Influence of polymerized siloxanes on growth of nosocomial aerobic bacteria
Abstract
Health care associated infections are the fourth leading cause of disease in industrialized countries, and the most common complication affecting hospitalized patients. Recently a polymerized siloxane coating substrate was suggested as a promising candidate for the coating of materials which are aimed to be used in cardiovascular tissue engineering. To reduce the risk of tissue remodeling failure after implantation of such engineered tissue implants, coatings substrates for tissue engineering scaffolds might be helpful to lower the risk of bacterial attachment and growth during and after implantation.
In the presented study a coating based on polymerized siloxanes was tested with respect to these properties. Tests were performed with aerobic nosocomial bacteria (Escherichia coli and Staphylococcus epidermidis) and an exemplary selection of materials (stainless steel, polycarbonate, soda-lime glass). The siloxane coating was able to significantly reduce bacterial adherence, and it is supposed that this effect is mainly a result of the high hydrophobicity of the coating substrate.
1Introduction
Recently a substrate based on polymerized siloxanes was suggested as a promising candidate for the coating of materials which are aimed to be used in cardiovascular tissue engineering approaches. The siloxanes become electrostatically bond on biomaterials creating a hydrophobic surface [1]. Surface hydrophobicity is well known as a key factor to govern bacterial cell attachment. It was shown that coating of exemplary biomaterials like silicone with an aqueous emulsion of polydimethylsiloxane (PDMS) did not negatively impact cell morphology and cell layer formation [1]. Cell layer confluence was even higher after cell growth with extracts of the coated materials in contrast to cell growth in extracts of the non-coated samples. Additionally the PDMS coating resulted in a decreased LDH release, and in the literature it is described that silica conditioned media significantly induce proliferation of fibroblasts from rats (RAT-2 cells) [2] and hamsters [3] and also from other cell types (e.g. osteoblasts [4, 5]).
However, before PDMS-based coating substrates can be used for therapeutic approaches it has to be guaranteed, that the coating does not promote bacterial growth. The latter can cause destruction of the engineered tissue and engineered tissue failure respectively. Main factors influencing bacterial reactivity and bacterial growth on siloxane surfaces are the local charge distribution on the surface [6], and the surface hydrophobicity [7, 8]. In the absence of nearby isomorphic cation substitutions that create negative charge a siloxane surface functions only as a mild charge donor [9]. The predominant effect of the siloxane coating comes therefore from the hydrophobicity, which makes the surface less resistant to bacterial adhesion than hydrophilic surfaces [10, 11].
The presented study aimed to evaluate the potential of the polymerized siloxane coating to influence bacterial growth. Test were performed with exemplary nosocomial gram-negative and gram-positive bacteria (Escherichia coli, Staphylococcus epidermidis), and an exemplary selection of materials (stainless steel, polycarbonate, soda-lime glass).
2Experimental
2.1Bacteria
Tests were performed with an S. epidermidis strain provided by ATCC (strain 12228). Additionally an E. coli strain was used that was isolated from the feces of a conventional housed C57Bl/6N mouse. Strain identification was done with an automated system (Vitek 2 System, Biomérieux). To exclude tests with E. coli parovars (EAEC, EHEC, EPEC, EIEC, ETEC) real-time multiplex PCR was performed (R-Biopharm). S. epidermidis was used as a representative for nosocomial aerob gram-positive bacteria, and E. coli served as representative for nosocomial aerob gram-negative bacteria. Both bacterial strains were initially grown on glucose nutrient agar plates (2 vol-% agar, SIFIN) at 36°C, and then transferred into glucose nutrient broth to establish suspension cultures (36°C, 100 rpm). Bacterial growth was controlled by measuring optical density at 590 nm. For test purposes the exact bacterial concentration was calculated by plating the suspended bacteria on glucose nutrient agar plates and subsequent counting of the colony forming units (cfu).
After 1 hour of incubation of the samples in the bacterial suspension at 36°C under permanent mild shaking at 100 rpm samples were washed three times in sterile physiological saline solution to get rid of the non- or loosely adherent bacteria. Thereafter samples were transferred into sterile physiological saline solution, and bacteria were detached by ultrasonic treatment (1 min, room temperature, frequency: 60 Hz, non-modulated). Detached bacteria were quantified by spread-plate method as described above.
