Moonlighting proteins - an approach to systematize the concept
Abstract
Moonlighting refers to a protein with at least two unrelated, mechanistically different functions. As a concept, moonlighting describes a large and diverse group of proteins which have been discovered in a multitude of organisms. As of today, a systematized view on these proteins is missing. Here, we propose a classification of moonlighting proteins by two classifiers. We use the function of the protein as a first classifier: activating - activating (Type I), activating - inhibiting (Type II), inhibiting - activating (Type III) and inhibiting - inhibiting (Type IV). To further specify the type of moonlighting protein, we used a second classifier based on the character of the factor that switches the function of the protein: external factor affecting the protein (Type A), change in the first pathway (Type B), change in the second pathway (Type C), equal competition between both pathways (Type D). Using a small two-pathway model we simulated these types of moonlighting proteins to elucidate possible behaviors of the types of moonlighting proteins. We find that, using the results of our simulations, we can classify the behavior of the moonlighting types into Blinker, Splitter andSwitch.
1Background
The term moonlighting comes from economics, where it describes a person taking two jobs [1, 2]. In biology, it refers to a protein with at least two unrelated, mechanistically different functions, which are performed by the same domain in the protein. These two functions should be independent of each other, meaning that a mutation, which impedes one function, should not affect the other.
Moonlighting is conceptually different from multi-functional proteins, referring to, e.g., an enzyme which has multiple different substrates [1, 3–6].
As a concept, moonlighting is used to describe a rather large and diverse group of proteins. Moon-lighting proteins have been discovered in many different organisms, including bacteria, yeast, plants and vertebrates [6]. In all of these organisms, moonlighting proteins have diverse first functions and also take very different secondary functions. It seems that often metabolic enzymes moonlight, taking a second function in signaling or regulation of, for example, transcription or translation [6]. In some cases these two functions link two related or dependent processes. An example for this is Aconitase from Mycobacterium tuberculosis [7–9], which needs an iron cluster to perform its function in the TCA cycle, where it converts isocitrate to cis-aconitate. Upon losing this iron cluster due to low iron concentrations in the cell, it binds mRNA molecules to upregulate the iron uptake into the cell (Fig. 1a). Another example of a moonlighting protein linking two related pathways is DLA2 from the chloroplast of Chlamydomonas reinhardtii [10, 11]. This protein is a part of the Pyruvate Dehydrogenase Complex (PDC), which converts pyruvate to acetyl-CoA [10, 12]. The acetyl-CoA is further converted to malonyl-CoA and used to synthesize thylakoid membrane [13, 14]. If acetyl-CoA can be directly produced from acetate, the DLA2 can leave the PDC and bind mRNA, thereby upregulating the translation of the D1 subunit of Photosystem II, which is inserted into the newly synthesized membranes (Fig. 1b). This way DLA2 links a metabolic pathway to the regulation of translation [10, 12]. Also in glycolysis moonlighting proteins have been found, seemingly linking metabolism to signaling. Hexokinase is the enzyme responsible for trapping glucose in the cell, by converting glucose to glucose-6-phosphate [15]. In yeast, this enzyme has been found to bind the transcription factor Mig1 and take part in the repression of genes involved in carbohydrate metabolism [16, 17]. A large group of moonlighting proteins also seems to perform different tasks outside and inside of the cell, for example phosphoglucose isomerase, which is another enzyme involved in glycolysis. This enzyme has several secondary functions when being secreted by the cell, for example as Neuroleukin, which stimulates the survival of embryonic neurons [18, 19].
Fig.1
Two examples of moonlighting proteins. A) Aconitase is a TCA cycle enzyme from Mycobacterium tuberculosis, which can bind mRNA upon iron depletion [7–9]. B) Hexokinase in yeast cells has been found in the nucleus, binding the transcription factor Mig1[16, 17].
![Two examples of moonlighting proteins. A) Aconitase is a TCA cycle enzyme from Mycobacterium tuberculosis, which can bind mRNA upon iron depletion [7–9]. B) Hexokinase in yeast cells has been found in the nucleus, binding the transcription factor Mig1[16, 17].](https://content.iospress.com:443/media/isb/2020/13-3-4/isb-13-3-4-isb190473/isb-13-isb190473-g001.jpg)
For many moonlighting proteins, the mechanism that makes them switch between their two functions is not clear. There are cases, in which loss of a cofactor (for example the iron cluster in aconitase) or a modification on the moonlighting protein itself, provokes the switch from one function to the other. Many moonlighting proteins seem to perform their different tasks in different compartments of the cell, different cell types or intra- andextra-cellularly.
Moonlighting seems to play an important role in many cellular functions in different organisms and has also been found in human cells [18, 19]. The discovery of new moonlighting proteins has mostly been based on chance encounters of a secondary function of known proteins. Reports about moonlighting proteins mostly describe the newly discovered function, but a systematized view on the concept of moonlighting is missing. Here, we propose a classification of moonlighting proteins by two classifiers, sorting them into types according to their function in the pathways they participate in and the factor that switches their function. This leads to sixteen types of moonlighting proteins. Using ODE modeling we exemplarily simulated possible behaviors of these sixteen types and analyzed the emerging system characteristics. We find that most reported moonlighting proteins can be described by our classification and we propose the usage of this classification to make communication on new findings more efficient.
2Results
2.1Classification of moonlighting proteins
To systematize the research on moonlighting proteins, we propose a classification of these proteins based on their function in the two pathways they participate in and the factor that switches their function and makes them change from one pathway to the other.
The moonlighting protein can either be activating or inhibiting in each of these pathways. This means there are four possible combinations, leading to four main classes of moonlighting proteins:activating - activating (Type I), activating - inhibiting (Type II), inhibiting - activating (Type III) and inhibiting - inhibiting (Type IV) (see Table 1a). The difference between Type II and Type III will be explained below. To further specify the type of moonlighting protein, we used a second classifier based on the character of the factor that switches the function of the protein. This classification leads to four sub-types of moonlighting proteins: external factor affecting the moonlighting protein directly (Type A), change in the first pathway (Type B), change in the second pathway (Type C), equal competition between both pathways (Type D) (see Table 1b). Combining these two classifiers leads to a total of 16 moonlighting types. This also justifies the difference between Type II and Type III. The effect of the moonlighting protein on the network depends on which pathway it serves its primary function in. The primary function may be defined as the function the protein serves in that particular moment, as the effects of the switch will be dependent on where the protein functions when the switching occurs. The term primary function is related to our investigation of cells. Objectively, we cannot know what is primary in a biological sense, we only know which function has been analyzed first or most. However, to ease description, we used the term here to express our perspective of investigation. In some cases, a primary and secondary function could be assigned due to the evolutionary development of a protein. In cases where this is not the case, the classification of a moonlighting protein could be context-dependent. A protein might be defined as Type II in one case and could be defined as Type III in another case.
Table 1
Classification of moonlighting proteins by their function in pathway A (primary function) and pathway X (secondary function)
Activating in Pathway A | Inhibiting Pathway A | |
Activating in Pathway X | Type I | Type III |
Inhibiting in Pathway X | Type II | Type IV |
A protein is seen as either being and activator or an inhibitor in one of these pathways. See Figs. 3–6 for network examples and simulations.
Table 2
Classification of moonlighting proteins by the factor that switches their function and causes a relocation of the protein
Factor | |
External Factor | Type A |
Change in Pathway A | Type B |
Change in Pathway X | Type C |
Equal Competition | Type D |
See Figs. 3–6 for network examples and simulations.
