Effects of hydrocortisone combined with vitamin C and vitamin B1 versus hydrocortisone alone on microcirculation in septic shock patients: A pilot study
Abstract
OBJECTIVE:
To investigate the effects of hydrocortisone combined with vitamin C and vitamin B1 versus hydrocortisone on sublingual microcirculation in septic shock patients.
METHODS:
This pilot study enrolled septic shock patients admitted to the ICU of a tertiary teaching hospital from February 2019 to January 2020. We randomly assigned the enrolled patients to the treatment group (hydrocortisone combined with vitamin C and vitamin B1 added to standard care) and the control group (hydrocortisone alone added to standard care) in a 1 : 1 ratio. The primary outcome was perfused small vascular density (sPVD) monitored by a sublingual microcirculation imaging system at 24 hours after treatment.
RESULTS:
Twelve patients in the treatment group and ten in the control group completed the study. The baseline characteristics were comparable between the groups. No statistically significant difference was found in the sPVD between the groups at baseline. The sPVD in the treatment group was significantly higher than that in the control group at 4 hours after treatment (mean difference, 7.042; 95% CI, 2.227-11.857; P = 0.009) and 24 hours after treatment (mean difference, 7.075; 95% CI, 2.390-11.759; P = 0.008).
CONCLUSIONS:
Compared with hydrocortisone, hydrocortisone combined with vitamin C and vitamin B1 significantly improves microcirculation in septic shock patients.
1Background
Sepsis is a life-threatening organ dysfunction caused by a dysregulated host response to infection. Septic shock is a subset of sepsis and leads to high mortality. Globally, approximately 48 million incident cases of sepsis and 11 million sepsis-related deaths (accounting for approximately 20% of all deaths) occur annually [1]. A national multicenter epidemiological investigation in China showed that the 90-day mortality of patients with septic shock was more than 50% [2]. Effectively reducing mortality and improving the prognosis in patients with sepsis and septic shock is a crucial task for critical care medicine.
Hydrocortisone combined with vitamin C and vitamin B1 (combination therapy) is a promising treatment for septic shock. Marik and colleagues explored the effect of the combination therapy on mortality in patients with septic shock for the first time, revealing that the combination therapy significantly reduces the in-hospital mortality, sequential organ failure assessment (SOFA) score, and duration of vasopressor use [3]. However, this study has some shortcomings, such as the small sample size and before-after study design. Since the publication of this study, the use of combination therapy has increased gradually [4], and numerous studies have begun to explore the effect of combination therapy on the prognosis of septic shock patients [5–14]. But these studies have produced inconsistent results and a lack of mechanistic research.
Dysregulated host response to infection can cause endothelial cell death, loss of endothelial barrier integrity, endothelial dysfunction, disseminated intravascular coagulation, and mitochondrial dysfunction. These microcirculation dysfunctions can lead to impaired tissue oxygenation and thus cause organ function injury. Therefore, endothelial and microcirculation dysfunction play key roles in the occurrence and development of septic shock. Theoretically, the combination therapy could protect endothelial function and microcirculation in many ways, including reducing endothelial apoptosis, maintaining endothelial integrity, immunomodulatory function, maintaining vasopressor responsiveness, improving mitochondrial function, and increasing lactate clearance. [15] Additionally, hydrocortisone and vitamin C have a synergistic effect on endothelial protection [16]. But there is a lack of studies to explore the effect of the combination therapy on microcirculation in patients with septic shock. This study aimed to observe the effects of the combination therapy versus hydrocortisone alone on sublingual microcirculation monitored by sidestream dark-field imaging in septic shock patients.
2Methods
2.1Study design
This pilot study used a prospective, double-blind, randomized, controlled trial method and aimed to evaluate the effects of hydrocortisone combined with vitamin C and vitamin B1 versus hydrocortisone alone on the perfused small vessel density (sPVD, sublingual microcirculation parameters monitored by sidestream dark-field imaging) in patients with septic shock. We randomly assigned the enrolled patients to the treatment group (hydrocortisone combined with vitamin C and vitamin B1 therapy added to standard care) and control group (hydrocortisone alone added to standard care) in a 1 : 1 ratio. The Clinical Research Ethics Committee of the Zhongda Hospital affiliated with Southeast University approved this study (2018ZDSYLL057-P01), and informed consent forms were signed by relatives of the participants by law. The clinical trial protocol was registered at clinicaltrial.gov (NCT03821714).
2.2Participants
From February 1, 2019, to January 1, 2020, we enrolled patients with septic shock admitted to the intensive care unit (ICU) of the Zhongda Hospital affiliated with Southeast University. All newly admitted patients were screened four times on the first day of ICU admission to determine whether they met the study’s inclusion criteria and ensure early enrollment. Because of the difficulty of screening late at night, the screening time points were 7 : 00, 13 : 00, 19 : 00, and 23 : 00. The main inclusion criteria were septic shock patients with norepinephrine doses≥15μg/min. According to the requirements of the ethics committee, the included patients were aged at least 18 years and had a maximum age of 80 years. The exclusion criteria included the following: uncontrolled malignancy; clinicians judging that glucocorticoid therapy should not be used; glucocorticoid therapy being used within seven days before admission; patients meeting the inclusion criteria for more than 12 hours; patients or relatives refusing to sign the informed consent form; patients expected to die within 24 hours. Sepsis was defined as an acute change in the total SOFA score≥2 points consequent to infection. Septic shock was defined as sepsis with persisting hypotension requiring vasopressors to maintain a mean arterial pressure (MAP)≥65 mmHg despite adequate volume resuscitation [17]. Some patients have lactate less than 2 mmol/L at enrollment due to the treatment with fluid resuscitation and norepinephrine after the diagnosis of septic shock.
2.3Randomization and blinding
We randomly assigned the enrolled patients to the treatment and control groups in a 1 : 1 ratio. We used a 1-100 random number table for simple randomized assignments. If the random number was odd, the patient was assigned to the treatment group; otherwise, the patient was assigned to the control group. Patients were numbered according to their enrollment order, and the random results of each patient were concealed by the envelope method. Screening researchers opened the envelope based on the patient’s enrollment number and then informed the treating nurse of the treatment plan. Finally, the treating nurse completed the medication according to the prescribed usage. Participants and microcirculation evaluators were blinded to the grouping scheme.
2.4Intervention
The treatment group was treated with hydrocortisone combined with vitamin C and vitamin B1 added to standard care. Hydrocortisone (200 mg) was continuously pumped intravenously for 24 hours. Vitamin C (1.5 g) was dissolved in 100 ml of normal saline and infused intravenously. The infusion time was 30-60 min, and the interval was 6 hours; 4 doses were used. Vitamin B1 (200 mg) was dissolved in 50 ml of normal saline and infused intravenously. The infusion time was 30-60 min, and the interval was 12 hours; 2 doses were used. The control group was treated with hydrocortisone alone added to standard care. The use of hydrocortisone was the same as that in the treatment group. Additionally, the same amount of saline as the treatment group was used as a control. Standard care was performed according to the international guidelines for the management of sepsis and septic shock, such as infection source control, fluid resuscitation, norepinephrine use, and antimicrobial use [18].
