Superior preservation of capillaries, myofibrils and mitochondria after long-term extracorporeal perfusion of free muscle flaps – A descriptive electron microscopy study
Abstract
BACKGROUND:
Extracorporeal perfusion (ECP) is a promising technique for prolonged tissue preservation, but might have side effects. For instance, increased radical oxygen species or capillary endothelial damage.
OBJECTIVE:
To assess ultra-morphological muscle damage during 36-hour ECP of porcine musculocutaneous flaps, hypothesizing that it would delay the onset of damage compared to static cold storage (SCS).
METHODS:
Bilateral flaps were retrieved from three Dutch Landrace pigs. Three flaps were preserved for 36 hours by hypothermic storage 4-6°C (control group) and three flaps by ECP with cooled University of Wisconsin solution. Muscle biopsies were taken at 0 h, 12 h and 36 h and assessed with transmission electron microscopy.
RESULTS:
Muscle architecture was best preserved by ECP, with a delayed onset and decreased severity of muscle damage. After 36 hours, damage was two-fold lower in ECP-flaps compared to SCS-flaps. Myofibril architecture was best preserved. Mitochondria were greatly preserved with swelling being the most prominent feature. Capillaries were moderately but differently damaged during ECP, with focal endothelial thinning as opposed to luminal obstruction in SCS-preserved flaps.
CONCLUSIONS:
This experiment described favourable cellular preservation of skeletal muscle flaps during ECP compared to SCS. Results showed less severe ultra-morphological damage and a later onset of damage.
Abbreviations
ECP | extracorporeal perfusion |
SCS | static cold storage |
EM | Electron microscopy |
UW | University of Wisconsin (preservation solution) |
1Introduction
Skeletal muscle is highly sensitive to ischemia. Its ischemic tolerance can be compared to that of the heart, with a maximum cold ischemia time of approximately six hours [1–3]. It therefore remains a challenge to safely prolong its tolerable ischemia time. The most important structures to preserve in skeletal muscle are mitochondria. Mitochondrial integrity is of high importance for muscle function. Damage to mitochondria causes a temporary or complete halt of muscle’s most crucial energy source: oxidative phosphorylation. With the resulting deficiency in ATP and glucose, metabolism will rapidly deteriorate as will the contractile function of myocytes [3, 4]. Another prerequisite during tissue preservation is the optimal preservation of capillary endothelial integrity, preventing vascular leaking and thrombosis [5, 6].
A promising technique for prolonged skeletal muscle preservation is continuous extracorporeal perfusion (ECP) or machine perfusion. Preservation by ECP has multiple benefits over static cold storage (SCS), such as the washout of toxic metabolites, buffering and cell-protecting characteristics of the preservation solutions and the ability to oxygenate or cool the tissue [7–9]. The overall effect is a reduction of ischemia-reperfusion injury to the tissue resulting in improved (functional) outcomes after tissue replantation [9, 10]. ECP of muscle tissue will predominantly be applicable in the clinical setting of preservation of composite tissues that contain a large degree of skeletal muscle. The technique might improve outcomes after revascularization of traumatic amputated extremities or after transplantation of vascularized composite allografts (e.g. hand- or face transplantation) [11–14]. Both are lengthy and complex procedures that push the boundaries of muscle preservation to its limits.
However, ECP might have potential side effects. Concerns are the development of radical oxygen species in case of oxygenated perfusion or the onset of damage to the endothelial cell lining [2]. Therefore, research aiming to gain more insight into the effects of perfusion with the goal to further improve perfusion conditions is desirable. The work of Kueckelhaus et al. has set a first step, when they compared 12 h ECP to 4 h SCS. They presented electron microscopy (EM) findings that were superior in the ECP group after 7 days of revascularization [15]. However, they presented descriptive outcomes and only assessed two time points (after preservation and at 7 days of revascularisation). Therefore, the exact extent of cellular damage during prolonged ECP and the timing of onset of this damage still remain unclear.
This descriptive experiment assessed the cellular changes in porcine musculocutaneous rectus abdominis flaps during ECP and SCS using transmission electron microscopy. These flaps provide a highly ischemia-sensitive model with optimal observational characteristics. The aim was to evaluate damage to myofibrils, mitochondria and capillaries over time, hypothesizing that ECP could delay the onset of skeletal muscle damage compared to SCS.