The classification into sub-classes based on the mechanism of switching the function of the moonlighting protein means that it will make a difference for the regulation of the network whether the moonlighting protein is as an inhibitor or an activator in its primary function.
It is important to point out that the terms inhibitor and activator in this classification can be interpreted very broadly, as a moonlighting protein can be an enzyme or a signaling component and can therefore also be activating and inhibiting in different ways. It can, for example, serve as an enzyme, activating a pathway by catalyzing a reaction inside it.
Likewise, the interpretation of external factor (Type A) is very broad. The external factor can be interpreted as every factor, which affects the moonlighting protein directly. In contrast to this, Type B and Type C are changes within the pathway, affecting the other proteins in the pathway.
To exemplify this classification and to be able to show the effects of a moonlighting protein on the pathways it takes part in, we used a small generic network (see Fig. 2). In this network, the moonlighting protein M fulfills functions in both pathways. The pathways were named A and X and each consisting of three components with two reactions happening between them.
Fig.2
Representation of the simple network model used to classify and simulate moonlighting proteins. The model has two pathways, named pathway A and pathway X. A moonlighting protein M has a function in both pathways and can switch between these. The final products of the pathways, C and Z, are used to assess pathway behavior.
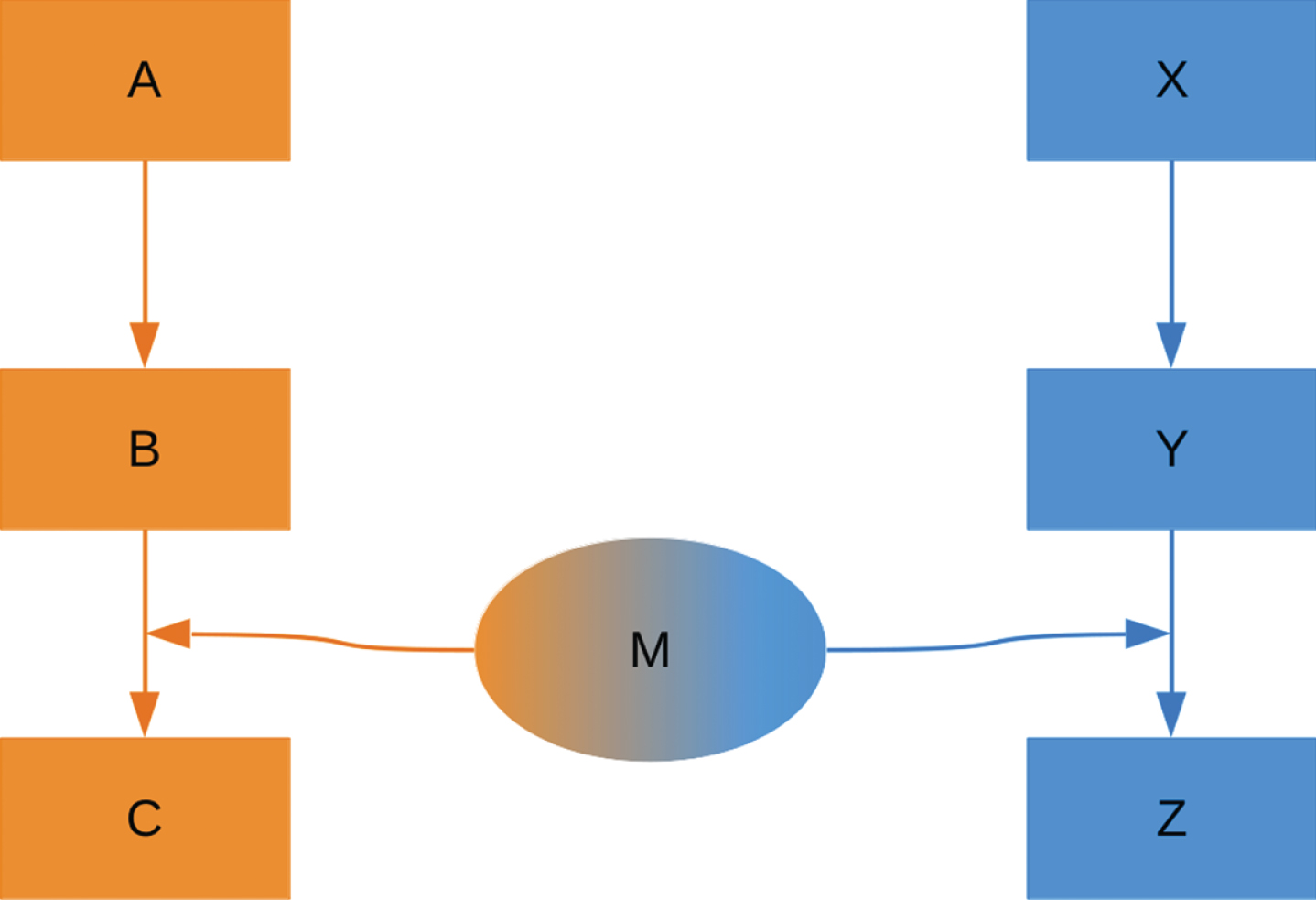
To analyze the behavior of the 16 moonlighting types from our classification, we simulated simple network motifs reflecting the functions and factors used for the classification. The simple networks used here are only examples of potentially more complex networks involving moonlighting proteins and are supposed to give a general overview of possible behaviors of these networks.
The schematic two-pathway system shown in Fig. 2 was used as a starting point for building the individual networks representing the 16 moonlighting types. For each type of moonlighting protein a possible mechanism realizing this type was simulated.
2.2Type I moonlighting proteins are activating both pathways
The first class of moonlighting proteins, type I moonlighting proteins (Fig. 3), are activating in both pathways. This means, that they are necessary components in both pathways. In the networks used here to exemplify this, M is forming a complex with B and Y, which is necessary to produce the final products of the respective pathways. The basic structure of the two pathways is the same in the four sub types A to D, but the factor that switches the function of the moonlighting protein from one pathway to the other changes (see networks inFig. 3).
Fig.3
Network motifs and simulations of moonlighting proteins type I.A to type I.D. The type I moonlighting proteins are activating both in pathway A and pathway X. The time courses of the exemplary networks were simulated until they reached a steady state. At the time point indicated by the purple arrow, the switching factor was activated. The initial concentration of the moonlighting protein M was varied for types B, C and D. The resulting time courses for C and Z are shown.