2.2Materials
Tests were performed using discs (diameter 2.6 cm, thickness 0.2 cm) with a centered fixation hole (diameter 0.5 cm). The discs were made of stainless steel, polycarbonate or soda-lime glass, respectively. All discs were cleaned before usage. The cleaning procedure started with incubating all discs for 12 hrs in 100% ethanol (Sigma Aldrich), and a subsequent treatment for 20 min in an ultrasonic bath (35 kHZ, Elmasonic One) filled with ethanol. Thereafter the discs were rinsed three times with ddH2O (sterile, endotoxin-free), dried at 37°C for 2 hrs, coated with the PDMS substrate, and sterilized using autoclaving (121°C, 20 min).
The discs made of soda lime glass were initially rinsed in acetone, then for 12 hrs in 2% HCl, and finally once with ddH2O, before the cleaning procedure was started.
2.3Coating substrate
The coating substrate consisted of an aqueous emulsion of polydimethylsiloxane (PERMANON), which was used with and without supplementation of 0.5 vol-% of a quaternary ammonium compound (Advisal 400, ENVISAL, Reppenstedt, Germany). The constitution of the PDMS coating substrate is described in more details elsewhere [1].
2.4Coating
Dip coating was used to apply the polymerized siloxanes onto the materials. Therefore the materials were dipped into an aqueous emulsion of 2.0 vol-% PDMS three times for 10 min each (overall coating time 30 min) at 22±2°C.
2.5Counting of adherent bacteria
Detached bacteria were serial diluted with physiological saline solution and plated with T-spatula on glucose nutrient agar plates. Plates were incubated overnight at 36°C. CFU were counted manually and values converted to original concentration.
2.6Scanning electron microscopic (SEM) examination
The samples were washed two times in 0.1 M cacodylate buffer at 21°C, three times in physiological saline solution, transferred into a modified Karnovsky solution (7.5% glutaraldehyde, 3% paraformaldehyde, buffered at pH 7.4) for 24 hours at 7°C, and afterwards postfixed in 1% osmium tetroxide mixed with 0.2 M cacodylate buffer. The postfixed samples were washed twice with buffer, dehydrated in an ascending series of ethanol (starting with 30%, and finally into two changes of absolute ethanol) and then covered twice with hexamethyldisilazane (HMDS, Roth) for 15 minutes. After drying overnight under the fume hood to remove HDMS, the samples were sputter-coated with gold-palladium for 2 min (approx. thickness 2 nm) and examined using a FEI Quanta 200 ESEM at an accelerating voltage of 15 kV.
2.7Statistics
Data were reported as mean value±standard deviation for continuous variables, and were analysed by Student’s t-test (paired). A p value of less than 0.05 was considered significant.
3Results
The number of adherent bacteria was highest on PDMS polycarbonate and lowest on PDMS coated glass (p < 0.05, Fig. 1), and on all coated materials S. epidermidis was present in significantly higher numbers than E. coli.
However, the PDMS coating proved to be able to lower the adherence of E. coli and S. epidermidis. The number of adherent E. coli (see Fig. 2) and S. epidermidis on PDMS coated stainless steel, polycarbonate, and glass was reduced in comparison to the number of adherent bacteria on the non-coated materials (p < 0.05), with PDMS coating of stainless steel being most effective for adherence reduction of both bacterial strains (see Fig. 3).
Exemplarily tests with glass coated with the polysiloxane emulsion after supplementation with a quaternary ammonium compound revealed comparable results to the tests with the non-supplemented coating substrate. After 1 hr of incubation the number of adherent E. coli was not significantly different between glasses which were coated with PDMS and disinfectant and with non-supplemented PDMS (see Fig. 4).
