Approach to diagnosis of metabolic diseases
Abstract
Inborn errors of metabolism are generally categorized as rare diseases. Their presentations are often so subtle and insidious as to cause daunting diagnostic challenges for even the most astute clinicians. Thus, irreversible morbidity and preventable mortality have been unavoidable until recent decades because of delayed diagnoses. This unfortunate circumstance has led to newborn screening programs worldwide for 40 or more hereditary metabolic disorders beginning with the dramatic improvements for patients with phenylketonuria in the 1960’s. Increasingly sophisticated testing procedures such as tandem mass spectrometry and other multiplex technologies applied to dried blood spot specimens are now having greater impact without raising costs significantly. The advent of next generation sequencing methods is likely to stimulate further progress and lead to whole genome or exome sequencing as prenatal and neonatal screening expands further. With early diagnosis through screening and expedited therapies better outcomes are routinely possible, and even preventive therapies amounting to “cures” can be anticipated through research.
1Conceptual overview
The term “inborn errors of metabolism” was first used by Sir Archibald Garrod in his Croonian Lectures in 1908 [1] and in his monograph Inborn Errors of Metabolism in 1909 [2]. He defined these inborn errors as genetically determined diseases caused by blocks in the metabolic pathways due to deficient activity of an enzyme [3]. From Sir Garrod’s observations of patients with alkaptonuria, albinism, cystinuria, and pentosuria, he developed the concept that certain diseases of lifelong duration arise because an enzyme governing a single metabolic step is reduced in activity or missing altogether [4]. Most of these disorders may be classified as rare diseases because newborn screening data show clearly that their incidence ranges from 1:4,000 for congenital hypothyroidism to >1:250,000 (many disorders such as Maple Syrup Urine Disease (branched chain ketoaciduria).
The definition of an inborn error of metabolism is arbitrary. Scriver et al. [5] include several hundred diseases with definite biochemical genetic bases. McKusick’s catalog [6] contains several thousand diseases and disease states whose genetic abnormalities are described or assumed. Scriver et al. [5] solved the problem of exclusivity by adding “molecular” before encompassing every disease of the “textbook of the future.” The 2nd Edition of Metabolic Diseases: Foundations of Clinical Management, Genetics, and Pathology [7] is limited to those diseases with either: 1) recognized biochemical abnormalities supplemented with pathologic and genetic information, and in which a mutation leads to an enzyme deficiency that causes a metabolic disease such as phenylketonuria (PKU); or 2) disorders associated with known genetic mutations that have altered cellular physiology such as the chloride channel defect in cystic fibrosis or caused severe structural, cellular, or subcellular abnormalities. Emphasis in the book has been given to the more common and/or well defined hereditary biochemical/metabolic disorders. Prototype conditions such as Lesch Nyhan Disease, an excellent example of a nucleotide (purine) metabolism disorder, are also emphasized.
Investigation of the molecular functions of genes has determined that they control the cellular metabolic function in the pathogenesis of disease far beyond that commonly understood as a metabolic disease. The genetics of variant human phenotypes is discussed by Scriver et al. [5] and Champion [8]. The relationship between gene and enzyme was described as “one gene, one enzyme” by Beadle and Tatum [9–11]. Tatum expanded this concept as follows: 1) all biochemical processes in all organisms are under genetic control; 2) these biochemical processes are resolvable into series of individual stepwise reactions; 3) each biochemical reaction is under the ultimate control of a different single gene; and 4) mutation of a single gene results only in an alteration in the ability of the cell to carry out a single primary chemical reaction.
Pauling et al. [12] discovered direct evidence that human mutations actually produce an alteration in the primary structure of proteins. Their seminal research revealed a valine substitution for glutamic acid in sickle cell hemoglobin. Ingram [13] showed that inborn errors of metabolism were caused by mutant genes that produced abnormal proteins whose functional activities were altered.
It is estimated that some 30,000 genes constitute the human genome. A variety of genome maps are available, including some that are disease specific such as the “cancer cell map” [14]. The genome map is being constantly improved, with updated gene sequences and locations of intronic elements, revised lists of benign SNPs, additional reference genomes, databases of variant frequencies that are ethnicity-specific, and associations of specific genes with specific human diseases. In addition, combinations of genes can result in a metabolic error. Thus, although ∼7000 hereditary metabolic disorders are currently known, at least the same number of significant genetic errors as the number of genes seems theoretically possible, and an undetermined but large percentage of these may cause biochemical disorders with clinical impact. An estimation of genetic disease frequencies is shown in Table 1.
2Diagnostic strategies and tactics
Clinical diagnosis of metabolic disease is made by specific tests, biochemical analyses, and histologic and genetic studies that are discussed by Leonard et al. [15] and Cleary et al. [16]. New methods such as next generation sequencing of genes [17, 18] and even whole genome sequencing [19, 20] are facilitating diagnoses and other goals such as genetic counseling. Clinicians seek diagnosis, effective treatment, an understanding of prognosis, and the capability to explain and counsel patients and their families. Parents and patients appreciate gaps in knowledge but may be left seriously disappointed or angry without a cure. Fortunately, our increased genetic knowledge and better understanding of molecular pathophysiology of the past two decades has led to dramatic improvements in therapies using novel methods such as small molecule modulators of defective proteins [e.g., CFTR modulators for cystic fibrosis [21], gene therapy [22], and stem cell infusions [23]]. Thus, investments in expedited diagnostic strategies such as newborn screening can pay great dividends when well targeted, highly effective therapies are available.
Identification of inborn errors of metabolism provides the basis for diagnosis, prognosis, genetic counseling, and, in an increasing number of patients, targeted treatments aimed directly at the fundamental defect. Many infants and children present as medical emergencies and life-preserving treatment is begun before a definite diagnosis is available. Diagnosis should be made rapidly and the implications and complexities of treatment explained in detail. Metabolic disease is usually not suspected in patients until more common diseases are considered. Those children with a history of consanguinity or those in families with a history of unexplained death, multiple spontaneous abortions, or previously diagnosed metabolic diseases have an increased likelihood of a metabolic disease. Frequently, children (and some adults) with metabolic diseases are first seen as critically ill patients with non-specific findings. Dehydration, acidosis, vomiting, ammonemia, hypoglycemia, or seizures must be managed aggressively. Inborn metabolic errors may be suspected if response to emergency treatment is not as expected.
Metabolic diseases can be divided into 3 main categories as listed in (Table 2). The majority of these disorders are inherited as autosomal recessive traits. Some such as Duchenne muscular dystrophy are X-linked. A few are inherited as dominant traits, and mitochondrial disorders form a genetically separate category. Mitochondrial enzymes are coded by both the maternal nuclear genome and by the mitochondrial DNA.
Clinical findings associated with metabolic diseases are listed in Table 3. It has been well recognized that symptoms may be quite variable, even in a group of patients with the same mutation. Thus, it has become clear that genetic modifiers may alter the expression of signs/symptoms and that gene-environment and nutrition-metabolism relationships can also influence the disease liability of pathologic genetic mutations.
The newborn with a severe metabolic abnormality may present with symptoms of apparent sepsis or asphyxia. These symptoms usually consist of irritability, failure to feed or suck, flaccidity, or coma. Previous miscarriages or sudden unexpected death in a sibling should trigger a screening investigation for metabolic disease. Sophisticated newborn screening using 4-5 blood spots on filter paper [“Guthrie cards” named for Robert Guthrie, the originator of population-based newborn screening with dried blood spots [24]], combined with tandem mass spectrometry as discussed by Ziadeh et al. [25] and Millington et al. [26] or molecular analyses [21] can identify a number of life-threatening diseases with a single, multiplex assay. The hereditary metabolic diseases that can now be screened for from filter paper blood spots in the newborn are listed in Table 4. Recently, severe combined immunodeficiency (SCID) has been added in many regions as well as “point of care” screening methods such as oxygen saturation measurements for critical congenital heart diseases.
Metabolic abnormalities may be suspected in infants with hydrops fetalis Table 3, or with placental abnormalities. Routine placental examination may disclose fetal metabolic storage disease by the presence of vacuolations of syncytiotrophoblasts, intermediate trophoblasts, and stromal Hofbauer cells. Suspected metabolic errors in the newborn are emergencies and require prompt identification and treatment. After obtaining the appropriate specimens for testing, emergency treatment must be instituted. If the patient dies, regardless of age, preparation should be made for a metabolic disease autopsy as discussed by Ernst et al. [27].