2.5Outcome
The primary outcome was the sPVD at 24 hours after treatment. We used a sublingual microcirculation imaging system to monitor the sPVD at baseline, 4 hours and 24 hours after treatment. Perfused small vessel was defined as blood vessel 0 to 20μm in diameter. The secondary outcomes included other sublingual microcirculation parameters at 24 hours after treatment (proportion of perfused small vessels [sPPV], small vessel density [sTVD], and microvascular flow index [MFI]). To further support the primary outcome, we performed renal contrast-enhanced ultrasound (CEUS) at baseline and 24 hours after treatment to observe the differences in renal perfusion between the treatment and control groups. Other secondary outcomes were renal CEUS parameters at 24 hours after treatment (peak intensity [PI], regional blood flow [RBF], time to peak [TTP], mean transit time [MTT]); lactate level at 24 hours after treatment; norepinephrine dose; ICU stay; total hospital stay; and 28-day mortality.
2.6Sublingual microcirculation monitoring
We used sublingual microcirculation imaging system (MicroSee, V100, Guangzhou Medsoft System Ltd., China) for microcirculation monitoring and followed the second consensus on the assessment of sublingual microcirculation in critically ill patients [19]. MicroSee is a kind of sidestream dark-field imaging system with image size 656×492 pixels, resolution 1.1μm/pixel, field of view 730×550μm, frame rate 30 frames/s.
Sublingual microcirculation was monitored at baseline, 4 hours, and 24 hours after treatment. After evaluating the safety of endotracheal intubation, we used cotton balls to clean the patient’s oral secretions. Then, the probe was placed under the tongue to capture microcirculation images. At each time point, we collected images of sublingual microcirculation at 3-5 locations. In the final data analysis, the microcirculation parameters were averaged. The duration of each image was greater than 4 seconds. To ensure good image quality, we scored each image according to the scoring system recommended by the second consensus on the assessment of sublingual microcirculation in critically ill patients. A score greater than 6 indicates that the image is unqualified.
After images were collected, two trained image analysts used the MircoSee Information Management Software (MicroSee, V100, Guangzhou Medsoft System Ltd., China) to independently analyze the microcirculation images. The final parameters were averaged. The image analysis process is shown below.
Firstly, we described each vessel manually. Secondly, we assigned a value to each blood vessel. Vessels with continuous blood flow or blood flow time greater than 50% were labeled as perfused vessels. Vessels with no blood flow and blood flow time less than 50% were labeled as nonperfused vessels. Then, small vessel density (sTVD), proportion of perfused small vessels (sPPV) and perfused small vessel density (sPVD) can be calculated automatically. As small vessels are directly involved in tissue perfusion and substance exchange, the microcirculation parameters of small vessels were used for the final data analysis. Small vessels were defined as blood vessels 0 to 20μm in diameter. Thirdly, we calculated microvascular flow index (MFI) by the four-quadrant method. For each quadrant, stop flow was marked with a score of 0; intermittent flow was marked with a score of 1; sluggish flow was marked with a score of 2; normal flow was marked with a score of 3. MFI was the average red blood cell velocity per quadrant.
2.7Renal contrast-enhanced ultrasound monitoring
We used renal contrast-enhanced ultrasound (CEUS) to investigate the effects of hydrocortisone combined with vitamin C and vitamin B1 versus hydrocortisone alone on renal perfusion to support the primary outcome. The Esaote MyLab Twice ultrasound with a CA541 convex probe was used to perform CEUS, and Sonovue (Bracco company, Italy) was used as the contrast agent. CEUS was monitored at baseline and 24 hours after treatment. At each time point, we collected CEUS images of the bilateral kidneys. The time between the two collections was at least 15 minutes. In the final data analysis, CEUS parameters were averaged. Besides, the CEUS image analyst was blinded to the grouping scheme.
The process of CEUS was described as follows. First, we dissolved a sonovue in 5 mL normal saline. The dose of sonovue used in a single CEUS was 0.02 mL/kg. Then, under two-dimensional ultrasound, the long axis of the kidney was shown. We injected the contrast agent and started the CEUS examination. The machine parameters were selected as frequency 10 Hz, mechanical index 0.07, gray scale 90%, and focusing depth 5 cm. The CEUS images were collected continuously for 120 seconds. Finally, we used the analysis software of the ultrasound machine to perform contrast-enhanced ultrasound analysis and obtain the parameters of renal perfusion.
2.8Statistical analysis
This study was a parallel randomized controlled trial. The treatment group was treated with hydrocortisone combined with vitamin C and vitamin B1 added to standard care, and the control group was treated with hydrocortisone alone added to standard care. The primary outcome was perfused small vessel density (sPVD) at 24 hours after treatment. We conducted a preliminary study involving four septic patients, two in the treatment group and two in the control group. The difference in sPVD at 24 hours after treatment between the treatment group and the control group was 4.21 mm/mm2, and the standard deviation (SD) was 2.93 mm/mm2. Set α=0.05 and β=0.2, the calculated sample size was 9 in each group. The loss of follow-up rate was set as 10%, and the final sample size was 10 cases per group.
Continuous variables were shown as the mean±standard deviation(SD) or median (interquartile range) according to the data distribution. Categorical variables were shown as numbers and percentages. For repeated measurement data (measurement number≥3), we used two-way repeated-measures ANOVA to determine the influence of different interventions on microcirculation over time. If Mauchly’s test of sphericity was not satisfied, we used the Greenhouse-Geisser method for correction. For data measured before and after treatment (measurement number = 2), we used baseline data as covariates and used covariance analysis to compare posttreatment differences between the treatment and control groups. We used either the independent samples t-test or Mann-Whitney U test for continuous variables to compare the baseline characteristics of patients in the treatment and control groups and Fisher’s exact test for categorical variables. Two-sided P values less than 0.05 were considered statistically significant. Statistical analysis was performed using SPSS (Version 23.0; IBM Corp.) and R (Version 3.6.3; R Project for Statistical Computing).
3Results
3.1Participants
We screened 108 patients with septic shock. Among them, 27 patients were randomized after excluding 81 patients. The main reasons for exclusions were as follows: a norepinephrine dose less than 15μg/min, age older than 80 years, malignancy, and clinicians judging that hydrocortisone should not be used. Among the randomized patients, 15 were allocated to the treatment group, and 12 to the control group. All the randomized patients received the allocated intervention. In the treatment group, sublingual microcirculation was not monitored in 2 patients because of ventilation in the prone position, and 1 patient discontinued intervention because of an ICU stay of less than 24 hours. In the control group, sublingual microcirculation was not monitored in 2 patients because of ventilation in the prone position. Finally, 12 patients in the treatment group and 10 patients in the control group completed the study and were included in the analysis (Fig. 1).