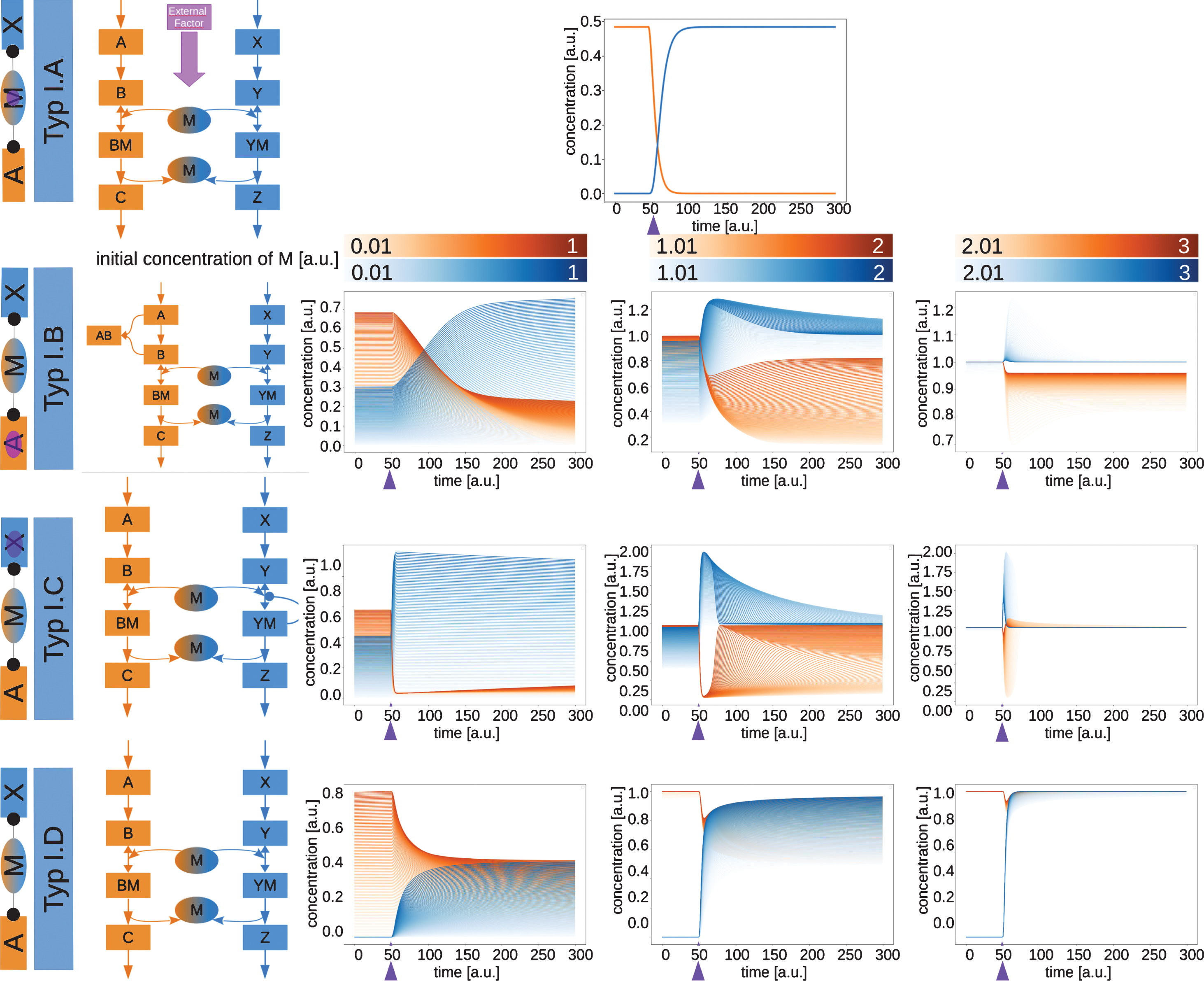
In type I.A (Fig. 3, upper row), the moonlighting protein is switched by an external factor. An example for this type is the Aconitase from Mycobacterium tuberculosis [7–9], which was already described in the introduction. The external factor here is a low iron concentration, which leads to a loss of the iron cluster in Aconitase. This switches its function from an enzymatic reaction to mRNA binding.
For the simulation shown in Fig. 3 the external factor completely switches the activity of the moonlighting protein from pathway A to pathway X. This is independent of the concentration of themoonlighting protein, as the external factor switches all of the available moonlighting protein.
For type I.B (Fig. 3, second row), the switching occurs when a change in pathway A releases the moonlighting protein from its first function and makes it possible for the protein to perform another function. A potential example for this was described in the introduction as well, the DLA2 from Chlamydomonas reinhardtii [10–14]. A possible mechanism for the switching of this protein is a substrate inhibition in its primary pathway, which sets DLA2 free to bind mRNA.
To simulate the behavior of type I.B, a reaction was added to pathway A. A binding between A and B serves as switching factor for the moonlighting protein. This binding makes less A and B available for the pathway, therefore also limiting the availability of B to bind M. This leads to more M being available for the binding of Y in pathway X, which leads to a higher production rate of Z and a lower production rate of C. This behavior depends on the relative concentration of M. If M is available in lower concentration than B and Y, the activation of the reaction A + B ->AB will lead to a switch between the two pathways. When more moonlighting protein is available, the effect of this switching will become less pronounced, as the moonlighting protein can then fulfill its function in both pathways at the same time.
The type I.C (Fig. 3, third row) moonlighting proteins are pulled over from their primary pathway A into their secondary function in pathway X by a reaction occurring in pathway X. This can for example be a self-enforcing complexation involving the moonlighting protein.
In the simulation of this type we used a self-enforcing binding reaction between Y and M. The self-enforcement was used as the switch for the moonlighting protein. Depending on the relative concentration of the moonlighting protein, it can either lead to a switch between the two pathways or lead to only a short activation of the second pathway. Like for type I.B, at higher relative concentrations of M, the moonlighting protein can fulfill both its functions simultaneously.
The simplest case of two pathways sharing a protein is an equal competition, in which both pathways have a reaction involving the moonlighting protein (Type I.D, Fig. 3, lowest row).
For simulation of the equal competition both pathways were given the same reaction rates and therefore the same strength in recruiting the moonlighting protein. The switch in this case is the activation of pathway X. If the amount of moonlighting protein is limiting, this leads to a reduction of the production rate of C. At higher relative concentrations of the moonlighting protein, the activation of pathway X has very little to no effect on the concentration of C and Z.
2.3Type II moonlighting proteins are activating in pathway A and inhibiting in pathway X
Type II moonlighting proteins are activating in pathway A and inhibiting in pathway X (Fig. 4). A possible realization of this type could be a metabolic enzyme acting as transcriptional repressor. The mechanisms of switching by which the type II moonlighting proteins are further classified into sub types are analogue to the ones for type I (see networks in Fig. 4).
Fig.4
Network motifs and simulations of moonlighting proteins type II.A to type II.D. The type II moonlighting proteins are activating in pathway A and inhibiting pathway X. The time courses of the exemplary networks were simulated until they reached a steady state. At the time point indicated by the purple arrow, the switching factor was activated. The initial concentration of the moonlighting protein M was varied for types B, C and D. The resulting time courses for C and Z are shown.