4Discussion
Most bacteria are able to adhere to material surfaces and to form complex and heterogeneous microbial communities and biofilms [12]. Significant efforts are currently being directed to prevention of bacterial adhesion and subsequent formation of biofilms. Many surface modification techniques have been used to investigate inhibition of bacterial adhesion and biofilm formation. Among these methods chemical coating strategies are promising approaches for bacterial adherence reduction [13–16]. The presented study was aimed to test the effect of a PDMS coating substrate on bacterial adherence, and proved this substrate to be able to reduce adherence of defined aerobic nosocomial bacterial strains(E. coli, S. epidermidis).
Bacterial adhesion is generally described as a twostep process. Initially the bacteria became reversible bonded to the material surface based on physico-chemical forces. Thereafter the contact becomes irreversible due to hydrophobic interactions and interactions between functional groups on bacterial cells and material surfaces [13]. It has been reported that physico-chemical properties, e.g. surface potential, roughness and hydrophobicity, affect the rate of the initial bacterial adhesion [17–20]. Hydrophilic materials are described to be more resistant to bacterial adhesion than hydrophobic materials. For instance in a study of Fletcher and Loeb [23] the adherence of Pseudomonas sp. to hydrophobic plastics with low surface charges was pronounced in comparison to hydrophilic metals with a positive or neutral surface charge, and glass which is characterized by a hydrophilic and negatively charged surface.
Beside of the hydrophobicity of the material surface also the hydrophobicity of the bacterial cell wall determines bacterial adhesion [21–24]. The hydrophobicity of bacteria varies according to bacterial species and is influenced by growth medium, bacteria age, and bacterial surface structure. In the presented study the bacterial were exposed to the materials in a liquid, and were therefore expected to be tested with a hydrophilic cell wall. The polysiloxane coating established a hydrophobic surface on the tested materials [1], and resulted in a change of the metal and glass surface from hydrophilic to hydrophobic. Due to this change of the surface hydrophobicity the number of E. coli and S. epidermidis that became adherent to these materials might have been reduced after surface coating.
5Conclusion
The hydrophilic siloxane coating was able to lower bacterial adherence of aerobic nosocomial bacteria (E. coli, S. epidermidis) on stainless steel, polycarbonate, and soda-lime glass. Bacterial adherence on the polysiloxane coating alone was not different to adherence after supplementation with 0.5 vol-% of a quaternary ammonium compound.
Acknowledgments
We thank R. Eichentopf for supporting the isolation of an E. coli strain from feces und Dr. G. Hause for providing Karnovsky solution.
References
[1] | Hiebl B , Hopperdietzel C , Hünigen H , Jung F , Scharnagl N . Influence of a silicon (Si14)-based coating substrate for biomaterials on fibroblast growth and human C5a. Clin Hemorheol Microcirc (2013) ;55: (4):491–9. |
[2] | Valentini RF , Vargo TG , Gardella Jr JA , Aebischer P . Electrically charged polymeric substrates enhance nerve fibre outgrowth in vitro, Biomaterials (1992) ;13: :183–90. |
[3] | Valerio P , Pereira MM , Goes AM , Leite MF . The effect of ionic products from bioactive glass dissolution on osteoblast proliferation and collagen production, Biomaterials (2004) ;25: :2941–8. |
[4] | Williams DF . On the mechanisms of biocompatibility. Biomaterials (2008) ;29: :2941–53. |
[5] | Wu C , Zhou Y , Fan W , Han P , Chang J , Yuen J , Zhang M , Xiao Y . Hypoxia-mimicking mesoporous bioactive glass scaffolds with controllable cobalt ion release for bone tissue engineering. Biomaterials (2012) ;33: :2076–85. |
