluctuations. The automated parametric analysis performed by the software is therefore not subject to operator-dependent variations. Parametric analysis allows the detection of specific perfusion deficits in the small intestine and is therefore a particularly suitable examination method for the evaluation of the microcirculation of the small intestine in severe COVID-19 disease.
2Materials and methods
2.1Patients
We studied thirteen patients with severe SARS-CoV-2 infection and COVID-19 ARDS (Tables 1 and 2). Twelve patients were mechanically ventilated, and five patients received extracorporeal membrane oxygenation therapy (ECMO). Based on the clinical performance status of the patients in the ICU, intestinal barrier dysfunction was suspected. Modern ultrasound including strain elastography, CEUS, and parametric analysis was performed. The study was approved by the local ethics committee of the University of Regensburg, Regensburg, Germany (Reg.-No.: 21-2693-104). In all cases there was written informed consents for contrast enhanced imaging modalities.
Table 1
Presents the demographic and clinical data of the patients included in the study. The study included 13 patients with a median age of 54 years. Eight male and five female patients were included in the study. Eight patients suffered from obesity at different levels. 85% of the severely ill patients were not vaccinated for SARS-CoV-2. The delta variant of COVID-19 represents the dominant variant in the study cohort. Five patients required ECMO therapy. Arterial hypertension (n = 4), diabetes mellitus (n = 3), and chronic kidney disease (n = 4) were the most common comorbidities. At the time of ultrasound, the median diagnosis of SARS-CoV-2 infection had been established 39 days prior. The median D-dimer value in the studied cohort was 7.7 mg/l. the median platelet value was quantified as 233/nl
Demographics and Characteristics | Patients (n = 13) |
of the Patients | |
Median age, y | 54 |
Mean age, y (SD) | 52 (13) |
Sex | |
Male (%) | 8 (62) |
Female (%) | 5 (38) |
Weight [BMI] (%) | |
Normal weight [18,5–24,9] | 4 (31) |
Overweight [25–29,9] | 3 (23) |
Obese class 1 [30 –34,9] | 3 (23) |
Obese class 2 [35 –39,9] | 0 |
Obese class 3 [≥40] | 3 (23) |
Median body mass index kg/m2 | 29 |
Mean body mass index kg/m2 (SD) | 30 (7) |
Concomitant disease | |
Hypertension | 4 (31) |
Diabetes mellitus | 3 (23) |
Chronic kidney disease | 4 (31) |
Chronic heart failure | 1 (7,5) |
Oncologic underlying disease | 1 (7,5) |
Vaccination status (%) | |
Unvaccinated | 11 (85) |
Vaccinated once | 0 (0) |
Vaccinated twice | 2 (15) |
Boosted | 0 |
Median days since first diagnosis of infection with SARS-CoV-2 | 39 |
Mean days since first diagnosis of infection with SARS-CoV-2 (SD) | 39 (14) |
Performance of COVID-Sequencing (%) | |
Yes | 6 (46) |
No | 7 (54) |
Virus-variant (%) | |
Delta | 5 (38,5) |
Omikron | 1 (7,5) |
Unknown | 7 (54) |
Non-invasive Ventilation (NIV) at the time of examination (%) | |
Yes | 1 (7,5) |
No | 12 (92,5) |
Median duration NIV before, d | 2 |
Ventilator dependent at the time of examination (%) | |
Yes | 12 (92,5) |
No | 1 (7,5) |
Median time on the ventilator, d | 35 |
ECMO at the time of examination (%) | |
Yes | 5 (38,5) |
No | 8 (61,5) |
Median time on ECMO, d | 18,5 |
Dialysis | |
Yes | 5 |
No | 5 |
Median time since start of dialysis, d | 0,5 |
Median platelets/nl | 233 |
Mean platelets/nl | 205 |
Median D-Dimer mg/l | 7,7 |
Mean D-Dimer mg/l | 10,04 |
Table 2
B-Mode/bowel wall thickness: 0 (3–4 mm, normal), 1 (5–6 mm, little), 2 (7–8 mm, moderate), 3 (>8 mm, severe); Elastography: 0 (normal), 1 (indurated), 2 (widened with edema), 3 (homogenous soft); CEUS: contrast-enhanced ultrasound: y (yes), n (no); Presence of Invasive devices at the time of US: D (Dialysis) E (ECMO) I (Intubated and ventilated) S (Spontaneous breathing without circulatory support)
Patient | Age, y | Sex (female/ male) | Days since infection with SARS-CoV2 at the time of US | B-Mode | CEUS | Elastography | Presence of Invasive devices at the time of US | SARS-CoV-2-RNA from respiratory material at the time of US, cop./ml | SARS-CoV-2-RNA in Serum samples at the time of US, cop./ml | Outcome |
1 | 45 | f | 35 | 3 | y | 2 | I, E | 0 | 0 | Deceased |
2 | 35 | f | 39 | 2 | y | 2 | I | 1,2×103 | 0 | ICU-Treatment |
3 | 81 | f | 8 | 2 | y | 2 | S | 2,9×106 | – | Hospital discharge |
4 | 62 | m | 44 | 2 | y | 2 | I, E, D | 0 | 0 | ICU-Treatment |
5 | 30 | m | 34 | 2 | y | 2 | I, E, D | 0 | 0 | Deceased |
6 | 54 | m | 46 | 2 | y | 2 | I, E | 0 | 0 | ICU-Treatment |
7 | 53 | m | 38 | 1 | y | 1 | I | 0 | 0 | Transfer to other hospital for Weaning |
8 | 55 | m | 39 | 1 | y | 1 | I | 103 bis 106 | 0 | Transfer to ICU in referring hospital |
9 | 60 | m | 65 | 2 | y | 1 | I, D | 0 | 0 | ICU-Treatment |
10 | 61 | m | 24 | 2 | y | 2 | I | 0 | 0 | ICU-Treatment |
11 | 59 | m | 29 | 1 | y | 2 | I, E, D | 1,4×104 | 0 | ICU-Treatment |
12 | 37 | w | 56 | 3 | y | 2 | I | 0 | 0 | Transfer to ICU in referring hospital |
13 | 50 | w | 44 | 2 | y | 2 | I | 0 | 0 | Transfer to ICU in referring hospital |
2.2Ultrasound Examination
The indication for the performance of modern high resolution ultrasound examinations including contrast-enhanced ultrasound had been made by the attending physicians at the intensive care units (ICU). CEUS investigations of critically ill patients at the ICU and the performance of intraoperative CEUS are supported by various clinical trials and the new “European Federation of Societies for Ultrasound in Medicine” (EFSUMB) guidelines [24] [25]. All examinations were performed by one experienced investigator (who has performed more than 2500 examinations/year) using a mobile ultrasound unit (GE LOGIQ E9, GE, Milwaukee, WI, USA) with the option to store dynamic digital cine-loops in DICOM format for CEUS. The requirements for ultrasound examinations of patients with COVID-19 can only be achieved using high-performance ultrasound equipment exhibiting maximum mobility. High-performance ultrasound combines different functionalities such as native B-mode procedure, elastography and CEUS.
Ultrasonography of the abdomen was performed according to protocol as previously described [26]. First, a sweep B-mode scan was performed using a multifrequency convex sector transducer (1–6 MHz, GE LOGIQ E9, GE, Milwaukee, WI, USA) to detect potentially affected areas of the small and large bowel. The bowel was initially examined for segmental or generalized thickening of the bowel wall > 3 mm with associated mural edema with or without accompanying free fluid, concomitant lymphadenopathy, hyperemia, and enlargement of intestinal fat. Color-coded Doppler sonography (CCDS) and power Doppler were used to screen for hyper vascularized areas. Intestinal loops that showed wall thickening > 3 mm and edema were then examined with a high-resolution linear multifrequency transducer ((6–9 MHz) LOGIQ E9, GE, Milwaukee, WI, USA) to clearly distinguish intestinal loops with acute edema from those with chronic changes of the intestinal wall.
In addition, color-coded strain elastography was performed (see below). Contrast-enhanced CT scan with an arterial, portal venous, and venous phase with 5-mm slices of the abdomen served as a reference method for direct comparison of image morphology of the bowel.
2.3Strain Elastography
Elastography is a novel ultrasound technique for measuring tissue stiffness or elasticity. A color representation of tissue elasticity is superimposed on the normal ultrasound image. Analogous to manual palpation, elastography uses the fact that tissues differ in their viscoelasticity [27].
Color-coded strain elastography was performed in bowel areas suspicious for bowel wall barrier disruption based on B-mode findings, as previously described [26]. To exclude fibrous stenoses, strain elastography was additionally performed in acute reactive bowel wall lesions to show soft bowel wall portions. As such, elastography implemented in the high-end ultrasound device allows assessment and visualization of elastic properties and stiffness of tissue acting against shear deformation.