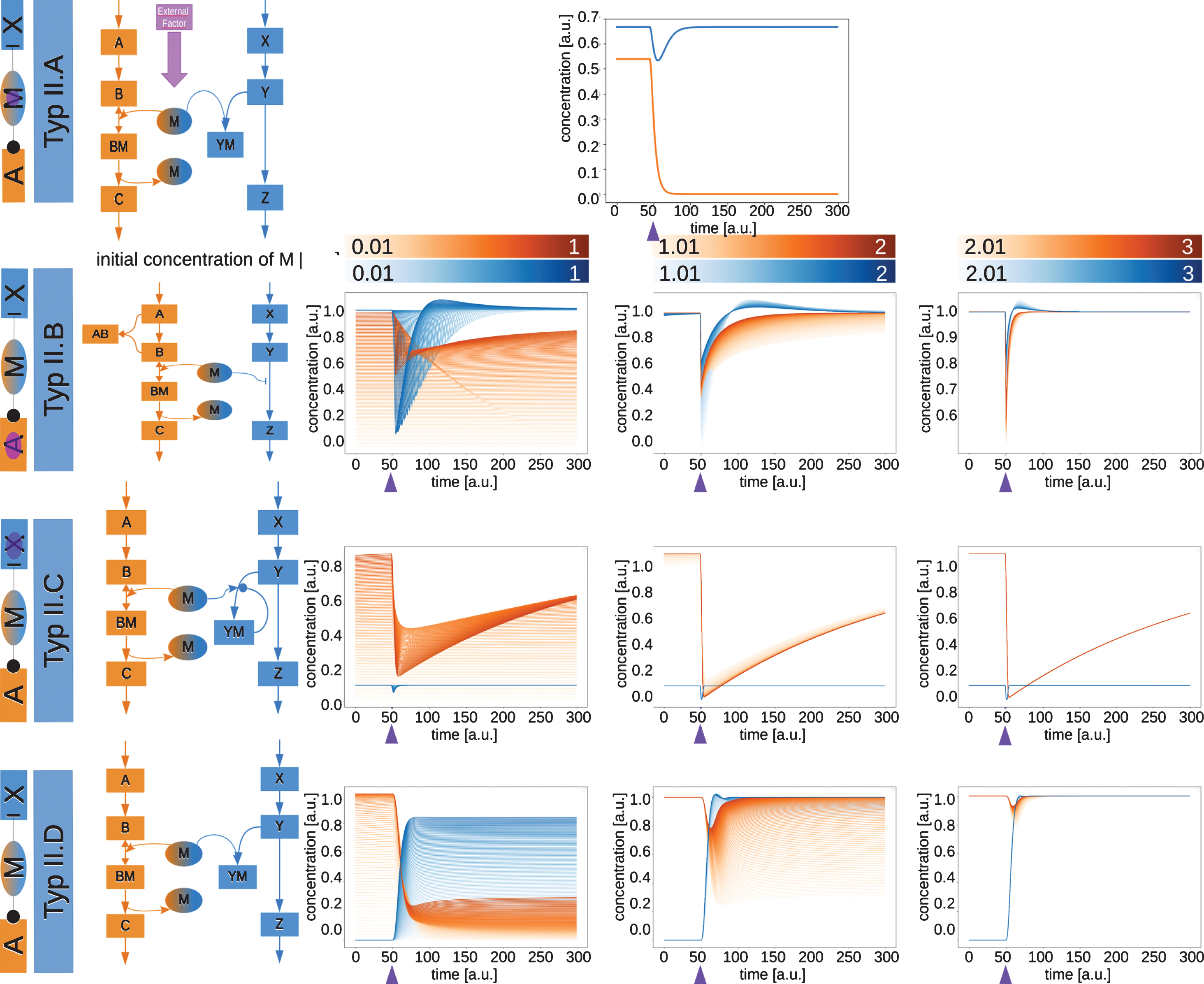
Type II.A (Fig. 4, upper row) describes a moonlighting protein, which is switched by an external factor. M acts as an enzyme in pathway A and can inhibit the production of Z by binding Y. Before the External Factor is switched on, the moonlighting protein acts as an enzyme in pathway A and C is produced. Pathway X is also active and Z is produced, since M is not involved in this pathway yet. Upon activation of the external factor, M binds Y and sequesters it from the reaction, thereby temporarily inhibiting the production of Z. Since M now cannot fulfill its function as an enzyme in pathway A anymore, the production of C also disappears.
For the type II.B (Fig. 4, second row) the switching reaction is again the complex formation between A and B. As this removes B from the reaction in pathway A, M becomes available to inhibit the production of Z. The concentrations of C and Z both decrease, as less C is produced due to the complex formation between A and B. If the relative concentration of M is higher, the effect of the switch is less clear.
An example for a moonlighting protein which can be classified as either type II.A or type II.B, is the large subunit (LSU) of Rubisco from Chlamydomonas reinhardtii. In plants and green algae, Rubisco fixes CO2 . It consists of eight large and eight small subunits. Under oxidizing conditions or when the process of assembly is interrupted, the LSU can bind any mRNA and repress its translation [20]. The classification into type II.A or II.B depends on the exact mechanism, which is not known yet, but might be either an external factor (the oxidizing conditions) or a change in pathway A, the translation and assembly of Rubisco subunits.
For type II.C (Fig. 4, third row) the inhibition occurs by M binding to Y and thereby sequestering it. This reaction is reversible and self-enforcing. The self-enforcement is the switching reaction and isactivated after the simulation reached a steady state. This leads to the removal of M from pathway A, where it is essential as an enzyme to convert B to C and therefore to a decrease in concentrations of C. The effect of the inhibition on Z strongly depends on the rates of the formation of the complex YM and its disassembly.
A similar behavior for the concentrations of C can be seen in type II.D (Fig. 5, lowest row). Here, the activation of the second pathway is the event, which switches the moonlighting protein. Once pathway X is active, the moonlighting protein is shared between the two pathways, which leads to a loss of M as an enzyme in pathway A. When more moonlighting protein is available, the effect is less pronounced in pathway A.
Fig.5
Network motifs and simulations of moonlighting proteins type III.A to type III.D. The type III moonlighting proteins are inhibiting in pathway A and activating pathway X. The time courses of the exemplary networks were simulated until they reached a steady state. At the time point indicated by the purple arrow, the switching factor was activated. The initial concentration of the moonlighting protein M was varied for types B, C and D. The resulting time courses for C and Z are shown.