[6] | Hogt AH , Dankert J , Feijen J . Adhesion of Staphylococcus epidermidis and Staphylococcus saprophyticus to a hydrophobic biomaterials. J Gen Microbiol (1985) ;131: :2485–91. |
[7] | Reynolds EC , Wong A . Effect of adsorbed protein on hydroxyapatite zeta potential and Streptococcus mutans adherence. Infect Immunol (1983) ;39: :1285–90. |
[8] | Pringle JH , Fletcher M . Influence of substratum hydration and adsorbed macromolecules on bacterial attachment to surfaces. Appl Environ Microbiol (1986) ;51: :1321–5. |
[9] | Sposito G , Skipper NT , Sutton R , Park SH , Soper AK , Greathouse JK . Surface geochemistry of the clay minerals. PNAS (1999) ;96: :3358–64. |
[10] | Hogt AH , Dankert J , de Vries JA , Feijen J . Adhesion of coagulase-negative staphylococci to biomaterials. J Gen Microbiol (1983) ;129: :1959–68. |
[11] | Ludwicka A , Jansen B , Wadstrom T , Pulverer G . At tachment of staphylococci to various synthetic polymers. Zbl Bakt Hyg A (1984) ;256: :479–89. |
[12] | Bos R , van der Mei HC , Busscher HJ . Physicochemistry of initial microbial adhesive interactions – its mechanisms and methods for study. FEMS Microbiol Rev (1999) ;23: :179–230. |
[13] | Terada , Yuasa A , Kushimoto T , Tsuneda S , Katakai A , Tamada M . Bacterial adhesion to and viability on positivelycharged polymer surfaces. Microbiolgy (2006) ;152: :3575–83. |
[14] | Groll J , Fiedler J , Bruellhoff K , Moeller M , Brenner RE . Novel surface coatings modulating eukaryotic cell adhesion and preventing implant infection. Int J Artif Organs (2009) ;32: (9):655–62. |
[15] | Darouiche RO . Antimicrobial coating of devices for prevention of infection: Principles and protection. Int J Artif Organs (2007) ;30: (9):820–7. |
[16] | Darouiche RO . Device-associated infections: A macroproblem that starts with microadherence. Clin Infect Dis (2001) ;33: (9):1567–72. |
[17] | Gottenbos B , van der Mei HC , Busscher HJ , Grijpma DW , Feijnen J . Initial adhesion and surface growth of Pseudomonas aeruginosa on negatively and positively charged poly(methacrylates). J Mater Sci Mater Med (1999) ;10: :853–5. |
[18] | Gottenbos B , van der Mei HC , Busscher HJ . Initial adhesion and surface growth of Staphylococcus epidermidis and Pseudomonas aeruginosa on biomedical polymers. J Biomed Mater Res (2000) ;50: :208–14. |
[19] | Gottenbos B , Grijpma DW , van der Mei HC , Feijnen J , Busscher HJ . Antimicrobial effects of positively charged surface on adhering Gram-positive and Gram-negative bacteria. J Antimicrob Chemother (2001) ;48: :7–13. |
[20] | Hibiya K , Tsuneda S , Hirata A . Formation and characteristics of nitrifying biofilm on a membrane modified with positively-charged polymer chains. Colloids Surf B Biointerfaces (2000) ;18: :105–12. |
[21] | Hogt AH , Dankert J , de Vries JA , Feijen J . Adhesion of coagulase-negative staphylococci to biomaterials. J Gen Microbiol (1983) ;129: :168–95. |
[22] | Satou N , Satou J , Shintani H , Okuda K . Adherence of streptococci to surface-modified glass. J Gen Microbiol (1988) ;134: :1299–1305. |
[23] | Fletcher M , Loeb GI . Influence of substratum character istics on the attachment of a marine pseudomonad to solid surfaces. Appl Environ Microbiol (1979) ;37: :67–72. |
[24] | Ludwicka A , Jansen B , Wadstrom T , Pulverer G . Attachment of staphylococci to various synthetic polymers. Zbl Bakt Hyg A (1984) ;256: :479–89. |
Figures and Tables
Fig.1
Bacterial adherence on stainless steel, polycarbonate, and glass after coating with 2 vol-% PDMS, and subsequent incubation in a bacterial suspension (0.2 OD590 nm in glucose nutrient broth) for 1 hr; number of adherent bacteria expressed in as 103cfu/ml; means and standard deviation, n = 10.