While examining the intestinal area of interest with B-mode ultrasound, a strain elastography is generated by applying compression and decompression and by visualizing the resulting changes in the B-mode image. The image is transferred into a color-coded map using a scale from red (high strain, soft) to blue (low strain, hard).
As a result, scar formation appears with higher stiffness and therefore coded in blue as opposed to regions of acute intestinal inflammation displayed by tissue softening and coded in red or yellow [27, 28].
2.4Contrast-enhanced ultrasound (CEUS)
Microbubble contrast agent has expanded the capabilities of diagnostic medical ultrasound imaging and, in particular, improved the dignity assessment of focal liver lesions. CEUS provides enhanced echoes from the blood pool and has enabled diagnostic ultrasound to assess and quantify microvascular blood flow [20].
CEUS was applied by intravenous bolus injection of 1.5–2.4 mL Sulphur hexafluoride microbubbles (SonoVue, BRACCO, Italy) via a central venous catheter followed by a 10 mL saline solution flush. DICOM loops up to 1 min were continuously recorded, starting from the early arterial phase after 10–15 s up to 1 min and then short loops up to 10 s until the late phase (up to 5 min) and were digitally stored as DICOM loops of approximately 10 s for independent retrospective reading by two experienced ultrasound examiners.
Incipient contrast of the mesenteric tissue and bowel wall was considered the reference. Rapid contrast < 10 seconds was regarded as hyperemia with also increased flow signal in the duplex. For this purpose, flow parameters were optimized for low venous flow components, scale < 1000 Hz, wall filter < 100, optimization of the gain to the onset of artifacts or aliasing.
Evaluation of the intestinal loops regarding the contrast agent behavior was performed as previously described by our group [15]. Harmonic pulse inversion imaging (PIHI) and reduced mechanical index (MI < 0.2) were used to eliminate artifacts and ensure reproducibility of results. The use of PIHI and an MI < 0.2 increases tangential and lateral image discrimination. Translocation of microbubbles into the intestinal lumen were interpreted as a consequence of severe SARS-CoV-2 involvement of the small intestine and concomitant relevant intestinal barrier disruption.
2.5Ultrasound imaging analysis
B-mode ultrasound, strain elastography and CEUS was performed and interpreted during the same investigation by an experienced examiner. Whether microbubble penetration into the bowel lumen as a correlate of a significant intestinal barrier disorder was observed, was evaluated independently by two experienced examiners. Observed abnormalities in bowel wall thickness on B-mode (coding 0 (3-4 mm), 1 (5-6 mm, little), 2 (7-8 mm, moderate) (3 > 8 mm, severe) and strain elastography were coded from 0 to 3 to assess severity (coding: 0 normal), 1 (indurated), 2 (widened with edema), 3 (homogenous soft).
2.6Parametric analysis
Parametric analysis visually quantifies contrast enhancement in the vessels of the small intestine and therefore allows valid imaging of low-grade perfusion deficits. Parametric analysis made it possible to detect hypoperfused areas as consequences of microemboli.
During the CEUS examination, DICOM loops were generated, which were then processed by experienced investigators to generate parametric images.
This was achieved by using a software program integrated into the high-end ultrasound equipment (LOQIC E9 GE, Milwaukee, WI, USA). In this context, parametric analysis serves as a tool for visualizing microbubble dynamics by color encoding the bubble arrival time. Based on the stored dynamic DICOM loops, the time during which the contrast agent passes from the mesenteric vessels to the vessels of the intestinal wall was determined. Parametric analysis can then be initiated using the integrated software, and the parametric images are automatically generated by this software. This software algorithm for interpreting dynamic vascularization requires no further manual adjustments by the examiner and therefore generates examiner-independent results. In this way, parametric imaging serves as an analysis tool based on color-coded changes in micro vascularization or wash-in rate. The color bars can be customized by the user; at our institution, we chose the following setting: Early arterial enhancement was coded in red, intermediate as yellow, and prolonged as green shades. Late and low enhancement were represented in blue colors.
Assessment of an intestinal manifestation of SARS-CoV-2 infection in the areas examined with parametric CEUS imaging was based on early arterial hyperenhancement of the intestinal wall coded as red color, and according to the interpretation of conventional CEUS images, microbubble invasion of the intestinal lumen, represented in parametric false colors, was suspected as a significant disturbance of intestinal integrity. Therefore, dynamic evaluation of the red color signal detectable in the intestinal lumen, independent of the movement of artefacts into the lumen, was used to detect GI affection by SARS-CoV-2.
3Results
We analyzed 13 patients with severe COVID-19 infection (Table 1, 2). Ultrasound-based diagnosis of significant intestinal barrier disruption was established by two experienced ultrasound examiners based on the combined evaluation of intestinal wall thickening in B-mode ultrasound, hypervascularization in color-coded doppler sonography, and early arterial enhancement and transmural translocation of microbubbles in CEUS as described before [29].
In our patient cohort, we investigated whether there was early contrast accumulation in the mesenteric vessels. In addition, we assessed whether there was transmural enhancement in the intestinal wall sections of the small intestine and whether contrast enhancement was more likely to be continuous or segmental, early or delayed. Parametric analysis of contrast-enhanced ultrasound was used for this evaluation. We observed morphological signs of pathological bowel wall morphology in all patients. Signs such as hypervascularization, bowel wall edema, contrast enhancement and even intraluminal microbubble accumulation were present with varying degrees (Table 2, 3).
In 5 patients, we observed early and delayed segmental contrast accumulation in the small intestine (Table 3). In the parametric analysis, a mixed color pattern was documented in this subgroup (Figs. 2–5). Homogeneous, dense red and yellow tones dominated in the hyper perfused small intestine sections. In the hypo perfused small bowel sections, green and blue tones were in the foreground, which were partially scattered. The findings of parametric analysis of contrast-enhanced ultrasound and the findings of computed tomography were consistent in these patients. The simultaneous hyper- and hypo perfusion of the small bowel suspected by contrast enhanced ultrasound could be confirmed by computed tomography.
Table 3
Evaluation of parametric analysis of contrast ultrasound; m-Male, f-Female; CT: + pulmonary consolidations; ++ severe pulmonary consolidations
Patient, Gender and Age | Early contrast enhancement in the mesenteric vessels | Transmural accumulation of the contrast agent in the intestinal layers of the small intestine | Early segmental contrast enhancement of the small intestine | Delayed segmental contrast enhancement of the small intestine | Continuous contrast enhancement of the small intestine | CT |
1: f-45 | + | + | + + | |||
2: f-35 | + | + | + | + | ||
3: f-81 | + | + | ||||
4: m-62 | + | + | + | |||
5: m-30 | + | + | + | + + | ||
6: m-54 | + | + | + | |||
7: m-53 | + | + | + | + + | ||
8: m-55 | + | + | ||||
9: m-60 | + | + | + | |||
10: m-61 | + | + | + + | |||
11: m-59 | + | + | + | + + | ||
12: f-37 | + + | + | + | + | ||
13: f-50 | + | + | + | + + |
Fig. 2
CT images of a 59-year-old patient. A: CT image demonstrated simultaneous hyper- and hypo perfusion of the small bowel segments. B: In the parametric analysis of contrast ultrasound the image is congruent with the CT (Fig. 3). Segmental areas of the small bowel are hyper perfused and other areas are hypo perfused.The parametric images visualized simultaneous hypo- (white arrows) and hyper perfusion (gray arrows) 10 and 16 seconds after contrast administration.