Pregnancy may occur in patients with metabolic diseases. Some of these conditions can be teratogenic. Many such pregnancies can be successfully managed with treatment. Treatment of these disorders is discussed in each chapter.
Examples of inborn errors of metabolism potentially occurring in high frequency among specific ethnic groups are listed in Table 5. However, it should be noted that some of these disorders are quite rare now in the era of prenatal diagnosis. For instance, the incidence of Tay Sachs disease in targeted Jewish populations is extremely low worldwide [28, 29].
The most common presentations of inborn errors are without gross physical anomalies and include disorders of amino acid, fatty acid, organic acid, and carbohydrate metabolism Table 6. Others present with dysmorphic features, neonatal deaths, self-mutilation, abnormal body or urine odor, hypotonia, deafness, or recurrent acidosis with or without ketosis. Failure to recognize and, when possible, treat these infants can result in irreversible neurologic damage.
Diagnostic strategy consists of meticulous attention to history and physical examination followed by appropriate screening tests, including biochemistry, radiology, or other modalities, and specific enzyme, protein, or gene analysis. Special educational services for children with inborn errors of metabolism may be available and therefore accuracy of diagnosis is essential as discussed by Powell et al. [30]. Clinical investigation is augmented by a large number of common blood and urine tests (blood, sugar, urine pH, anion gap, cytopenia, liver function tests, sweat test, electroencephalogram, and radiography of bones). Radiologic examination may include X-rays, CT scans, and MR imaging. MRI scans should be substituted for CT scans whenever possible to avoid radiation exposure. Ophthalmologic and neurophysiologic investigations are often important. Laboratory findings in inborn errors of metabolism are listed in Table 7. Abnormal metabolites are shown in Table 8.
Abnormalities in serum electrolytes can be caused by vomiting or diarrhea. Inclusion of bicarbonate and pH assessment may indicate a large anion gap due to the presence of organic acids. Electrolytes, however, may be normal with organic acidemias.
Neutropenia or pancytopenia suggests the presence of one of the organic acidemias. A urinalysis may indicate the presence of reducing substances and the presence or absence of ketones. Ketonuria is not found in normal newborns. Urine pH above 5.0 in an acidotic infant may suggest renal tubular acidosis. Absence of ketones in association with hypoglycemia may indicate an error in fatty acid metabolism. It is helpful to save a small amount of frozen urine and plasma for later evaluation of keto acids, carnitine, and organic acids.
Elevated serum ammonia levels are found in patients with liver disease and in those with errors of the urea cycle and several of the organic acidemias. Transient hyperammonemia occurs in some newborns, and this is reversible when treatment is prompt. Urea cycle errors are frequently associated with very low blood urea levels. Some metabolic disorders cause liver cirrhosis in infants and children and some liver disorders progress to stupor and coma.
Liver function tests commonly indicate primary liver diseases. However, abnormal liver function test findings result from many of the metabolic diseases, including disorders of organic and amino acid metabolism, and many of the storage diseases.
Elevated serum lactate is usually due to hypoxemia or poor perfusion and occurs with sepsis. It may be due, however, to improper technique in drawing blood without good blood flow. Lactic acid is produced in the anaerobic metabolism of glucose through pyruvate, which is then converted in the liver to glucose. Deficiency of pyruvate dehydrogenase complex is the most common error of metabolism that causes lactic acidosis.
Myopathy can be caused by a few of the glycogen storage diseases or mitochondrial disorders. Muscle biopsy or fibroblast cultures may be diagnostic. Myoglobinuria may be present, and serum free carnitine may be decreased.
Peroxisomal action is responsible for β-oxidation of fatty acids, and deficiency results in developmental retardation. Peroxisomal disorders with such manifestations include Zellweger syndrome, adrenoleukodystrophy, and oxalosis [31]. Very-long-chain fatty acids are elevated in the serum with most forms of peroxisomal disorders.
Many chemical screening programs such as determination of organic and amino acids, oligosaccharides, and glycosaminoglycans in blood and urine are available for diagnostic workup of suspected progressive degenerative metabolic diseases. Distinguishing biochemical findings of inborn errors of metabolism are shown in Table 9.
Some inherited metabolic diseases significantly increase the risk of intercurrent illness. For example, recurrent treatment-resistant otitis media is a common problem in children with mucopolysaccharide storage diseases because distortion of the Eustachian tube and production of particularly tenacious mucus combine to create a favorable environment for bacterial colonization of the middle ear. The neutropenia that is a prominent feature of glycogen storage disease type Ib, and some of the organic acidopathies, predisposes to pyogenic infections. Classic galactosemia predisposes infants to neonatal Escherichia coli sepsis [32].
Major congenital malformations, such as meningomyelocele, complex congenital heart disease, and major congenital limb deformities, are not generally considered signs of an underlying inherited metabolic disease; however, some inherited metabolic conditions occur with dysmorphism so characteristic that a strong presumptive diagnosis can be made on physical examination alone [32]. A large number of metabolic diseases present with gross abnormalities of appearance Table 10A and 10B. Many of these have lysosomal defects, and most will have hepatosplenomegaly. These include such diseases as mucopolysaccharidoses, sphingolipidoses, and other lysosomal storage diseases. These diseases are not acutely life-threatening. Children with these conditions commonly have developmental delay. Many appear normal at birth and progressively deteriorate Table 3.
An important step between the clinical findings and the biochemical findings is the anatomic pathology, including histology, histochemistry, immunochemistry, and electron microscopy (EM). For example, screening peripheral blood for cytoplasmic vacuoles in the lymphocytes as shown in Fig. 1 may be the first indication of a storage disorder.
Some metabolic disorders present as acute encephalopathy. The earliest signs of encephalopathy may be no more obvious than excessive drowsiness, unusual behavior, or some unsteadiness of gait. Acute or intermittent ataxia is a common sign of acute encephalopathy in older children with inborn errors of metabolism. A history of recurrent attacks of unsteadiness of gait or ataxia, especially when associated with vomiting or deterioration of consciousness, should stimulate investigation of a possible inherited metabolic disease Table 11 [25].
In addition to the biochemical tests of blood and urine, solid tissues and cell cultures may be needed for biochemical assays. A biopsy specimen should be divided for biochemical analysis and morphologic investigation. This is particularly important in liver, muscle, and intestinal biopsies and in skin biopsies made for the fibroblast cultures.
Hypoglycemia occurs with starvation in young infants but also may indicate an error in gluconeogenesis, errors in fat metabolism and defects in hormone metabolism. A rapid response to glucose, especially in the presence of hepatomegaly, may suggest glycogen storage disease or fructose-1,6-diphosphatase deficiency. An approach to diagnosis when a patient presents with hypoglycemia is shown in Fig. 2. This algorithm will guide clinicians toward a proper sequence of diagnostictests.
Even after death, some of the techniques used in the living can be helpful in detecting inborn errors of metabolism. For example, even small amounts of urine may remain in the bladder and should be aspirated and saved. Tissues and body fluids should be obtained and maintained in a state suitable for the tests desired. New diseases and diagnostic tests are identified at a surprisingly rapid rate. Very few laboratories perform all the tests.
3Further development of prenatal and neonatal screening
As techniques such as next generation sequencing [17, 18] and whole genome sequencing are developed further for routine use, there will be many more commercial laboratories offering comprehensive testing as has already proved helpful for newborn intensive care units [19, 20]. However, reporting and ethical issues need to be resolved before whole genome sequencing can be used widely [33].