2Materials and methods
2.1Flap procurement
Bilateral free rectus abdominis muscle flaps measuring 9x12 cm were retrieved from three Dutch Landrace pigs (mean weight 57 kg, range 40–67 kg). All flaps received a flush with 100cc of heparin- solution (200E heparin in 100cc NaCl 0.9%) via the superior epigastric artery directly after pedicle transection to washout blood. One of the two flaps per pig was treated as control and was preserved for 36 hours in dry gauzes in a bag on ice slurry (SCS group, n = 3). The contralateral flap was preserved by ECP for 36 hours with University of Wisconsin (UW) solution with a temperature of 8–10°C (perfusion group, n = 3). The perfusion pressure was set to an in-line maximum of 50mmHg systolic pressure and the fluid was oxygenated with a carbogen mixture (95% O2/5% CO2). Flaps were randomly assigned to either of the storage groups. The use of animals was approved by the local and national animal experimentation committee (protocol-number 2016-0034-001) and was in accordance with the European Directive 2010/63/EU for the use and care of laboratory animals and ARRIVE guidelines [16].
2.2Transmission electron microscopy
Muscle biopsies were taken from pre-defined locations at three time points in the experiment: 1) T = 0; before vascular pedicle transection, serving as a normal control sample, 2) T = 12; at 12 h of flap preservation and 3) T = 36; at 36 h of flap preservation. Biopsies measured 2x4 mm and were stored in 2% Glutaraldehyde buffered with 0.1M Sodium,-Cacodylate pH 7.4 and postfixed in 0.5% Potassiumhexacyanoferrat (II)-Trihydrate in 1% Osmium tetroxide in Paladebuffer. Samples were dehydrated in ethanol, propylenoxid and embedded in Epon. Semithin sections (0.7μm) and ultrathin sections (80 nm) were cut using the Leica ultracut UC6 ultramicrotome. The ultrathin sections were examined using a Jeol TEM1400 transmission electron microscope. The electron microscopy images were photographed with a Catan digital micrograph camera. Five areas per biopsy were scored by two pathologists that were blinded to the method of flap preservation. A simple scoring system was designed to equally evaluate the samples, since none was available in literature. The degree of damage to myofibrils, mitochondria and capillaries were scored from 0 to 2 in this scoring system. A total score of 0 resembled no damage to the observed structure, a score of 2 resembled severe destruction (Table 1). A mean score (0 to 2) was calculated as a measure for overall muscle damage.
Table 1
Self-developed scoring system for evaluation of skeletal muscle damage with transmission electron microscopy
Score | 0 (no damage) | 1 (mild damage) | 2 (severe damage) |
Myofibrils | Normal architecture | Mild or focal disturbances to architecture (e.g. minor Z-band changes, decrease of glycogen storage) | Severe architectural disturbances (e.g. major Z-band changes, depletion of glycogen storage) |
Mitochondria | Normal size and shape | Some swelling, mild or focal signs of damage (e.g. vesiculation, distortion of cristae) | Severe swelling and destruction (e.g. rupture of membranes) |
Capillaries | No endothelial damage, open lumen | Some endothelial damage (e.g. small vacuoles or intraluminal debris) | Severe endothelial damage (e.g. loss of endothelial cell integrity, obstructed lumen) |
2.3Analysis
The EM scores were used to compare the degree of muscle damage in flaps taken from individual pigs, but also to compare the muscle damage between groups. Descriptive outcomes (mean, SD) were provided. Statistical analysis were not performed given the small number of flaps per groups.
3Results
Mean preservation time was 36.5 hours in the control group (SCS) and 36.7 hours in the ECP group. The perfusion pressure of 50mmHg during ECP corresponded to a mean perfusion flow of 8–10 ml/min. The mean initial flap weight was 245±73 g in the SCS group and 263±75 g in the ECP group. The flap weight increased with an average of 86 g (35±24%) during UW perfusion, there was no weight increase during SCS (–1.8±1.5%).