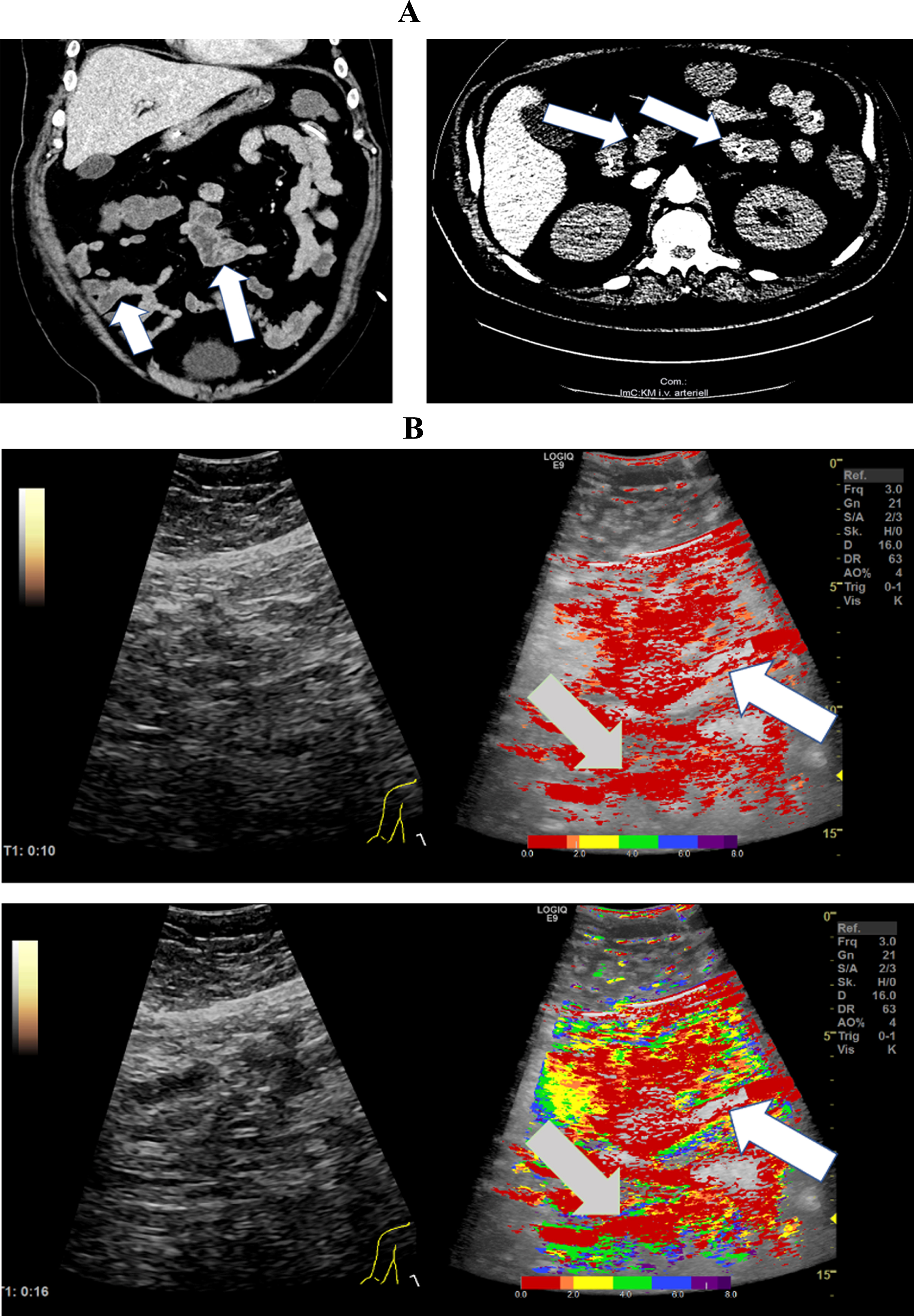
Fig. 3
A: CT images from a 50-year-old patient with severe COVID-19 ARDS. CT images document micro emboli in the kidney and spleen (white arrows). B: Parametric Image of a 50-year-old patient with severe COVID-19 disease.The parametric images visualized simultaneous hypo- (yellow areas, white arrows) and hyper perfusion (gray arrows) of the small intestine 49 seconds after contrast administration.
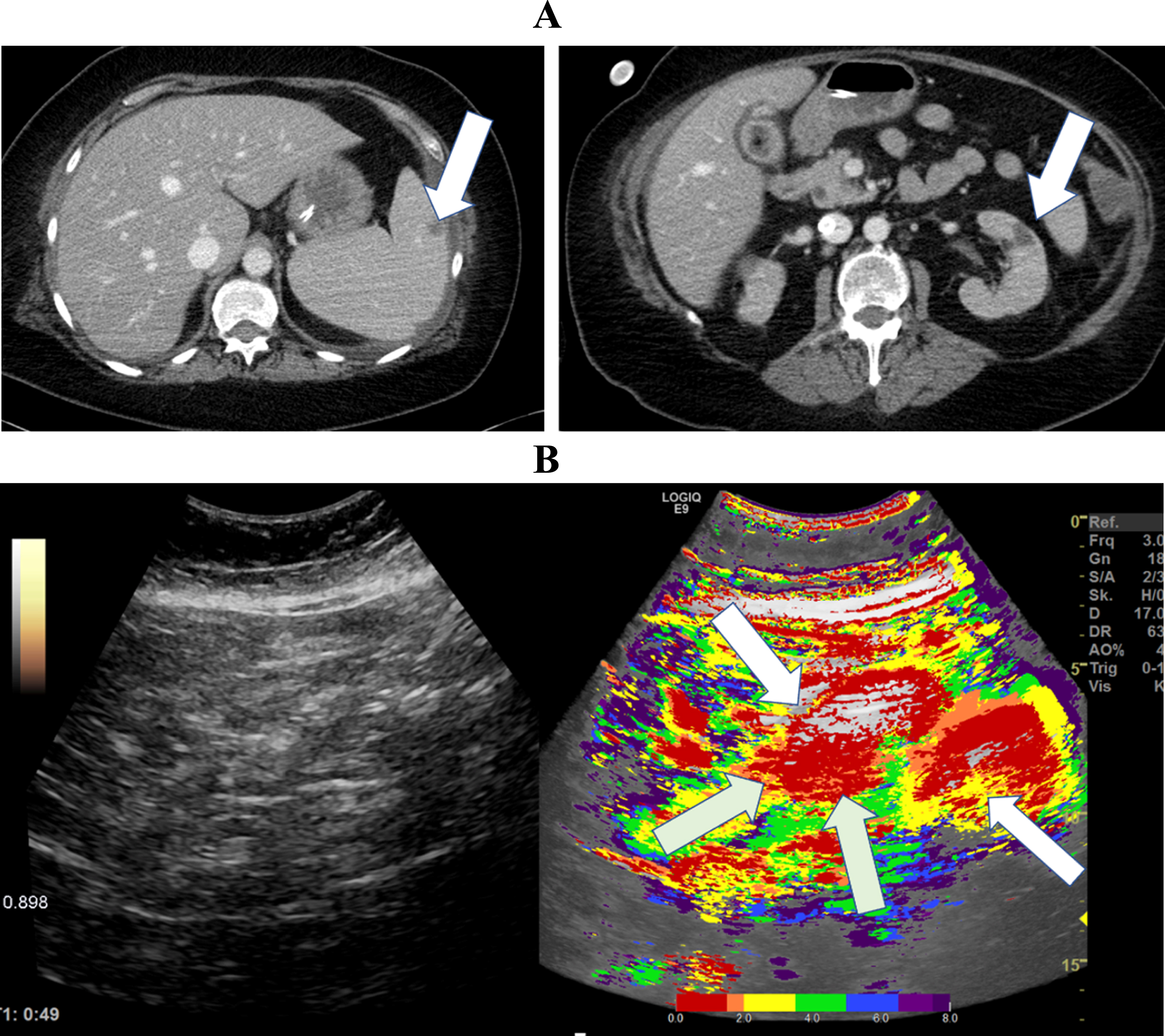
Fig. 4
A: CT Image of a 30-year-old patient with severe COVID-19 ARDS showing simultaneous segmental early and delayed contrast enhancement of the small intestine. B: Parametric Images of a 30-year-old patient with severe COVID-19 disease.The parametric images visualized simultaneous early- (white arrows) and delayed (gray arrows) segmental perfusion of the small intestine 39 seconds after contrast administration.