References
[1] | Garrod A.E. , Inborn errors of metabolism (Croonian Lectures). Lancet 2: , ((1908) ) 1. |
[2] | Garrod A.E. , Inborn Errors of Metabolism, London, (1909) , Oxford University Press. |
[3] | Garrod A.E. , Inborn Errors of Metabolism, London, (1923) , Oxford University Press. |
[4] | Bearn A.G. and Miller E.D. , Archibald Garrod and the development of the concept of inborn errors of metabolism. Bull Hist Med 53: ((1979) ), 315–325. |
[5] | Scriver C.R. , Beaudet A.L. , Sly W.S. and Valle D. , editors: The Metabolic and Molecular Bases of Inherited Disease, 7th ed, New York, (1995) , McGraw-Hill. |
[6] | McKusick V.A. , Mendelian Inheritance in Man, 11th ed, Baltimore, (1994) , The Johns Hopkins University Press. |
[7] | Gilbert-Barness E. , Barness L. and Farrell P. , (Eds.), Metabolic Diseases: Foundations of Clinical Management, Genetics, and Pathology. IOS Press, Amsterdam, In press. |
[8] | Champion M.O. , An approach to the diagnosis of inherited metabolic disease. Arch Dis Child Educ Pract Ed 95: ((2010) ), 40–46. |
[9] | Beadle G.W. , Biochemical genetics. Chem Rev 37: ((1945) ), 15. |
[10] | Beadle G.W. and Tatum E.L. , Genetic control of biochemical reactions in Neurospora. Proc Natl Acad Sci USA 27: ((1941) ), 499–506. |
[11] | Tatum E.L. , A case history of biological research. Science 129: ((1959) ), 1711–1715. |
[12] | Pauling L. , Itano H.A. , Singer S.J. and Wells I.C. , Sickle cell anemia: A molecular disease. Science 110: ((1949) ), 543–548. |
[13] | Ingram V.M. , A specific chemical difference between the globins of normal human and sickle cell anaemia haemoglobin. Nature 178: ((1956) ), 792–794. |
[14] | Krogan N.J. , Lippman S. , Agard D.A. , Ashworth A. and Ideker T. , The cancer cell map initiative: Defining the hallmark networks of cancer. Mol Cell 58: ((2015) ), 690–698. |
[15] | Leonard J.V. and Morris A.A. , Diagnosis and early management of inborn errors of metabolism presenting around the time of birth. Acta Paediatr 95: ((2006) ), 6–14. |
[16] | Cleary M.A. and Green A. , Developmental delay: When to suspect and how to investigate for an inborn error of metabolism. Arch Dis Child 90: ((2005) ), 1128–1132. |
[17] | Francescatto L. and Katsanis. N. , Newborn screening and the era of medical genomics. Semin Perinatol 39: ((2015) ), 617–622. |
[18] | Baker M.W. , Atkins A.E. , Cordovado S.K. , Hendrix M. , Earley M.C. and Farrell P.M. , Improving newborn screening for cystic fibrosis using next-generation sequencing technology: A technical feasibility study. Genet Med 18: (3) ((2016) ), 231–238. |
[19] | Saunders C.J. , Miller N.A. , Soden S.E. , Dinwiddie D.L. , Noll A. , Alnadi N.A. , Andraws N. , Patterson M.L. , Krivohlavek L.A. , Fellis J. , Humphray S. , Saffrey P. , Kingsbury Z. , Weir J.C. , Betley J. , Grocock R.J. , Margulies E.H. , Farrow E.G. , Artman M. , Safina N.P. , Petrikin J.E. , Hall K.P. and Kingsmore S.F. , Rapid whole-genome sequencing for genetic disease diagnosis in neonatal intensive care units. Sci Transl Med 4: ((2012) ), 154ra135. |
[20] | Worthey E.A. , Mayer A.N. , Syverson G.D. , Helbling D. , Bonacci B.B. , Decker B. , Serpe J.M. , Dasu T. , Tschannen M.R. , Veith R.L. , Basehore M.J. , Broeckel U. , Tomita-Mitchell A. , Arca M.J. , Casper J.T. , Margolis D.A. , Bick D.P. , Hessner M.J. , Routes J.M. , Verbsky J.W. , Jacob H.J. and Dimmock D.P. , Making a definitive diagnosis: Successful clinical application of whole exome sequencing in a child with intractable inflammatory bowel disease. Genet Med 13: ((2011) ), 255–262. |
[21] | Amaral M.D. , Novel personalized therapies for cystic fibrosis: Treating the defect in all patients. J Int Med 277: ((2015) ), 155–166 basic. |
[22] | Kolb S.J. and Kissel J.T. , Spinal muscular atrophy. Neurol Clin 33: ((2015) ), 831–846. |
[23] | Falzarano M.S. , Scotton C. , Passarelli C. and Ferlini A. , Duchenne muscular dystrophy: From diagnosis to therapy. Molecules 20: ((2015) ), 18168–18184. |
[24] | Guthrie R. and Susi A. , A simple phenylalanine method for detecting PKU in large populations of newborn infants. Pediatrics 32: ((1963) ), 338–343. |
[25] | Ziadeh R. , Hoffman E.P. and Finegold D.N. , et al., Medium chain acyl-CoA dehydrogenase deficiency in Pennsylvania: Neonatal screening shows high incidence and unexpected mutation frequencies. Pediatr Res 37: ((1995) ), 675–678. |
[26] | Millington D.S. , Kodo N. , Norwood D.L. and Roc C.R. , Tandem mass spectrometry: A new method for acylcarnitine profiling with potential for neonatal screening for inborn errors of metabolism. J Inherit Metab Dis 13: ((1990) ), 321–324. |
[27] | Ernst L.M. , Sondheimer N. and Deardorff M.A. , et al., The value of the metabolic autopsy in the pediatric hospital setting. J Pediatr 148: ((2006) ), 779–783. |
[28] | Kaback M. , Lim-Steele J. , Dabholkar D. , Brown D. , Levy N. and Zeiger K. , Tay-Sachs disease–carrier screening, prenatal diagnosis, and the molecular era. An international perspective, to The International TSD Data Collection Network. JAMA 270: ((1993) ), 2307–2315. |
[29] | Lew R.M. , Burnett L. , Proos A.L. , Barlow-Stewart K. , Delatycki M.B. , Bankier A. , Aizenberg H. , Field M.J. , Berman Y. , Fleischer R. and Fietz M. , Ashkenazi Jewish population screening for Tay-Sachs disease: The international and Australian experience. J Paediatr Child Health 51: ((2015) ), 271–279. |
[30] | Powell K. , Braun Van Naarden K. and Singh R., et al., Prevalence of developmental disabilities and receipt of special education services among children with an inborn error of metabolism. J Pediatr 156: ((2010) ), 420–426. |
[31] | Braverman N.E. , D’Agostino M.D. and Maclean. G.E. , Peroxisome biogenesis disorders: Biological, clinical and pathophysiological perspectives. Dev Disabil Res Rev 17: ((2013) ), 187–196. |
[32] | Clarke J.T.R. , (Ed.), A Clinical Guide to Inherited Metabolic Diseases – 3rd ed, Cambridge University Press, (2006) . |
[33] | Deem M.J. , Whole-Genome Sequencing and Disability in the NICU: Exploring Practical and Ethical Challenges. Pediatrics 137: (Suppl 1) ((2016) ), S47–S55. |
Figures and Tables
Fig.1
Vacuolated lymphocyte in the peripheral blood of a patient with mannosidosis.