3.1Electron microscopy
There was more muscle damage after 12 and 36 hours in the flaps that were preserved by SCS compared to the contralateral flaps from the same pig that were preserved by ECP (Fig. 1). When comparing groups, the onset and severity of damage to mitochondria, myofibrils and capillaries were all decreased in the ECP group compared to the SCS group (Table 2). After 12 h of SCS, the mean injury score was 1.1, representing mild damage, which was most prominently present in capillaries and mitochondria. Characteristic findings at this timepoint were swelling or vesiculation of mitochondria, the presence of debris extending in the capillary lumen and mild Z-band changes in myofibrillar arrangement. In contrast, after 12 h ECP, the mean innjury score was 0.56, with mild focal thinning of the capillary endothelium as most prominent feature. After 36 h SCS, the ischemic damage further increased to a mean injury score of 1.3. EM images showed total mitochondrial degeneration and capillaries filled with debris and endothelial sheddings, obstructing the lumen (Fig. 2). Myofibril architecture was best preserved and mainly showed diffuse Z-band streaming, but in some areas also separation of cells. The mean injury score after 36 h ECP was 0.7. Mitochondria were greatly preserved throughout the entire 36-hour extracorporeal perfusion period; swelling was the only sign of damage. Capillaries were moderately damaged, with the main finding being focal endothelial thinning as opposed to luminal obstruction in the SCS group. Myofibril architecture was well preserved until the end of the experiment (Table 2, Fig. 2).
Fig. 1