Fig. 1
Flow diagram.
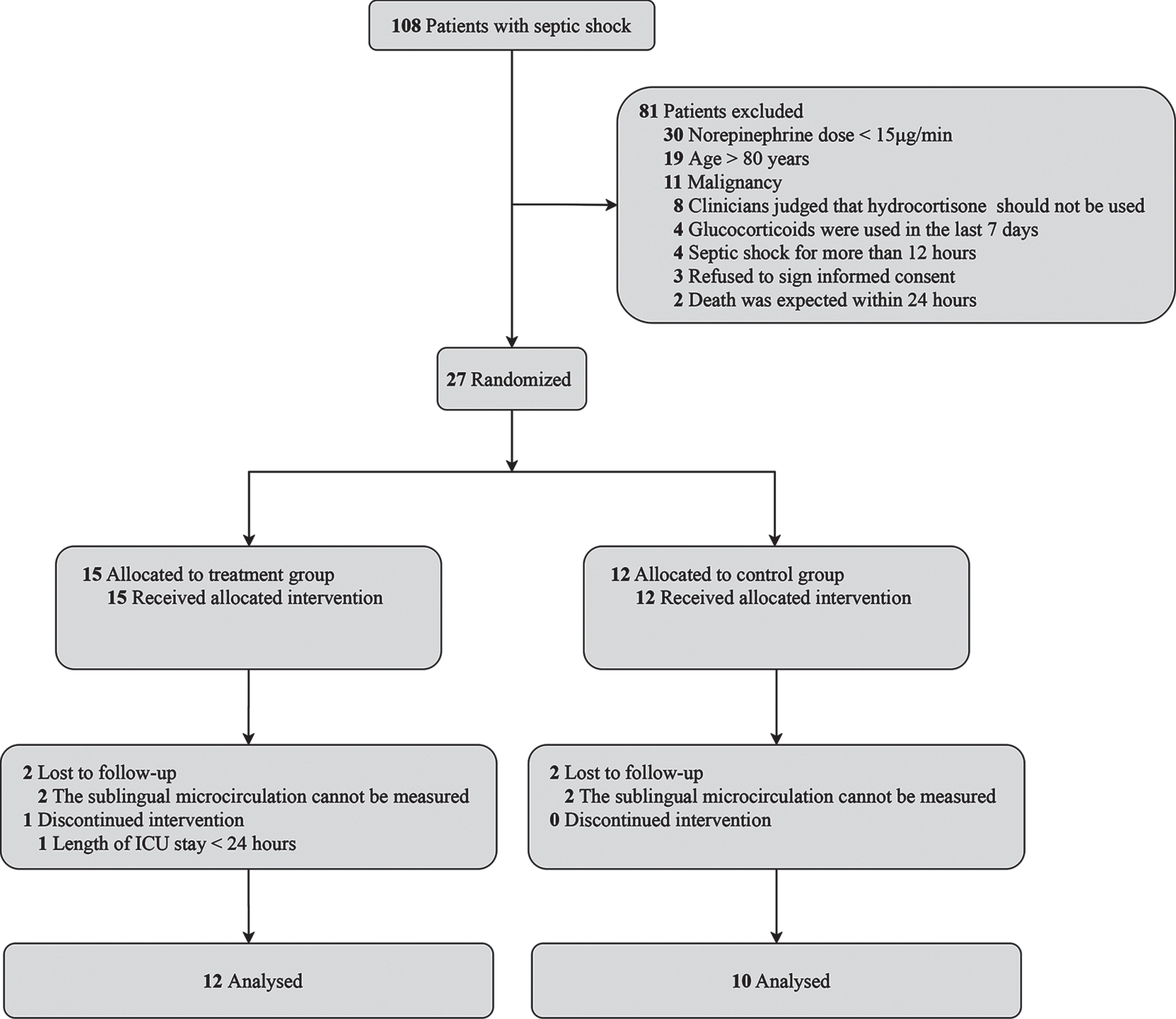
No significant differences were found in sex, age, height, weight, comorbidities, infection sites, disease severity, fluid management, or laboratory parameters between the treatment and control groups (Table 1). Among the included patients, 15 (68.2%) were male, and the mean age was 57 years. The main comorbidities were hypertension, cerebrovascular disease, diabetes, and coronary heart disease. The main sites of infection were the respiratory system and abdominal cavity. 19 (86.4%) patients received mechanical ventilation. No significant differences were found between the treatment and control groups in the length of ICU stay, total hospital stay, or 28-day mortality. No statistically significant differences were found in hemodynamics between the treatment and control groups at baseline, 4 hours and 24 hours after treatment (Table 2).
Table 1
Characteristics of the enrolled patients
Variables | Overall | Control group | Treatment group |
(n = 22) | (n = 10) | (n = 12) | |
Male, No. (%) | 15 (68.2) | 9 (90) | 6 (50) |
Age, years, mean±SD | 57±18 | 59±16 | 56±20 |
Height, cm, mean±SD | 167±7 | 171±6 | 166±7 |
Weight, kg, mean±SD | 66±12 | 70±12 | 63±11 |
Comorbidity, No. (%) | |||
Hypertension | 10 (45.5) | 3 (30) | 7 (58.3) |
Cerebrovascular disease | 7 (31.8) | 3 (30) | 4 (33.3) |
Diabetes | 4 (18.2) | 1 (10) | 3 (25) |
Coronary heart disease | 3 (13.6) | 2 (20) | 1 (8.3) |
The site of infection, No. (%) | |||
Respiratory system | 11 (50) | 5 (50) | 6 (50) |
Abdominal cavity | 10 (45.5) | 5 (50) | 5 (41.7) |
Urinary system | 1 (4.5) | 0 (0) | 1 (8.3) |
Mechanical ventilation, No. (%) | 19 (86.4) | 8 (80) | 11 (91.7) |
APACHE II score, mean±SD | 21.5±5.2 | 20.4±6.1 | 22.8±3.8 |
SOFA score, mean±SD | 8.5±2.9 | 8.3±2.9 | 8.8±2.9 |
Norepinephrine dose, μg/kg/min, median (IQR) | 0.50 (0.36, 0.85) | 0.52 (0.30, 1.27) | 0.50 (0.38, 0.85) |
Fluid management | |||
Fluid intake at first 24 hours, mL, mean±SD | 4711±1983 | 4847±2136 | 4598±1935 |
Fluid output at first 24 hours, mL, mean±SD | 3531±2085 | 3732±2228 | 3365±2044 |
Laboratory parameters | |||
White blood cells,×109/L, mean±SD | 15.2±6.6 | 15.3±8.1 | 15.1±5.5 |
Procalcitonin, ng/mL, median (IQR) | 8.2 (2.8,17.5) | 8.8 (2.9,17.5) | 7.3 (3.8,14.1) |
C-reactive protein, mg/L, median (IQR) | 163 (95,186) | 158 (85,186) | 167 (103,180) |
Hemoglobin, g/L, mean±SD | 106±25 | 111±20 | 103±29 |
Platelet count,×109/L, median (IQR) | 128 (81,241) | 139 (85,218) | 128 (70,266) |
PT, S, mean±SD | 15.2±2.3 | 15.6±2.9 | 14.8±1.6 |
APPT, S, median (IQR) | 37 (33,52) | 37 (33,40) | 36 (34,60) |
FDP, μg/mL, median (IQR) | 10.4 (8.8,16) | 9.9 (8.7,13.6) | 13 (9.8,21.7) |
D-Dimer, mg/L, median (IQR) | 1.44 (1.14,2.59) | 1.21 (1.10,3.58) | 1.60 (1.13,2.30) |
Lactate, mmol/L, median (IQR) | 3 (1.7,4.1) | 3.2 (2.4,5.2) | 2.6 (1.5,3.8) |
ICU stay, days, median (IQR) | 7 (4,17) | 11 (4,19) | 7 (5,11) |
Hospital stay, days, median (IQR) | 15 (7,21) | 17 (12,20) | 12 (6,22) |
28-day mortality, No. (%) | 8 (36.4) | 4 (40) | 4 (33.3) |
APACHE II, Acute Physiology and Chronic Health Evaluation II; APTT, Activated partial thromboplastin time; FDP, Fibrin degradation products; ICU, intensive care unit; IQR, interquartile range; PT, Prothrombin time; SD, standard deviation; SOFA, Sequential Organ Failure Assessment.