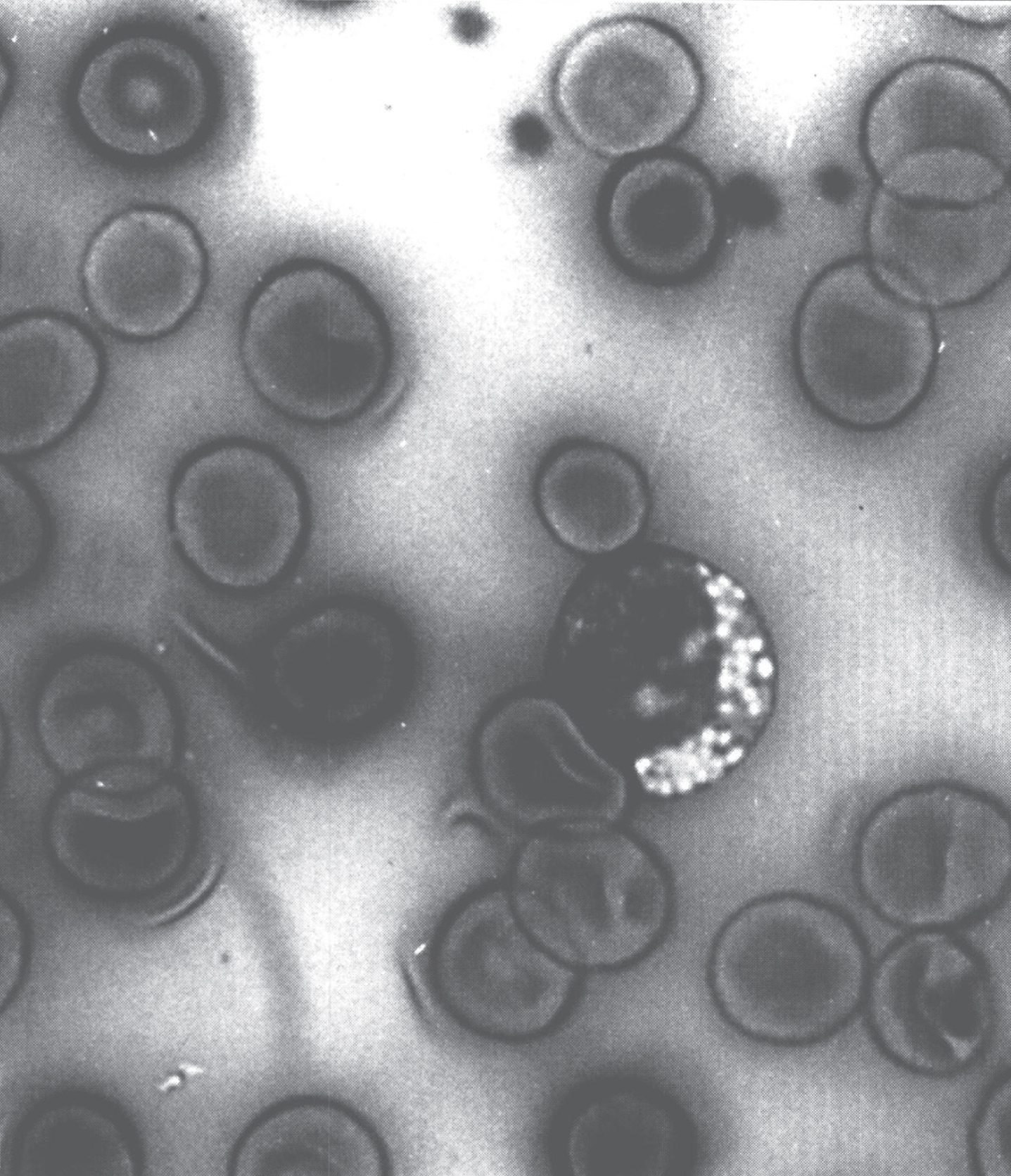
Fig.2
Approach to the differential diagnosis of hypoglycemia. Abbreviations: SGA, small for gestational age; IDM, infant of diabetic mother; HFI, hereditary fructose intolerance; AA, amino acids; OA, organic acids; FFA, free fatty acids; FAOD, fatty acid oxidation defect; hGH, human growth hormone; T4, thyroxine; GSD, glycogen storage disease; FDPase, fructose-6-diphosphatase. Clarke JTR, (Ed.): A Clinical Guide to Inherited Metabolic Diseases – 3rd ed., Cambridge University Press, 2006.
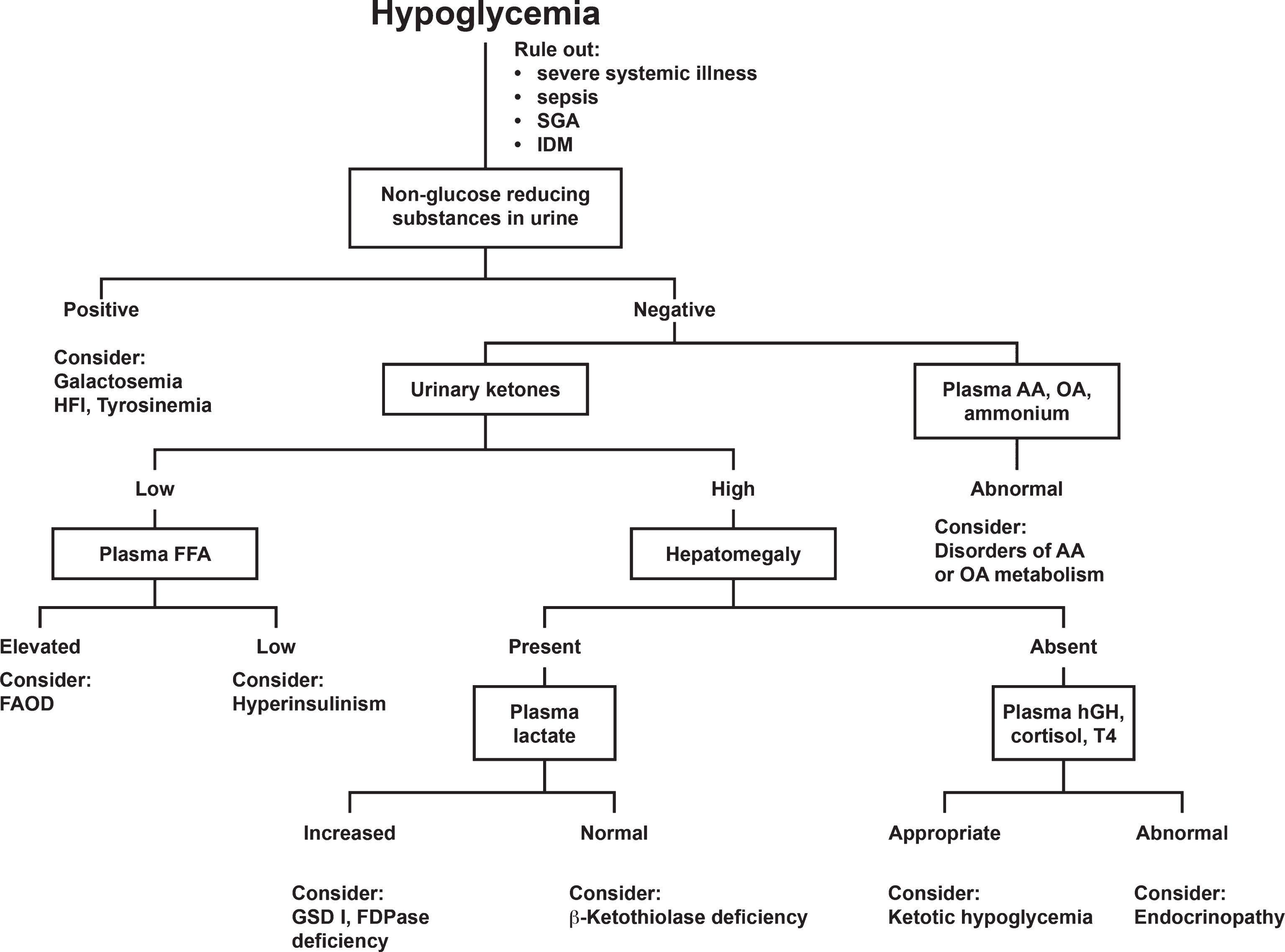
Table 1
Estimation of genetic disease frequencies
• 1% of live-born infants have monogenic disease |
• 5% of individuals under 25 can expect to have a disease due to a genetic component |
• 6% – 8% of hospitalized children have monogenic disease |
• 29% – 41% of hospitalized children have gene-influenced disease |
• 60% of older individuals have genetically influenced diseases, if multifactorial diseases are included |
• 100% of humans carry 6–8 lethal genes |
• 20% – 30% of all infant deaths are due to genetic disorders |
• 30% – 50% of post-neonatal deaths are due to congenital malformations |
• 50% of mental retardation has a genetic basis |
Table 2
Categories of metabolic disease (Examples)
Large Complex Molecule Diseases |
Complex lipid degradation (Gaucher, Niemann-Pick, Tay-Sachs, metachromatic leukodystrophy) |
Glycoprotein degradation (fucosidase and mannosidase deficiency) |
Mucopolysaccharidoses (Sanfilippo and Hurler syndrome) |
Lipofuscin storage disorders (Batten disease) |
Glycogen storage disease type I (von Gierke disease), type II (Pompe disease), type III (debrancher deficiency) |
Peroxisomal disorders (Zellweger syndrome) |
Small Molecule Diseases |
Amino acid metabolism disorders (phenylketonuria, maple syrup urine disease, homocystinuria, tyrosinemia) |
Ammonia metabolism disorders (ornithine transcarbamylase deficiency) |
Organic acid metabolism disorders (methylmalonic acidemia, propionic acidemia, isovaleric acidemia) |
Monosaccharides (galactosemia, hereditary fructose intolerance) |
Pyruvic and lactic acid metabolism disorders (primary lactic acidosis, mitochondrial disorders) |
Fatty acid metabolism disorders (medium-chain acyl-CoA dehydrogenase deficiency) |
Purines and pyrimidine metabolism disorders (Lesch-Nyhan syndrome) |
Vitamin and cofactor metabolism disorders (biotinidase deficiency) |
Other Diseases |
Metal metabolism disorders (Wilson and Menkes disease, hemochromatosis) |
Lipoprotein metabolism disorders (Abetalipoproteinemia, familial hypercholesterolemia) |
Porphyrin metabolism disorders (porphyria) |
Membrane transport disorders (cystinuria, cystinosis) |
Intestinal disaccharidases |
Collagen and connective tissue disorders (Ehlers-Danlos disease, Marfan syndrome) |
Congenital adrenal hyperplasia |
Hyperinsulinism |
Table 3
Clinical findings associated with specific metabolic diseases
Abdomen |
Hepatomegaly |
Galactosemia |
Glycogen storage disease, type 1 |
Hereditary fructose intolerance |
Fructose-1,6-diphosphatase deficiency |
Methylmalonic acidemia |
Propionic acidemia |
Glutaric acidemia, type II |
Very-long-chain acyl-CoA deficiency |
Medium-chain acyl-CoA deficiency |
Short-chain acyl-CoA deficiency |
Long-chain 3-OH acyl-CoA, dehydrogenase deficiency |
Carnitine transporter defect |
Carnitine palmityl transferase I o II deficiency |
Acylcarnitine translocase deficiency |
3-hydroxy-3-methylglutaryl-CoA lyase deficiency |
Phosphoenolpyruvate carboxykinase deficiency |
Mitochondrial respiratory/electron transport chain defects |
Hereditary tyrosinemia, type I |
Argininosuccinicaciduria |
α1-Antitrypsin deficiency |
Smith-Lemli-Opitz syndrome |
Zellweger syndrome |
Neonatal adrenoleukodystrophy |
Nieman-Pick disease, type C |
Hepatosplenomegaly |
GM1 gangliosidosis |
I-cell disease |
Gaucher disease, type I |
Niemann-Pick disease, type A, C |
Galactosialidosis |
Sialidosis |
Mucopolysaccharidosis, type VII |
Wolman disease |
Pancreatitis |
Glycogen storage disease, type I |
Lipoprotein lipase deficiency |
Homocystinuria |
Hydroxymethylgiutaryl-CoA lyase deficiency |
Methylmalonic acidemia |
Propionic acidemia |
Pearson syndrome |
Chest |
Cardiomyopathy |
Glycogen storage |
disease, type I |
Glycogen storage |
disease, type II |
Glycogen storage disease, type III |
Electron transport chain abnormalities |
Disorders of fatty acid oxidation |
Mucopolysaccharidoses |
Myocardial infarction |
Familial Hypocholesterolemia |
Fabry disease |
Homocystinuria |
Menkes disease |
Diarrhea |
Lysinuric protein intolerance |
Shwachman syndrome |
Johansson Blizzard syndrome |
Pearson syndrome |
Congenital chloride diarrhea |
Glucose galactose malabsorption |
Lactase deficiency |
Sucrase deficiency |
Abetalipoproteinemia |
Wolman disease |
Diarrhea (Persistent) |
Sucrase isomaltase deficiency |
Acrodermatitis enteropathica |
Congenital folate malabsorption |
Dysmorphic Features |
Glutaric aciduria, type II |
Glomerulopathy |
Renal cystic dysplasia |
Cerebral dysgenesis |
Facial dysmorphism |
Congenital heart disease |
Genital anomalies |
Pyruvate dehydrogenase deficiency |
Microcephaly |
“;Fetal alcohol”; facies |
Agenesis of corpus callosum |
Peroxisomal Disorders |
Zellweger Syndrome |
Renal microcysts |
Epiphyseal calcification |
Facial dysmorphism |
Congenital heart disease |
