Activation changes in patients with post-stroke cognitive impairment receiving intermittent theta burst stimulation: A functional near-infrared spectroscopy study
Abstract
BACKGROUND:
Intermittent theta burst stimulation (iTBS) has demonstrated efficacy in patients with cognitive impairment. However, activation patterns and mechanisms of iTBS for post-stroke cognitive impairment (PSCI) remain insufficiently understood.
OBJECTIVE:
To investigate the activation patterns and potential benefits of using iTBS in patients with PSCI.
METHODS:
A total of forty-four patients with PSCI were enrolled and divided into an iTBS group (iTBS and cognitive training) or a control group (cognitive training alone). Outcomes were assessed based on the activation in functional near-infrared spectroscopy (fNIRS), as well as Loewenstein Occupational Therapy Cognitive Assessment (LOTCA) and the modified Barthel Index (MBI).
RESULTS:
Thirty-eight patients completed the interventions and assessments. Increased cortical activation was observed in the iTBS group after the interventions, including the right superior temporal gyrus (STG), left frontopolar cortex (FPC) and left orbitofrontal cortex (OFC). Both groups showed significant improvements in LOTCA and MBI after the interventions (p < 0.05). Furthermore, the iTBS group augmented superior improvement in the total score of MBI and LOTCA compared to the control group, especially in visuomotor organization and thinking operations (p < 0.05).
CONCLUSION:
iTBS altered activation patterns and improved cognitive function in patients with PSCI. The activation induced by iTBS may contribute to the improvement of cognitive function.
1Introduction
With the increasing prevalence of cerebrovascular disease, stroke has become the leading cause of disability in the elderly, significantly impacting their quality of life and increasing the burden on their families and society (Bordet et al., 2017). It has been reported that approximately two-third of stroke survivors experienced varying degrees of functional limitation (Jiang et al., 2019). Cognitive dysfunctions commonly manifest in stroke patients within three to six months post-stroke, a condition identified as post-stroke cognitive impairment (PSCI) (Rost et al., 2022). The onset time of PSCI is defined differently, with cognition declining either immediately or within six months (Cogo et al., 2021). Despite spontaneous cognitive recovery in the first three months, 24-75% patients experience persistent cognitive impairment, resulting in a two- to ninefold increase in the risk of progression towards dementia (Wang et al., 2022; Ihara & Kalaria, 2014; Nijsse et al., 2017). PSCI is characterized by cognitive decline in various domains, including attention, memory, language, learning and executive function. It is closely associated with disability, dependency, a poor quality of life, and deterioration of long-term stroke prognoses (Rost et al., 2022; Nie et al., 2022). The substantial burdens on patients, their caregivers, society underscore the need for the development of interventions for PSCI.
There is a lack of FDA-approved treatments for PSCI aimed at preventing or slowing progression towards dementia after a stroke, as well as a dearth of understanding regarding the exact mechanisms of PSCI (Farooq & Gorelick, 2013; Peng et al., 2016). Pharmacological treatments lack consensus on the generalizability of effects, including cholinesterase inhibitors and memantine nootropics (Brainin et al., 2015; Quinn et al., 2021). Meanwhile, drugs may have short-term benefits but come with and drug-related side effects (Tomassoni et al., 2008). Consequently, non-pharmacological treatments have rapidly evolved as a significant aspect of alternative interventions for PSCI. Cognitive training has shown the potential to improve cognitive function to some extent, although there is insufficient evidence to make a recommendation (Quinn et al., 2021). Limitations of cognitive training include the requirement for patient active participation, long course treatment and slow onset of effect.
Repetitive transcranial magnetic stimulation (rTMS), a non-invasive brain stimulation (NIBS), utilizes electromagnetic fields to modulate the excitability of brain regions (Murphy et al., 2023), leading to alterations in cortical excitability and synaptic plasticity (Huerta & Volpe, 2009). Intermittent theta burst stimulation (iTBS) is a specialized patterned form of rTMS that mimics neural oscillatory patterns contributing to effective cognitive process (Grossheinrich et al., 2009; Wischnewski & Schutter, 2015). iTBS involves applying bursts of high-frequency rTMS repeated at intervals of 200 ms in an intermittent fashion (Chung et al., 2015). Previous studies have indicated that iTBS had some advantages compared to high-frequency rTMS, yielding more facilitatory effects that outlasted the stimulation time for 60 minutes (Wischnewski & Schutter, 2015; Huang et al., 2005). Moreover, iTBS is applied with low stimulation intensity and short duration of a stimulation session, resulting in long-term efficacy (Nowak et al., 2010). iTBS generates N-methyl-D-aspartate receptor-dependent synaptic plasticity of long-term potentiation (LTP) (Lefaucheur et al., 2014), modulates the release of several neurotransmitters, such as dopamine (Cho & Strafella, 2009), and improves microglial and astrocyte functions to exert a protective impact on neurodegenerative and neuroinflammatory diseases (Stanojevic et al., 2022). Chu et al. (2022) found that iTBS applied to patients with PSCI improved cognitive function and changed cortical activation in the dorsolateral prefrontal cortex (DLPFC), frontopolar cortex and Broca area. Two meta-analysis studies revealed that iTBS was a beneficial intervention for improving cognitive function in both healthy individual and patients with cognitive dysfunction (Pabst et al., 2022; Zheng et al., 2024). These studies indicated that iTBS showed a trend toward improvement of cognitive function.
Although iTBS has been recommended for mild to moderate cognitive impairment due to better neuroplasticity of the DLPFC in these patients (Wu et al., 2022), there is a lack of established efficacy. He et al. (2021) demonstrated discernible effects of iTBS on cognition in patients with Parkinson’s disease and cognitive impairment, which were not observed in the study by Lang et al. (2020). Therefore, this study aims to further investigated the effects of iTBS on mild to moderate PSCI.
Functional near-infrared spectroscopy (fNIRS) is an optical imaging technique based on neurovascular coupling, where alterations in the concentrations of oxygenated (HbO2) and deoxygenated (HbR) hemoglobin serve as surrogates for neural activation (Menant et al., 2020; Hramov et al., 2020). In comparison to established neuroimaging techniques (such as functional magnetic resonance imaging and electroencephalography), fNIRS offers advantages in terms of portability, cost-effectiveness, and reduced motion-related artifacts, making it a feasible alternative in research applications (Ehlis et al., 2014).
Neuronal activity necessitates oxygen consumption for glucose metabolism to provide energy. In the absence of an energy reserve in the brain, oxygen must be stably transported to neurons in the form of HbO2. This process leads to a decrease in HbO2 concentration and an increase in HbR concentration. The feedback mechanism involving increased metabolites (such as CO2 and lactic acid) and the feedforward mechanism of vasoactive product release from synaptic activity result in the local expansion of small arteries and microvessels, leading to local increase in blood flow increase (functional hyperaemic response). The augmented blood flow supplies oxygen and glucose to active neurons. This neuronal activity, accompanied by vasodilation, is identified as neurovascular coupling, reflecting brain metabolism (Zhu et al., 2022; Rahman et al., 2020; Segarra et al., 2019).
The increased blood flow and oxygen transporting capacity surpass the metabolic requirements at the site of brain activation, along with oxygen consumption. This leads to an increase in HbO2 concentration and a decrease in HbR concentration (Kisler et al., 2017). Pirondini et al. (2022) reported that the activation pattern of neural networks could indicate the recovery of cognitive function, representing functional reorganization of neural networks. Chu et al. (2022) demonstrated that iTBS result in better activation of the left DLPFC, frontopolar cortex (FPC) and Broca area compared to transcranial direct current stimulation.
This study aims to investigate the effects of iTBS combining with cognitive training on PSCI compared to cognitive training alone, utilizing fNIRS. The primary objective was to investigate the impact of iTBS on patients with PSCI. The secondary objective was to investigate the neural activation patterns associated with iTBS to unearth potential underlying neural mechanisms.
2Methods
2.1Study design
The study was a prospective, monocentric and randomized trial. The study was approved by the local institutional review board (Zhejiang Rehabilitation Medical Center; ZKLL22072503) and registered in the Chinese Clinical Trial Registry (ChiCTR2400079447). Written informed consent was obtained from all patients or their legal representatives. The study took place at Zhejiang Rehabilitation Medical Center and adhered to the Consolidated Standards of Reporting Trials (CONSORT) reporting guideline for clinical trials (Schulz et al., 2010).
2.2Participants
Patients were eligible if they had a confirmed diagnosis of stroke according to Chinese Guidelines for Diagnosis and Treatment of Acute Intracerebral Hemorrhage 2019 and Chinese Guidelines for Diagnosis and Treatment of Acute Ischemic Stroke 2018. Additional inclusion criteria include: patients who: (1) were aged between 18 and 75 years; (2) had a first-ever stroke; (3) were 1-6 months after stroke onset; (4) had a Mini-Mental State Examination (MMSE) score of 11-26, indicating mild-to-moderate cognitive impairment; (5) were right-handedness; and (6) provided informed written consent to participate in the study. Exclusion criteria encompassed a history of stroke, cerebral aneurysm or traumatic brain injury; personal or close family history of a seizure disorder; neurodegenerative disorders; psychotic disorders; presence of metal in the head; or the presence of implanted cranial or thoracic devices.
2.3Sample size calculation
A sample size of 22 per intervention group was based on the predicted effect size of the intervention on cognitive changes (Chu et al., 2022) and the formula (Hickey et al., 2018) with a type I error of 5% and 90% power.
2.4Randomization
There were two parallel groups: the iTBS group (iTBS and cognitive training) and the control group (cognitive training alone). These patients were randomly allocated in 1 : 1 ratio to the iTBS group or the control group through a randomized numbers table. Patients in the iTBS group received a 5-session iTBS over left DLPFC following cognitive training each week for a period of 6-weeks, while patients in the control group received cognitive training only.