Table 2
Hemodynamic characteristics of the patients
Variables | Group | Baseline | 4 hours after | 24 hours after |
treatment | treatment | |||
Heart rate, bpm, mean±SD | Treatment | 106±24 | 89±20* | 93±18* |
Control | 123±27 | 101±15 | 102±15 | |
CVP, mmHg, mean±SD | Treatment | 10±2 | 9±3 | 8±3* |
Control | 11±3 | 11±2 | 9±2 | |
MAP, mmHg, mean±SD | Treatment | 81±10 | 83±12 | 87±9 |
Control | 77±12 | 84±10 | 84±9 | |
CO, L/min, mean±SD | Treatment | 6.5±1.6 | 6.1±1.2 | 6.7±0.7 |
Control | 6.3±1.1 | 6.8±0.6 | 6.7±0.8 | |
GEDV, mL, mean±SD | Treatment | 1355±369 | 1349±400 | 1304±402 |
Control | 1255±197 | 1182±290 | 1240±228 | |
SVRI, dyn.s.cm-5.m2, mean±SD | Treatment | 1513±366 | 1662±498 | 1563±454 |
Control | 1508±283 | 1385±388 | 1340±297 |
Hemodynamic characteristics were monitored using the pulse-indicated continuous cardiac output. CO, cardiac output; CVP, central venous pressure; GEDV, global end-diastolic volume; MAP, mean arterial pressure; SD, standard deviation; SVRI, systemic vascular resistance index. *Compared with baseline, P < 0.05.
3.2Primary outcome
We scored the sublingual microcirculation images according to the second consensus on the assessment of sublingual microcirculation, and a score greater than 6 points indicated that image quality was substandard. A total of 282 images were collected, of which 15 were discarded and 267 were included in image analysis. The sublingual microcirculation image scores included in the final image analysis were fewer than 6 points (Supplementary Fig. 1).
The interaction of different interventions and time had a statistically significant effect on sPVD. No statistically significant difference was found in the sPVD between the treatment and control groups at baseline (mean difference, 2.603; 95% CI, -0.847-6.053; P = 0.122). The sPVD in the treatment group was significantly higher than that in the control group at 4 hours after treatment (mean difference, 7.042; 95% CI, 2.227-11.857; P = 0.009) and 24 hours after treatment (mean difference, 7.075; 95% CI, 2.390-11.759; P = 0.008) (Fig. 2). In the treatment group, the influence of time factor on sPVD was statistically significant (P = 0.033). In the control group, time factor had no statistically significant effect on sPVD (P = 0.773).
Fig. 2
Effects of hydrocortisone combined with vitamin C and vitamin B1 versus hydrocortisone alone on microcirculation in septic shock patients. A shows the effect on sPVD. B shows the effect on sPPV. C shows the effect on sTVD. D shows the effect on MFI. sPVD, Perfused small vessel density; sPPV, Proportion of perfused small vessel; sTVD, Small vessel density; MFI, Microvascular flow index. The error bars indicates standard deviation. *Compared with the control group, P < 0.05.
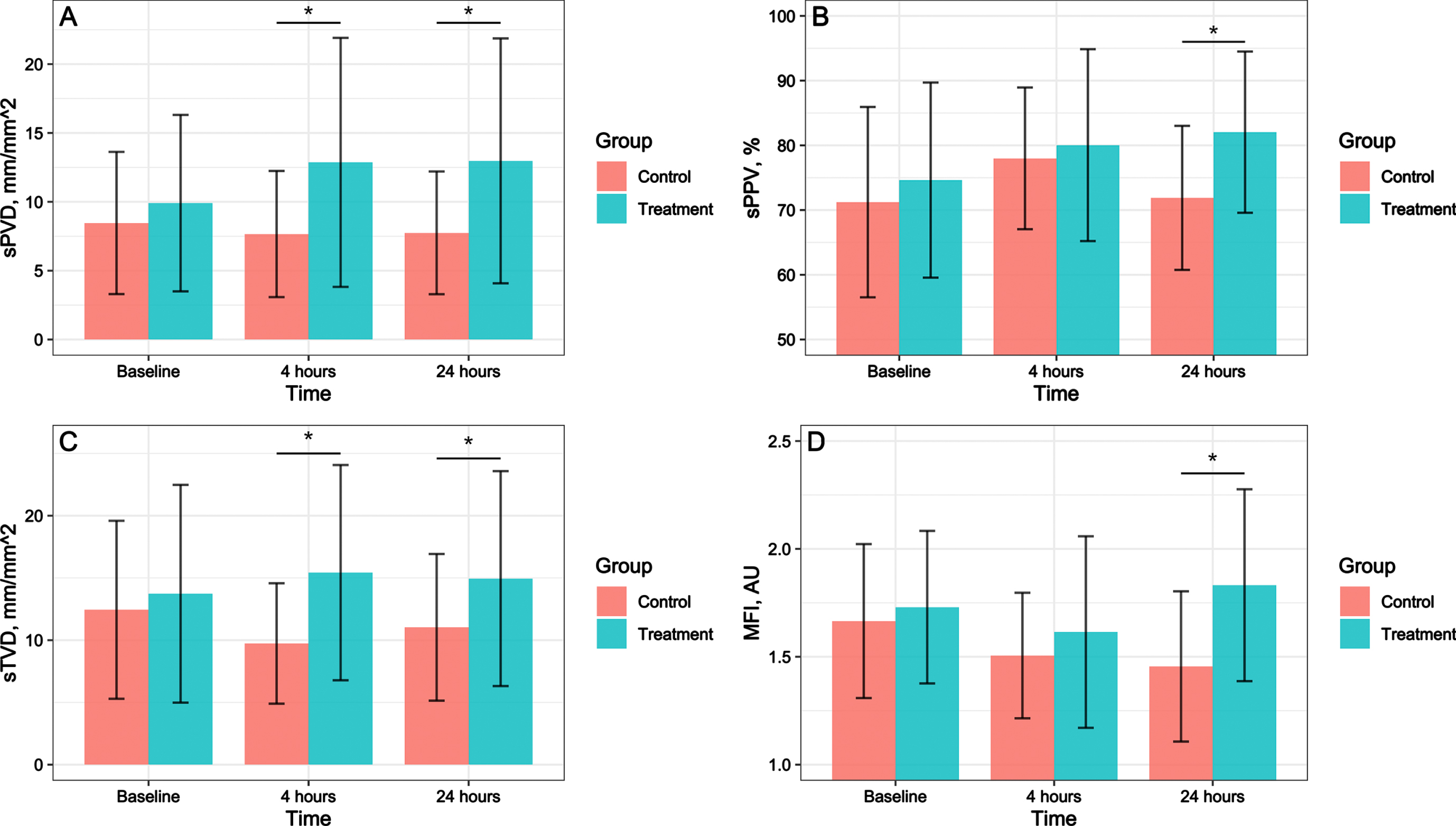
3.3Secondary outcomes
No statistically significant differences were found in the sPPV, sTVD, or MFI between the treatment and control groups at baseline. The sPPV (mean difference, 13.490; 95% CI, 2.798-24.182; P = 0.019), sTVD (mean difference, 5.659; 95% CI, 1.340-9.979; P = 0.016), and MFI (mean difference, 0.459; 95% CI, 0.033-0.885; P = 0.037) were significantly higher in the treatment group than in the control group at 24 hours after treatment (Fig. 2).
