Impact of rehabilitation on volumetric muscle loss in subjects with traumatic spinal cord injury: A systematic review
Abstract
BACKGROUND:
Spinal cord injury (SCI) leads to spinal nerve fiber tract damage resulting in functional impairments. Volumetric muscle loss (VML), a skeletal muscle volume abnormal reduction, is represented by atrophy below the injury level. The strategies for VML management included personalized approaches, and no definite indications are available.
OBJECTIVE:
To identify the rehabilitation effects of VML in subjects with SCI (humans and animals).
METHODS:
PubMed, Scopus, and Web of Science databases were systematically searched to identify longitudinal observational studies with individuals affected by traumatic SCI as participants; rehabilitation treatment as intervention; no control, sham treatment, and electrical stimulation programs as control; total lean body and lower limb lean mass, cross-sectional area, functional gait recovery, muscle thickness, and ultrasound intensity, as outcome.
RESULTS:
Twenty-four longitudinal observational studies were included, evaluating different rehabilitation approaches’ effects on the VML reduction in subjects affected by SCI. The data showed that electrical stimulation and treadmill training are effective in reducing the VML in this population.
CONCLUSION:
This systematic review underlines the need to treat subjects with traumatic SCI (humans and animals) with different rehabilitation approaches to prevent VML in the subacute and chronic phases. Further clinical observations are needed to overcome the bias and to define the intervention’s timing and modalities.
1Introduction
Spinal cord injury (SCI) is a devastating condition that leads to spinal nerve fiber tracts damage resulting in motor, sensory, and autonomous functions impairments below the injury level (Rupp, 2020), with significant personal and societal loss (Hachem et al.., 2017). Worldwide, the incidence of SCI is 0.9 (95% UI, 0.7–1.2) million cases for both sexes, with an age-standardized incidence rate of 12 (95% UI, 9–15) per 100,000 (Ding et al.., 2022). SCI may be due to traumatic (e.g., contusion, compression, laceration) or non-traumatic events (e.g., degenerative cervical myelopathy, cancer, infection, intervertebral disc disease, vertebral injury, and spinal cord vascular disease) (Ge et al.., 2018), and the cervical spine is the most involved tract (Singh et al.., 2014). Particularly, the traumatic spinal cord injury (TSCI)’s documented incidence ranges between 12.1 and 57.8 cases per million inhabitants in high-income countries and between 12.7 and 29.7 in low-income countries (Barbiellini Amidei et al.., 2022). Even if no significant changes in the incidence of SCI were observed over the last 25 years, it is necessary to underline relevant differences concerning its demographic characteristics: the average age of onset increased from 29 to 42 years, reflecting a higher number of people who get this injury as a result of accidental falls, which, more frequently, resulting in incomplete tetraplegia (Algahtany et al.., 2021; Bárbara-Bataller et al.., 2018; Devivo, 2012).
The International Standards for Neurological Classification of Spinal Cord Injury (ISNCSCI), endorsed by the American Spinal Injury Association (ASIA) and the International Spinal Cord Society (ISCoS), is the most widely used classification (ASIA and ISCoS International Standards Committee, 2019). This classification allows to examine of sensory and motor functions and define the lesion completeness or incompleteness according to the ASIA Impairment Scale (AIS), with a huge prognostic role if applied within the first 72 hours after the traumatic event (Kirshblum, Botticello, et al.., 2020; Kirshblum, Snider, et al.., 2020). Furthermore, van Middendorp et al.. and the European Multicenter Study about Spinal Cord Injury (EMSCI), in a longitudinal cohort study, stated that sensitivity and muscle strength assessed on L3 and S1 within 2-weeks of injury might predict with 95% confidence the probability of independent walking one year after injury (van Middendorp et al.., 2011).
From a pathophysiological point of view, SCI is characterized by a primary and a second phase (McDonald & Sadowsky, 2002; Tator, 1995). Primary SCI results directly from the physical forces due to the traumatic event and is often the injury severity principal determinant (Ackery et al., 2004; Yip & Malaspina, 2012). The second phase is a delayed and progressive tissue lesion during which macrophages, microglia, T-cells, and neutrophils in the marrow stimulate the inflammatory cytokines release, such as tumor necrosis factor (TNF)α, interleukin (IL)-1α, IL-1β, and IL-6 (Nakamura et al., 2003). Moreover, the mitochondrial dysfunction and the excitatory amino acid receptors’ hyperactivation result in excitotoxicity with neuronal loss due to necrosis and apoptosis (H. Liu et al., 2015). These mechanical and inflammatory damages induce central and peripheral nervous structures significant alterations, resulting in motor, sensory, and autonomous functions impairments below the injury level (Invernizzi, de Sire, Renò, et al., 2020); furthermore, considering the functional, embryological, and biochemical linkages between nerves and muscles, this condition may lead to musculoskeletal sequelae both in the acute and chronic phases (Clark & Findlay, 2017).
In particular, volumetric muscle loss (VML), usually defined as the abnormal decrease of skeletal muscle volume, is associated with variable degrees of functional deficits (Corona et al., 2018). Its most frequent clinical manifestation is represented by sublesional muscle atrophy according to the spine lesion level and severity (Carda et al., 2013; Invernizzi et al., 2015). During the acute phase following SCI, the pro-inflammatory state established results in a rapid series of muscle modifications with consequential muscle catabolism through autophagy supported by mitochondrial mechanisms (Gorgey & Dudley, 2007a; Invernizzi et al., 2021), as depicted in Fig. 1. This could result in a decrease of up to 45% in the thigh muscle cross-section approximately six weeks after the injury (Panisset et al., 2016); this process slowly stabilizes towards the chronic phase, a few months after the injury, with a huge impact on delayed recovery from trauma and functional outcome (Invernizzi, de Sire, Carda, et al., 2020).