Cerebral dysgenesis |
Hepatopathy |
Infantile Refsum disease |
Facial dysmorphism |
Hepatopathy |
Rhizomelic chondrodysplasia punctata |
Facial dysmorphism |
Rhizomelic limb shortening |
GM1 gangliosidosis |
Frontal bossing, low-set ears |
Congenital adrenal hyperplasia |
Ambiguous genitalia |
Sialidosis |
Coarse facial features, stippled epiphyses |
Mucolipidosis II |
Coarse facial features |
Mucopolysaccharidoses |
Coarse facial features |
Infantile sialic acid storage disease |
Coarse facial features |
β-Hydroxyisobutyryl-CoA deacylase deficiency |
Congenital heart defects |
Agenesis of corpus callosum |
Dysmorphic facies |
Gaucher-like storage disease |
Arthrogryposis |
Muscle phosphorylase deficiency |
Arthrogryposis |
Smith-Lemli-Opitz syndrome |
Genital and digital abnormalities |
Ears |
Otic atrophy |
Pyruvate dehydrogenase com plex deficiency |
Leigh disease |
Zellweger syndrome |
Extremities |
Arthritis |
Alkaptonuria |
Gaucher disease, type I |
Lesch-Nyhan disease |
Farber disease |
I-cell disease |
Mucolipidosis III |
Homocystinuria |
Mucopolysaccharidosis IS, HS |
Bone or limb deformity |
Dyostosis Multiplex |
Hurler, Hurler-Scheie disease |
Hunter disease |
Sanfilippo disease |
Generalized GM1 gangliosidosis |
Mucolipidosis II, I-cell disease |
Mucolipidosis III |
Galactosialidosis |
Maroteaux-Lamy disease |
Sly disease |
Multiple sulfatase deficiency |
Osteoporosis and/or fractures |
Propionic acidemia |
Methylmalonic acidemia |
Glycogen storage disease, type I |
Homocystinuria |
Adenosine deaminase deficiency |
Lysinuric protein intolerance |
Menkes disease |
Infantile Refsum disease |
Gaucher disease |
I-cell disease |
Eyes |
Cataract |
Galactosemia |
Lowe syndrome |
Mitochondrial respiratory electron transport chain defects |
Zellweger syndrome |
Rhizomelic chondrodysplasia punctata |
Mevalonic aciduria |
Corneal Clouding |
I-cell disease |
Steroid sulfatase deficiency |
Dislocated lens |
Methionine synthetase deficiency |
Sulfite oxidase deficiency |
Macular cherry-red spot |
GM1 gangliosidosis |
Galactosialidosis |
Niemann-Pick Disease, type A |
Tay-Sachs disease (GM2 gangliosidosis) |
Retinitis pigmentosa |
Mitochondrial respiratory electron transport chain defects |
Methylmalonic acidemia/ |
Homocystinuria |
Sjögren-Larsson syndrome |
Zellweger syndrome |
Neonatal adrenoleukodystrophy |
Infantile Refsum disease |
Abetalipoproteinemia |
Long-chain 3-OH acyl-CoA dehydrogenase deficiency |
Head |
Alopecia |
Multiple carboxylase deficiency |
Cerebral calcification |
Adrenoleukodystrophy |
Abnormalities of folate metabolism |
L-2-Hydroxyglutaric aciduria |
Biopterin abnormalities |
Coarse facial features |
GM1 gangliosidosis |
I-cell disease |
Mucopolysaccharidosis, type VII |
Sialidosis |
Galactosialidosis |
Macrocephaly |
4-Hydroxybutyric aciduria |
Glutaric aciduria, type I |
L-2-Hydroxyglutaric aciduria |
Neonatal adrenoleukodystrophy |
Tay-Sachs disease |
Hurler disease |
Krabbe disease |
Multiple sulfatase deficiency |
Canavan disease |
Mannosidosis |
3-Hydroxy-3-methylglutaric aciduria |
Pyruvate carboxylase deficiency |
Multiple acyl-CoA dehydrogenase deficiency |
Microcephaly |
Short-chain Acyl-CoA dehydrogenase deficiency |
Mitochondrial respiratory electron transport chain defects |
Leigh disease |
Steely hair |
Menkes disease |
Hematology |
Hematologic abnormalities |
Leukopenia with or without thrombopenia and anemia |
Methylmalonic acidemia |
Propionic acidemia |
Isovaleric acidemia |
3-Oxothiolase deficiency |
Abnormalities of folate metabolism |
Transcobalamin II deficiency |
Shwachman syndrome |
Pearson syndrome |
Johansson Blizzard syndrome |
Megaloblastosis |
Cobalamin metabolic errors: |
Methylmalonic acidemia |
Homocystinuria |
Transcobalamin II deficiency |
Orotic aciduria |
Mevalonic aciduria |
Pearson syndrome |
Abnormalities of folate metabolism |
Shwachman syndrome |
Johansson Blizzard syndrome |
Hydrops fetalis |
Gaucher disease |
GM1 gangliosidosis |
Salla disease |
Sialidosis |
Wolman disease |
β-Glucuronidase deficiency |
Congenital disorders of protein glycosylation |
Deficiencies of red cell glycolytic/pentose phosphate pathway enzymes (eg, G6PDH deficiency, private kinase deficiency) |
Farber disease |
Fumarase deficiency |
GSD IV |
MPS VII (Sly disease) |
MPS IVA (Morquio type A) |
Mucolipidosis I (Sialidosis) |
Mucolipidosis II (I-cell disease) |
Neonatal hemochromatosis |
Niemann-Pick C |
Primary carnitine deficiency |
Respiratory chain disorders |
Sialic acid storage disease |
Morquio syndrome |
Neuraminidase deficiency |
Myotonic dystrophy |
Perinatal iron storage syndrome |
Carnitine deficiency |
Niemann-Pick type C |
I-cell disease |
Farber disease |
Mucopolysaccharidosis type VII |
Carbohydrate-deficient glycoprotein storage disease |
Mouth and throat |
Macroglossia |
GM1 gangliosidosis |
Macroglossia |
Neurological |
MLD, Niemann-Pick A and B, and Gaucher, type III |
Gaucher disease, type II |
Glutaric acidemia, type I |
Krabbe disease |
Crigler-Najjar syndrome |
Phenylketonuria caused by a biopterin defect |
Stroke-like episodes |
Carbamoyl phosphate synthetase |
MELAS |
Carbohydrate deficient glycoprotein storage disease |
Ethylmalonic aciduria |
Fabry disease |
Glutaric aciduria, type I |
Homocystinuria |
Isovaleric acidemia |
Menkes disease |
3-MTHF reductase deficiency |
Methylmalonic acidemia |