2.5Intervention
2.5.1iTBS
iTBS was administered using a CCYI–type stimulator equipped with an 80-mm figure-of-eight coil (Yiruide Co., Wuhan, China). The left DLPFC was the stimulation site, aligned with the placement of F3 in the 10-20 international EEG system (Klem et al., 1999). The coil was rotated at 45° relative to the midline and positioned with the handle backward. Patients were seated in a comfortable chair and remained static. We measured the rest motor threshold (RMT) of the contralesional M1 to determine a hot spot, where the contraction of the abductor pollicis brevis muscle was induced. Once the hot spot was located, we adjust the TMS intensity down in steps of 5% to determine RMT. RMT was defined as the minimum stimulation intensity needed to produces motor evoked potentials (MEPs) with peak-to-peak amplitude of ≥50μV in 50% trials when ten consecutive single pulses are applied with the TMS coil fixed on the hot spot of the abductor pollicis brevis muscle (Rotenberg et al., 2014). Intensity was set at 70% RMT. During the iTBS session, patients remained static. iTBS consisted of bursts of 3 pulses at 50 Hz repeated at 200 msec intervals (5 Hz) for 2 seconds (10 bursts), with a total of 600 pulses and 200 seconds per session. The intervention was given once daily, over a course of thirty consecutive working days.
The rationale for choosing the intervention was based on two main considerations: (1) a desire to use evidence-based iTBS intervention for individuals with PSCI, and (2) the role of the left DLPFC as a critical brain region for integrating information relevant to the cognitive and executive (Xia et al., 2021).
2.5.2Cognitive training
Cognitive training was conducted using the cognitive rehabilitation and evaluation system (Jizhi, Hangzhou, China), which is referred to computer-assisted cognitive rehabilitation. The training included the following content with a focus on attention, executive function, memory, calculation and reasoning ability, lasting for 30 minutes. Cognitive training comprised a total of 30 sessions, conducted over six weeks with five consecutive daily sessions.
2.6Outcome measures
The primary and secondary outcomes were assessed by trained therapists at baseline (T0) and 6 weeks (T1), while remaining blinded to the group allocation of participants.
2.6.1fNIRS data acquisition and analysis
fNIRS data was acquired using an ETG-4000 Optical Topography System (Hitachi Medical Co., Tokyo, Japan) at two wavelengths of infrared light (695 and 830 mm) to measure changes in brain tissue concentration of oxygenated (HbO2) and deoxygenated (HbR) hemoglobin following neural activation based on the modified Beer-Lambert Law (Almajidy et al., 2020). The system consisted of seventeen sources and sixteen detectors, with a three-centimeter distance between a pair of sources and detectors. The area between a pair of source and detector probes was defined as a channel. A 33-probe set arranged in a 3×11 configuration with 52 channels was used for the prefrontal cortex (PFC) and superior temporal cortex (Fig. 1). The data sampling rate is 10 Hz, providing a better track of the shape of the hemodynamic response function (HRF) (Pinti et al., 2020). According to the 10-20 international EEG system, the lowest probes were positioned along the Fp1-Fp2 line.
Fig. 1
The placement of 52 channels following the Montreal Neurological Institute (MNI) coordinates.
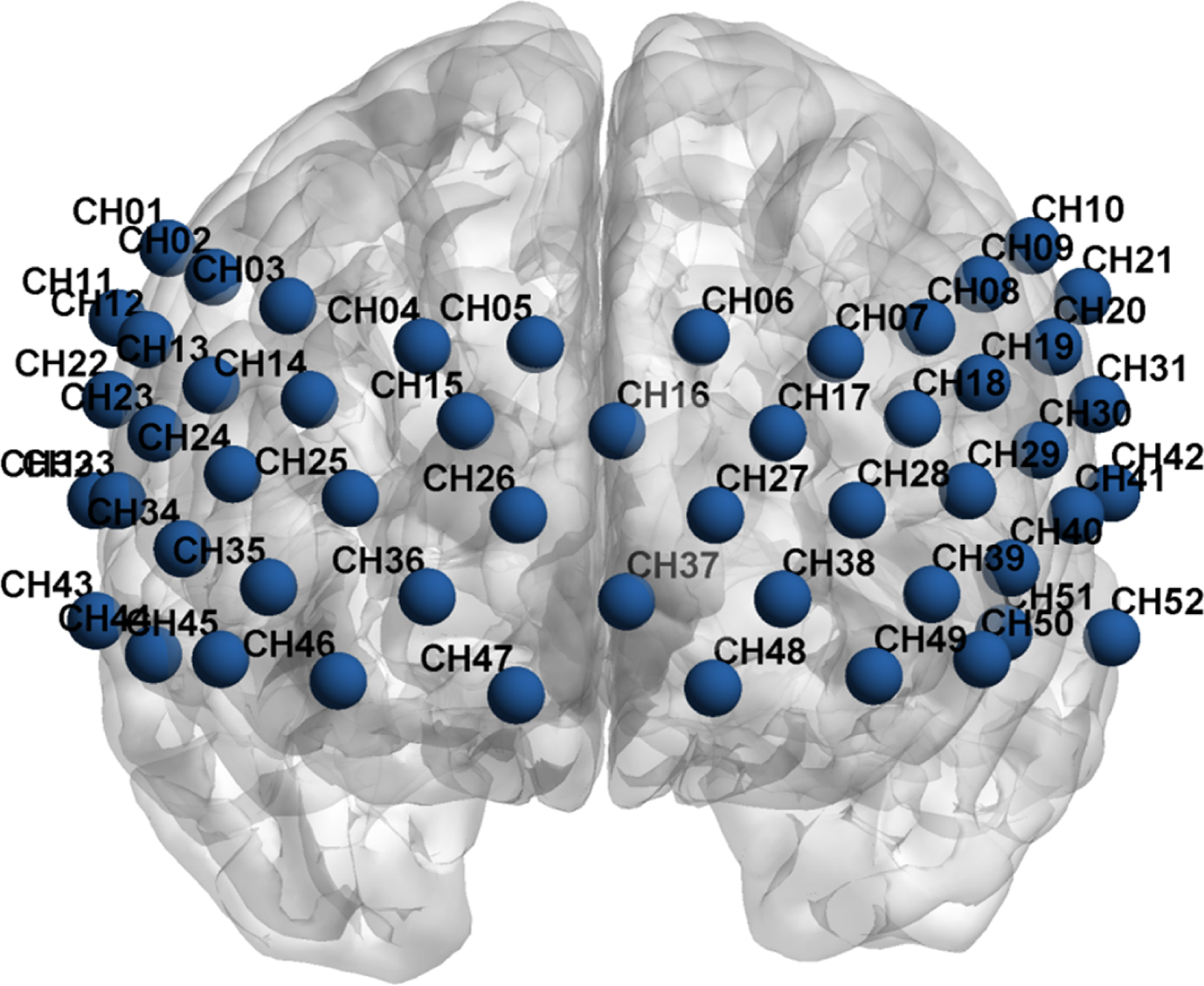
Due to the comparative nature of the fNIRS technique (Menant et al., 2020), the verbal fluency task (VFT) was designed to detect the hemodynamic alterations. Accumulating evidence suggested that VFT can elicit hemodynamic responses in cognitive brain regions (e.g. prefrontal cortex), rapidly evolving into a significant fNIRS task to detect changes in brain function in the realm of cognition (Luo et al., 2018; Wei et al., 2022; Yang et al., 2023). The fNIRS data were acquired when patients performed VFT at baseline (T0) and 6 weeks (T1). VFT comprised a 30-second pre-task period, a 60-second task period and a 70-second post-task period. Patients were instructed to repeat one to five during the pre-task period and the post-task period. These periods were performed to correct the data during the fluency task for activation due to vocalization. During the task period, patients generated as many words as possible based on three given Chinese characters (‘tian’, ‘xiao’ and ‘bai’, indicating sky, small and white), with each character phase lasting for 20 seconds. fNIRS measurements were conducted by trained researchers. The experiments were conducted in a room where the environmental conditions were kept stable to ensure that patients did not experience any discomfort and that the electronic devices performed optimally. To maintain patient attention and minimize movement, patients were instructed to look at the red asterisk on the screen, which was kept at approximately 1 meter in front of them.
fNIRS data were analyzed using a toolbox (NIRS-SPM) running on MATLAB R2020b (Mathworks Inc., Natick, MA, USA). The data processing was as follows: (1) All raw data was first converted to MATLAB compatible format (.mat) using NIRS-SPM. (2) The wavelet-MDL detrending algorithm was employed to attenuate low-frequency noise caused by physiological artifacts (e.g. heart rate, respiration, blood pressure) and environmental conditions of laboratory settings (e.g. sound, light), which may confound the fNIRS signal and increase the risk of ‘false positive’ findings (Menant et al., 2020). High frequency noise was reduced using a low pass filter based on HRF. (3) Pervious evidence suggested that changes in HbO2 reflected the positive correlation with the blood oxygenation level-dependent (BOLD) response (Pinti et al., 2020; Strangman et al., 2002; Cui et al., 2011), indicating that HbO2 is the most sensitive index to detect cerebral activation regarding changes in fNIRS measurement values (Yamaya et al., 2021; Mihara et al., 2021). Therefore, time series and HbO2 signals related to VFT task served as independent and dependent variables, respectively. Further, HRF was estimated through a general linear deconvolutionmodel (GLM). (4) We calculated the beta value of each independent variable using the GLM model. (5) The beta values of all 52 channels under each condition were obtained. In the present study, regions of interest (ROIs) based on Brodmann area included the left DLPFC (channels 6, 7, 8, 9, 17, 18, 28, 29, 39, 49, 50), right DLPFC (channels 2, 3, 4, 5, 14, 25, 35, 45, 46), frontopolar area (channels 5, 6, 16, 17, 25, 26, 27, 28, 36, 37, 38, 39, 46, 49), right superior temporal gyrus (channels 32, 33, 43), left superior temporal gyrus (channels 42), left orbitofrontal cortex (channels 38, 48, 49), right orbitofrontal cortex (channels 36, 46, 47).
Within-groups factors and between-groups factors were served as different time (pre-treatment and post-treatment) and groups (the iTBS group and the control group), respectively. A 2*2 mixed Two-way ANOVA was performed to obtain the simple effect, main effect and interaction effect of the groups. To control for alpha errors induced by multiple comparisons of 52 channels, a false discovery rate (FDR) correction was utilized with p < 0.05 considered significant. The channels with statistical significance underwent post-hoc analysis to identify the source of the statistical significance.