Mean EM scores of flaps per group per timepoint during 36-hour flap preservation Scores: 0 = no damage, 1 = mild damage, 2 = severe damage. Dark bars represent SCS-preserved flaps, lighter bars represents the ECP-preserved flaps.
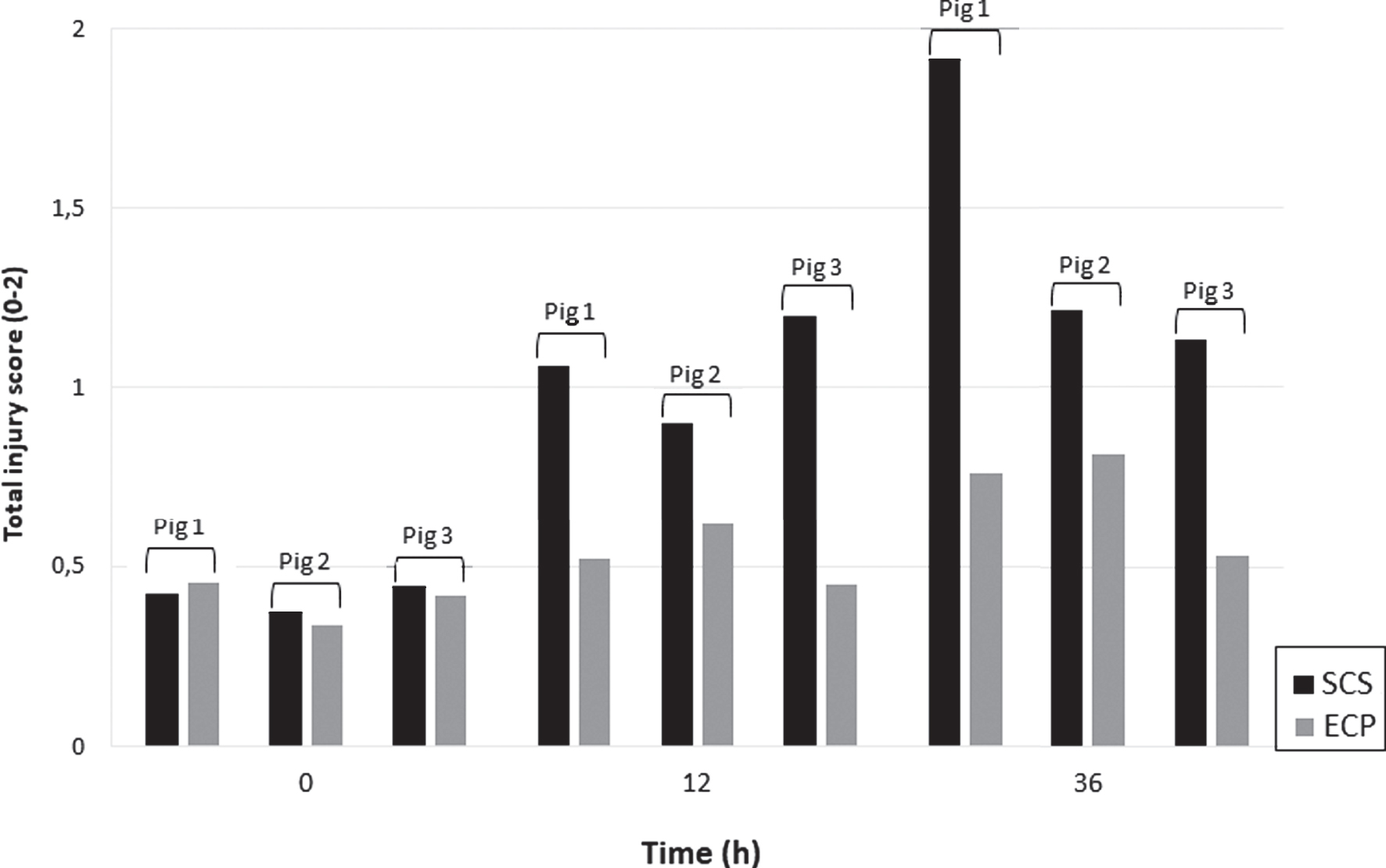
Fig. 2
Representative electron microscopy images of muscle biopsies taken during extracorporeal perfusion and static cold storage throughout the preservation period. Left top row: myofibrillar arrangement during ECP showing preserved architecture with only minor Z-band changes. Left middle row: mitochondria during ECP showing mild swelling over time, but no degeneration. Left bottom row: capillaries during ECP with focal thinning at 12 h and 36 h of preservation (1). Right top row: myofibrillar arrangement during SCS showing Z-band changes at 12 h (2) and severe distortion of arrangement at 36 h of preservation. Right middle row: mitochondria during SCS showing swelling at 12 h and destruction at 36 h with rupture of membrane and leaking of contents (3). Right bottom row: capillaries during SCS with vacuoles and debris extending into the lumen (4) at 12 h, evolving into luminal obstruction at 36 h of preservation.
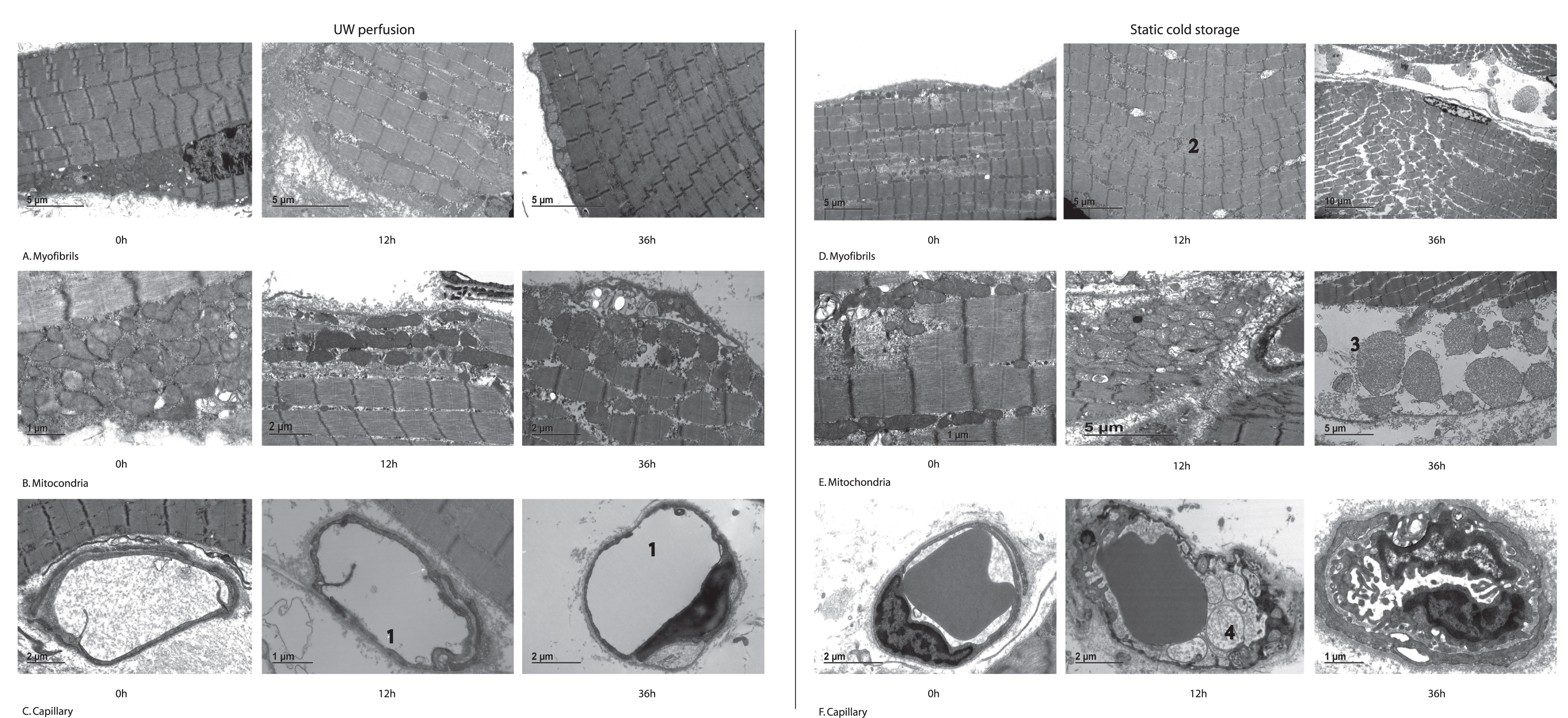
Table 2
EM scores per structure per timepoint during 36-hour flap preservation (score 0-2; Mean with SD)
Time point | Extracorporeal perfusion (n = 3) | Static cold storage (n = 3) | ||||||
(h) | Myofibrils | Mitochondria | Capillaries | Mean injury score | Myofibrils | Mitochondria | Capillaries | Mean injury score |
0 | 0.44 (0.24) | 0.28 (0.10) | 0.49 (0.31) | 0.4 | 0.45 (0.2) | 0.26 (0.09) | 0.50 (0.30) | 0.4 |
12 | 0.52 (0.24) | 0.34 (0.18) | 0.83 (0.06) | 0.6 | 0.75 (0.36) | 1.20 (0.02) | 1.21 (0.29) | 1.1 |