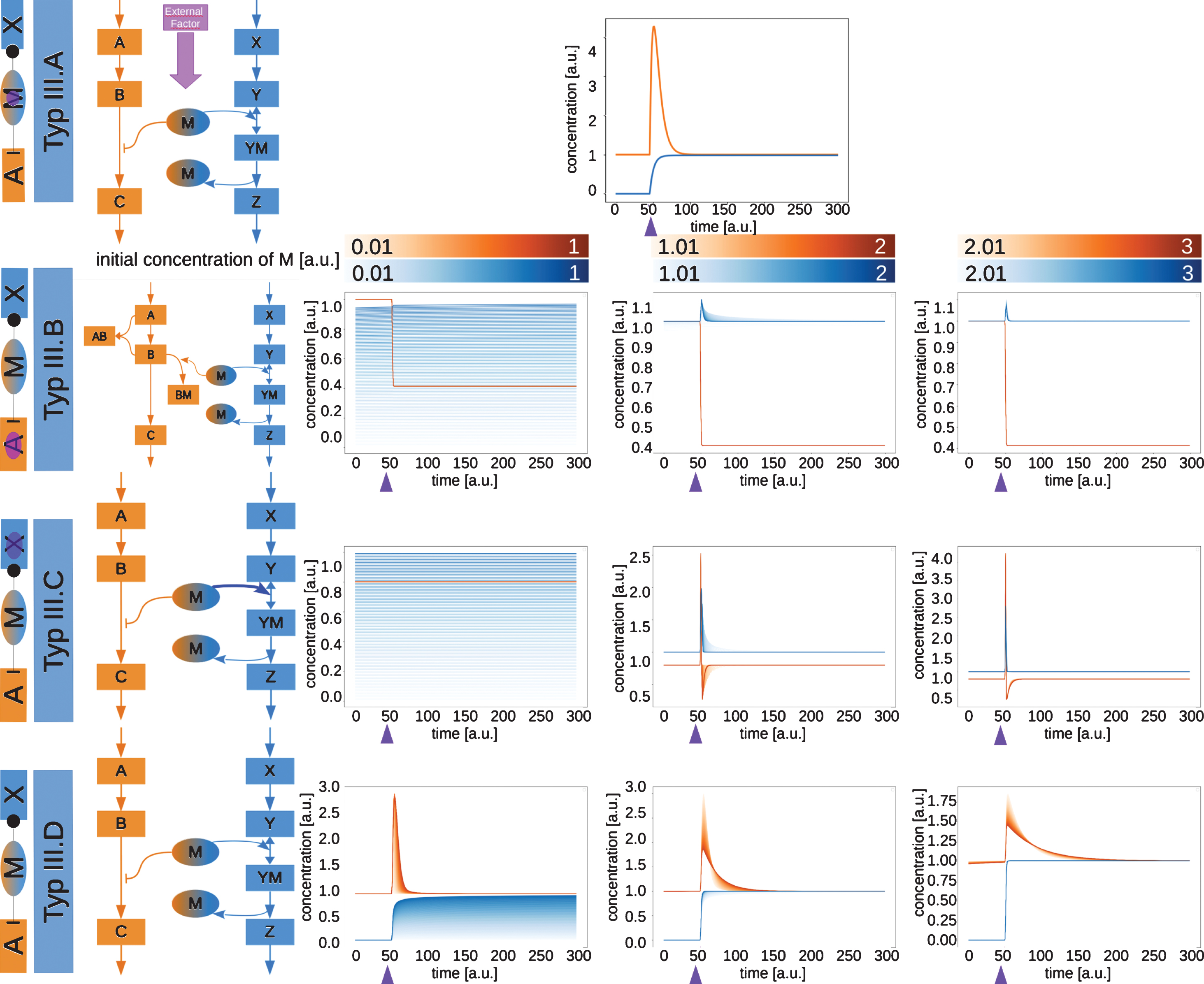
For many moonlighting proteins, the mechanisms that cause the change from one function to the other are not clear. An example of this is the hRoDH-E2 enzyme from human cells. This enzyme functions as a retinol dehydrogenase in the cytoplasm and as a transcriptional repressor in the nucleus [21]. How these two functions are linked is not clear. It can therefore only be classified as a type II moonlighting protein, as it is activating in one pathway in its function as a retinol dehydrogenase and inhibiting in another pathway in its function as transcriptionalrepressor.
The same problem in classification occurs for the Glutamate racemase (MurI) from Mycobacterium tuberculosis. This enzyme is involved in the cell wall biosynthesis and converts L-glutamate toD-glutamate. It has also been found to inhibit DNA gyrase function [22, 23]. Also here, the mechanism of switching between the two functions isnot clear.
2.4Type III moonlighting proteins are inhibiting in pathway A and activating in pathway X
Type III is the inverted case of type II. The moonlighting protein is inhibiting in pathway A and activating in pathway X (Fig. 5).
For type III.A (Fig. 5, upper row) the moonlighting protein is at first inhibiting the production of C in pathway A. When the external factor is switched on, the inhibition is released and M starts acting as an enzyme in pathway X. The release of the inhibition leads to a spike in the production of C, as precursors, which accumulated during the inhibition, are used. This behavior is observed, because the steps prior to the inhibition are notcontrolled.
As for the other type B moonlighting proteins, also for type III.B (Fig. 5, second row) the switchingreaction is the formation of a complex between A and B. The activation of this reaction also here leads to a decrease in the concentration of C, as A and B are pulled out of the pathway.
In type III.C (Fig. 5, third row) the mechanisms, which pulls M over into the pathway X, is a stronger binding affinity of Y to M than of B to M. This binding affinity becomes stronger, which is the switching reaction. Once this stronger binding affinity is activated, the inhibition of the production of C is partially released, which leads to a spike in C. Since M is now more likely to bind Y, the production of Z also increases.
In the case of equal competition in type III.D (Fig. 5, lowest row), the activation of the second pathway also leads to a spike in C due to the release from inhibition and a rapid activation of the productionof Z.
A problem occurs when trying to find examples for the type III moonlighting proteins. The difference to type II moonlighting proteins lies in the definition of the initial pathway. Essentially, every moonlighting protein classified as type II moonlighting protein could instead be type III. The initial pathway (pathway A) should be the main function of this protein. For many moonlighting proteins, two functions have been observed, but it is not always clear which function is the main function. The definition of the main function is possibly problematic and debatable and might be solved by looking at the evolutionary history of a moonlighting protein.
2.5Type IV moonlighting proteins are inhibiting in both pathways
The moonlighting proteins of type IV are inhibiting in both pathways (Fig. 6). The mechanism of inhibition is the same for all four types in this group. The moonlighting protein binds B and Y to form thecomplexes BM and YM, which sequesters B and Y from the pathway reactions.
Fig.6
Network motifs and simulations of moonlighting proteins type IV.A to type IV.D. The type IV moonlighting proteins are inhibiting in both pathways. The time courses of the exemplary networks were simulated until they reached a steady state. At the time point indicated by the purple arrow, the switching factor was activated. The initial concentration of the moonlighting protein M was varied for types B, C and D. The resulting time courses for C and Z are shown.
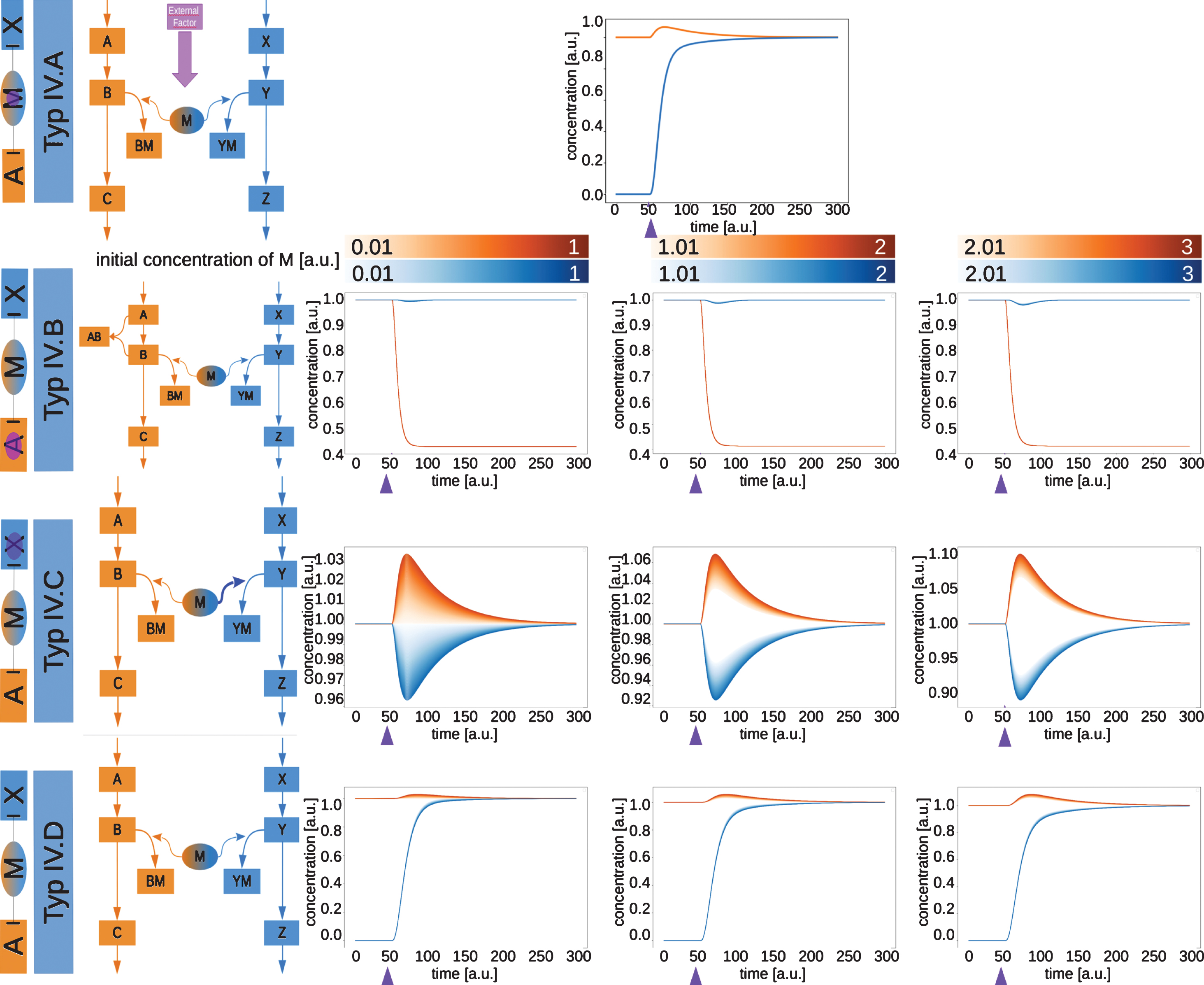
For type IV.A (Fig. 6, upper row) the external factor switches the function of M from binding B to binding Y. As the binding of M to B or Y only sequesters part of the component from the pathway reactions, the inhibited pathway is not blocked, but the production of C or Z is decreased. Once the external factor switches the moonlighting protein from pathway A to pathway X, the production of C increases and the concentration of Z increases, as the pathway becomes active.