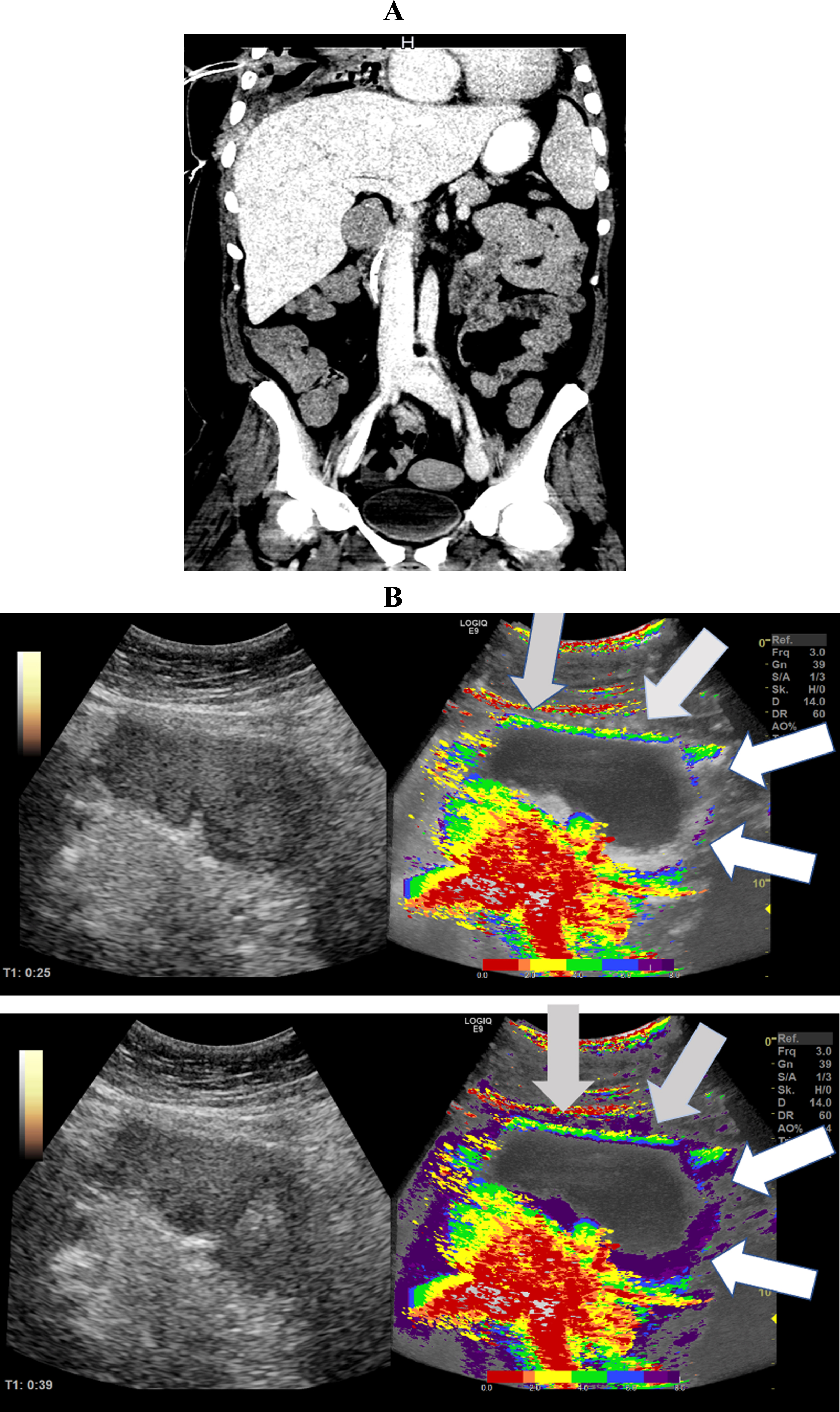
Fig. 5
A: CT Image of a 61-year-old patient with severe COVID-19 ARDS, micro- and macro embolism of the pulmonary arteries and severe pulmonary consolidations. B: Parametric Image of the small bowel of a 61-year-old patient with severe COVID-19 disease.The image documents a hyper- (grey arrows) and a hypo perfusion (white arrows) 54 seconds after contrast agent administration.
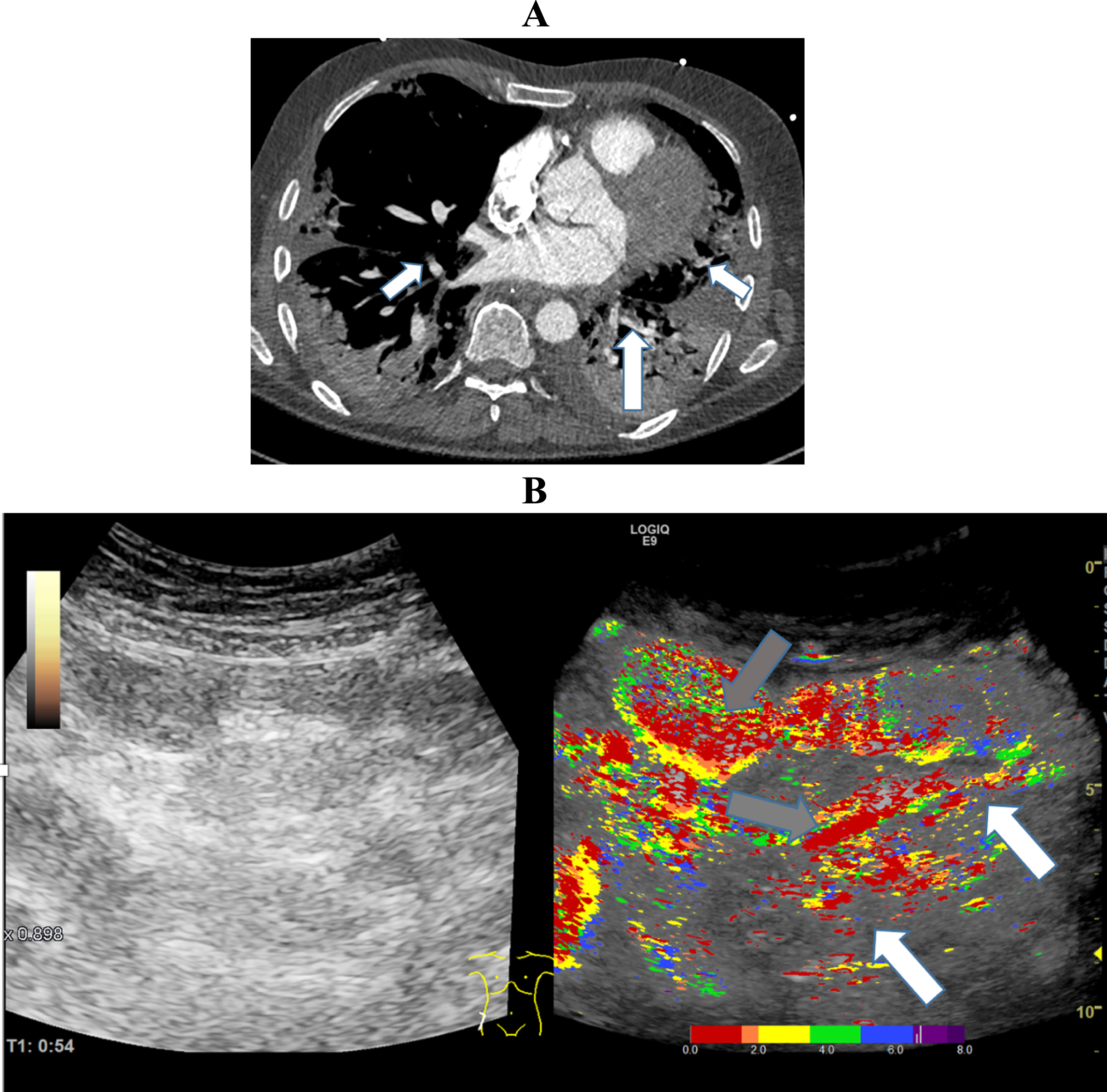
In addition, micro- and macro emboli were diagnosed in the kidney, spleen and lung in this patient population (Fig. 3 and 5).
Transmural contrast enhancement of the small intestine was observed in 8 patients by parametric analysis. Accordingly, red and yellow tones dominated the parametric analysis (Fig. 1). In 6 of the 8 patients, the findings of the small bowel on computed tomography were congruent. (Table 3).
Fig. 1
CT images of a 37-year-old patient with severe COVID-19 ARDS. A: The CT images documented hyperperfused small bowel areas (white arrows) and an early contrast enhancement in the mesenteric vessels (white arrows). B: The broad red coloration in the parametric analysis indicated early transmural enhancement (white arrows) of the small bowel sections.