2.6.2Secondary outcomes
The secondary outcome included the Loewenstein Occupational Therapy Cognitive Assessment (LOTCA) and the modified Barthel Index (MBI).
Compared with MMSE, LOTCA showed superiority in detection for insidious cognitive and functional decline (Wang et al., 2014), exhibiting satisfactory construct validity and internal consistency reliability (Almomani et al., 2018). LOTCA comprised 22 sub-items in five areas: orientation (2 subtests), perception (6 subtests), visuomotor organization (7 subtests), thinking operations (6 subtests) and attention and concentration. A 4- or 5-point rating scale is used to score each subtest, with a total score of 91.
MBI is a measure of independence in activities of daily living (ADL) (Ohura et al., 2017). Proposed by Canadian scholars in 1989, MBI divides each item into five levels based on the Barthel Index (BI). The modified version shows superiority in assessing the degrees of the need for help and the changes of rating (Shah et al., 1989). MBI measures an individual’s performance on 10 ADL functions as follows: personal hygiene, bathing self (5 points each); feeding, toilet use, stair climbing, dressing, bowel management, bladder management (10 points each); ambulation and chair or bed transfers (15 points each). The highest score is 100, with higher scores indicating increased independence in ADL.
2.7Statistical analysis
Statistical analyses were conducted using SPSS software version 25.0 (IBM Corp., Armonk, NY, USA). Descriptive statistics summarized patient characteristics using mean (SD) and number (percentage) as appropriate. Demographic and clinical variables were compared between groups using the two-sample t-test and chi-square test. The Shapiro-Wilk test and visual inspection of plots were used to assess skewness of continuous data. The paired t-test was conducted to compare the baseline and post-treatment results. The two-sample t-test was employed for between-group comparisons. All tests were two-sided, and a statistical significance was set at p < 0.05.
3Results
3.1Demographic and clinical characterization
Forty-four patients were initially enrolled, but two patients withdrew from the study due to protocol violations and four patients withdrew from the study for discharging. Finally, both groups consisted of nineteen patients (Fig. 2). No statistically significant differences were observed between the two groups in any of the demographics and clinical data (Table 1). The mean (SD) baseline MMSE score of 16.21 (3.90) and 16.00 (3.90) indicated that the patients had mild to moderate cognitive impairment.
Fig. 2
Flow diagram of the study.
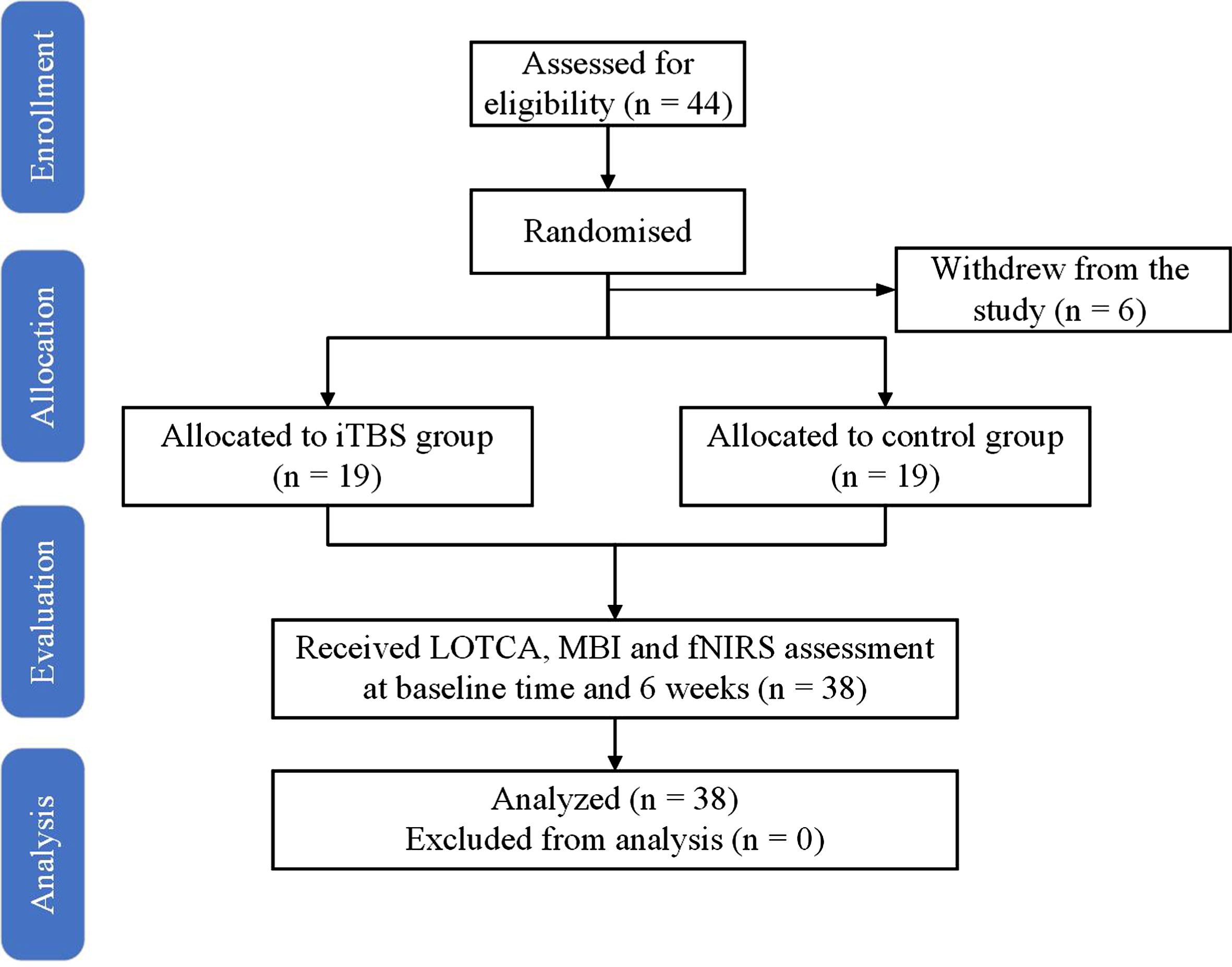
Table 1
Baseline patients’ demographics and clinical characteristics
iTBS group | Control group | p value | |
Age, years | 62.84 (12.67) | 62.58 (12.65) | 0.949 |
Sex, female | 4 (21%) | 3 (16%) | 0.676 |
Education, years | 8.84 (4.71) | 7.26 (2.31) | 0.197 |
Time since stroke, months | 2.84 (1.86) | 3.26 (1.73) | 0.475 |
Type of stroke | |||
Hemorrhagic/Ischemic | 3 (16%)/16 (84%) | 5 (26%)/14 (74%) | 0.426 |
Injury site, left | 12 (63%) | 16 (84%) | 0.276 |
MMSE | 16.21 (3.90) | 16.00 (3.90) | 0.869 |
LOTCA | 57.32 (11.20) | 56.53 (11.67) | 0.833 |
Orientation | 5.53 (1.78) | 4.89 (1.79) | 0.282 |
Perception | 20.68 (3.00) | 20.05 (3.36) | 0.545 |
Visuomotor organization | 15.21 (5.23) | 15.42 (5.41) | 0.904 |
Thinking operations | 13.16 (4.32) | 13.32 (3.72) | 0.905 |
Attention and concentration | 2.74 (0.81) | 2.84 (0.76) | 0.682 |
MBI | 57.00 (20.68) | 52.84 (22.79) | 0.560 |
Data are mean (SD) or number of patients in each group (% of total). MMSE: Mini-mental State Examination; LOTCA: Loewenstein Occupational Therapy Cognitive Assessment; MBI: Modified Barthel Index.
3.2fNIRS data
After FDR correction, there were group × time interaction effects in channel 32 (F = 10.24, p = 0.04937, η _ p2 = 0.221), 37 (F = 13.30, p = 0.043, η _ p2 = 0.269) and 38 (F = 10.92, p = 0.049, η _ p2 = 0.233), indicating that the beta values in the iTBS group changed over time, and that the beta values of channel 32, 37 and 38 (Figs. 3, 4) were significantly increased after the intervention in the iTBS group compared to the control group (p < 0.05). This gain was not observed in other channels.
Fig. 3
Violin plots of the interaction effects (*p < 0.05). (a) channel 32. (b) channel 37. (c) channel 38.