After adjusting for the PI and RBF at baseline, we observed that the PI (mean difference, 8.151; 95% CI, 2.748-13.553; P = 0.005) and RBF (mean difference, 9.402; 95% CI, 3.092-15.712; P = 0.006) in the treatment group were significantly higher than those in the control group at 24 hours after treatment. No statistically significant differences were found in the TTP, or MTT at 24 hours after treatment in the treatment group compared with that in the control group after adjusting for baseline value (Fig. 3).
Fig. 3
Effects of hydrocortisone combined with vitamin C and Vitamin B1 versus hydrocortisone alone on renal perfusion in septic shock patients. A shows the effect on PI. B shows the effect on RBF. C shows the effect on TTP. D shows the effect on MTT. MTT, mean transit time; PI, peak intensity; RBF, regional blood flow; TTP, time to peak. The error bars indicates standard deviation. *Compared with the control group, P < 0.05.
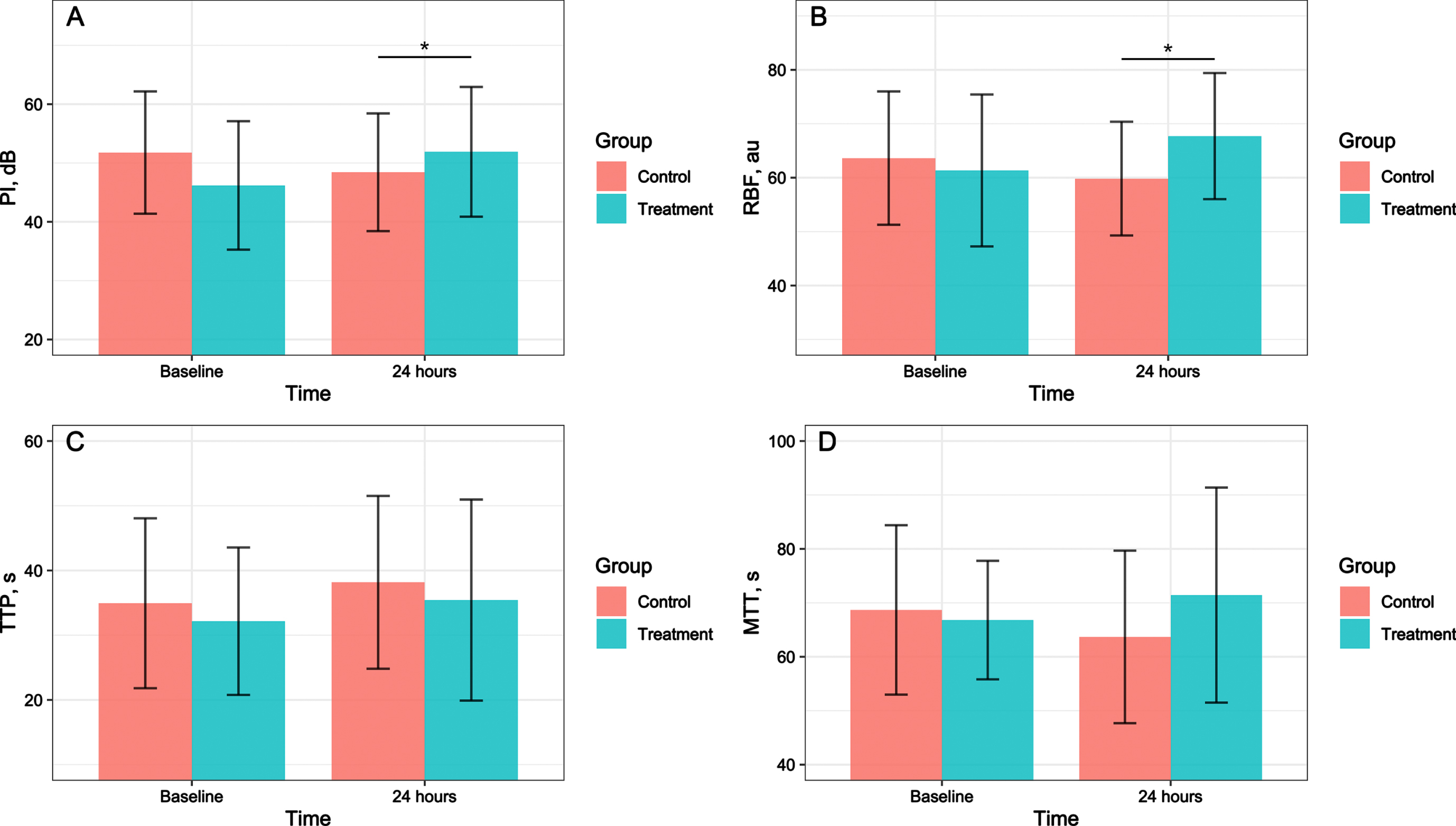
After adjusting for the lactate levels at baseline, the lactate levels were significantly lower in the treatment group than in the control group at 24 hours after treatment (mean difference, 2.839; 95% CI, 0.280-5.398; P = 0.032) (Fig. 4). No significant differences were found in the norepinephrine dose between the treatment and control groups at baseline, 4 hours, and 24 hours after treatment (Supplementary Fig. 2).