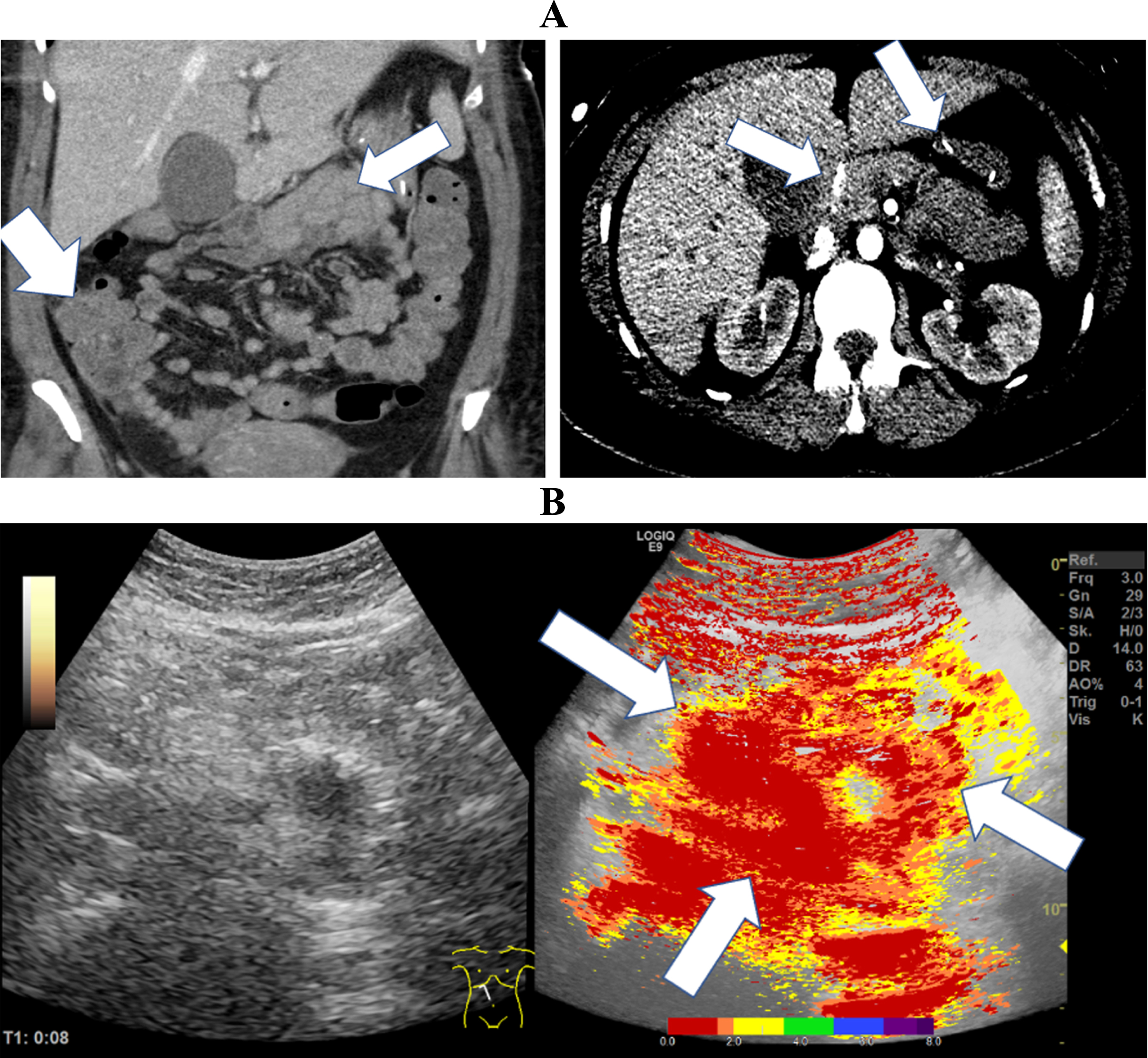
In Fig. 1, CT and CEUS images of a 37-year-old female patient are presented. The patient suffered from severe COVID-19 ARDS without relevant co-morbidities. The images demonstrate an early, almost fulminant accumulation of contrast agent in the wall of the small intestine 8 seconds after contrast application. We observed an early contrast enhancement in the mesenteric vessels and a continuous transmural accumulation of the contrast agent in the intestinal layers of the small intestine.
Figure 2 shows CT images of a 59-year-old patient with severe COVID-19 infection. In these images, early and delayed contrast accumulation in the small intestine is evident (Table 3).
Figure 3 shows CT images of a 50-year-old patient with severe COVID-19 disease and severe pulmonary consolidations. Micro emboli in the kidney and spleen are evident in the images. The parametric images visualized simultaneous hypo- (white arrows) and hyper perfusion (gray arrows) of the small intestine 49 seconds after contrast administration. The yellow and green coloration visualizes a delayed segmental small intestinal perfusion compared to the red and orange coloration.
In Fig. 4, parametric analysis of CEUS and a CT image of a 30-year-old patient severely affected by COVID-19 are shown. Parametric analysis visualized early contrast enhancement in the mesenteric vessels and segmental early and delayed contrast accumulation of the small intestine. The yellow and green tones represent early segmental perfusion, and the blue and purple tones represent a delayed segmental perfusion. The patient had no severe previous diseases before the infection with SARS-CoV-2.
Figure 5 visualizes segmental early and delayed small bowel perfusion. Dense red and yellow tones illustrate early segmental perfusion and scattered green and blue tones illustrate delayed segmental perfusion. The CT image shows severe pulmonary consolidations with segmental pulmonary emboli.
Table 2 presents the results using different morphological contrast agent distribution criteria.
In 10 patients, elastography demonstrated bowel wall edema with a high rate of soft fractions. The contrast agent SonoVue was applied in all included patients. In 7 patients, the microbubbles passed into the intestinal lumen. In 10 patients, the small bowel wall was moderately thickened (7-8 mm) on native ultrasound. In two patients segmental thickening of the small bowel wall to up to 10 mm could be demonstrated. In addition, duration of COVID-19 disease and infectivity is shown. SARS-CoV-2 RNA from respiratory samples and SARS-CoV- 2 RNA from serum samples at the time of the ultrasound examination are presented.
In summary, we identified pathologies of the small bowel in all included patients with severe COVID-19 disease. These pathologies could be identified by native ultrasound, elastography, CEUS and parametric analysis of contrast enhanced ultrasound (Table 2). In particular, the high proportion of soft bowel wall edema (n = 8) and the detection of microbubbles in the intestinal lumen (n = 7) support the hypothesis of a small bowel manifestation in the context of COVID-19 systemic disease.
Table 3 demonstrates the evaluation of the parametric analysis of the contrast enhanced ultrasound. Early contrast enhancement in the mesenteric vessels was detected in 4 patients and very early contrast enhancement in one patient. Transmural accumulation of the contrast agent in the intestinal layers of the small intestine was observed in 8 patients. In 5 patients the combination of segmental early and delayed contrast enhancement in the small intestine could be identified. Global contrast agent enhancement could be described in 5 patients. Severe pulmonary consolidations were seen in 6 patients. Table 3 shows the following trend: The more severe the Covid-19 disease, the earlier the contrast agent accumulated in the mesenteric vessels and the more likely there was delayed contrast agent uptake in the small intestine. In our cohort, it is evident that the unvaccinated patients undergo a more severe course of COVID-19 disease. In the studied collective, embolism in various organ systems and also in the intestine is observed in a majority of the unvaccinated patients.
4Discussion
The aim of our work was to assess the increasing evidence for GI tract involvement during SARS-CoV-2 infection [30]. An additional focus was to better understand the nature of small intestinal involvement by image morphology and to gain insights into pathophysiology. For this purpose, we applied advanced ultrasound techniques using parametric analysis of contrast enhanced ultrasound.
CEUS is an excellent diagnostic tool for monitoring severe cases of intermediate and intensive care patients with early changes of abdominal microcirculatory disorders and small as well as large bowel pathologies [24, 31, 32]. The advantage of CEUS with sulphur hexafluoride microbubbles is the dynamic assessment including the capillary level and strictly intravascular from the early arterial phase (10 to 15 s) to the late phase of 5 min. In addition, sulphur hexafluoride microbubbles has the decisive advantage of not being nephrotoxic and not having any effect on thyroid function [25]. CEUS has the great advantage in comparison to sectional imaging that it can be –repeatedly - performed bedside. In the hands of a skilled examiner, the diagnostic value of CEUS in the bowel is high [33, 34].
CEUS has limitations compared to cross-sectional imaging because only sections of the bowel can be assessed. The global overview of the bowel is wider in cross-sectional imaging. CEUS is not able to record dynamically and continuously all intestinal structures in their micro vascularization at the same time. Experienced examiners and special equipment are required. The price for 2.4 ml ultrasound contrast medium is 40-50 euros. The time expenditure with the necessary hygiene measures lies with up to one hour with respect to all necessary processes for the examination under COVID-19 protective measures. Modern sonography including CEUS has proven its value in the COVID-19 pandemic and was able to detect microcirculatory dysfunction at an early stage [35–37]. It is necessary to monitor the microcirculation at the bedside in intensive care patients who have serious COVID-19 disease [30]. The use of CEUS allows bedside investigations for a variety of clinical questions relevant to clinical practice and decision making. It is possible to quickly rule out organ infarction and to dynamically record the mesenteric arterial and venous blood flow [36]. CEUS can also detect peripheral reduced blood flow, micro-infarctions, embolisms in the context of pulmonary artery embolism and reactive hyperemia in the case of consolidations and pleural irritation in the periphery of the lungs [38–40]. CEUS enables the dynamic assessment of organ micro perfusion, especially of the liver, spleen and kidneys [25].
There is growing evidence that the combination of endothelial dysfunction with a generalized inflammatory state and complement involvement may contribute to the overall pro-coagulative state described in COVID-19 patients. This leads to venular as well as to arteriolar occlusions [12, 41–43].