In type IV.B (Fig. 6, second row) the switching reaction is the same as in the other type B moonlighting proteins. Also here, the concentration of C decreases, as does the concentration of Z, as the moonlighting protein inhibits itsproduction.
The type IV.C (Fig. 6, third row) moonlighting protein shows a different behavior. As the inhibition is released from pathway A, the production of C increases, while simultaneously the production of Z decreases. This effect becomes stronger the more moonlighting protein is available.
For type IV.D (Fig. 6, lowest row) a behavior similar to type IV.A could be observed. The first pathway shows a slight increase, once the second pathway is activated. This is due to the release from inhibition. The second pathway is activated, but partly inhibited and can only slowly overcome this inhibition.
No examples for this type of moonlighting protein could be found in the literature. This could indicate that this is not a motif used in cells or that it has not yet been found in a biological system. Nevertheless, this type of moonlighting protein might be an interesting motif for synthetic biology.
2.6General characterization of moonlighting types
The effect of the moonlighting protein on the 16 exemplary networks can be classified by four categories: Switch, Blinker, Splitter or No Effect (see Fig. 7). The classification of these categories was inspired by [24], but needed to be adapted for moonlighting proteins. A switch is here classified as a moonlighting protein, which deactivates or activates a pathway.
Fig.7
General classification of the characteristics of Moonlighting Proteins on the individual pathways. The simulated behavior of the 16 exemplary networks was classified into four different types: Switch, Blinker, Splitter or No Effect.
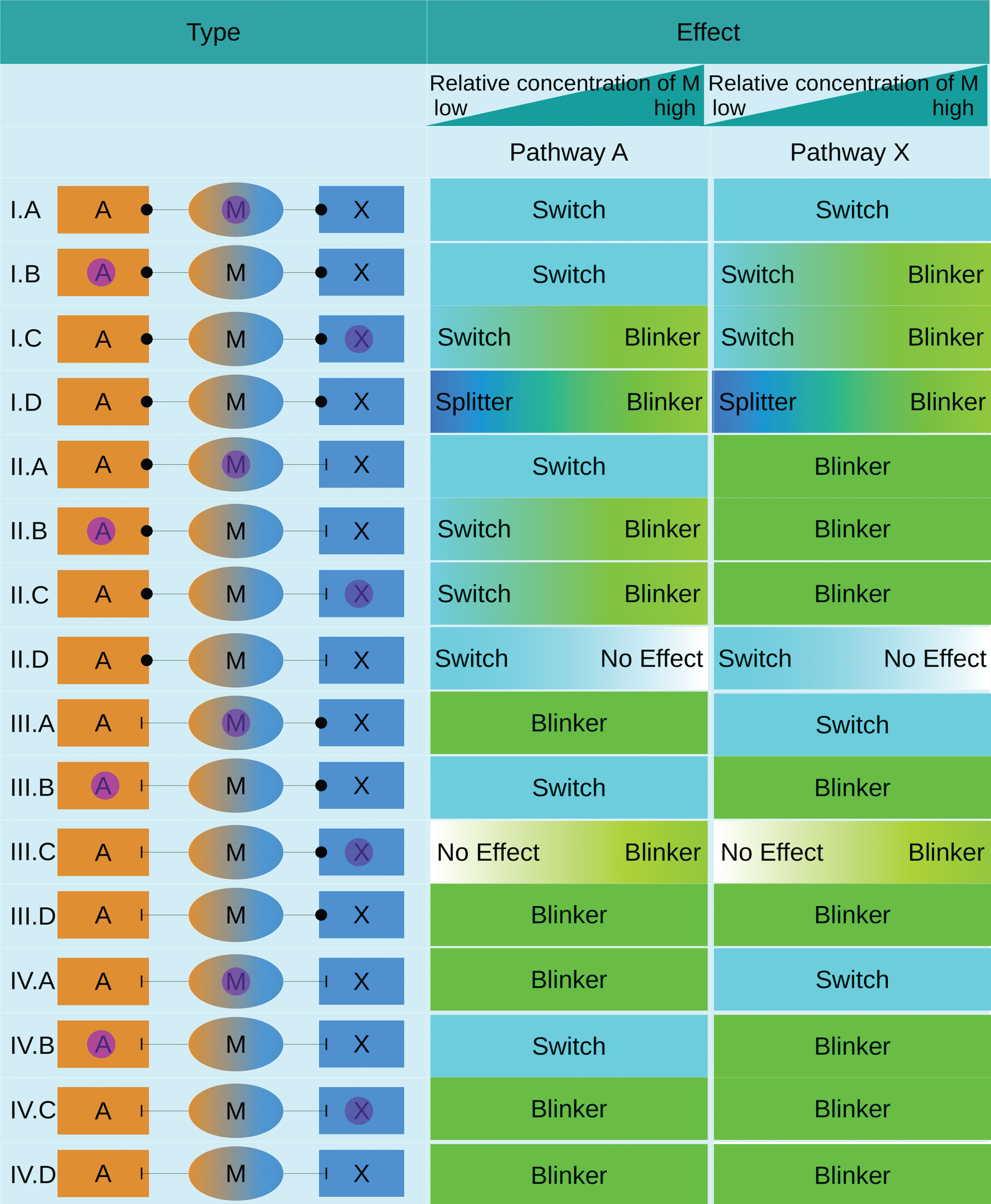
A Blinker leads to a short burst or drop in pathway activity, after which the levels of the measured component (here C and Z) return to the same steady state as before the switch of the moonlighting protein. This is due to the fact, that the total amount of M stays constant. The total amount of M is given by
A splitter splits its activity between two pathways. Some moonlighting effects were also classified as having no effect on the pathway.