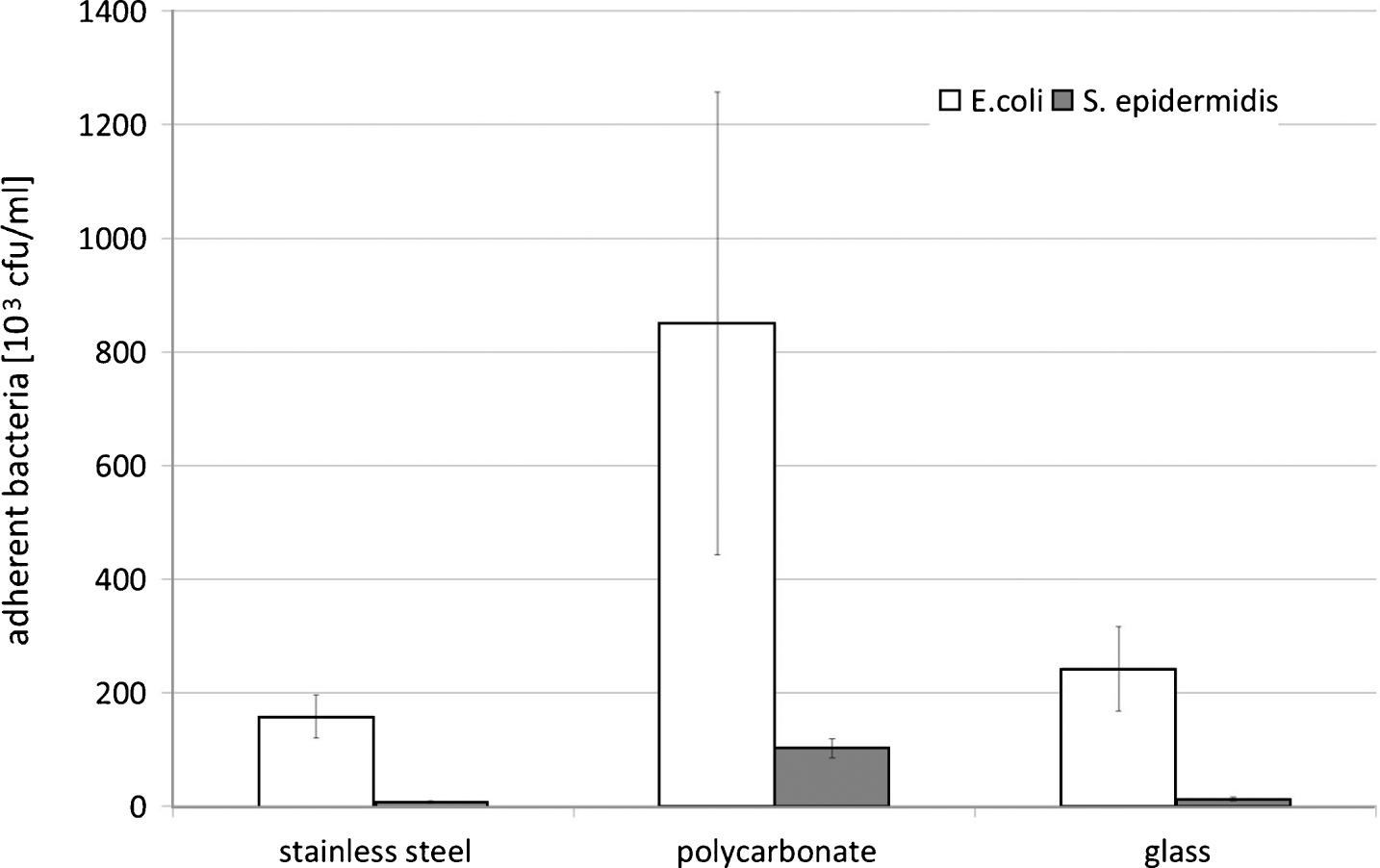
Fig.2
Bacterial adherence on stainless steel, polycarbonate, and glass after coating with 2 vol-% PDMS, and subsequent incubation in a bacterial suspension (0.2 OD590 nm in glucose nutrient broth) for 1 hr; number of adherent bacteria expressed in percentage of adherent cfu on non-coated glass; means and standard deviation, n = 10.