Ornithine transcarbamylase |
deficiency |
Propionic acidemia |
Purine nucleoside phosphorylase deficiency |
Odor |
Sweaty feet, strong |
Glutaric aciduria (type II) |
Isovaleric acidemia |
Mousy or musty |
Phenylketonuria |
Maple syrup |
Maple syrup urine disease |
Tomcat urine |
β-Methylcrotonylglycinuria |
Cabbage |
Methionine malabsorption |
Rotting fish |
Trimethylaminuria |
Rancid fishy or cabbage |
Tyrosinemia |
Hoplike |
Oasthouse disease |
Swimming pool |
Hawkinsinuria |
Psychiatric |
Sanfilippo syndrome (MPS III) |
Hunter syndrome (MPS II) |
X-linked ALD |
Late-onset MLD |
Late-onset GM2 gangliosidosis |
Lesch-Nyhan syndrome |
Porphyria |
Wilson disease |
Cerebrotendinous xanthomatosis |
UCED |
Homocystinuria due to MTHF reductase deficiency |
Adult-onset NCL |
Skin |
Angiokeratoma |
Fabry disease |
GM1 gangliosidosis |
Fucosidosis |
Galactosialidosis |
Sialidosis |
Desquamating, eczematous, or vesiculobullous lesions |
Acrodermatitis enteropathica |
Multiple carboxylase deficiency |
Methylmalonic acidemia |
Propionic acidemia |
Hepatoerythropoietic porphyria |
Congenital erythropoietic porphyria |
Ichthyosis |
Multiple sulfatase deficiency |
X-linked ichthyosis (steroid sulfatase deficiency) |
Gaucher disease |
Krabbe disease |
Refsum disease |
Sjögren-Larsson syndrome |
Nodules |
Farber disease (Ceramidase deficiency) |
Thick skin |
I-cell disease |
GM2 gangliosidosis |
Mucopolysaccharidosis, type VII |
Sialidosis |
Galactosialidosis |
Xanthomas |
Familial hypercholesterolemia |
Lipoprotein lipase deficiency |
Niemann-pick disease |
ALD, adrenoleukodystrophy; MLD, metachromatic leukodystrophy; UCED, urea cycle enzyme defects; MTHF, methylenete-trahydrofolate; NCL, neuronal ceroid lipofuscinosis; MELAS, myoclonic epilepsy, lactic acidosis, and stroke.
Table 4
Newborn screening panel: Core panel and secondary targets*
MS/MS | ||||
Acylcarnitines | Amino acids | |||
9 Organic | 5 Fatty Acid | 6 Aminoacidopathies | 3 Hemoglobinopathies | 7 Other Genetic |
Acidemia Disorders | Oxidation Defects | Disorders | ||
Core panel | ||||
Isovaleric academia | Medium-chain acyl-COA dehydrogenase deficiency | Phenlketonuria | Sickle cell anemia | Congenital Hypothyroidism |
Glutaric acidemia type I | Very long-chain acyl-CoA | Maple syrup disease | (hemoglobin SS disease)* | Biotinidase deficiency |
3-hydroxy-3-methyl glutaric aciduria (HMG) | dehydrogenase deficiency | Homocystinuria* | Hemoglobin S/β-thalassemia* | Congenital adrenalhyperplasia* |
Multiple carboxylase deficiency | Long-chain L-3-hydroxy | (due to cystathionine beta synthase deficiency) | Hemoglobin S/C disease* | (21-hydroxylase deficiency) |
Methylmalonic acidemia (mutase deficiency)* | acyl-CoA dehydrogenase | Citrullinemia | Classical galactosemia | |
3-Methylcrotonyl-CoA | deficiency | Argininosuccinic academia | Hearing loss | |
carboxylase deficiency* | Trifunctional protein deficiency | Tyrosinemia type I* | Cystic fibrosis | |
Methylmalonic acidemia | Carnitine uptake defect | Severe combined | ||
(cobalamin disorders A &B)* | immunodeficiency | |||
Propionic acidemia | ||||
B-Ketothiolase deficiency |
Secondary targets | ||||
6 More Organic | 8 More Fatty Acid | 8 More | Other | 2 Other Hereditary |
Acidemia Disorders | Oxidation Defects | Aminioacidopathies | Hemoglobinopathies | Metabolic Disorders |
Methylmalonic academia* (cobalamin disorders C &D) | Short-chain acyl-CoA dehydrogenase deficiency | Benign hyperphenylalaninemia | Variant hemoglobinopathies* (including hemoglobin E) | Galactokinase deficiency* |
Glutaric acidemia type II | Tyrosinemia type II | Galactose epimerase deficiency | ||
Malonic academia | Medium/short-chain L-3-hydroxy acyl-CoA dehydrogenase deficiency | Defects of biopterin cofactor biosynthesis | ||
Isobutyryl-CoA dehydrogenase deficiency | Medium-chain ketoacyl-CoA thiolase deficiency | Argininemia | ||
2-Methyl 3-hydroxy butyric aciduria | Carnitine palmitoyltransferase II deficiency | Tyrosinemia type III | ||
2-Methylbutyryl-CoA dehydrogenase deficiency | Carnitine: acylcarnitine translocase deficiency | Defects of biopterin cofactor regeneration | ||
3-Methylglutaconic aciduria | Carnitine palmitoyltransferase I deficiency (liver) | Hypermethioninemia | ||
Dienoyl-CoA reductase deficiency | Citrullinemia type II |
*From Newborn Screening: Toward a Uniform Screening Panel and System, Maternal and Child Health Bureau. [http://mchb.hrsa.gov/screening/].
Table 5
Ethnic group incidence of inborn errors of metabolism
Inborn Error | Ethnic Group | Estimated Incidence |
(per 100000 births) | ||
Hepatorenal tyrosinemia | French-Canadians (Saguenay-Lac Saint-Jean region) | 54 (before the introduction of preventive prenatal diagnosis) |
Tay-Sachs disease | Canavan disease Ashkenazi Jews | 33 (before the introduction of preventive prenatal diagnosis) |
Gaucher disease, type 1 | Canavan disease Ashkenazi Jews | 100 |
Phenylketonuria (PKU) | Canavan disease Ashkenazi Jews | 5 |
Yemenite Jews | 19 | |
Turkish | 38.5 | |
Porphyria variegata | South African (white) | 300 |
Congenital adrenal hyperplasia | Yupik Eskimos | 200 |
Glutaric aciduria, type 1 | Ojibway Indians (Canada) | >50 |
Maple syrup urine disease | Mennonites (Pennsylvania) | 568 |
From: Clarke JTR, (Ed.): A Clinical Guide to Inherited Metabolic Diseases – 3rd ed., Cambridge University Press, 2006.