36 | 0.46 (0.16) | 0.58 (0.31) | 1.07 (0.30) | 0.7 | 0.94 (0.17) | 1.47 (0.59) | 1.44 (0.42) | 1.3 |
4Discussion
Following positive results in organ preservation, ECP found its way into the reconstructive research field over the past two decades. Despite of the growing interest in this technique, the evidence related to this topic is still limited [17].
This experiment is the first to describe cellular changes in skeletal muscle during ECP using transmission electron microscopy and provided a unique insight into the processes during ECP. A 36-hour preservation period of porcine skeletal muscle flaps was assessed and storage using ECP was compared to SCS. Results showed a drastic decrease in ischemic damage during ECP, both when comparing differently preserved flaps originating from the same pig as when comparing outcomes between groups as a whole. There was a more than two-fold decrease of muscle damage on EM evaluation when applying ECP. Myofibrils and mitochondria were affected less severely and much later during ECP compared to SCS. Capillaries seemed to be damaged less severely as well, but interestingly, the damage presented differently compared to SCS. These results provide further evidence towards ECP as a successful technique for long-term skeletal muscle/VCA preservation.
Myofibrils were the best preserved structure during preservation, and pronounced damage was only visible after 36 hours of SCS but not during ECP. The pattern of ischemic damage to myofibrils agrees to the descriptions of ischemic changes in rabbit and rat limbs by Harman [4]. Increasing with time, structural ischemic changes consisted of Z-band streaming, hypercontraction and eventually depletion of the glycogen storage and separation of the cells by structureless spaces.