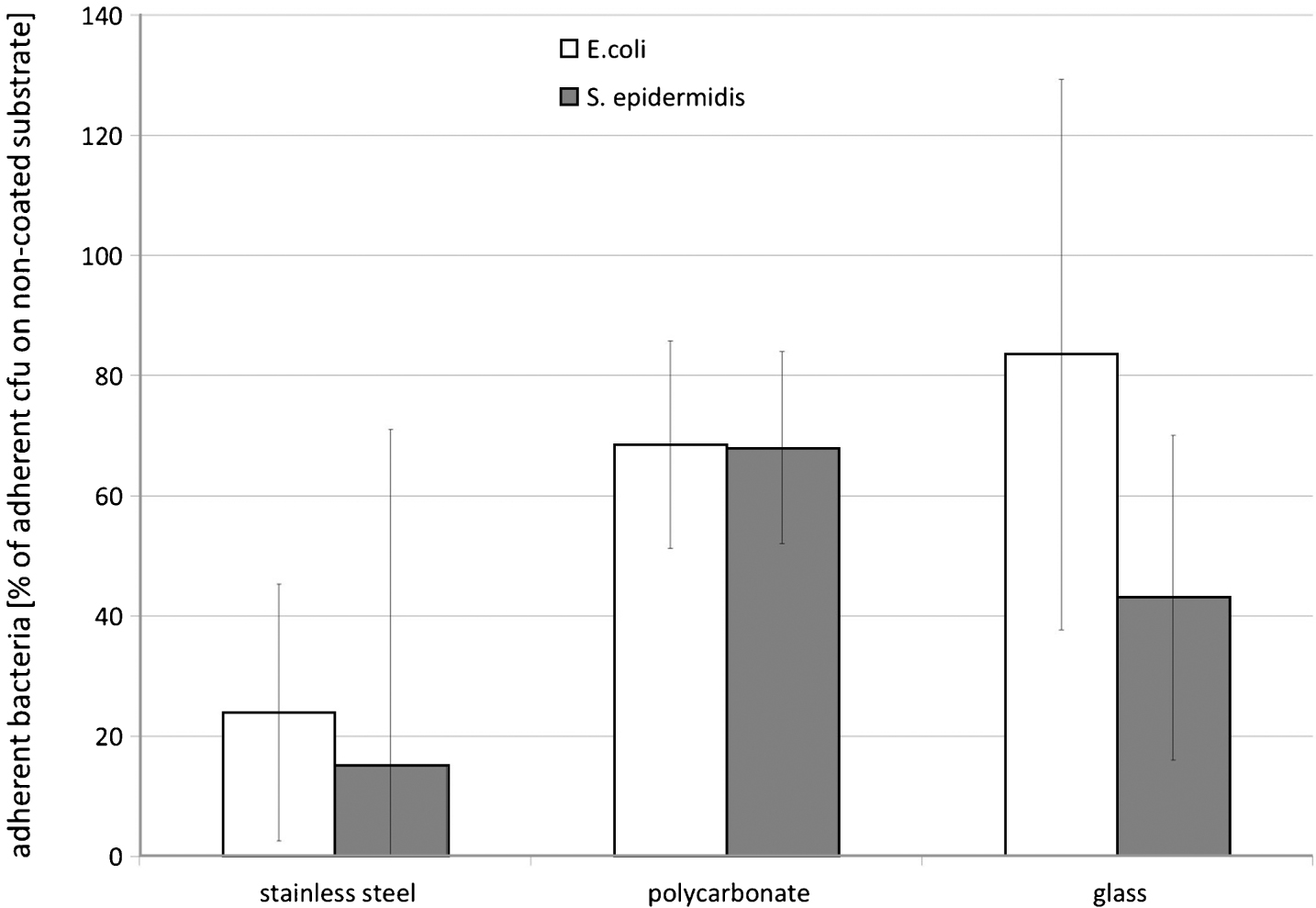
Fig.3
Bacterial adherence on glass, polycarbonate (PC), and stainless steel after coating with 2 vol-% PDMS (w/siloxanes), and subsequent incubation in a E. coli-suspension (0.2 OD590 nm in glucose nutrient broth) for 1 hr, scanning electron microscopy.