Type I moonlighting proteins show very interesting behaviors. Type I.A is always a switch between two pathway activities, as the external factor completely switches the function between these two pathways. Type I.B and Type I.C act as a switch at low relative concentrations of M, while at higher concentrations Type I.B turns into a blinker for one of the pathways, while type I.C becomes a blinker in both pathways. Type I.D can serve as a splitter, meaning it reduces the activity of one pathway, or both pathways, therefore splitting its activity between the two pathways. This effect also disappears at higher relative concentrations. It is important to note that the behavior observed in the simulations for the type D moonlighting proteins is due to the simulation layout. To show the effect of the switching, one pathway is seen as inactive at the start of the simulation. The switching on of the second pathway is therefore not due to the moonlighting protein, but it shows the effect the moonlighting protein has. Therefore both pathways are seen as equal in the classification.
For the type II moonlighting proteins, the effect on pathway A can be seen as a switch for type A to C, while for pathway X the moonlighting protein leads to a blinker behavior. In type II.B and II.C higher concentrations of moonlighting protein lead to a blinker in pathway A, meaning that for these types at high relative concentrations of M both pathways show a blinker behavior. Type II.D serves as a switch at low concentration, but looses this effect at higher relative concentrations of M, as the protein is able to fulfill its function in both pathwayssimultaneously.
In the type III moonlighting proteins can be classified as either switch or blinker, with the exception of type III.C, which only has an effect on the pathways at higher concentration, then serving as a blinker.
For type IV moonlighting proteins the classification is similar to type III, with the exception of type IV.C, which is a blinker also at low concentration.
The blinker behavior mostly originates from the release from inhibition. As only one reaction in the pathway is controlled by the moonlighting protein, the prior reactions are still active and produce precursors. Once the inhibition is released, this leads to a sudden burst of activity in the pathway. A similar effect can lead to a sudden drop in activity, when an inhibition is established, but prior reactions are left uncontrolled. The inhibition can then be overcome, as precursors outcompete the inhibitor.
These behaviors are also influenced by the rates of the reactions. The simulations in Figs. 3 to 6 only show examples of this. A blinker can be a very minor change in behavior, but will still be classified as blinker, as changes in rates and concentrations can lead to much stronger behavior changes in the pathways.
3Discussion
Moonlighting is a concept used to describe proteins with diverse functions which perform twoindependent tasks using the same domain. A classification can be helpful to systematize this diverse concept and simplify communication about new discoveries. We sought to classify moonlighting by the function the proteins can have in two pathways and by the event that makes them switch from one function to the other. The resulting 16 types can be used to describe existing and yet to be discovered moonlighting proteins in the future. Through simulations of these types using a model of two very simple pathways, we could show possible behaviors of these 16 types and classify their behavior into fourgroups.
The classification of the moonlighting proteins was done using two aspects of the function of these proteins. First, which function can it have in each of the pathways (activating/inhibiting). Second, how does it switch from one pathway to the other. For the switching factor, four options were considered: an external factor, pathway A releasing the moonlighting protein, pathway X pulling the moonlighting protein over or equal competition. Using this classification, known moonlighting proteins could be assigned one type. Failure to assign a moonlighting protein to a type was due to missing information. Especially the switching factor is unknown in many cases.
Using a simple two-pathway model we simulated the 16 types of moonlighting proteins and found general behavior patterns. Only type I moonlighting proteins show a switch behavior, as they are able to switch the activity between two pathways. Most other moonlighting proteins show a blinker behavior, leading to only a short burst or drop in activity. It was also obvious, that the behavior of the moonlighting protein strongly depends on the relative concentration of the protein in comparison to the other proteins of the pathway. When a moonlighting protein is present in excess, it can serve both its functions at the same time. This does of course not hold for moonlighting proteins that are switched by an external factor, as this always leads to a complete switch infunction.
The pathways shown here are only examples of possible mechanisms of moonlighting proteins, many more are theoretically and biologically possible. The external factor in type A moonlighting proteins can for example be the pH in the cell, as well as a location change of the protein itself. The mechanisms for the other types, which lie in the pathways themselves, can also be of different nature then exemplified here. Likewise, the activation and inhibition function used to classify the moonlighting proteins into type I to IV should be seen as very loose definitions of activation and inhibition. When a protein is necessary for a pathway to function or enhances its performance, it can be seen as activating in this pathway.
For some proteins, the assignment of primary and secondary function could be problematic. It is often not clear which function of the moonlighting protein should be seen as its primary function. For some, this might be possible with an evolutionary perspective, while for other the primary and secondary function could be context-dependent. In this case, also the classification of the moonlighting protein into the types proposed here could be context-dependent. This could be in the nature of moonlighting proteins or due to our limited understanding of their function.
The behaviors observed in the simulations are possible behaviors of these moonlighting proteins. These are entirely dependent on relative concentrations and parameters. The parameters used for the simulations are, just as the concentrations, relative quantities. The behavior observed for the moonlighting types is dependent on the relative values of the parameters and quantities. In this work, we show how the behavior of the system changes due to changes in the concentration of the moonlighting protein. For the reactions containing the moonlighting protein, rates are formed by a simple multiplication of the concentration of the moonlighting protein times a rate constant. We therefore did not perform parameter scans, which might limit the biological insights gained from our models. Since the importance of moonlighting proteins is only beginning to emerge, experimentally measured rates for the reactions are often not available. The rates and concentrations in our models are therefore not actual biological rates, but were chosen to exemplify possible behaviors of thesesystems.
Some moonlighting proteins might serve more than two functions. With the scheme presented here, a classification of these proteins would be possible by classifying them as two or three types, one for each function and switch. As an example we could imagine three pathways: A, X and Q. One type could be defined for the switch between A and X and one for the one between A and Q. A third type would be necessary if a switch between X and Q is also possible.
Further investigation into moonlighting proteins, their function, the events that lead to the change in their function and their role in metabolism, signaling, development and disease will be essential in the future.
We expect that this classification will help researchers to better communicate and exchange experimental results on moonlighting proteins and give a better understanding of the concept.
4Materials and Methods
The simulations were performed using the Stimator 0.9.110 package in python3. Analysis of the simulation results was done using the numpy package and visualization was carried out using the matplotlib package.
The network models were assembled to reassemble small possible network motifs. The complete reactions for all 16 types can be found in the supplement.
To simulate the effect of the moonlighting protein, the pathways had a constant influx and outflux of components, meaning that there was a constant influx rate of the first component of each pathway and a constant outflux of the last. The total amount of the moonlighting protein was kept constant to simulate competition between two pathways.
Declarations
Ethics Approval and consent to participate
Not applicable.
Consent for publication
Not applicable.
Availability of data and material
Not applicable.
Competing interests
The authors declare that they have no competing interests.
Funding
This work was supported by the Deutsche Forschungsgemeinschaft (DFG, TRR 175).
Authors’ contributions
Acknowledgments
MK would like to thank Marcus Krantz for advise on the manuscript.
Supplementary material
[1] The supplementary material is available in the electronic version of this article: https://dx.doi.org/10.3233/ISB-190473.