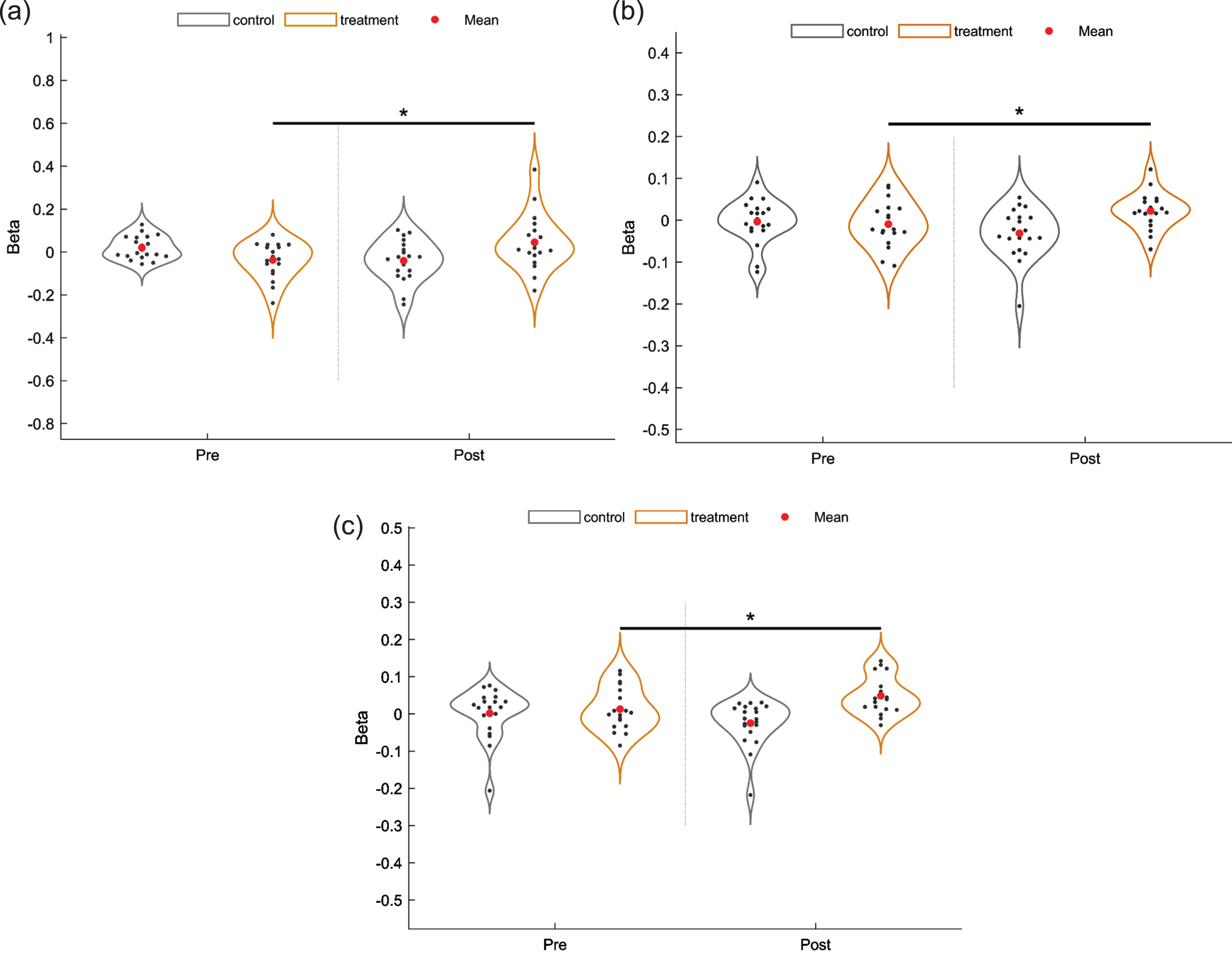
Fig. 4
Cortical activation map based on beta values during VFT in different groups. (a) T0 of the control group. (b) T1 of the control group. (c) T0 of the iTBS group. (d) T1 of the iTBS group.
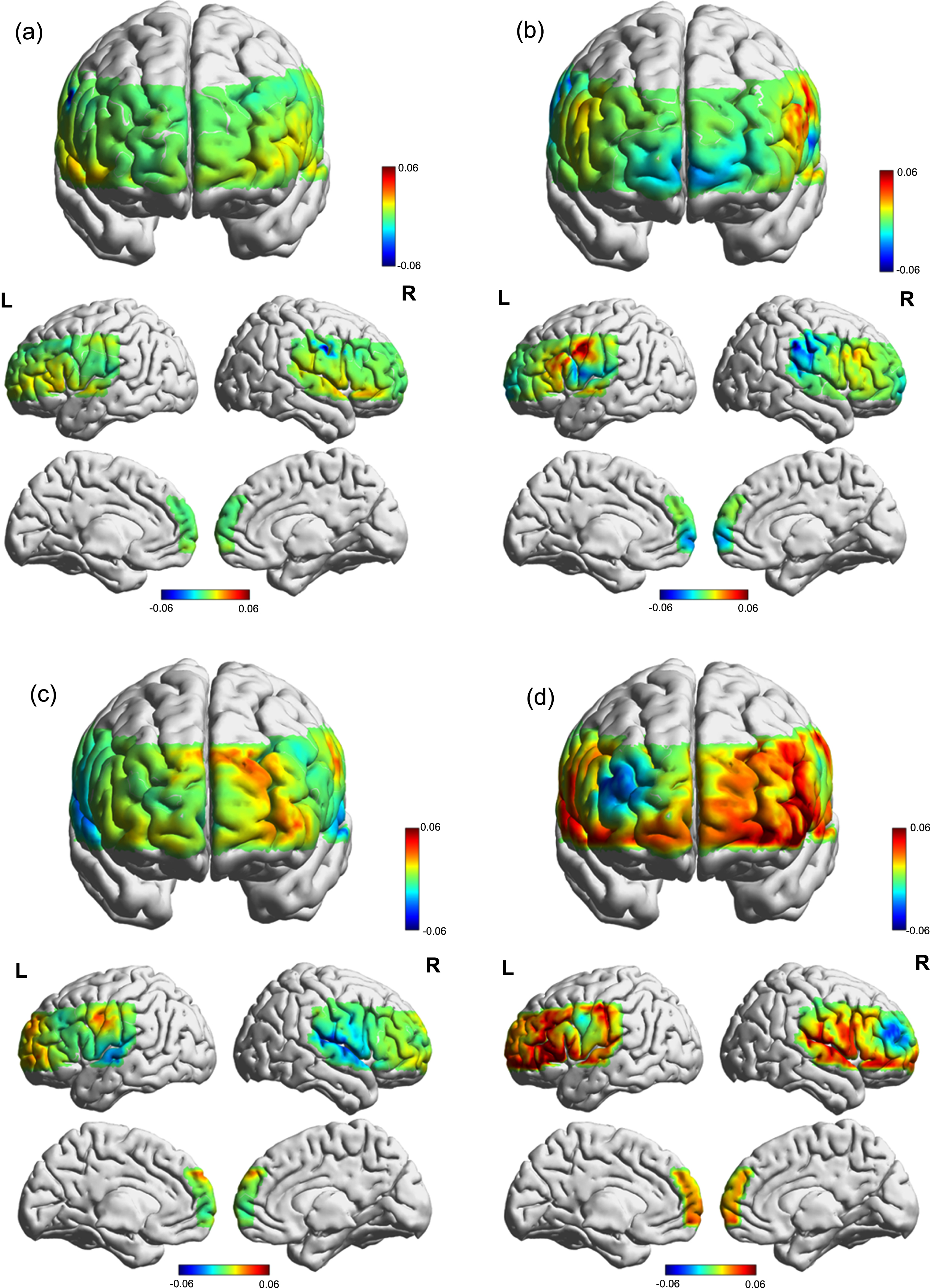
3.3Secondary outcomes
3.3.1LOTCA
Regarding the total score and the sub-items of LOTCA, both the iTBS group and the control group conferred significant improvements from the baseline to 6 weeks (p < 0.05). Furthermore, the iTBS group demonstrated better improvements in the total score (F = 0.274, p = 0.012), visuomotor organization (F = 0.433, p = 0.013) and thinking operations (F = 0.041, p = 0.028) compared to the control group after interventions. Figure 5. showed the graphs of the results.
Fig. 5
Results of Loewenstein Occupational Therapy Cognitive Assessment (LOTCA). Estimated changes and effects of within-groups and between-groups (*p < 0.05, **p < 0.01, ***p < 0.001).
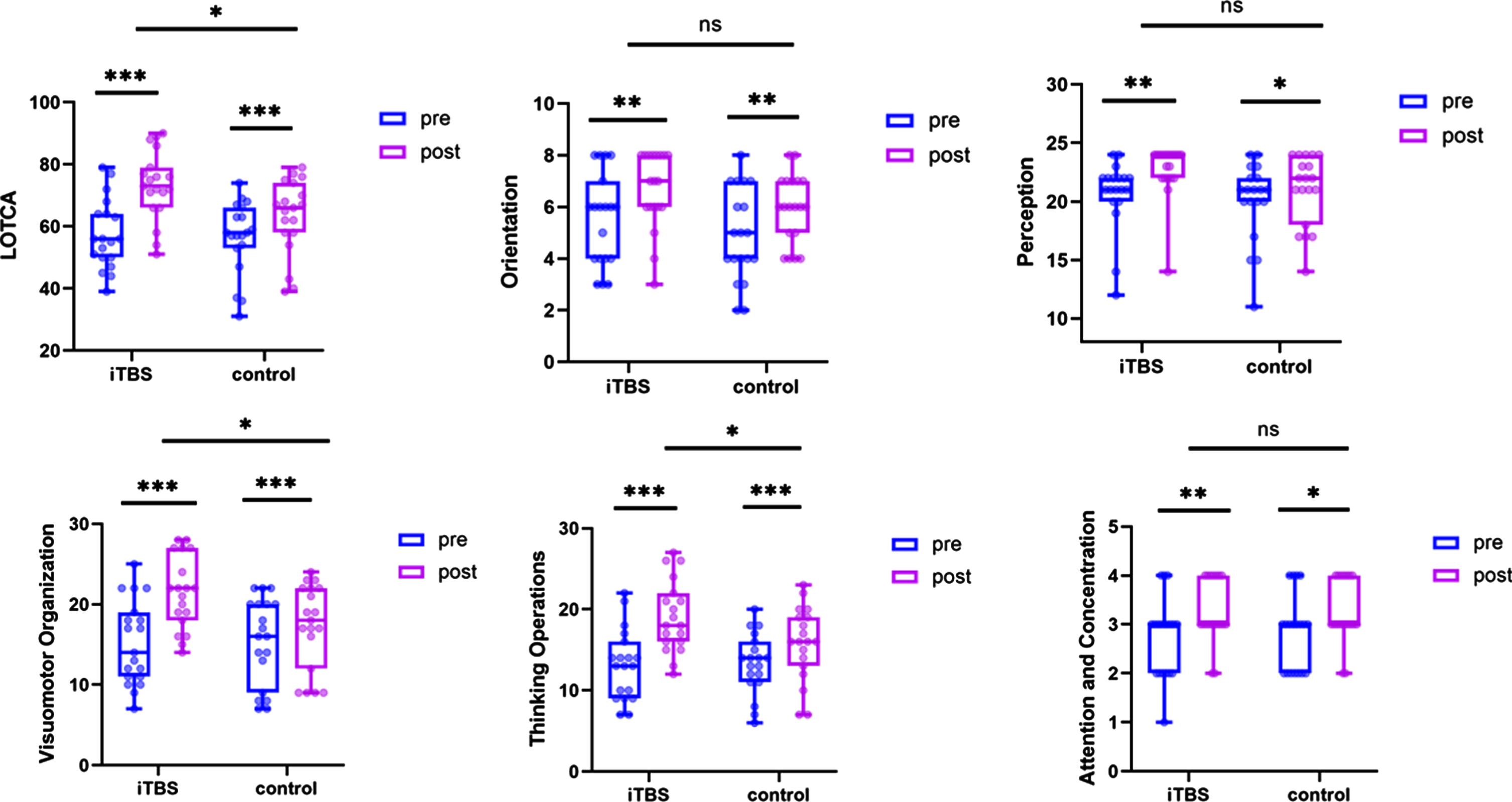
3.3.2MBI
Regarding MBI (Fig. 6), both groups exhibited significant improvements after intervention (p < 0.05). Furthermore, the iTBS group showed greater improvements in MBI compared to the control group after interventions (F = 0.003, p = 0.043).