Mitochondria were greatly damaged during SCS, already showing profound damage at 12 h follow-up. The first signs of damage were mitochondrial swelling with distention of cristae, later followed by disruption of membranes and loss of cytosolic contents [15]. Several factors account for mitochondrial ischemic damage; opening of universal membrane transporters causing ion shifts, an increased cytosolic calcium concentration and increased levels of reactive oxygen species [2, 18]. The most common sign of damage after 36 hours of ECP was mitochondrial swelling, without destruction of mitochondrial architecture. Therefore it can be concluded that oxygenated ECP seems to have a protective rather than a destructive effect on mitochondrial preservation.
Capillary damage during SCS typically initiated with finger like protrusions of cell membrane extending into the lumen (12 h of preservation) that evolved into complete capillary obstruction over time (36 h of preservation) [15]. Blaisdell described this phenomenon in 2002 and the accompanying ‘no reflow phenomenon’ that was clinically present upon reperfusion [1]. Kueckelhaus et al. described disintegration of the endothelial lining after a period of only 4 hours of SCS of porcine limbs. In contrast, they found no signs of capillary damage after 12 hours of acellular ECP or reperfusion in their experiment [15]. In this set-up, all capillary lumens remained open during the entire ECP period. However, a feature that typically presented at≥12 hours of ECP was a focal capillary wall thinning. Usually one or two spots per capillary were affected, without complete endothelial wall damage. The underlying mechanism of this capillary wall thinning remains unclear and to our knowledge, this feature has not yet been described in literature before. Our hypothesis is that continuous artificial perfusion flow is causing mechanical stress on the vessel walls.
The currently used arterial perfusion pressure of 50mmHg might be too high for ECP of free flaps. This might have contributed to the mean weight increase of 35% of initial flap weight after 36 h ECP. Kueckelhaus et al. used a maximum perfusion pressure of 30mmHg and had a mean weight increase of only 10% after 12 h ECP [15]. On the other end of the spectrum, Taeger et al. perfused porcine rectus abdominis flaps with pressures around 95mmHg and experienced a weight gain of 84% after 6 hours of ECP [19]. It would be interesting to see how the characteristic capillary wall thinning would evolve after lowering the pressure during ECP, and if endothelial integrity will either restore or further damage after flap reperfusion with blood. Further experiments should therefore focus on optimizing perfusion pressure, assessing capillary wall thinning and weight increase.
This study is limited by its group size, but more importantly by the absence of a validated evaluation scale for skeletal muscle damage on electron microscopy [15]. With the goal to uniformly evaluate the muscle biopsies and to quantify ischemic damage, a simple 2-point scoring system was developed together with a senior pathologist (BK) and laboratory technician (LE). We consider that this experiment has shown an advantage of ECP over SCS, but the ultimate proof of better preservation would be a replantation experiment comparing ECP to SCS with long-term follow-up. However, the SCS preservation time in such an experiment should be decreased, since the profound damage in the SCS group at≥12 h of preservation prevented successful replantation in this group.
5Conclusion
This study is the first to assess skeletal muscle damage during long-term extracorporeal perfusion using electron microscopy. Extracorporeal perfusion showed superior results to static cold storage during 36 h flap preservation. This provides further evidence for ECP as a promising technique for prolonged skeletal muscle preservation. This research furthermore identified a different nature of capillary damage during ECP compared to SCS. The evolution of capillary thinning during ECP and its relation to perfusion pressure poses an interesting topic for future research.
Conflict of interest
No.
Funding
None.
Ethical statement
All applicable international, national, and institutional guidelines for the care and use of animals were followed, the experiment was approved by the regional and national ethical committees for animal welfare.