Fig. 4
Effect of hydrocortisone combined with vitamin C and Vitamin B1 versus hydrocortisone alone on lactate in septic shock patients. Boxplot indicates median (interquartile range) with maximum and minimum values. *Compared with the control group, P < 0.05.
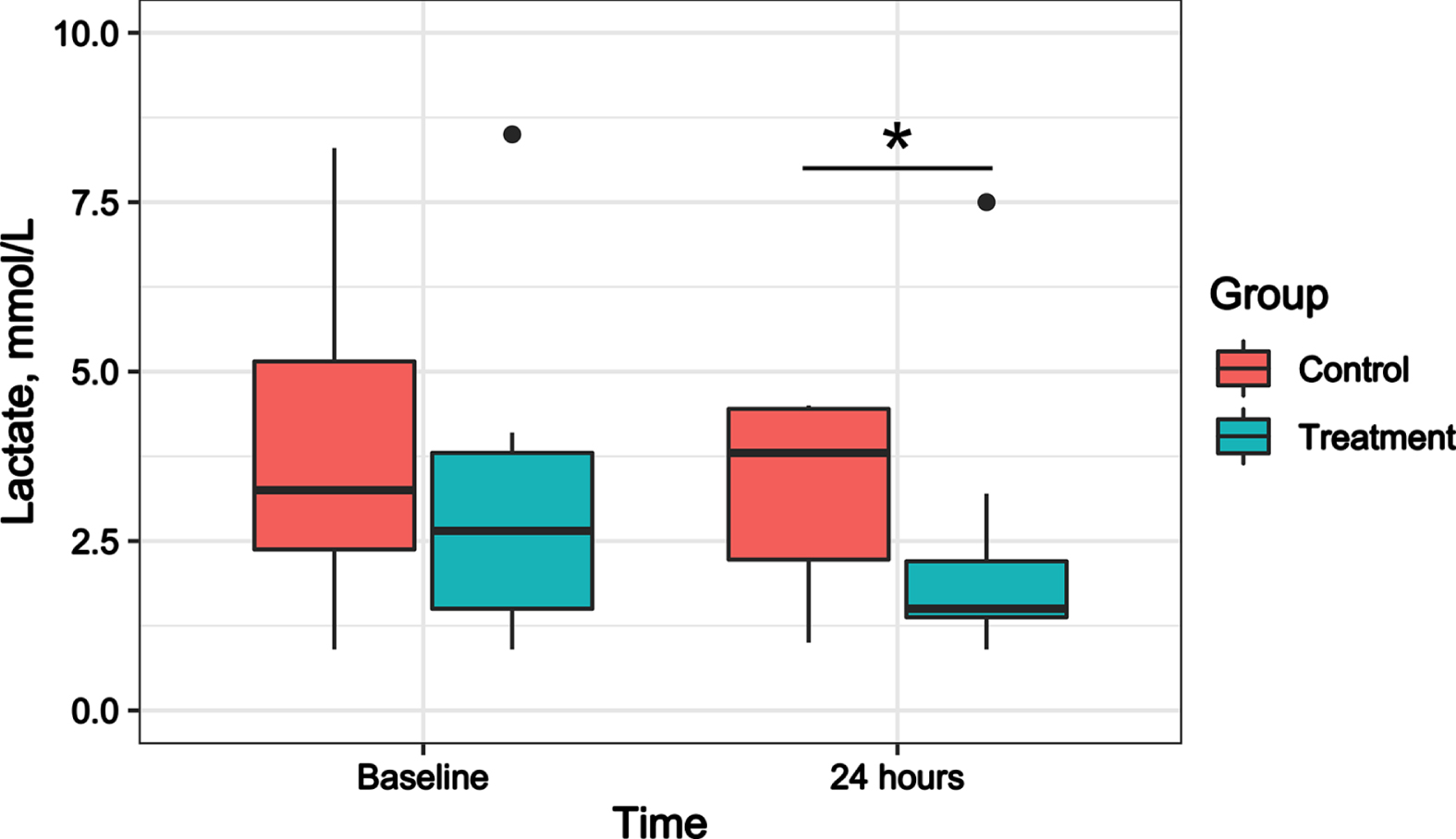
4Discussion
This pilot study aimed to observe the effects of hydrocortisone combined with vitamin C and vitamin B1 versus hydrocortisone alone on sublingual microcirculation in patients with septic shock. The sublingual microcirculation in the treatment group was significantly better than that in the control group at 24 hours after treatment. It suggests that combination therapy can protect microcirculation. The results were further supported by the protective effect of the combination therapy on renal perfusion monitored by renal CEUS.
Septic shock is associated with micro thrombosis, impaired endothelial barrier function, microvascular abnormalities, and mitochondrial dysfunction [20–22]. Hydrocortisone combined with vitamin C and vitamin B1 protects endothelial function and improves microcirculation through multiple pathways. First, the antioxidant effect of vitamin C can reduce the permeability of endothelial cells, protect the function of microcirculation and reduce apoptosis by maintaining endothelial integrity [23–25]. Second, vitamin C has an immunomodulatory function, which reduces the production of inflammatory mediators and regulates the function of macrophages [26, 27]. Third, vitamin C plays an important role in the generation of vasopressors and in maintaining vasopressor responsiveness [28]. Fourth, vitamin B1 is a key component of cell metabolism and can improve mitochondrial function, increase lactate clearance and protect organ function [29, 30]. However, vitamin C and vitamin B1 deficiency is common in septic shock patients and is associated with a poor prognosis [31, 32]. Therefore, supplementation with vitamin C and vitamin B1 in septic shock patients protects endothelial barrier function and improves microcirculation.
Hydrocortisone and vitamin C have synergistic effects on protecting endothelial barrier function and microcirculation. First, oxygen-free radicals reduce the activity of glucocorticoid receptors, and vitamin C may protect and restore glucocorticoid receptor function. Second, vitamin C uptake by cells is mediated by the sodium-vitamin C transporter 2 (SVCT2). However, SVCT2 is downregulated in septic shock, and glucocorticoids increase the expression of SVCT2 [33]. Thus, the combination of hydrocortisone and vitamin C can improve their physiological effects on protecting endothelial cells and microcirculation.
Current studies have drawn inconsistent conclusions [34]. In the VITAMINS study, the combination therapy did not reduce the 90-day mortality but reduced the SOFA score at day 3 in septic shock patients [11]. In the ACTS trial, the combination therapy did not result in a statistically significant reduction in the SOFA score during the first 72 hours after enrollment and 30-day mortality [12]. However, the subsequent meta-analysis showed that vitamin C alone or in combination with hydrocortisone/thiamine (treatment 3 to 4 days) reduces the 30-day mortality in patients with septic shock [5]. The heterogeneity of these results may be related to the differences in the efficacy of the combination therapy for different septic shock subtypes. A retrospective cohort study explored the effects of combination therapy on different septic shock subtypes and found that clinical outcomes may be better for patients with hyperinflammatory subtypes [35]. Additionally, procalcitonin may be an effective biomarker for monitoring the efficacy of the combination therapy [36]. Our study supports using combination therapy in septic patients because of its protective effect on microcirculation.