Fig. 6
Results of modified Barthel Index (MBI). Estimated changes and effects of within-groups and between-groups (*p < 0.05, ***p < 0.001).
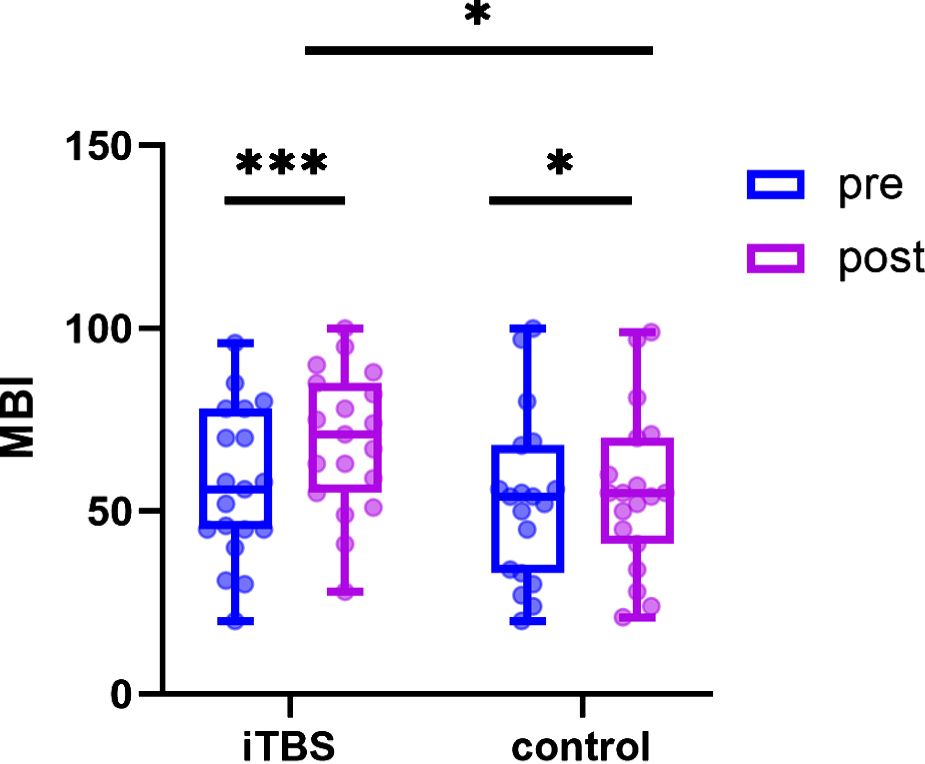
4Discussion
This trial compared the effects of iTBS applied over the left DLPFC with cognitive training against cognitive training alone in patients with PSCI. There were three cortical regions with significantly increased activation in the iTBS group compared to the baseline, including the right superior temporal gyrus (STG, channel 32), left frontopolar cortex (FPC, channel 37, 38) and left orbitofrontal cortex (OFC, channel 38). Both groups showed improvements in cognitive performance and ADL, as indicated by LOTCA and MBI. Additionally, the iTBS group showed better enhancement in the total score of MBI and LOTCA compared to the control group, particularly in visuomotor organization and thinking operations. The present study indicates that iTBS has a therapeutic effect on PSCI, as evidenced by improvements in performance and neural mechanisms.
After a stroke, impairment in neurovascular units result in neurovascular uncoupling (Lourenço et al., 2017), altering the reactivity of the cerebral circulation to vasomotor stimuli, and reducing the increased cerebral blood flow produced by functional activation (Girouard & Iadecola, 2006). In the early stage following a stroke, new pathways are activated to compensate for the impacted regions. This activation, as revealed by BOLD signals, may mimic the learning process typically observed during development (Crofts et al., 2020). A previous study reported decreased activation in PSCI patients in the bilateral superior temporal cortex, right DLPFC and bilateral ventrolateral prefrontal cortex compared to healthy individuals (Yang et al., 2022). Furthermore, Keser et al. (2021) reported that patients with post-stroke aphasia experienced the secondary degeneration of the thalamic nuclei and pathways of the left hemisphere, necessitating NIBS to prevent this secondary degeneration and improve prognosis.
Compared with the previous similar studies (Chu et al., 2022; Zhang et al., 2023), this study provided more robust fNIRS evidence contributing to cortical activation induced by iTBS, indicating that iTBS could restore of brain network plasticity. The activation of the left FPC aligns with the findings of Chu et al. (2022) and Zhang et al. (2023), suggesting its significance in executive function. The interaction among the FPC, the posterior cingulate gyrus and the ventrolateral prefrontal cortex supports goal-directed behavior, provides a working memory system, responsible for activating and selecting task-related information stored in the posterior cortex, and is involved in making a plan (Domic-Siede et al., 2021; Costa et al., 2013). The OFC receives contextual information to maintain accurate self-perception and is also involved in executive function (Rudebeck & Rich, 2018). A previous study showed that the atrophy of selective regional pyramidal neurons in DLPFC was associated with executive dysfunction in PSCI (Foster et al., 2014). rTMS has the potential to reduce the inhibitory control, promote morphological plasticity of pyramidal cells, and increase excitatory output (Han et al., 2023; Cambiaghi et al., 2021). Moreover, patients with PSCI may experience reduced frontal oxygenation changes (Mk & As, 2020). TMS induced a compensatory mechanism of autonomic control over the prefrontal haemoglobin levels (Struckmann et al., 2021). In addition, our study also observed the activation of right STG, which was associated with working memory (Wei et al., 2022). The VFT requires the retrieval of items from long-term memory storage and the working memory capacity to hold generated words (Henry & Crawford, 2004). Therefore, the activation of the right STG may contribute to cognitive amelioration.
Supporting evidence for improvement in visuomotor organization and thinking operations can be found in the fNIRS data. Our results showed the activation of the left FPC and OFC in patients receiving iTBS. FPC, as proved, is involved in higher cognitive functions, including problem-solving, reasoning and episodic memory retrieval (Braver & Bongiolatti, 2002). The OFC, responsible for making decisions, receives highly processed sensory information and outputs information to the medial striatum mediodorsal thalamus and other parts of the prefrontal cortex (Rudebeck & Rich, 2018). The OFC is one of the prefrontal circuits that control specific aspects of executive function (Foster et al., 2014). Moreover, both domains of cognitive improvement are crucial to higher cognitive processes. Visuomotor organization, supporting thinking operations, is involved in perceptual-motor integration with spatial components, referred to as high-order perception (Katz et al., 1989). Thinking operations include higher cognitive processes (e.g. problem solving, abstraction and logical operation) (Almomani et al., 2018). Both domains are associated with executive function. Therefore, our results may be interpreted as suggesting that iTBS could enhance cognitive performance mediated by the FPC and OFC.
However, the prefrontal cortex contains a large number of networks, responsible for connecting motor, perceptual, and limbic regions of the brain. Almost all cortical and subcortical regions affect the prefrontal cortex through direct projection or indirectly through several prominent relays. Due to the channel limitation of the fNIRS equipment, the activation effect of remote brain regions failed to be obtained in this study. Liu et al. reported that patients with post-ischemic stroke executive impairment exhibited activation in the left DLPFC, right PMC and right SM1 when they received a single session of rTMS (Liu et al., 2023).
Both groups showed significant improvement in MBI, but the iTBS group augmented better gains compared to the control group. Our result was consistent with a meta-analysis study where high-frequency rTMS could improve ADL in patients with PSCI (Chen et al., 2023). In addition, previous studies demonstrated that a higher cognitive score was associated with better performance on ADL (Chu et al., 2022; Yin et al., 2020). Visuomotor organization and thinking operations may contribute to the transfer of gains to ADL. Better cognitive function could enhance their ability to learn and perform the daily living task.
This study has limitations. Firstly, our study may overlook the effects of iTBS on memory. The pattern of iTBS resembles the brain’s natural theta rhythm in the hippocampus (Klomjai et al., 2015), which, along with the left DLPFC, is engaged in working memory (Chu et al., 2021; Marshall & Binder, 2013; Zhang et al., 2019). However, LOTCA fails to provide the information of memory. Secondly, due to the lack of follow-up, our study failed to observe the long-term effects of iTBS. The effects of rTMS may take time to fully develop and translate into clinical benefits (Sabbagh et al., 2020), necessitating further studies to explore the duration of effects and determine whether repeating iTBS is needed, setting a long-term intervention protocol. Thirdly, the lack of a sham or placebo design may introduce bias of effects (Broadbent et al., 2011).
There is a lack of consensus regarding the rTMS protocol for PSCI. Regarding the stimulation target, previous studies revealed that the application of high-frequency rTMS on the ipsilesional DLPFC exerted ameliorative roles in cognition of PSCI and provided evidence of the anti-inflammtory response and changes in brain networks (Cha et al., 2022). It remains questionable whether bilateral iTBS could lead to cognitive control potentiation. Although iTBS remains underutilized in the field of PSCI, we anticipate that, in the near future, advancements will offer a more profound understanding of the mechanisms and the optimal dosage of iTBS.