References
[1] | Blaisdell FW , The pathophysiology of skeletal muscle ischemia and the reperfusion syndrome: a review, Cardiovasc Surg (2002) ;10: (6):620–30. |
[2] | Guibert EE , Petrenko AY , Balaban CL , Somov AY , Rodriguez JV , Fuller BJ , Organ Preservation: Current Concepts and New Strategies for the Next Decade, Transfus Med Hemother (2011) ;38: (2):125–42. |
[3] | Eckert P , Schnackerz K , Ischemic tolerance of human skeletal muscle, Ann Plast Surg (1991) ;26: (1):77–84. |
[4] | Harman JW , A histologic study of skeletal muscle in acute ischemia, Am J Pathol (1947) ;23: (5):909. |
[5] | Gidlof A , Lewis DH , Hammersen F , The effect of prolonged total ischemia on the ultrastructure of human skeletal muscle capillaries, A morphometric analysis. Int J Microcirc Clin Ex (1988) ;7: (1):67–86. |
[6] | Sternbergh WC , 3rd, Adelman B , The temporal relationship between endothelial cell dysfunction and skeletal muscle damage after ischemia and reperfusion, J Vasc Surg (1992) ;16: (1):30–9. |
[7] | Ferrara PJ , Verkerke ARP , Brault JJ , Funai K , Hypothermia Decreases O2 Cost for Ex Vivo Contraction in Mouse Skeletal Muscle, Med Sci Sports Exerc (2018) ;50: (10):2015–23. |
[8] | Bolognesi MP , Chen LE , Seaber AV , Urbaniak JR , Protective effect of hypothermia on contractile force in skeletal muscle, J Orthop Res, (1996) ;14: (3):390–5. |
[9] | Kruit AS , Brouwers K , van Midden D , Zegers H , Koers E , van Alfen N , et al. Successful 18-h acellular extracorporeal perfusion and replantation of porcine limbs - Histology versus nerve stimulation, Transpl Int (2021) ;34: (2):365–75. |
[10] | Henry SD , Nachber E , Tulipan J , Stone J , Bae C , Reznik L , et al., Hypothermic machine preservation reduces molecular markers of ischemia/reperfusion injury in human liver transplantation, Am J Transplant (2012) ;12: (9):2477–86. |
[11] | Shimizu F , Okamoto O , Katagiri K , Fujiwara S , Wei FC , Prolonged ischemia increases severity of rejection in skin flap allotransplantation in rats, Microsurgery (2010) ;30: (2):132–7. |
[12] | Messner F , Grahammer J , Hautz T , Brandacher G , Schneeberger S , Ischemia/reperfusion injury in vascularized tissue allotransplantation: tissue damage and clinical relevance, Curr Opin Organ Transplant (2016) ;21: (5):503–9. |
[13] | Datta N , Devaney SG , Busuttil RW , Azari K , Kupiec-Weglinski JW , Prolonged Cold Ischemia Time Results in Local and Remote Organ Dysfunction in a Murine Model of Vascularized Composite Transplantation, Am J Transplant (2017) ;17: (10):2572–9. |
[14] | Hovius SER , Kruit AS , Ulrich DJO , [The first bilateral hand transplantation in the Netherlands: results after 1 year]. Ned Tijdschr Geneeskd. 2021;165. |
[15] | Kueckelhaus M , Dermietzel A , Alhefzi M , Aycart MA , Fischer S , Krezdorn N , et al. Acellular Hypothermic Extracorporeal Perfusion Extends Allowable Ischemia Time in a Porcine Whole Limb Replantation Model, Plast Reconstr Surg (2017) ;139: (4):922e–32e. |
[16] | Percie du Sert N , Hurst V , Ahluwalia A , Alam S , Avey MT , Baker M , et al., The ARRIVE guidelines 2updated guidelines for reporting animal research, J Physiol (2020) ;598: (18):3793–801. |
[17] | Kruit AS , Winters H , van Luijk J , Schreinemachers MJM , Ulrich DJO , Current insights into extracorporeal perfusion of free tissue flaps and extremities: a systematic review and data synthesis, J Surg Res (2018) ;227: :7–16. |
[18] | Paradis S , Charles AL , Meyer A , Lejay A , Scholey JW , Chakfe N , et al., Chronology of mitochondrial and cellular events during skeletal muscle ischemia-reperfusion, Am J Physiol Cell Physiol.C (2016) ;310: (11):968–82. |
[19] | Taeger CD , Friedrich O , Drechsler C , Weigand A , Hobe F , Geppert CI , et al., Hydroxyethyl starch solutionfor extracorporeal tissue perfusion. Clin Hemorheol Microcirc. 2016. |