This study has some strengths. First, it used a prospective randomized controlled study design to ensure patient comparability between the treatment and control groups. We concealed the randomized results to avoid patient selection bias. Second, two researchers independently analyzed the microcirculation images, and we blinded microcirculation evaluators to the grouping scheme. We scored all microcirculation images to evaluate image quality and eliminated substandard images. Third, we performed bedside monitoring of renal perfusion using renal CEUS and compared the effects of different treatments on renal perfusion to support the primary outcome.
The limitations of this study were as follows. First, this was a single-center study with a small sample size, characteristics that could affect the generality of the findings. A large randomized controlled trial was needed to verify the conclusions. Second, our grouping scheme did not blind clinicians. Each patient was comanaged by at least three clinicians, and no conflict of interest was found between the clinicians and the study. Third, we did not consider other vasoactive agent doses (epinephrine, dopamine, and vasopressin) when comparing the effects of different treatments on norepinephrine doses. Of the 22 patients included in the analysis, only one in the control group received epinephrine 24 hours after treatment. Fourth, according to the study design, the duration of the three drugs was 24 hours. It makes this study insufficient to evaluate the effects of the combination therapy on clinical outcomes, such as ICU mortality. Fifth, we did not monitor the plasma vitamin C and vitamin B1 levels.
5Conclusions
Compared with hydrocortisone alone, the combination therapy significantly improved microcirculation in septic shock patients. This finding is further supported by improved renal perfusion in the treatment group. Large randomized controlled trials are needed to explore the effect of the combination therapy on outcome in septic shock patients and determine which subgroup of septic shock is more likely to respond to the combination therapy.
Acknowledgments
We sincerely thank all patients who participated in this study. We thank Bingjie Yang and Xiaoling Lu (Department of Ultrasound Medicine, Zhongda Hospital, School of Medicine, Southeast University) for helping us perform the renal contrast-enhanced ultrasound.
Authors’ contributions
JLW, LLH, QL, JYX, JFX, and YZH contributed to the conception of the study; JLW, QWS, SHY, HFW, and SSM contributed to data collection; JLW, QWS, SHY, HFW, and YZH contributed to data analysis and interpretation; JLW drafted the manuscript; and JLW, QWS, SHY, HFW, SSM, LLH, QL, JYX, JFX, and YZH revised the manuscript. All authors read and approved the final manuscript.
Supplementary material
[1] The supplementary material is available in the electronic version of this article: https://dx.doi.org/10.3233/CH-221444.
References
[1] | Rudd KE , Johnson SC , Agesa KM , Shackelford KA , Tsoi D , Kievlan DR , et al. Global, regional, and national sepsis incidence and mortality, -analysis for the Global Burden of Disease Study. Lancet. (2020) ;395: (10219):200–11. 10.1016/s0140-6736(19)32989-7. |
[2] | Xie J , Wang H , Kang Y , Zhou L , Liu Z , Qin B , et al. The Epidemiology of Sepsis in Chinese ICUs: A National Cross-Sectional Survey. Crit Care Med. (2020) ;48: (3):e209–e18. 10.1097/ccm.0000000000004155. |
[3] | Marik PE , Khangoora V , Rivera R , Hooper MH , Catravas J . Hydrocortisone, Vitamin C, and Thiamine for the Treatment of Severe Sepsis and Septic Shock: A Retrospective Before-After Study. Chest. (2017) ;151: (6):1229–38. 10.1016/j.chest.2016.11.036. |
[4] | Vail EA , Wunsch H , Pinto R , Bosch NA , Walkey AJ , Lindenauer PK , et al. Use of Hydrocortisone, Ascorbic Acid, and Thiamine in Adults with Septic Shock. Am J Respir Crit Care Med. (2020) ;202: (11):1531–9. 10.1164/rccm.202005-1829OC. |
[5] | Scholz SS , Borgstedt R , Ebeling N , Menzel LC , Jansen G , Rehberg S . Mortality in septic patients treated with vitamin C: a systematic meta-analysis. Crit Care. (2021) ;25: (1):17. 10.1186/s13054-020-03438-9. |
[6] | Wani SJ , Mufti SA , Jan RA , Shah SU , Qadri SM , Khan UH , et al. Combination of vitamin C, thiamine and hydrocortisone added to standard treatment in the management of sepsis: results from an open label randomised controlled clinical trial and a review of the literature. Infect Dis (Lond). (2020) ;52: (4):271–8. 10.1080/23744235.2020.1718200. |
[7] | Sadaka F , Grady J , Organti N , Donepudi B , Korobey M , Tannehill D , et al. Ascorbic Acid, Thiamine, and Steroids in Septic Shock: Propensity Matched Analysis. J Intensive Care Med. (2020) ;35: (11):1302–6. 10.1177/0885066619864541. |
[8] | Mitchell AB , Ryan TE , Gillion AR , Wells LD , Muthiah MP . Vitamin C and Thiamine for Sepsis and Septic Shock. Am J Med. (2020) ;133: (5):635–8. 10.1016/j.amjmed.2019.07.054. |
[9] | Chang P , Liao Y , Guan J , Guo Y , Zhao M , Hu J , et al. Combined Treatment With Hydrocortisone, Vitamin C, and Thiamine for Sepsis and Septic Shock: A Randomized Controlled Trial. Chest. (2020) ;158: (1):174–82. 10.1016/j.chest.2020.02.065. |
[10] | Coloretti I , Biagioni E , Venturelli S , Munari E , Tosi M , Roat E , et al. Adjunctive therapy with vitamin c and thiamine in patients treated with steroids for refractory septic shock: A propensity matched before-after, case-control study. J Crit Care. (2020) ;59: :37–41. 10.1016/j.jcrc.2020.04.014. |
[11] | Fujii T , Luethi N , Young PJ , Frei DR , Eastwood GM , French CJ , et al. Effect of Vitamin C, Hydrocortisone, and Thiamine vs Hydrocortisone Alone on Time Alive and Free of Vasopressor Support Among Patients With Septic Shock: The VITAMINS Randomized Clinical Trial. Jama. (2020) ;323: (5):423–31. 10.1001/jama.2019.22176. |