5Conclusions
iTBS generated activation patterns supporting the restoration of brain network plasticity and the cognitive processes. iTBS could improve cognitive function and ADL, validating iTBS was an alternative and complementary approach to cognitive rehabilitation. fNIRS contributes to describe the neurophysiological mechanism relevant to cognitive control. Further studies are warranted to explore the activation of brain regions induced by iTBS associated with the neurophysiological underpinnings and mechanism.
Acknowledgments
The authors thank everyone who participated in this study.
Conflict of interest
The authors have no competing interests to declare.
Funding
This study was supported by Scientific Research Project of Zhejiang Rehabilitation Medical Center, China (ZKJC2203) and the General Scientific Research Project of Zhejiang Provincial Health Commission, China (2023KY1015).
References
1 | Almajidy R. K. , Mankodiya K. , Abtahi M. , & Hofmann U. G. ((2020) ). A newcomer’s guide to functional near infrared spectroscopy experiments, IEEE Reviews in Biomedical Engineering, 13: , 292–308. https://doi.org/10.1109/RBME.2019.2944351 |
2 | Almomani F. , Avi-Itzhak T. , Demeter N. , Josman N. , & Al-momani M. O. ((2018) ). Construct validity and internal consistency reliability of the Loewenstein occupational therapy cognitive assessment (LOTCA), BMC Psychiatry, 18: (1), 184. https://doi.org/10.1186/s12888-018-1776-x |
3 | Bordet R. , Ihl R. , Korczyn A. D. , Lanza G. , Jansa J. , Hoerr R. , & Guekht A. ((2017) ). Towards the concept of disease-modifier in post-stroke or vascular cognitive impairment: A consensus report, BMC Medicine, 15: (1), 107. https://doi.org/10.1186/s12916-017-0869-6 |
4 | Brainin M. , Tuomilehto J. , Heiss W. D. , Bornstein N. M. , Bath P. M. W. , Teuschl Y. , Richard E. , Guekht A. , & Quinn T. ((2015) ). Post-stroke cognitive decline: An update and perspectives for clinical research, European Journal Neurology, 22: (2), 229–238, e13-16. https://doi.org/10.1111/ene.12626 |
5 | Braver T. S. , & Bongiolatti S. R. ((2002) ). The role of frontopolar cortex in subgoal processing during working memory, NeuroImage, 15: (3), 523–536. https://doi.org/10.1006/nimg.2001.1019 |
6 | Broadbent H. J. , van den Eynde F. , Guillaume S. , Hanif E. L. , Stahl D. , David A. S. , Campbell I. C. , & Schmidt U. ((2011) ). Blinding success of rTMS applied to the dorsolateral prefrontal cortex in randomised sham-controlled trials: A systematic review, World Journal of Biological Psychiatry, 12: (4), 240–248. https://doi.org/10.3109/15622975.2010.541281 |
7 | Cambiaghi M. , Cherchi L. , Masin L. , Infortuna C. , Briski N. , Caviasco C. , Hazaveh S. , Han Z. , Buffelli M. , & Battaglia F. ((2021) ). High-frequency repetitive transcranial magnetic stimulation enhances layer II/III morphological dendritic plasticity in mouse primary motor cortex, Behavioural Brain Research, 410: , 113352. https://doi.org/10.1016/j.bbr.2021.113352 |
8 | Cha B. , Kim J. , Kim J. M. , Choi J. W. , Choi J. , Kim K. , Cha J. , & Kim M. ((2022) ). Therapeutic effect of repetitive transcranial magnetic stimulation for post-stroke vascular cognitive impairment: A prospective pilot study, Frontiers in Neurology, 13: , 813597. https://doi.org/10.3389/fneur.2022.813597 |
9 | Chen X. , Liu F. , Lyu Z. , Xiu H. , Hou Y. , & Tu S. ((2023) ). High-frequency repetitive transcranial magnetic stimulation (HF-rTMS) impacts activities of daily living of patients with post-stroke cognitive impairment: A systematic review and meta-analysis, Neurological Sciences, 44: (8), 2699–2713. https://doi.org/10.1007/s10072-023-06779-9 |
10 | Cho S. S. , & Strafella A. P. ((2009) ). rTMS of the left dorsolateral prefrontal cortex modulates dopamine release in the ipsilateral anterior cingulate cortex and orbitofrontal cortex, PloS One, 4: (8), e6725. https://doi.org/10.1371/journal.pone.0006725 |
11 | Chu C. S. , Li C. T. , Brunoni A. R. , Yang F. C. , Tseng P. T. , Tu Y. K. , Stubbs B. , Carvalho A. F. , Thompson T. , Rajji T. K. , Yeh T. C. , Tsai C. K. , Chen T. Y. , Li D. J. , Hsu C. W. , Wu Y. C. , Yu C. L. , & Liang C. S. ((2021) ). Cognitive effects and acceptability of non-invasive brain stimulation on Alzheimer’s disease and mild cognitive impairment: A component network meta-analysis, Journal of Neurology Neurosurgery and Psychiatry, 92: (2), 195–203. https://doi.org/10.1136/jnnp-2020-323870 |
12 | Chu M. , Zhang Y. , Chen J. , Chen W. , Hong Z. , Zhang Y. , Yu H. , Zhang F. , Ye X. , Li J. , & Yang Y. ((2022) ). Efficacy of intermittent theta-burst stimulation and transcranial direct current stimulation in treatment of post-stroke cognitive impairment, Journal of Integrative Neuroscience, 21: (5), 130. https://doi.org/10.31083/j.jin2105130 |
13 | Chung S. W. , Hoy K. E. , & Fitzgerald P. B. ((2015) ). Theta-burst stimulation: a new form of TMS treatment for depression, Depression and Anxiety, 32: (3), 182–192. https://doi.org/10.1002/da.22335 |
14 | Cogo A. , Mangin G. , Maïer B. , Callebert J. , Mazighi M. , Chabriat H. , Launay J. M. , Huberfeld G. , & Kubis N. ((2021) ). Increased serum QUIN/KYNA is a reliable biomarker of post-stroke cognitive decline, Molecular Neurodegeneration, 16: (1), 7. https://doi.org/10.1186/s13024-020-00421-4 |
15 | Costa A. , Oliveri M. , Barban F. , Bonnì S. , Koch G. , Caltagirone C. , & Carlesimo G. A. ((2013) ). The right frontopolar cortex is involved in visual-spatial prospective memory, PloS One, 8: (2), e56039. https://doi.org/10.1371/journal.pone.0056039 |
16 | Crofts A. , Kelly M. E. , & Gibson C. L. ((2020) ). Imaging functional recovery following ischemic stroke: Clinical and preclinical fMRI studies, Journal of Neuroimaging, 30: (1), 5–14. https://doi.org/10.1111/jon.12668 |
17 | Cui X. , Bray S. , Bryant D. M. , Glover G. H. , & Reiss A. L. ((2011) ). A quantitative comparison of NIRS and fMRI across multiple cognitive tasks, NeuroImage, 54: (4), 2808–2821. https://doi.org/10.1016/j.neuroimage.2010.10.069 |
18 | Domic-Siede M. , Irani M. , Valdés J. , Perrone-Bertolotti M. , & Ossandón T. ((2021) ). Theta activity from frontopolar cortex, mid-cingulate cortex and anterior cingulate cortex shows different roles in cognitive planning performance, NeuroImage, 226: , 117557. https://doi.org/10.1016/j.neuroimage.2020.117557 |
19 | Ehlis A. C. , Schneider S. , Dresler T. , & Fallgatter A. J. ((2014) ). Application of functional near-infrared spectroscopy in psychiatry, NeuroImage, 85: Pt 1, 478–488. https://10.1016/j.neuroimage.2013.03.067 |
20 | Farooq M. U. , & Gorelick P. B. ((2013) ). Vascular cognitive impairment, Current Atherosclerosis Reports, 15: (6), 330. https://doi.org/10.1007/s11883-013-0330-z |
21 | Foster V. , Oakley A. E. , Slade J. Y. , Hall R. , Polvikoski T. M. , Burke M. , Thomas A. J. , Khundakar A. , Allan L. M. , & Kalaria R. N. ((2014) ). Pyramidal neurons of the prefrontal cortex in post-stroke, vascular and other ageing-related dementias, Brain, 137: (Pt 9), 2509–2521. https://doi.org/10.1093/brain/awu172 |
22 | Girouard H. , & Iadecola C. ((2006) ). Neurovascular coupling in the normal brain and in hypertension, stroke, and Alzheimer disease, Journal of Applied Physiology, 100: (1), 328–335. https://doi.org/10.1152/japplphysiol.00966.2005 |
23 | Grossheinrich N. , Rau A. , Pogarell O. , Hennig-Fast K. , Reinl M. , Karch S. , Dieler A. , Leicht G. , Mulert C. , Sterr A. , & Padberg F. ((2009) ). Theta burst stimulation of the prefrontal cortex: Safety and impact on cognition, mood, and resting electroencephalogram, Biological Psychiatry, 65: (9), 778–784. https://doi.org/10.1016/j.biopsych.2008.10.029 |
24 | Han K. , Liu J. , Tang Z. , Su W. , Liu Y. , Lu H. , & Zhang H. ((2023) ). Effects of excitatory transcranial magnetic stimulation over the different cerebral hemispheres dorsolateral prefrontal cortex for post-stroke cognitive impairment: A systematic review and meta-analysis, Fronters in Neuroscience, 17: , 1102311. https://doi.org/10.3389/fnins.2023.1102311 |
25 | Huang Y. Z. , Edwards M. J. , Rounis E. , & Rothwell J. C. ((2005) ). Theta burst stimulation of the human motor cortex, Neuron, 45: (2), 201–206. https://doi.org/10.1016/j.neuron.2004.12.033 |
26 | He W. , Wang J. C. , & Tsai P. Y. ((2021) ). Theta burst magnetic stimulation improves parkinson’s-related cognitive impairment: A randomised controlled study, Neurorehabiltation and Neural Repair, 35: (11), 986–995. https://doi.org/10.1177/1545968321104131 |