Table 6
Laboratory findings in some inborn errors of metabolism
Lactic acidosis ± Hyperammonemia |
Methylmalonic acidemia |
Propionic acidemia |
Isovaleric acidemia |
Multiple carboxylase deficiency |
Maple syrup urine disease |
Glutaric acidemia, type I |
Glutaric acidemia, type II |
Short-chain acyl-CoA dehydrogenase deficiency |
Long-chain 3-OH acyl-CoA dehydrogenase deficiency |
Ketothiolase deficiency |
Acetoacetate CoA ligase deficiency |
3-hydroxy-3-methylglutaryl CoA Iyase deficiency |
Pyruvate dehydrogenase complex deficiency |
Pyruvate carboxylase deficiency |
Phosphoenolpyruvate carboxykinase deficiency |
Mitochondrial respiratory/electron transport chain defects |
Leigh disease |
Hereditary galactosemia |
Glycogen storage disease, type I |
Hereditary fructose intolerance |
Fructose-1,6-diphosphatase deficiency |
Hereditary tyrosinemia, type I |
Respiratory alkalosis |
Omithine transcarbamylase deficiency |
Carbamoyl phosphate synthetase deficiency |
Argininosuccinicaciduria |
Citrullinemia |
Hyperammonemia |
Ornithine transcarbamylase deficiency |
Carbamoyl phosphate synthetase deficiency |
Argininosuccinicaciduria |
Citrullinemia |
Methylmalonic acidemia |
Propionic acidemia |
Isovaleric acidemia |
Multiple carboxylase deficiency |
Glutaric acidemia, type II |
Very-long-chain acyl-CoA dehydrogenase deficiency |
Medium-chain acyl-CoA dehydrogenase deficiency |
Short-chain acyl-CoA dehydrogenase deficiency |
Acylcarnitine translocase deficiency |
Ketosis |
Methylmalonic acidemia |
Propionic acidemia |
Isovaleric acidemia |
Multiple carboxylase deficiency |
Maple syrup urine disease |
Glutaric acidemia, type II |
Short-chain acyl-CoA dehydrogenase deficiency |
Ketothiolase deficiency |
Acetoacetate-CoA ligase deficiency |
Pyruvate carboxylase deficiency |
Glycogen storage disease, type I |
Fructose-1,6-diphosphatase deficiency |
Hypoglycemia |
Hyperinsulinism |
Glycogen storage disease, type I |
Hereditary fructose intolerance |
Fructose-1,6-diphosphatase deficiency |
Glutaric acidemia, type I |
Glutaric acidemia, type II |
Very-long-chain acyl-CoA dehydrogenase deficiency |
Medium-chain acyl-CoA dehydrogenase deficiency |
Short-chain acyl-CoA dehydrogenase deficiency |
Long-chain 3-OH acyl-CoA dehydrogenase deficiency |
Carnitine transporter defect |
Carnitine palmitoyl transferase I deficiency |
Carnitine palmitoyl transferase II deficiency |
Acylcarnitine translocase deficiency |
Ketothiolase deficiency |
Acetoacetate-CoA ligase deficiency |
3-hydroxy-3-methylglutaryl-CoA Iyase deficiency |
Hereditary galactosemia Neonatal hemochromatosis |
Mitochondrial respiratory/electron transport chain defects |
Lipemia |
Glycogen storage disease, type I |
Table 7
Laboratory evaluation for suspected inborn errors of metabolism
Comments | |
Initial Evaluation* | |
Blood tests | |
CBC with differential | |
Blood glucose | |
Electrolytes, BUN, creatinine, uric acid | |
Arterial blood gas | |
Serum ammonia | Should be obtained from artery or vein without a tourniquet; the tube should be placed on ice for transport to the laboratory and analyzed immediately. If the plasma ammonia concentration is >100 micromol/L (1.7 mcg/mL), the measurement should be repeated immediately |
AST, ALT, bilirubin, PT | If the patient has signs or symptoms of myopathy |
LDH, aldolase, creatine, kinase | |
Urine Tests | |
Color, odor | |
Urinalysis | |
Reducing substances | |
Myoglobin | If the patient has signs or symptoms of myopathy |
Specialized Tests | |
Blood tests | |
Quantitative plasma amino acids | Plasma amino acid analysis must be performed quantitatively rather than qualitatively |
Lactate and pyruvate | Lactate and pyruvate should be measured in arterial blood that is, transported on ice |
Acylcarnitine profile | Analysis of acylcarnitine conjugates is performed by tandem mass spectrometry and can be measured in a plasma sample or a filter-paper bloodspot; serum is preferred because of inherent problems in quantitating compounds from a filter-paper blood spot |
Urine Tests | |
Qualitative urine organic acids | Minimum of 2 to 5 mL in sterile container without preservative |
CBC: complete blood count; ALT: alanine aminotransferase; BUN: blood urea nitrogen; PT: prothrombin time; AST: aspartate aminotransferase; LDH: lactate dehydrogenase. *If possible, blood and urine samples should be obtained for both the initial and specialized tests at the time of presentation. Samples for specialized tests should be processed and stored appropriately for further testing if indicated.
Table 8
Abnormal metabolites in metabolic disorders
Disorder | Abnormal Metabolite (s)* |
Organic Acidemias | |
Methylmalonic academia | Methylmalonic and methylcitric acids |
Propionic academia | 3-Hydroxpropionic acid propionylglycine, methylcitric acid |
Isovaleric academia | Isovalerylglycine |
Glutaric academia type I | Glutaric and 3-hydroxyglutaric acids |
3-Methylglutaconic aciduria | 3-Methylglutaconic acid |
2-Hydroxy-3-methylbutyryl-CoA dehydrogenase deficiency | 2-Hydroxy-3-methylblutyric acid, tiglyglycine, 2-methyl-3-hydroxyacetoacetic acid |
2-Hydroxyglutaric aciduria | 2-Hydroxyglutaric acid |
Disorders of Ketogenesis | |
Medium chain acyl-CoA dehydrogenase (MCAD) deficiency | Hexanoylglycine and suberylglycine |
3-ketothiolase deficiency | 2-hydroxy-3-methylbutyric acid, tiglyglycine, 2-methyl-3-hydroxyacetoacetic acid |
3-Hydroxy-3-methylglutaryl (HMG)-CoA lyase deficiency | 3-Hydroxy-3-methylglutaric, 3-methylglutaric and 3-methylglutaconic acids |
Other Disorders | |
Canavan disease | N-acetylaspartic acid |
Glutaric academia type II (multiple acyl-CoA dehydrogenase deficiency) | Glutaric and 2-hydroxyglutaric acids |
Mevalonate kinase deficiency | Mevalonic acid |
Maple syrup urine disease | 2-Hydroxyisovaleric and 2-hydroxy-3-methylvaleric |
Phenylketonuria | Phenylpyruvic and phenyllactic acids |
Fumarase deficiency | Fumaric acid |
Glutathione synthetase deificneyc | 5-Oxoproline (pyroglutamic acid) |
Biotinidase deficiency* | 3-Hydroxyisovaleric acid, methylcitric acid, 3-methylcrotonlglycine, propionylglycine |
Holocarboxylase synthetase deficiency* | 3-Hydroxyisovaleric acid, methylcitric acid, 3-methylcrotonylglycine, propionylglycine |
Glycerol kinase deficiency | Glycerol |
Ethylmalonic encephalopathy | Ethylmalonic and methylsuccinic acids |
Disorders of Uncertain Consequence | |
3-methylcrotonyl-CoA carboxlase deficiency | 3-methylcrotonylglycine |
Short chain acyl-CoA dehydrogenase deficiency | Ethylmalonic acid, butyrylglycine |
*Other abnormal metabolites may be seen in these disorders but those listed are the most characteristic for the diagnosis.