References
[1] | Huberts D.H.E.W. , van der Klei I.J. Moonlighting proteins: An intriguing mode of multitasking, Biochim Biophys Acta - Mol Cell Res [Internet]. 1803: (4) ((2010) ), 520–525. Available from: http://www.sciencedirect.com/science/article/pii/S0167488910000352. |
[2] | Shishko R. , Rostker B. The economics of multiple job holding, Am Econ Rev [Internet] 66: (3) ((1976) ), 298–308. Available from: http://www.jstor.org/stable/1828164. |
[3] | Copley S.D. Enzymes with extra talents: Moonlighting functions and catalytic promiscuity, Curr Opin Chem Biol [Internet] 7: (2) ((2003) ), 265–72. Available from: http://www.sciencedirect.com/science/article/pii/S13675931367593. |
[4] | Espinosa-Cantú A. , Ascencio D. , Barona-Gómez F. , DeLuna A. Gene duplication and the evolution of moonlighting proteins, Front Genet [Internet] 6: ((2015) ), 227. Available from: https://www.ncbi.nlm.nih.gov/pubmed/26217376. |
[5] | Jeffery C.J. What is protein moonlighting and why is it important? [Internet], Moonlighting Proteins ((2017) ). (Wiley Online Books). Available from: https://doi.org/10.1002/9781118951149.ch1. |
[6] | Jeffery C.J. Why study moonlighting proteins? Front Genet [Internet] 19: (6) ((2015) ), 211. Available from: https://www.ncbi.nlm.nih.gov/pubmed/26150826. |
[7] | Banerjee S. , Nandyala A.K. , Raviprasad P. , Ahmed N. , Hasnain S.E. Iron-dependent RNA-binding activity of Mycobacterium tuberculosis aconitase, J Bacteriol [Internet] 189: (11) ((2007) ) 4046–4052. Available from: https://www.ncbi.nlm.nih.gov/pubmed/17384188. |
[8] | Beinert H. , Kennedy M.C. Aconitase, a two-faced protein Enzyme and iron regulatory factor, FASEB J [Internet] 7: (15) ((1993) ), 1442–1449. Available from: https://doi.org/10.1096/fasebj.7.15.8262329. |
[9] | Beinert H. , Kennedy M.C. , Stout C.D. Aconitase as iron– sulfur protein, enzyme, and iron-regulatory protein, Chem Rev [Internet] 96: (7) ((1996) ), 2335–2374. Available 708 from: https://doi.org/10.1021/cr950040z. |
[10] | Bohne A.-V. , Schwarz C. , Schottkowski M. , Lidschreiber M. , Piotrowski M. , Zerges W. , Reciprocal regulation of protein synthesis and carbon metabolism for thylakoid membrane biogenesis, PLOS Biol [Internet] 11: (2) ((2013) ), e1001482. Available from: https://doi.org/10.1371/journal.pbio.1001482. |
[11] | Bohne A.-V. , Nickelsen J. Metabolic control of chloroplast gene expression: An emerging theme, Mol Plant [Internet] 10: (1) ((2017) ), 1–3. Available from: http://www.sciencedirect.com/science/article/pii/S1674205216301629. |
[12] | Guan Y. , Rawsthorne S. , Scofield G. , Shaw P. , Doonan J. Cloning and characterization of a dihydrolipoamide acetyltransferase (E2) subunit of the pyruvate dehydrogenase complex from arabidopsis thaliana, J Biol Chem [Internet] 270: (10) ((1995) ), 5412–5417. Available from: http://www.jbc.org/content/270/10/5412.abstract. |
[13] | Ke J. , Behal R.H. , Back S.L. , Nikolau B.J. , Wurtele E.S. , Oliver D.J. The role of pyruvate dehydrogenase and acetyl-coenzyme a synthetase in fatty acid synthesis in developing arabidopsis seeds, Plant Physiol [Internet] 123: (2) ((2000) ), 497LP–508. Available from: http://www.plantphysiol.org/content/123/2/497.abstract. |
[14] | Mooney B.P. , Miernyk J.A. , Randall D.D. The complex fate of α-Ketoacids, Annu Rev Plant Biol [Internet] 53: (1) ((2002) ), 357–375. Available from: https://doi.org/10.1146/annurev.arplant.53.100301.135251. |
[15] | Wilson J.E. Isozymes of mammalian hexokinase: Structure, subcellular localization and metabolic function, J Exp Biol [Internet] 206: (12) ((2003) ), 2049LP–2057 Available from: http://jeb.biologists.org/content/206/12/2049.abstract. |
[16] | Gancedo J.M. Yeast carbon catabolite repression, Microbiol Mol Biol Rev [Internet] 62: (2) ((1998) ), 334–361. Available from: https://www.ncbi.nlm.nih.gov/pubmed/9618445. |
[17] | Boukouris A.E. , Zervopoulos S.D. , Michelakis E.D. Metabolic enzymes moonlighting in the nucleus: Metabolic regulation of gene transcription, Trends Biochem Sci [Internet] 41: (8) ((2016) ) 712–730. Available from: https://doi.org/10.1016/j.tibs.2016.05.013. |
[18] | Faik P. , Walker J.I.H. , Redmill A.A.M. , Morgan M.J. Mouse glucose-6-phosphate isomerase and neuroleukin have identical 3′ sequences, Nature [Internet] 332: ((1988) ), 455. Available from: https://doi.org/10.1038/332455a0. |
[19] | Chaput M. , Claes V. , Portetelle D. , Cludts I. , Cravador A. , Burny A. , The neurotrophic factor neuroleukin is 90% homologous with phosphohexose isomerase, Nature [Internet] 332: ((1988) ), 454. Available from: https://doi.org/10.1038/332454a0. |
[20] | Cohen I. , Sapir Y. , Shapira M. A conserved mechanism controls translation of rubisco large subunit in different photosynthetic organisms, Plant Physiol [Internet] 141: (3) ((2006) ) 1089LP–1097 Available from: http://www.plantphysiol.org/content/141/3/1089.abstract. |
[21] | Markova N.G. , Pinkas-Sarafova A. , Simon M. A metabolic enzyme of the short-chain dehydrogenase/reductase superfamily may moonlight in the nucleus as a repressor of promoter activity, J Invest Dermatol [Internet] 126: (9) ((2006) ), 2019–2031 Available from: http://www.sciencedirect.com/science/article/pii/S0022202X15330542. |
[22] | Sengupta S. , Ghosh S. , Nagaraja V. Moonlighting function of glutamate racemase from Mycobacterium tuberculosis: Racemization and DNA gyrase inhibition are two independent activities of the enzyme, Microbiology [Internet] 154: (9) ((2008) ), 2796–2803. – Available from: http://mic.microbiologyresearch.org/content/journal/micro/10.1099/mic.0.2008/020933-0. |
[23] | Ashiuchi M. , Kuwana E. , Yamamoto T. , Komatsu K. , Soda K. , Misono H. Glutamate racemase is an endogenous DNA gyrase inhibitor, J Biol Chem [Internet] 277: (42) ((2002) ) 39070–39073. Available from: http://www.jbc.org/content/277/42/39070.abstract. |
[24] | Tyson J.J. , Chen K.C. , Novak B. Sniffers, buzzers, toggles and blinkers: Dynamics of regulatory and signaling pathways in the cell, Curr Opin Cell Biol [Internet] 15: (2) ((2003) ), 221–231. Available from: http://www.sciencedirect.com/science/article/pii/S0955067403000176. |