27 | Henry J. D. , & Crawford J. R. ((2004) ). A meta-analytic review of verbal fluency performance following focal cortical lesions, Neuropsychology, 18: (2), 284–295. https://doi.org/10.1037/0894-4105.18.2.284 |
28 | Hickey G. L., Grant S. W., Dunning J., & Siepe M. tatistical primer: sample size and power calculations-why, when and how, European Journal of Cardio-thoracic Surgery, 54: (1), 4–9. https://doi.org/10.1093/ejcts/ezy169 |
29 | Hramov A. E. , Grubov V. , Badarin A. , Maksimenko V. A. , & Pisarchik A. N. ((2020) ). Functional near-infrared spectroscopy for the classification of motor-related brain activity on the sensor-level, Sensors, 20: (8), 2362. https://doi.org/10.3390/s20082362 |
30 | Huerta P. T. , & Volpe B. T. ((2009) ). Transcranial magnetic stimulation, synaptic plasticity and network oscillations, Journal of Neuroengineering and Rehabilitation, 6: , 7. https://doi.org/10.1186/1743-0003-6-7 |
31 | Ihara M. , & Kalaria R. N. ((2014) ). Understanding and preventing the development of post-stroke dementia, Expert Review of Neurotherapeutics, 14: (9), 1067–1077. https://doi.org/10.1586/14737175.2014.947276 |
32 | Jiang M. , Su Y. , Liu G. , Chen W. , & Gao D. ((2019) ). Predicting the non-survival outcome of large hemispheric infarction patients via quantitative electroencephalography: Superiority to visual electroencephalography and the Glasgow Coma Scale, Neuroscience Letters, 706: , 88–92. https://doi.org/10.1016/j.neulet.2019.05.007 |
33 | Katz N. , Itzkovich M. , Averbuch S. , & Elazar B. ((1989) ). Loewenstein Occupational Therapy Cognitive Assessment (LOTCA) battery for brain-injured patients: Reliability and validity, American Journal of Occupational Therapy, 43: (3), 184–192. https://doi.org/10.5014/ajot.43.3.184 |
34 | Keser Z. , Meier E. L. , Stockbridge M. D. , Breining B. L. , Sebastian R. , & Hillis A. E. ((2021) ). Thalamic nuclei and thalamocortical pathways after left hemispheric stroke and their association with picture naming, Brain Connectivity, 11: (7), 553–565. https://doi.org/10.1089/brain.2020.0831 |
35 | Kisler K. , Nelson A. R. , Montagne A. , & Zlokovic B. V. ((2017) ). Cerebral blood flow regulation and neurovascular dysfunction in Alzheimer disease, Nature Reviews Neuroscience, 18: (7), 419–434. https://doi.org/10.1038/nrn.2017.48 |
36 | Klem G. H. , Lüders H. O. , Jasper H. H. , & Elger C. ((1999) ). The ten-twenty electrode system of the International Federation. The International Federation of Clinical Neurophysiology, Electroencephalography and Clinical Neurophysiology Supplement, 52: , 3-6. [PubMed: 10590970] |
37 | Klomjai W. , Katz R. , & Lackmy-Vallée A. ((2015) ). Basic principles of transcranial magnetic stimulation (TMS) and repetitive TMS (rTMS), Annals of Physical and Rehabilitation Medicine, 58: (4), 208–213. https://doi.org/10.1016/j.rehab.2015.05.005 |
38 | Lang S. , Gan L. S. , Yoon E. J. , Hanganu A. , Kibreab M. , & Cheetham J. ((2020) ). Theta-Burst stimulation for cognitive enhancement in parkinson’s disease with mild cognitive impairment: A randomized, double-blind, sham-controlled trial, Frontiers in Neurology, 11: , 584374. https://doi.org/10.1016/j.rehab.2015.05.005 |
39 | Lefaucheur J. P. , André-Obadia N. , Antal A. , Ayache S. S. , Baeken C. , Benninger D. H. , Cantello R. M. , Cincotta M. , de Carvalho M. , De Ridder D. , Devanne H. , Di Lazzaro V. , Filipović S. R. , Hummel F. C. , Jääskeläinen S. K. , Kimiskidis V. K. , Koch G. , Langguth B. , Nyffeler T. , Oliviero A. , Padberg F. , Poulet E. , Rossi S. , Rossini P. M. , Rothwell J. C. , Schönfeldt-Lecuona C. , Siebner H. R. , Slotema C. W. , Stagg C. J. , Valls-Sole J. , Ziemann U. , Paulus W. , & Garcia-Larrea L. ((2014) ). Evidence-based guidelines on the therapeutic use of repetitive transcranial magnetic stimulation (rTMS), Clinical Neurophysiology, 125: (11), 2150–2206. https://doi.org/10.1016/j.clinph.2014.05.021 |
40 | Liu Y. , Luo J. , Fang J. , Yin M. , Cao J. , Zhang S. , Huang J. , Cheng Q. , Ai Y. , Zheng H. , & Hu X. ((2023) ). Screening diagnosis of executive dysfunction after ischemic stroke and the effects of transcranial magnetic stimulation: A prospective functional near-infrared spectroscopy study, CNS Neuroscience, & Therapeutics, 29: (6), 1561–1570. https://doi.org/10.1111/cns.14118 |
41 | Lourenço C. F. , Ledo A. , Barbosa R. M. , & Laranjinha J. ((2017) ). Neurovascular-neuroenergetic coupling axis in the brain: master regulation by nitric oxide and consequences in aging and neurodegeneration, Free Radical Biology and Medicine, 108: , 668–682. https://doi.org/10.1016/j.freeradbiomed.2017.04.026 |
42 | Luo X. , Qi Y. , Wang H. , Wang Y. , He T. , Rong B. , Liu Z. , Zhou Y. , Wang G. , & Wang H. ((2018) ). Prefrontal cortex dysfunction during verbal fluency task after atypicalantipsychotic treatment in schizophrenia: A near-infrared spectroscopy imaging study, Neuroscience Letters, 686: , 101–105. https://doi.org/10.1016/j.neulet.2018.09.001 |
43 | Marshall L. , & Binder S. ((2013) ). Contribution of transcranial oscillatory stimulation to research on neural networks: an emphasis on hippocampo-neocortical rhythms, Frontiers in Humam Neuroscience, 7: , 614. https://doi.org/10.3389/fnhum.2013.00614 |
44 | Menant J. C. , Maidan I. , Alcock L. , Al-Yahya E. , Cerasa A. , Clark D. J. , de Bruin E. D. , Fraser S. , Gramigna V. , Hamacher D. , Herold F. , Holtzer R. , Izzetoglu M. , Lim S. , Pantall A. , Pelicioni P. , Peters S. , Rosso A. L. , St George R. , Stuart S. , Vasta R. , Vitorio R. , & Mirelman A. ((2020) ). A consensus guide to using functional near-infrared spectroscopy in posture and gait research, Gait Posture, 82: , 254–265. https://doi.org/10.1016/j.gaitpost.2020.09.012 |
45 | Mihara M. , Fujimoto H. , Hattori N. , Otomune H. , Kajiyama Y. , Konaka K. , Watanabe Y. , Hiramatsu Y. , Sunada Y. , Miyai I. , & Mochizuki H. ((2021) ). Effect of neurofeedback facilitation on poststroke gait and balance recovery: A randomized controlled trial, Neurology, 96: (21), e2587–e2598. https://doi.org/10.1212/WNL.0000000000011989 |
46 | Mk Y. , & As C. ((2020) ). Functional near-infrared spectroscopy reveals decreased resting oxygenation levels and task-related oxygenation changes in mild cognitive impairment and dementia: A systematic review, Journal of Psychiatric Research, 124: , 58–76. https://doi.org/10.1016/j.jpsychires.2020.02.017 |
47 | Murphy K. , Khan A. , Bachu A. , & Tampi R. ((2023) ). Treatment of behavioral and psychological symptoms of dementia using transcranial magnetic stimulation: A systematic review, International Psychogeriatrics, 35: (11), 611–622. https://doi.org/10.1017/S1041610223000091 |
48 | Nie P. , Liu F. , Lin S. , Guo J. , Chen X. , Chen S. , Yu L. , & Lin R. ((2022) ). The effects of computer-assisted cognitive rehabilitation on cognitive impairment after stroke: A systematic review and meta-analysis, Journal of Clinical Nursing, 31: (9-10), 1136–1148. https://doi.org/10.1111/jocn.16030 |
49 | Nijsse B. , Visser-Meily J. M. A. , van Mierlo M. L. , Post M. W. M. , de Kort P. L. M. , & van Heugten C. M. ((2017) ). Temporal evolution of poststroke cognitive impairment using the montreal cognitive assessment, Stroke, 48: (1), 98–104. https://doi.org/10.1161/STROKEAHA.116.014168 |
50 | Nowak D. A. , Bösl K. , Podubeckà J. , & Carey J. R. ((2010) ). Noninvasive brain stimulation and motor recovery after stroke, Restorative Neurology and Neuroscience, 28: (4), 531–544. https://doi.org/10.3233/RNN-2010-0552 |
51 | Ohura T. , Hase K. , Nakajima Y. , & Nakayama T. ((2017) ). Validity and reliability of a performance evaluation tool based on the modified Barthel Index for stroke patients, BMC Medical Research Methodology, 17: (1), 131. https://doi.org/10.1186/s12874-017-0409-2 |
52 | Pabst A. , Proksch S. , Médé B. , Comstock D. C. , Ross J. M. , & Balasubramaniam R. ((2022) ). A systematic review and meta-analysis of the efficacy of intermittent theta burst stimulation (iTBS) on cognitive enhancement, Neuroscience and Biobehavioral Reviews, 135: , 104587. https://doi.org/10.1016/j.neubiorev.2022.104587 |
53 | Peng C. Y. , Chen Y. C. , Cui Y. , Zhao D. L. , Jiao Y. , Tang T. Y. , Ju S. , & Teng G. J. ((2016) ). Regional coherence alterations revealed by resting-state fMRI in post-stroke patients with cognitive dysfunction, PloS One, 11: (7), e0159574. https://doi.org/10.1371/journal.pone.0159574 |