Table 9
Distinguishing biochemical findings of inborn errors of metabolism
Findings | MSUD | OA | UCD | DCM | FAO | MD | PD | LSD |
Metabolic acidosis | ± | ++ | – | ± | ± | ± | – | – |
Respiratory alkalosis | – | – | + | – | – | – | – | – |
Hyperammonemia | ± | + | ++ | – | ± | – | – | – |
Hypoglyclemia | ± | ± | – | + | + | ± | – | – |
Ketones | A/H | H | A | A/H | A/L | A/H | A | A |
Lactic acidosis | ± | ± | – | + | ± | ++ | – | – |
MSUD: maple syrup urine disease; OA: organic acidemias’ UCD: urea cycle disorders; DCM: disorders of carbohydrate metabolism; FAO: fatty acid oxidation disorders; MD: mitochondrial disorders; PD: peroxisomal disorders; LSD: lysosomal storage disorder. – : usually absent; ±: sometimes present; +: usually present; ++: always present. A: appropriate; H: inappropriately high; L: inappropriately low. Adapted from: Weiner DL, Metabolic Emergencies. In: Textbook of Pediatric Emergency Medicine, 5th ed. Fleisher GR, Ludwig S, Henretig FM (Eds). Lippincott, Williams & Wilkins, Philadelphia, 2006.
Table 10A
Physical examination findings as clues to inborn errors of Metabolism
Finding | Potential Inborn Error |
General | |
Tall, long-limbed body habitus | Homocysturia |
Head | |
Coarse facial features (eg, hirsuitismprominent brow ridge, and gingivalhypertrophy) | Oligosaccharidoses, mucopolysaccharidoses,mucolipidoses |
Microcephaly | Untreated phenylketonuria (PKU), maternal PKU syndrome, congenital disorders of protein glycosylation, leukodystrophies (late, organic acidemias, urea cycle disorders, maple syrup urine disease |
Macrocephaly | Canavan disease, glutaric academia type I, oligosaccharidoses, mucopolysaccharidoses, mucolipidoses, Tay-Sachs (early) |
Hair | |
Alopecia | Biotinidase deficiency, vitamin D resistant rickets |
Sparse | Biotinidase deficiency, Menkes disease |
Kinky, brittle | Argininosuccinic aciduria and citrullinemia (due to arginine deficiency), Menkes disease, mucopolysaccharidoses |
Eyes | |
Cataracts | Oligosaccharidoses, Fabry diseiase, neuronal ceroid lipofuscinosis, galactosemia, Smith-Lemli-Opitz syndrome peroxisome bioigenesis defects, rhizomelic chondrodysplasia punctata, Wilson disease, errors of mitochondrial oxidative phosphorylation |
Cherry red spot | Tay-Sachs disease, Sandhoff disease, Sialidosis type I and type II, GM1-gangliosidosis, Niemann-Pick disease type A, Gaucher disease type 2, metachromatic leukodystrophy, galactosialidosis |
Corneal clouding | Oligosaccharidoses, mucopolysaccharidoses, mucolipidoses, Tangier, sialidosis |
Corneal opacity | Oligosaccharidoses, Fabry disease, steroid sulfatase deficiency (X-linked ichthyosis), Tangier, molybdenum cofactor deficiency, sulfite oxidase deficiency |
Dislocated lens | Homocystinuria, sulfite oxidase deficiency |
Kayser-Fleischer rings | Wilson disease |
Retitinitis pigmentosa | Abetalipoproteinemia, peroxisome biogenesis disorders of protein glycosylation, fatty acid oxidation defects, mitochondrial defects, mucopolysaccharidoses, Krabbe disease, Menkes disease, disorders of cobalamin (vitamin B12) transport and synthesis, ornithine aminotransferase deficiency |
Adapted from Wappner Rs, Hainline BE. Inborn errors of metabolism. In: Oski’s Pediatrics, Principles and Practice, 3rd ed., McMillan JA, DeAngelis CD, Feigin RD, Warshaw JB (Eds), Lippincott, Williams and Wilkins, Philadelphia, 1999. Saudubray JM, Chappentier C. Clinical phenotypes: Diagnosis/algorithms. In: Metabolic and Molecular Bases of Inherited Disease, 8th ed, Scriver CR, Beaudet AL, Sly WS, Valle D (Eds), McGrall-Hill, New York, 2001.Lindor NM, Karnes PS. Initial assessment of infants and children with suspected inborn errors of metabolism. Mayo Clin Proc 70:987, 1995. Cleary MA, Green A. Developmental delay: when to suspect and how to investigate for an inborn error of metabolism, Arch Dis Child 90:1128, 2005.
Table 10B
Physical examination findings as clues to inborn errors of Metabolism
Finding | Potential Inborn Error |
Hearing loss | Peroxisomal disorders, mitochondrial disorders, lysosomal storage disorders, mucopolysaccharidoses |
Mouth | |
Gingival hyperplasia | Oligosaccharidoses, mucopolysaccharidoses, mucolipidoses |
Chest | |
Inverted nipples | Congenital disorders of protein glycosylation |
Abdomen | |
Hepatosplenomegaly | Lysosomal storage disease |
Hepatomegaly | Glycogen storage diseases, carnitine palmitoyltransferase II deficiency (infantile form), peroxisomal disorders, mitochondrial DNA depletion disorders, tyrosinemia type I (hepatorenal), mucolipidoses, congenital disorders of protein glycosylation, longer chain fatty acid oxidation disorders |
Musculoskeletal | |
Arthritis | Farber disease, purine metabolism disorders |
Neurologic | |
Dystonia | Glutaric acidemia I, organic acidemias, Wilson disease, mitochondrial disorders |
Myopathy | Fatty acid oxidation defects, mitochondrial disorders, Pompe disease and other glycogen storage diseases |
Paresthesia | Fabry disease, sialidosis |
Peripheral neuropathy | Congenital disorders of protein glycosylation, leukodystrophies, peroxisomal disorders, Tangier disease |
Psychoses | Adult MLD Tay-Sachs, homocystinuria, porphyrias, purine metabolism disorders |
Skin | |
Hypopigmentation or absent pigment | Cystinosis, Menkes disease, phenylketonuria, sialidosis |
Angiokeratoma | Fabry, fucosidosis, galactosialidosis, beta-mannosidosis, sialidosis |
Dermatitis | Biotinidase deficiency, Hartup disease, phenylketonuria, prolidase deficiency |
Edema | GM1 gangliosidosis, prolidase deficiency |
Hirsutism | Oligosaccharidoses, mucopolysaccharidoses, mucolipidoses |
Ichthyosis | Multiple sulfatase deficiency, isolated steroid sulfatase deficiency |
Photosentization | Porphyrias |
Xanthomas | Hyperlipoproteinemias and other disorders of lipoproteins |
Adapted from Wappner Rs, Hainline BE. Inborn errors of metabolism. In: Oski’s Pediatrics, Principles and Practice, 3rd ed., McMillan JA, DeAngelis CD, Feigin RD, Warshaw JB (Eds), Lippincott, Williams and Wilkins, Philadelphia, 1999. Saudubray JM, Chappentier C. Clinical phenotypes: Diagnosis/algorithms. In: Metabolic and Molecular Bases of Inherited Disease, 8th ed, Scriver CR, Beaudet AL, Sly WS, Valle D (Eds), McGrall-Hill, New York, 2001.Lindor NM, Karnes PS. Initial assessment of infants and children with suspected inborn errors of metabolism. Mayo Clin Proc 70:987, 1995. Cleary MA, Green A. Developmental delay: when to suspect and how to investigate for an inborn error of metabolism, Arch Dis Child 90:1128, 2005.
Table 11
Differential diagnosis of acute encephalopathy in metabolic diseases
UCED | MSUD | OAuria | FAOD | ETC Defects | |
Metabolic acidosis | 0 | ± | +++ | ± | ++ |
Plasma glucose | N | N or ↓ | ↓↓ | ↓↓↓ | N |
Urinary ketones | N | ↑↑ | ↑↑ | 0 | 0 |
Plasma ammonia | ↑↑↑ | N | ↑↑ | ↑ | N |
Plasma lactate | N | N | ↑ | ± | ↑↑↑ |
Liver function | ±N | N | N | ↑↑ | N |
Plasma carnitine | N | N | ↓↓↓ | ↓↓ | N |
Plasma amino acids | Abnormal | ↑ BCAA | ↑ glycine | ± | ↑ alanine |
Urinary organic acids | N | Abnormal | Abnormal | Abnormal | N |
UCED, urea cycle enzyme defect; MSUD, maple syrup urine disease; OAuria, organic aciduria; FAOD, fatty acid oxidation defect; ETC, mitochondrial electron transport chain; BCAA, branched-chain amino acids; ↑, elevated; ↓ decreased;+, present; ±, variably present; N, normal; O, not present. From: Clarke JTR, (Ed.): A Clinical Guide to Inherited Metabolic Diseases – 3rd ed., Cambridge University Press, 2006.