We could confirm this hypothesis using CEUS and parametric analysis.
In our cohort, we were able to demonstrate by parametric analysis in a subset of 5 patients an early and delayed contrast enhancement in the intestinal layers of the small intestine (Fig. 2, 3, 4, 5 and Table 3). On the one hand, we evaluated the early segmental hyperemia as an expression of systemic inflammation. On the other hand, the delayed perfusion in small bowel segments could be an expression of inadequate, reduced perfusion as a consequence of micro emboli.
SARS-CoV-2 infection has been described to increase the risk of venous and arterial thromboembolic events [44, 45]. In a subgroup of patients in whom we suspected segmental hypoperfusion of small intestinal segments because of emboli, emboli appeared in additional organ systems such as the kidney, spleen, and lung (Fig. 3, 5). Emboli in other organ systems, which are CT morphologically proved, support our pathophysiological considerations.
In our cohort, 2 subgroups can be classified (Table 3). In one subgroup (n = 5), global transmural contrast enhancement of the small bowel layers predominates. In the other group (n = 5), segmental early and delayed contrast enhancement predominates.
In patients with transmural continuous contrast agent accumulation of the small intestine, systemic infection is presumably predominant. In patients with combined segmental hypo- and hyper perfusion, prothrombic factors and micro emboli might play a crucial role.
The pathophysiology of severe COVID-19 disease is complex and the clinical course heterogeneous. Evidence is mounting that the gastrointestinal tracts plays a central role in the acute phase of SARS-CoV-2 infection and also in post-COVID-19 syndrome [6, 46–48].
There is progressive evidence on mesenteric thrombosis, prothrombotic factors, acute mesenteric ischemia, and pathophysiological principles in severe COVID -19 disease.
A metaanalysis by Patel et al. confirmed the significance of intestinal ischemia in severe COVID-19 disease and the high mortality of this subgroup [49].
In our cohort, a mean D-dimer of 10.04 mg/l could be described (Table 3). This supports the hypothesis of a prothrombogenic coagulation status. The limitation of evaluating the increased D-Dimer value is the high number of ECMO therapies in our cohort and the associated changes of the coagulation situation. However, it is likely that prothrombogenic coagulation status increases with the length of the disease course [44, 45].
The SARS-CoV-2 virus has a high affinity and tropism for binding to angiotensin-converting enzyme-2 (ACE-2) receptors on enterocytes. This mediates the tropism of the virus for enterocytes causing direct damage to the bowel tissue [50].
After virus binding to ACE-2, the ACE-2/SARS-CoV-2 complex is internalized, resulting in decreased surface expression of ACE2 protein [51, 52]. ACE2 is a carboxypeptidase that converts angiotensin 2 to angiotensin 1-7. Angiotensin 1-7 exerts anti-inflammatory properties by binding to the G protein-coupled receptor Mas. Virus-mediated downregulation of ACE2 leads to accumulation of angiotensin 2 and inhibits the formation of angiotensin 1-7, resulting in a shift toward pro-inflammatory status [51]. Furthermore, endothelial cells increase the expression of tissue factor and plasminogen activator inhibitor-1 because of the hypercoagulability caused by increased levels of angiotensin 2 [53].
Furthermore, virus-induced death of endothelial cells leads to the release of danger signals (DAMPs) that can activate the innate immune response and trigger a cytokine storm leading to the release of IL-6, IL-2 R, IL-10, and TNF [51]. This endothelial inflammation increases the formation of procoagulant factors like fibrinogen, factor VIII and von Willebrand factor secreted by the Weibel Palade bodies. The hypercoagulable state in COVID-19 can cause thrombosis of small mesenteric vessels and bowel ischemia [54, 55].
In numerous patients, multiple auto-antibodies normally found in other autoimmune diseases, such as antinuclear antibodies, antiphospholipid antibodies, and many others, have been detected in severe COVID-19 disease. In addition, numerous case reports of COVID-19-infected patients who developed autoimmune diseases during the course of COVID-19 have been published [56]. It is conceivable that autoimmune phenomena with an accompanying prothrombogenic component play a role in the small bowel involvement associated with COVID-19 disease in our cohort. The ACE-2 receptor is regarded to play a decisive role in COVID-19 pathophysiology as a portal of entry into the lung as well as the intestinal mucosal epithelial cells [57, 58]. ACE-2 is not only an enzyme but also a functional receptor on cell surfaces through which SARS-CoV-2 enters the host cells and is highly expressed in the heart, kidneys, lungs and the bowel and shed into the plasma [59]. Zhang et el. described a distinct ACE-2 expression on small intestinal cells in COVID-19 patients [60–62]. Notably, the results of the study by Hamming et al. indicate that ACE-2 is present in all parts of small intestinal epithelium cells, including the duodenum, the jejunum, and the ileum, but not in enterocytes of the colon [63].
This is very conclusive with our findings as we observed an accumulation of small bowel pathologies, but not of the large bowel. One explanation for this observation may be a different receptor occupancy of the ACE receptor in the small and large intestine.
Severe disease of COVID-19 infection presents with a clinical and laboratory overlap with other hyperinflammatory conditions such as hemophagocytic lymphohistiocytosis (HLH). Schimke et al. identified that COVID-19 and HLH share common immunological pathways (cytokine/chemokine signaling and neutrophil-mediated immune responses), including gene signatures that stratify COVID-19 patients admitted to the intensive care unit (ICU) from COVID-19 and non-ICU patients.
However, during the early cytokine storm in severe SARS-CoV-2 infection, enhanced neutrophil infiltration, neutrophil extracellular trap release and complement activation are thought to play critical roles [64]. In addition, there are several factors that influence the pro-thrombogenic coagulation situation in SARS-CoV-2 infection like increased vascular permeability, increased endothelial inflammatory response, decreased NO, and impaired angiogenesis [41, 65, 66].
Gut microbial metabolites, dysbiosis and dysregulation of the intestinal barrier influence inflammation, immune responses and disease progression of the lung [60]. The complex intercommunication through the gut-lung axis might be important in predetermining the susceptibility of the airways to SARS-CoV-2 infection and COVID-19 clinical outcome as a function of the inter-individual variations in microbiota composition and richness [67]. The high levels of circulating proinflammatory cytokines by viral infections are capable of altering gut microbiota and affecting intestinal integrity [68]. Small bowel malfunction leads to altered gut microbiota and inflammation due to the well-balanced bidirectional interaction between the gut microbiota and the immune system. Increased inflammation in the intestine leads to a leaky gut allowing bacterial antigens and toxins to translocate to the systemic circulation [69]. The mechanism of a disrupted gastrointestinal barrier and the role of the gut microbiota for epithelial integrity was described by our research group in patients with liver cirrhosis. A reduced mucus layer allows direct contact of bacteria and epithelial cells. This direct interaction leads to a degradation of crucial cell-cell contact proteins by bacterial proteases and also by bacterial-mediated induction of the proteasome. The reduction of these proteins then leads to a loss of epithelial integrity [70]. Similarities in pathophysiology in COVID-19 patients are conceivable [70].
In conclusion, using parametric analysis of small bowel contrast enhanced ultrasound, we were able to define different subgroups of patients with severe COVID-19 disease. To date, our work is the first to use dynamic parametric analysis to assess whether COVID-19 infected patients have segmental, continuous, early and/or delayed contrast enhancement of the small intestine. Prothrombogenic factors, microemboli, and segmental hypoperfusion seem to play a crucial role in the gastrointestinal involvement of severe COVID-19 disease in a subgroup of patients.
5Conclusion
Intestinal barrier disorders resulting from COVID-19 infection are common and can be diagnosed at the bedside by multiparametric US examinations. Parametric analyses of contrast enhanced ultrasound allow assessment of perfusion ratios and provide clues to the genesis of barrier disruption of the small intestine.
Our group hypothesized that intestinal barrier disruption in severe COVID-19 disease is a combination of hyperemia in the setting of systemic infection and hypoxemia in the setting of micro emboli in a subgroup of patients.