54 | Pinti P. , Tachtsidis I. , Hamilton A. , Hirsch J. , Aichelburg C. , & Gilbert S. ((2020) ). The present and future use of functional near-infrared spectroscopy (fNIRS) for cognitive neuroscience, Annals of the New York Academy of Sciences, 1464: (1), 5–29. https://doi.org/10.1111/nyas.13948 |
55 | Pirondini E. , Kinany N. , Sueur C. L. , Griffis J. C. , Shulman G. L. , Corbetta M. , & Van De Ville D. ((2022) ). Post-stroke reorganization of transient brain activity characterizes deficits and recovery of cognitive functions, NeuroImage, 255: , 119201. https://doi.org/10.1016/j.neuroimage.2022.119201 |
56 | Quinn T. J. , Richard E. , Teuschl Y. , Gattringer T. , Hafdi M. , O’Brien J. T. , Merriman N. , Gillebert C. , Huyglier H. , Verdelho A. , Schmidt R. , Ghaziani E. , Forchammer H. , Pendlebury S. T. , Bruffaerts R. , Mijajlovic M. , Drozdowska B. A. , Ball E. , & Markus HS. ((2021) ). European Stroke Organisation and European Academy of Neurology joint guidelines on post-stroke cognitive impairment, European Journal of Neurology, 28: (12), 3883–3920. https://doi.org/10.1177/23969873211042192 |
57 | Rahman M. A. , Siddik A. B. , Ghosh T. K. , Khanam F. , & Ahmad M. ((2020) ). A narrative review on clinical applications of fNIRS, Journal of Digital Imaging, 33: (5), 1167–1184. https://doi.org/10.1007/s10278-020-00387-1 |
58 | Rost N. S. , Brodtmann A. , Pase M. P. , van Veluw S. J. , Biffi A. , Duering M. , Hinman J. D. , & Dichgans M. ((2022) ). Post-stroke cognitive impairment and dementia, Circulation Research, 130: (8), 1252–1271. https://doi.org/10.1161/CIRCRESAHA.122.319951 |
59 | Rotenberg A. , Horvath J. C. , & Pascual-Leone A. ((2014) ). Transcranial magnetic stimulation. New York: Humana Press: Springer, 85. |
60 | Rudebeck P. H. , & Rich E. L. ((2018) ). Orbitofrontal cortex, Current Biology, 28: (18), R1083–R1088. https://doi.org/10.1016/j.cub.2018.07.018 |
61 | Sabbagh M. , Sadowsky C. , Tousi B. , Agronin M. E. , Alva G. , Armon C. , Bernick C. , Keegan A. P. , Karantzoulis S. , Baror E. , Ploznik M. , & Pascual-Leone A. ((2020) ). Effects of a combined transcranial magnetic stimulation (TMS) and cognitive training intervention in patients with Alzheimer’s disease, Alzheimers, & Dementia, 16: (4), 641–650. https://doi.org/10.1016/j.jalz.2019.08.197 |
62 | Schulz K. F. , Altman D. G. , & Moher D. ((2010) ). CONSORT statement: Updated guidelines for reporting parallel group randomised trials, Journal of Pharmacology, & Pharmacotherapeutics, 1: (2), 100–107. https://doi.org/10.4103/0976-500X.72352 |
63 | Segarra M. , Aburto M. R. , Hefendehl J. , & Acker-Palmer A. ((2019) ). Neurovascular interactions in the nervous system, Annual Review of Cell and Developmental Biology, 35: , 615–635. https://doi.org/10.1146/annurev-cellbio-100818-125142 |
64 | Shah S. , Vanclay F. , & Cooper B. ((1989) ). Improving the sensitivity of the Barthel Index for stroke rehabilitation, Journal of Clinical Epidemiology, 42: (8), 703–709. https://doi.org/10.1016/0895-4356(89)90065-6 |
65 | Stanojevic J. , Dragic M. , Stevanovic I. , Ilic T. , Stojanovic I. , Zeljkovic M. , & Ninkovic M. ((2022) ). Intermittent theta burst stimulation ameliorates cognitive impairment and hippocampal gliosis in the Streptozotocin-induced model of Alzheimer’s disease, Behavioural Brain Research, 433: , 113984. https://doi.org/10.1016/j.bbr.2022.113984 |
66 | Strangman G. , Culver J. P. , Thompson J. H. , & Boas D. A. ((2002) ). A quantitative comparison of simultaneous BOLD fMRI and NIRS recordings during functional brain activation, NeuroImage, 17: (2), 719–731. https://doi.org/10.1016/s1053-8119(02)91227-9 |
67 | Struckmann W. , Persson J. , Weigl W. , Gingnell M. , & Bodén R. ((2021) ). Modulation of the prefrontal blood oxygenation response to intermittent theta-burst stimulation in depression: A sham-controlled study with functional near-infrared spectroscopy, World Journal of Biological Psychiatry, 22: (4), 247–256. https://doi.org/10.1080/15622975.2020.1785007 |
68 | Tomassoni D. , Lanari A. , Silvestrelli G. , Traini E. , & Amenta F. ((2008) ). Nimodipine and its use in cerebrovascular disease: evidence from recent preclinical and controlled clinical studies, Clinical and Experimental Hypertension, 30: (8), 744–766. |
69 | Wang S. Y. , Gong Z. K. , Sen J. , Han L. , Zhang M. , & Chen W. ((2014) ). The usefulness of the Loewenstein Occupational Therapy Cognition Assessment in evaluating cognitive function in patients with stroke, European Review for Medical and Pharmacological Sciences, 18: (23), 3665–3672. [PubMed: 25535138] |
70 | Wang Y. , Duan W. , Dong D. , Ding L. , & Lei X. ((2022) ). A test-retest resting, and cognitive state EEG dataset during multiple subject-driven states, Scientific Data, 9: (1), 566. https://doi.org/10.1038/s41597-022-01607-9 |
71 | Wei Y. , Tang X. , Zhang T. , Su W. , Xu L. , Cui H. , Qian Z. , Zhang T. , & Wang J. ((2022) ). Reduced temporal activation during a verbal fluency test in clinical high risk of psychosis: A functional near-infrared spectroscopy-based study, General Psychiatry, 35: (2), e100702. https://doi.org/10.1136/gpsych-2021-100702 |
72 | Wischnewski M. , & Schutter D. J. L. G. ((2015) ). Efficacy and Time Course of Theta Burst Stimulation in Healthy Humans, Brain Stimulation, 8: (4), 685–692. https://doi.org/10.1016/j.brs.2015.03.004 |
73 | Wu X. , Ji G. J. , Geng Z. , Wang L. , Yan Y. , Wu Y. , Xiao G. , Gao L. , Wei Q. , Zhou S. , Wei L. , Tian Y. , & Wang K. ((2022) ). Accelerated intermittent theta-burst stimulation broadly ameliorates symptoms and cognition in Alzheimer’s disease: A randomized controlled trial, Brain Stimulation, 15: (1), 35–45. https://doi.org/10.1016/j.brs.2021.11.007 |
74 | Xia X. , Li Y. , Wang Y. , Xia J. , Lin Y. , Zhang X. , Liu Y. , & Zhang J. ((2021) ). Functional role of dorsolateral prefrontal cortex in the modulation of cognitive bias, Psychophysiology, 58: (10), e13894. https://doi.org/10.1111/psy13894 |
75 | Yamaya N. , Tsuchiya K. , Takizawa I. , Shimoda K. , Kitazawa K. , & Tozato F. ((2021) ). Effect of one-session focused attention meditation on the working memory capacity of meditation novices: A functional near-infrared spectroscopy study, Brain and Behavior, 11: (8), e2288. https://doi.org/10.1002/brb3.2288 |
76 | Yang C. , Zhang T. , Huang K. , Xiong M. , Liu H. , Wang P. , & Zhang Y. ((2022) ). Increased both cortical activation and functional connectivity after transcranial direct current stimulation in patients with post-stroke: A functional near-infrared spectroscopy study, Frontiers in Psychiatry, 13: , 1046849. https://doi.org/10.3389/fpsyt.2022.1046849 |
77 | Yang T. , Wang H. , Dai H. , Hui J. , Zhang J. , Li J. , Cui G. , Wang J. , Mu J. , & Zhang Z. ((2023) ). The fNIRS evaluation of frontal and temporal lobe cortical activation in Chinese first-episode medication-naïve and recurrent depression during a verbal fluency task, Frontiers in Psychiatry, 14: , 1132666. https://doi.org/10.3389/fpsyt.2023.1132666 |
78 | Yin M. , Liu Y. , Zhang L. , Zheng H. , Peng L. , Ai Y. , Luo J. , & Hu X. ((2020) ). Effects of rTMS treatment on cognitive impairment and resting-state brain activity in stroke patients: A randomized clinical trial, Frontiers in Neural Circuits, 14: , 563777. https://doi.org/10.3389/fncir.2020.563777 |
79 | Zhang F. , Qin Y. , Xie L. , Zheng C. , Huang X. , & Zhang M. ((2019) ). High-frequency repetitive transcranial magnetic stimulation combined with cognitive training improves cognitive function and cortical metabolic ratios in Alzheimer’s disease, Journal of Neural Transmission, 126: (8), 1081–1094. https://doi.org/10.1007/s00702-019-02022-y |
80 | Zhang Y. , Chu M. , Zheng Y. , Zhang F. , Yu H. , & Ye X. ((2023) ). Effects of combined use of intermittent theta burst stimulation and cognitive training on post-stroke cognitive impairment: A single-blind randomized controlled trial, American Journal of Physical Medicine, & Rehabilitation, 103: (4), 318–324. https://doi.org/10.1097/PHM.0000000000002344 |
81 | Zheng B. , Chen J. , Cao M. , Zhang Y. , Chen S. , Yu H. , & Liang K. ((2024) ). The effect of intermittent theta burst stimulation for cognitive dysfunction: A meta-analysis, Brain Injury, 23: , 1–12. https://doi.org/10.1080/02699052.2024.2344087 |
82 | Zhu W. M. , Neuhaus A. , Beard D. J. , Sutherland B. A. , & DeLuca G. C. ((2022) ). Neurovascular coupling mechanisms in health and neurovascular uncoupling in Alzheimer’s disease, Brain, 145: (7), 2276–2292. https://doi.org/10.1093/brain/awac174 |