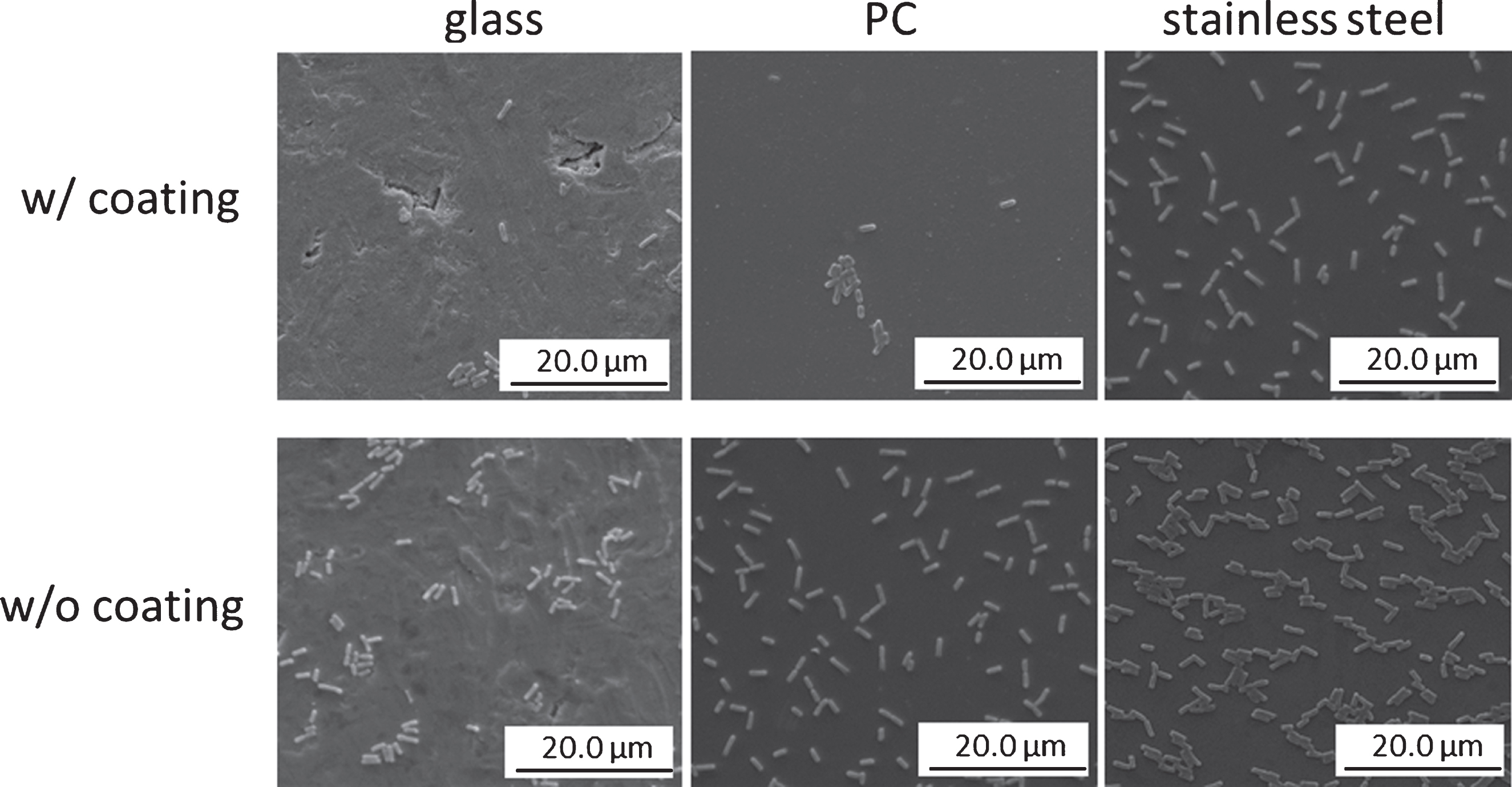
Fig.4
E. coli-adherence after incubation of glass coated with 2 vol-% PDMS (w/siloxanes), or with 2 vol-% PDMS plus 0.5-vol% disinfectant (Advisal 400, ENVISAL; w/siloxanes+disinfectant) in a E. coli-suspension (0.2 OD590 nm in glucose nutrient broth) for 1 hr; number of adherent bacteria expressed in percentage of adherent cfu on non-coated glass; means and standard deviation, n = 10.