REFERENCES
[1] | Hasöksüz M , Kiliç c S , Saraç c F , Coronaviruses and SARS-COV-2, Turk J Med Sci (2020) ;50: (SI-1):549–56. |
[2] | Gao Y , Ding M , Dong X , Zhang J , Kursat Azkur A , Azkur D.u.a , Risk factors for severe and critically ill COVID-19 patients: A review, Allergy (2021) ;76: (2):428–55. |
[3] | Ashraf UM , Abokor AA , Edwards JM , Waigi EW , Royfman RS , Hasan SAM.u.a. , SARS-CoV-2, ACE2 expression, and systemic organ invasion, Physiol Genomics (2021) ;53: (2):51–60. |
[4] | Synowiec A , Szczepański A , Barreto-Duran E , Lie LK , Pyrc K , Severe Acute Respiratory Syndrome Coronavirus 2 (SARS-CoV-2): aSystemic Infection, Clin Microbiol Rev. 17. Märze (2021) ;34: (2):00133–20. |
[5] | Hunt RH , East JE , Lanas A , Malfertheiner P , Satsangi J , Scarpignato C.u.a. , COVID-19 and Gastrointestinal Disease: Implications for the Gastroenterologist, Dig Dis (2021) ;39: (2):119–39. |
[6] | Mao R , Qiu Y , He JS , Tan JY , Li XH , Liang J.u.a. , Manifestations and prognosis of gastrointestinal and liver involvement in patients with COVID- a systematic review and meta-analysis, Lancet Gastroenterol Hepatol (2020) ;5: (7):667–78. |
[7] | Dhar D , Mohanty A , Gut microbiota and Covid-19- possible link and implications, Virus Res (2020) ;285: , 198018. |
[8] | Yeoh YK , Zuo T , Lui GCY , Zhang F , Liu Q , Li AY.u.a , Gut microbiota composition reflects disease severity and dysfunctional immune responses in patients with COVID-19, Gut (2021) ;70: (4):698–706. |
[9] | Zuo T , Wu X , Wen W , Lan P , Gut Microbiome Alterations in COVID-19, Genomics Proteomics Bioinformatics. S-(21) (2021) ;S1672-0229: (21):00206–0. |
[10] | Proal AD , VanElzakker MB , Long COVID or Post-acute Sequelae of COVID-19 (PASC): An Overview of Biological Factors That May Contribute to Persistent Symptoms, Front Microbiol (2021) ;12: , 698169. |
[11] | Nugroho J , Wardhana A , Mulia EP , Maghfirah I , Rachmi DA , A’yun MQ.u.a , Elevated fibrinogen and fibrin degradation product are associated with poor outcome in COVID-19 patients: A meta-analysis, Clin Hemorheol Microcirc (2021) ;77: (2):221–31. |
[12] | Xing Y , Yang W , Jin Y , Wang C , Guan X , D-dimer daily continuous tendency predicts the short-term prognosis for COVID-19 independently: A retrospective study from Northeast China, Clin Hemorheol Microcirc (2021) ;79: (2):269–77. |
[13] | Shaik A , Chen Q , Mar P , Kim H , Mejia P , Pacheco H.u.a , Blood hyperviscosity in acute and recentCOVID-19 infection. Clin Hemorheol Microcirc. 2022 18. April. doi: 10.3233/CH-221429. |
[14] | Henry BM , Vikse J , Benoit S , Favaloro EJ , Lippi G , Hyperinflammation and derangement of renin-angiotensin-aldosterone system in COVID- A novel hypothesis for clinically suspected hypercoagulopathy and microvascular immunothrombosis, Clin Chim Acta (2020) ;507: :167–73. |
[15] | Becker RC , COVID-19-associated vasculitis and vasculopathy, J Thromb Thrombolysis (2020) ;50: (3):499–511. |
[16] | McFadyen JD , Stevens H , Peter K . The Emerging Threat of (Micro)Thrombosis in COVID-19 and Its Therapeutic Implications, Circ Res (2020) ;127: (4):571–87. |
[17] | Mocci G , Migaleddu V , Cabras F , Sirigu D , Scanu D , Virgilio G.u.a , SICUS and CEUS imaging in Crohn’s disease: an update, J Ultrasound. März (2017) ;20: (1):1–9. |
[18] | Medellin A , Merrill C , Wilson SR Role of contrast-enhanced ultrasound in evaluation of the bowel. Abdom Radiol (NY) (2018) ;43: (4):918–33. |
[19] | Pausch AM , Kammerer S , Weber F , Herr W , Stroszczynski C , Holler E.u.a , Parametric Imaging of Contrast-Enhanced Ultrasound (CEUS) for the Evaluation of Acute Gastrointestinal Graft-Versus-Host Disease, Cells (2021) ;10: (5):1092. |
[20] | Yusefi H , Helfield B , Ultrasound Contrast Imaging: Fundamentals and Emerging Technology, Front Phys (2022) ;10: :791145. |
[21] | Ajmal S , Contrast-Enhanced Ultrasonography: Review and Applications, Cureus (2021) ;13: (9):e18243. |
[22] | Malone CD , Fetzer DT , Monsky WL , Itani M , Mellnick VM , Velez PA.u,.a , Contrast-enhanced US for the Interventional Radiologist: Current and Emerging Applications, Radiographics (2020) ;40: (2):562–88. |
[23] | Lamby P , Jung F , Graf S , Schellenberg L , Falter J , Platz-da-Silva N.u.a , Effect of iodinated contrast media on renal perfusion: A randomized comparison study in pigs using quantitative contrast-enhanced ultrasound (CEUS), Sci Rep (2017) ;7: (1):13125. |
[24] | Sidhu PS , Cantisani V , Dietrich CF , Gilja OH , Saftoiu A , Bartels E.u.a , The EFSUMB Guidelines and Recommendations for the Clinical Practice of Contrast-Enhanced Ultrasound (CEUS) in Non-Hepatic Applications: Update (Long Version), Ultraschall Med (2018) ;39: (2):e2–44. |
[25] | Dietrich CF , Averkiou M , Nielsen MB , Barr RG , Burns PN , Calliada F.u.a , How to perform Contrast-Enhanced Ultrasound (CEUS), Ultrasound Int Open (2018) ;4: (1):E2–15. |
[26] | Weber D , Weber M , Hippe K , Ghimire S , Wolff D , Hahn J.u,a , Non-invasive diagnosis of acute intestinal graft-versus-host disease by a new scoring system using ultrasound morphology, compound elastography, and contrast-enhanced ultrasound, Bone Marrow Transplant (2019) ;54: (7):1038–48. |
[27] | Ozturk A , Grajo JR , Dhyani M , Anthony BW , Samir AE , Principles of ultrasound elastography, Abdom Radiol (NY) (2018) ;43: (4):773–85. |
[28] | Dietrich CF , Bamber J , Berzigotti A , Bota S , Cantisani V , Castera L.u.a , EFSUMB Guidelines and Recommendations on the Clinical Use of Liver Ultrasound Elastography, Update (Long Version), Ultraschall Med (2017) ;38: (4):e16–47. |
[29] | Tews HC , Kandulski A , Schmid S , Peschel G , Gülow K , Schlosser S.u.a , Contrast enhanced ultrasonography (CEUS) a novel tool to detect intestinal epithelial barrier dysfunction in severe COVID-19 disease. CH. 2022;1-14. |
[30] | Tirumani SH , Rahnemai-Azar AA , Pierce JD , Parikh KD , Martin SS , Gilkeson R.u.a , Are asymptomatic gastrointestinal findings on imaging more common in COVID-19 infection? Study to determine frequency of abdominal findings of COVID-19 infection in patients with and without abdominal symptoms and in patients with chest-only CT scans, Abdom Radiol (NY) (2021) ;46: (6):2407–14. |
[31] | Lerchbaumer MH , Putz FJ , Rübenthaler J , Rogasch J , Jung EM , Clevert DA.u.a , Contrast-enhanced ultrasound (CEUS) of cystic renal lesions in comparison to CT and MRI in a multicenter setting, Clin Hemorheol Microcirc (2020) ;75: (4):419–29. |
[32] | Lerchbaumer MH , Kleemann T , Jung EM , Nagel S , Hamm B , Fischer T . Vascular pattern and diagnostic accuracy of contrast-enhanced ultrasound (CEUS) in spleen alterations, Clin Hemorheol Microcirc (2020) ;75: (2):177–88. |
[33] | Ma X , Ling W , Xia F , Zhang Y , Zhu C , He J , Application of Contrast-Enhanced Ultrasound (CEUS) in Lymphomatous Lymph Nodes: A Comparison between PET/CT and Contrast-Enhanced CT. Contrast Media Mol Imaging. (2019) ;2019: 5709698. |
[34] | Wilson SR , Lyshchik A , Piscaglia F , Cosgrove D , Jang HJ , Sirlin C.u.a ,>CEUS LI-RADS: algorithm, implementation, and key differences from CT/MRI, Abdom Radiol (NY) (2018) ;43: (1):127–42. |