[12] | Moskowitz A , Huang DT , Hou PC , Gong J , Doshi PB , Grossestreuer AV , et al. Effect of Ascorbic Acid, Corticosteroids, and Thiamine on Organ Injury in Septic Shock: The ACTS Randomized Clinical Trial. Jama. (2020) ;324: (7):642–50. 10.1001/jama.2020.11946. |
[13] | Litwak JJ , Cho N , Nguyen HB , Moussavi K , Bushell T . Vitamin C, Hydrocortisone, and Thiamine for the Treatment of Severe Sepsis and Septic Shock: A Retrospective Analysis of Real-World Application. J Clin Med. (2019) ;8: (4). 10.3390/jcm8040478. |
[14] | Chang K , Harbin M , Shuster C , Griesdale DEG , Foster D , Sweet D , et al. Adding vitamin C to hydrocortisone lacks benefit in septic shock: a historical cohort study. Can J Anaesth. (2020) ;67: (12):1798–805. 10.1007/s12630-020-01814-1. |
[15] | Marik PE . Vitamin C for the treatment of sepsis: The scientific rationale. Pharmacol Ther. (2018) ;189: :63–70. 10.1016/j.pharmthera.2018.04.007. |
[16] | Barabutis N , Khangoora V , Marik PE , Catravas JD . Hydrocortisone and Ascorbic Acid Synergistically Prevent and Repair Lipopolysaccharide-Induced Pulmonary Endothelial Barrier Dysfunction. Chest. (2017) ;152: (5):954–62. 10.1016/j.chest.2017.07.014. |
[17] | Singer M , Deutschman CS , Seymour CW , Shankar-Hari M , Annane D , Bauer M , et al. The Third International Consensus Definitions for Sepsis and Septic Shock (Sepsis-3). JAMA. (2016) ;315: (8):801–10. 10.1001/jama.2016.0287. |
[18] | Rhodes A , Evans LE , Alhazzani W , Levy MM , Antonelli M , Ferrer R , et al. Surviving Sepsis Campaign: International Guidelines for Management of Sepsis and Septic Shock: 2016. Intensive Care Med. (2017) ;43: (3):304–77. 10.1007/s00134-017-4683-6. |
[19] | Ince C , Boerma EC , Cecconi M , De Backer D , Shapiro NI , Duranteau J , et al. Second consensus on the assessment of sublingual microcirculation in critically ill patients: results from a task force of the European Society of Intensive Care Medicine. Intensive Care Med. (2018) ;44: (3):281–99. 10.1007/s00134-018-5070-7. |
[20] | Angus DC , van der Poll T . Severe sepsis and septic shock. N Engl J Med. (2013) ;369: (9):840–51. 10.1056/NEJMra1208623. |
[21] | Lelubre C , Vincent JL . Mechanisms and treatment of organ failure in sepsis. Nat Rev Nephrol. (2018) ;14: (7):417–27. 10.1038/s41581-018-0005-7. |
[22] | Brealey D , Brand M , Hargreaves I , Heales S , Land J , Smolenski R , et al. Association between mitochondrial dysfunction and severity and outcome of septic shock. Lancet. (2002) ;360: (9328):219–23. 10.1016/s0140-6736(02)09459-x. |
[23] | Oudemans-van Straaten HM , Spoelstra-de Man AM , de Waard MC . Vitamin C revisited. Crit Care. (2014) ;18: (4):460. 10.1186/s13054-014-0460-x. |
[24] | Amrein K , Oudemans-van Straaten HM , Berger MM . Vitamin therapy in critically ill patients: focus on thiamine, vitamin C, and vitamin D. Intensive Care Med. (2018) ;44: (11):1940–4. 10.1007/s00134-018-5107-y. |
[25] | Moskowitz A , Andersen LW , Huang DT , Berg KM , Grossestreuer AV , Marik PE , et al. Ascorbic acid, corticosteroids, and thiamine in sepsis: a review of the biologic rationale and the present state of clinical evaluation. Crit Care. (2018) ;22: (1):283. 10.1186/s13054-018-2217-4. |
[26] | Victor VV , Guayerbas N , Puerto M , Medina S , De la Fuente M . Ascorbic acid modulates in vitro the function of macrophages from mice with endotoxic shock. Immunopharmacology. (2000) ;46: (1):89–101. 10.1016/s0162-3109(99)00162-9. |
[27] | Cárcamo JM , Pedraza A , Bórquez-Ojeda O , Golde DW . Vitamin C suppresses TNF alpha-induced NF kappa B activation by inhibiting I kappa B alpha phosphorylation. Biochemistry. (2002) ;41: (43):12995–3002. 10.1021/bi0263210. |
[28] | Carr AC , Shaw GM , Fowler AA , Natarajan R . Ascorbate-dependent vasopressor synthesis: a rationale for vitamin C administration in severe sepsis and septic shock? Crit Care. (2015) ;19: :418. 10.1186/s13054-015-1131-2. |
[29] | Donnino MW , Andersen LW , Chase M , Berg KM , Tidswell M , Giberson T , et al. Randomized, Double-Blind, Placebo-Controlled Trial of Thiamine as a Metabolic Resuscitator in Septic Shock: A Pilot Study. Crit Care Med. (2016) ;44: (2):360–7. 10.1097/ccm.0000000000001572. |
[30] | Ikeda K , Liu X , Kida K , Marutani E , Hirai S , Sakaguchi M , et al. Thiamine as a neuroprotective agent after cardiac arrest. Resuscitation. (2016) ;105: :138–44. 10.1016/j.resuscitation.2016.04.024. |
[31] | Mallat J , Lemyze M , Thevenin D . Do not forget to give thiamine to your septic shock patient! J Thorac Dis. (2016) ;8: (6):1062–6. 10.21037/jtd.2016.04.32. |
[32] | Carr AC , Rosengrave PC , Bayer S , Chambers S , Mehrtens J , Shaw GM . Hypovitaminosis C and vitamin C deficiency in critically ill patients despite recommended enteral and parenteral intakes. Crit Care. (2017) 21: (1):300. 10.1186/s13054-017-1891-y. |
[33] | Fujita I , Hirano J , Itoh N , Nakanishi T , Tanaka K . Dexamethasone induces sodium-dependant vitamin C transporter in a mouse osteoblastic cell line MC3T3-E1. Br J Nutr.. (2001) ;86: (2):145–9. 10.1079/bjn2001406. |
[34] | Iglesias J , Vassallo AV , Patel VV , Sullivan JB , Cavanaugh J , Elbaga Y . Outcomes of Metabolic Resuscitation Using Ascorbic Acid, Thiamine, and Glucocorticoids in the Early Treatment of Sepsis: The ORANGES Trial. Chest. (2020) ;158: (1):164–73. 10.1016/j.chest.2020.02.049. |
[35] | Kim WY , Jung JW , Choi JC , Shin JW , Kim JY . Subphenotypes in Patients with Septic Shock Receiving Vitamin C, Hydrocortisone, and Thiamine: A Retrospective Cohort Analysis. Nutrients. (2019) ;11: (12). 10.3390/nu11122976. |
[36] | Marik PE . Procalcitonin is an essential biomarker for hydrocortisone, ascorbic acid, and thiamine (HAT) therapy in patients with sepsis. Crit Care. (2019) ;23: (1):151. 10.1186/s13054-019-2445-2. |