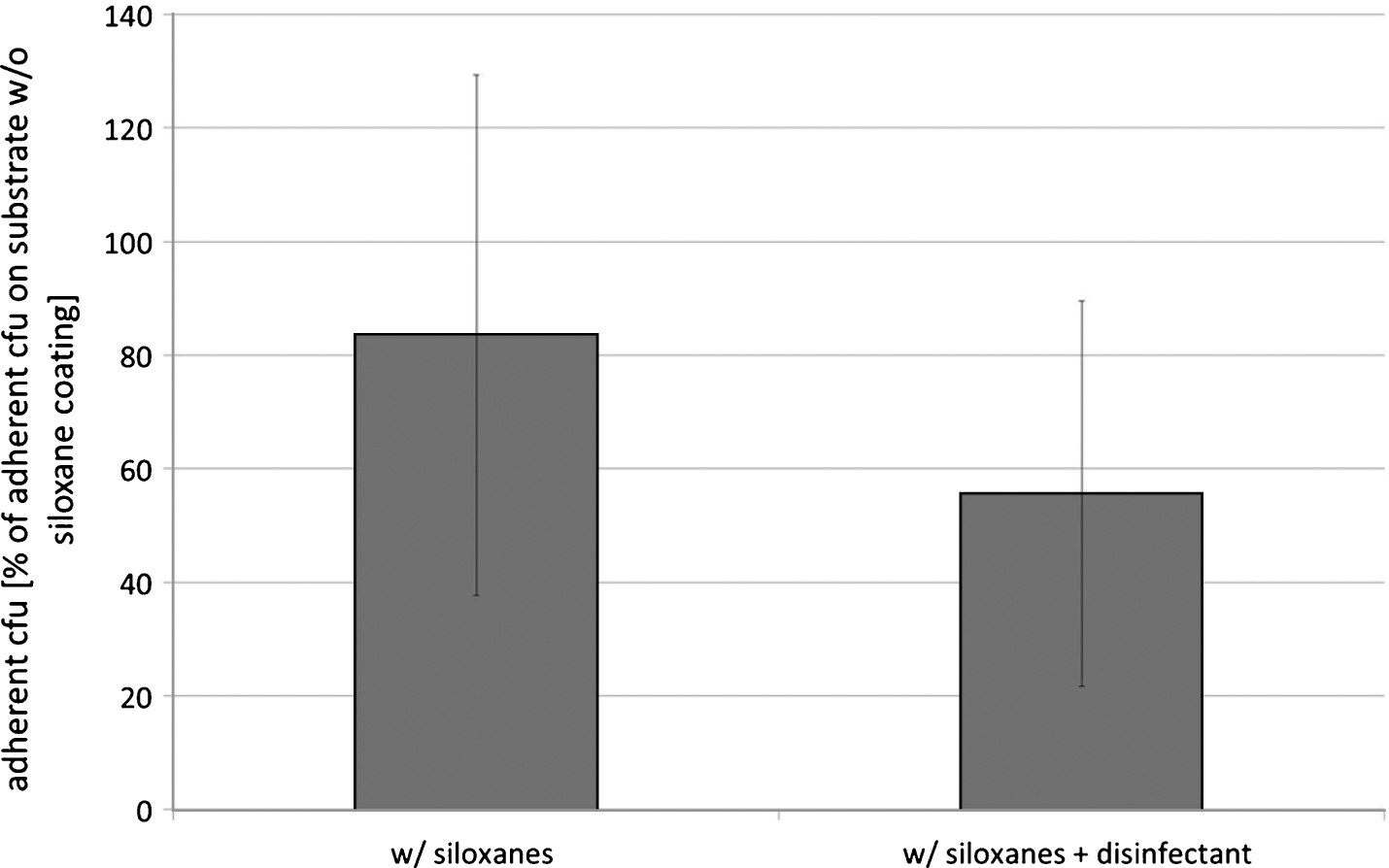