[35] | Peschel G , Jung EM , Fisser C , Putz FJ , Wertheimer T , Sinner B.u,a , Interstitial lung opacities in patients with severe COVID-19 pneumonia by bedside high-resolution ultrasound in association to CO2 retention, Clin Hemorheol Microcirc (2021) ;77: (4):355–65. |
[36] | Jung EM , Stroszczynski C , Jung F , Contrast enhanced ultrasonography (CEUS) to detect abdominal microcirculatory disorders in severe cases of COVID-19 infection: First experience, Clin Hemorheol Microcirc (2020) ;74: (4):353–61. |
[37] | Jung EM , Stroszczynski C , Jung F , Contrast enhanced ultrasound (CEUS) to assess pleural pulmonal changes in severe COVID-19 infection: First results, Clin Hemorheol Microcirc (2020) ;75: (1):19–26. |
[38] | Watchorn J , Huang DY , Joslin J , Bramham K , Hutchings SD , Critically Ill COVID-19 Patients With Acute Kidney Injury Have Reduced Renal Blood Flow and Perfusion Despite Preserved Cardiac Function: A Case-Control Study Using Contrast-Enhanced Ultrasound, Shock (2021) ;55: (4):479–87. |
[39] | Safai Zadeh E , Görg C , Dietrich CF , Görlach J , Alhyari A , Trenker C , Contrast-Enhanced Ultrasound for Evaluation of Pleural Effusion: A Pictorial Essay. J Ultrasound Med. 29. März 2021; |
[40] | Safai Zadeh E , Beutel B , Dietrich CF , Keber CU , Huber KP , Görg C.u.a , Perfusion Patterns of Peripheral Pulmonary Lesions in COVID-19 Patients Using Contrast-Enhanced Ultrasound (CEUS): A Case Series, J Ultrasound Med (2021) ;40: (11):2403–11. |
[41] | Jung F , Krüger-Genge A , Franke RP , Hufert F , Küpper JH , COVID-19 and the endothelium, Clin Hemorheol Microcirc (2020) ;75: (1):7–11. |
[42] | Watson O , Pillai S , Howard M , Cezar-Zaldua J , Whitley J , Burgess B.u.a , Impaired fibrinolysis in severe Covid-19 infection is detectable in early stages of the disease. CH. 2022;1-9.. |
[43] | Tripolino C , Pizzini AM , Zaccaroni S , Cicognani C , Dapporto S , Cipollini ML.u.a , Is SARS-CoV-2 infection an emerging risk factor for splanchnic venous thrombosis? Clin Hemorheol Microcirc (2021) ;79: (2):347–55. |
[44] | Gómez-Mesa JE , Galindo-Coral S , Montes MC , Muñoz Martin AJ , Thrombosis and Coagulopathy in COVID-19, Curr Probl Cardiol. März (2021) ;46: (3):100742. |
[45] | Ali MAM , Spinler SA , COVID-19 and thrombosis: From bench to bedside, Trends Cardiovasc Med (2021) ;31: (3):143–60. |
[46] | Mönkemüller K , Fry L , Rickes S , COVID-19, coronavirus, SARS-CoV-2 and the small bowel, Rev Esp Enferm Dig (2020) ;112: (5):383–8. |
[47] | Lamers MM , Beumer J , van der Vaart J , Knoops K , Puschhof J , Breugem TI.u,a ,SARS-CoV-2 productively infects human gut enterocytes, Science (2020) ;369: (6499)50–4. |
[48] | Hilpert K , Mikut R , Is There a Connection Between Gut Microbiome Dysbiosis Occurring in COVID-19 Patients and Post-COVID-19 Symptoms? Front Microbiol (2021) ;12: , 732838. |
[49] | Patel S , Parikh C , Verma D , Sundararajan R , Agrawal U , Bheemisetty N.u.a , Bowel ischemia in COVID- A systematic review., Int J Clin Pract (2021) ;75: (12):e14930. |
[50] | Singh B , Kaur P , COVID-19 and acute mesenteric ischemia: A review of literature, Hematol Transfus Cell Ther (2021) ;43: (1):112–6. |
[51] | Zhang H , Shao B , Dang Q , Chen Z , Zhou Q , Luo H.u.a , Pathogenesis and Mechanism of Gastrointestinal Infection With COVID-19, Front Immunol (2021) ;12: , 674074. |
[52] | Kuba K , Imai Y , Rao S , Gao H , Guo F , Guan B.u.a , A crucial role of angiotensin converting enzyme 2 (ACE2) in SARS coronavirus-induced lung injury, Nat Med (2005) ;11: (8):875–9. |
[53] | Rodriguez-Nakamura RM , Gonzalez-Calatayud M , Martinez Martinez AR , Acute mesenteric thrombosis in two patients with COVID-19, Two cases report and literature review. Int J Surg Case Rep (2020) ;76: :409–14. |
[54] | Parry AH , Wani AH , Yaseen M , Acute Mesenteric Ischemia in Severe Coronavirus-19 (COVID-19): Possible Mechanisms and Diagnostic Pathway, Acad Radiol (2020) ;27: (8):1190. |
[55] | Escher R , Breakey N , Lämmle B , Severe COVID-19 infection associated with endothelial activation, Thromb Res (2020) ;190: :62. |
[56] | Saad MA , Alfishawy M , Nassar M , Mohamed M , Esene IN , Elbendary A , COVID-19 and Autoimmune Diseases: A Systematic Review of Reported Cases, Curr Rheumatol Rev (2021) ;17: (2):193–204. |
[57] | Harrison AG , Lin T , Wang P , Mechanisms of SARS-CoV-2 Transmission and Pathogenesis, Trends Immunol (2020) ;41: (12):1100–15. |
[58] | Hatmal MM , Alshaer W , Al-Hatamleh MAI , Hatmal M , Smadi O , Taha MO.u.a , Comprehensive Structural and Molecular Comparison of Spike Proteins of SARS-CoV-2, SARS-CoV and MERS-CoV, and Their Interactions with ACE2, Cells (2020) ;9: (12):2638. |
[59] | Beyerstedt S , Casaro EB , Rangel ÉB , COVID-angiotensin-converting enzyme 2 (ACE2) expression and tissuesusceptibility to SARS-CoV-2 infection, Eur J Clin Microbiol InfectDis (2021) ;40: (5):905–19. |
[60] | Zhang H , Li HB , Lyu JR , Lei XM , Li W , Wu G.u.a ,Specific ACE2 expression in small intestinal enterocytes may cause gastrointestinal symptoms and injury after -nCoV infection, Int J Infect Dis (2020) ;96: :19–24. |
[61] | Dotan A , Shoenfeld Y , [COVID-19 AND AUTOIMMUNE DISEASES], Harefuah (2021) ;160: (2):62–7. |
[62] | Galeotti C , Bayry J , Autoimmune and inflammatory diseases following COVID-19, Nat Rev Rheumatol (2020) ;16: (8):413–4. |
[63] | Hamming I , Timens W , Bulthuis MLC , Lely AT , Navis GJ , van Goor H , Tissue distribution of ACE2 protein, the functional receptor for SARS coronavirus, A first step in understanding SARS pathogenesis. J Pathol (2004) ;203: (2):631–7. |
[64] | Parackova Z , Zentsova I , Bloomfield M , Vrabcova P , Smetanova J , Klocperk A.u.a , Disharmonic Inflammatory Signatures in COVID- Augmented Neutrophils’ but Impaired Monocytes’ and Dendritic Cells’ Responsiveness., Cells (2020) ;9: (10):2206. |
[65] | Amraei R , Rahimi N , COVID-19, Renin-Angiotensin System and Endothelial Dysfunction, Cells (2020) ;9: (7):1652. |
[66] | Astapenko D , Tomasova A , Ticha A , Hyspler R , Chua HS , Manzoor M.u.a , Endothelial glycocalyx damage in patients with severe COVID-19 on mechanical ventilation - A prospective observational pilot study, Clin Hemorheol Microcirc (2022) ;81: (3):205–19. |
[67] | Donati Zeppa S , Agostini D , Piccoli G , Stocchi V , Sestili P , Gut Microbiota Status in COVID- An Unrecognized Player? Front Cell Infect Microbiol (2020) ;10: :576551. |
[68] | Hussain I , Cher GLY , Abid MA , Abid MB , Role of Gut Microbiome in COVID- An Insight Into Pathogenesis and Therapeutic Potential, Front Immunol (2021) ;12: :765965. |
[69] | Wang H , Ma S , The cytokine storm and factors determining the sequence and severity of organ dysfunction in multiple organ dysfunction syndrome, Am J Emerg Med (2008) ;26: (6):711–5. |
[70] | Haderer M , Neubert P , Rinner E , Scholtis A , Broncy L , Gschwendtner H.u.a , Novel pathomechanism for spontaneous bacterial peritonitis: disruption of cell junctions by cellular and bacterial proteases. Gut. 2021;gutjnl-2020-321663. |