Fig. 1
Study flowchart.
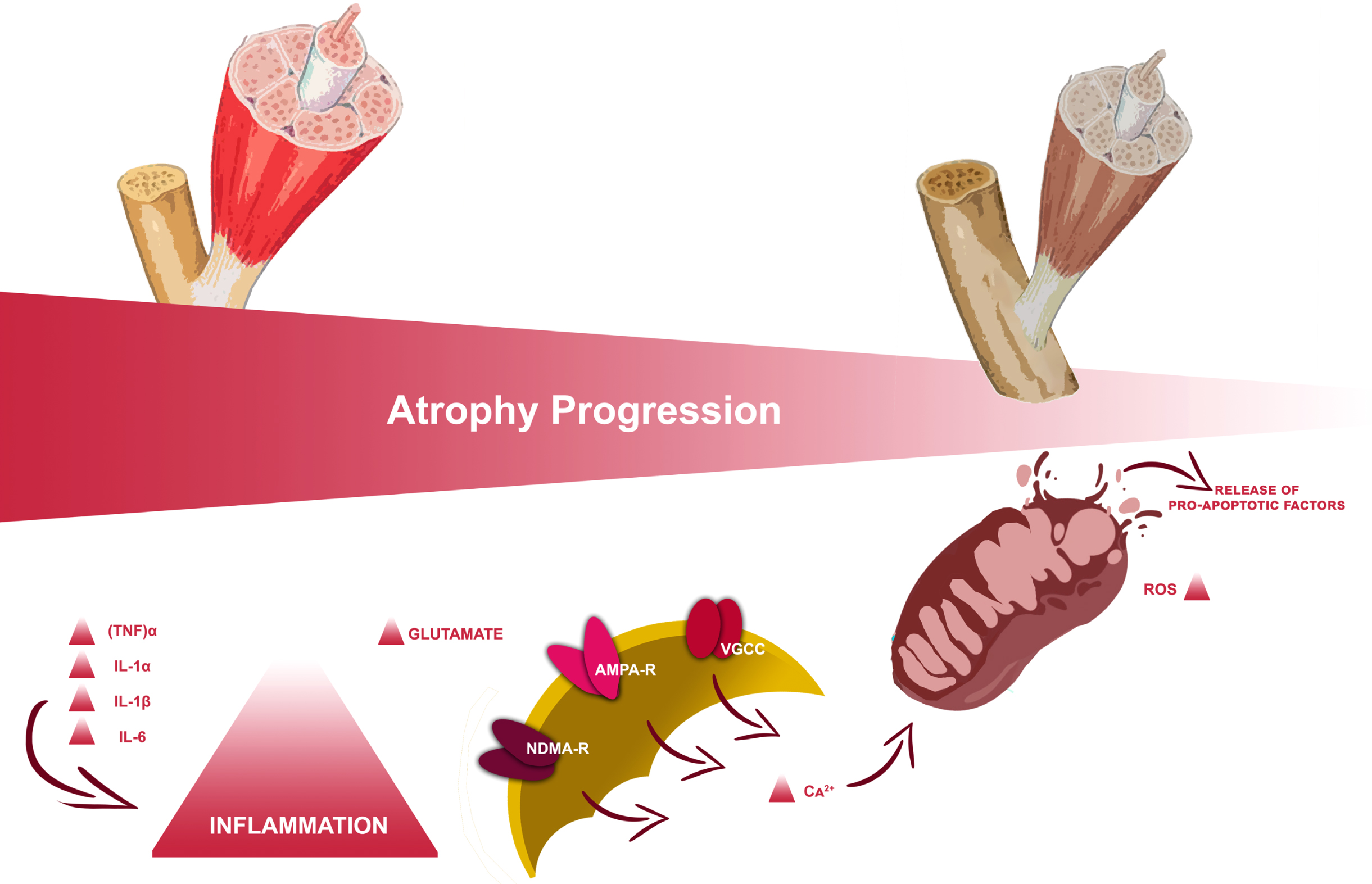
In this context, the early assessment and identification of this condition are essential to improve the overall outcome of patients affected by SCI who develop VML (Downing et al., 2021; Kojouharov et al., 2021). The main strategies proposed in the literature include personalized approaches consisting of dietary supplements (Farkas et al., 2019; Iolascon et al., 2017), drugs (Bauman & Cardozo, 2015; Schopp et al., 2006), and exercise (Beckwée et al., 2019; Durán et al., 2001; Maher et al., 2017; Sandrow-Feinberg & Houlé, 2015; Spangenburg et al., 2008).
A previous study by our group (Invernizzi et al., 2021) provided a portrayal of the state-of-the-art treatment of muscle modifications after SCI, focusing on the connections between the different types of approaches with the main pathophysiological mechanisms underpinning the VLM in subjects affected by SCI. However, the review did not provide clear data on the duration, timing, and effects of the different types of interventions.
Resistance training (RT) might increase muscle mass, however, in person with SCI it might be unfeasible, or need a partial weight lift. Nutritional supplementation is mandatory both to prevent VML and to avoid SCI systemic consequences (Farkas et al., 2019). In particular, essential and branched-chain amino acids, such as leucine (An et al., 2020), creatine, a non-protein nitrogenous tripeptide (Jacobs et al., 2002), vitamin D (Mailhot et al., 2018), irisin, and L-β-aminoisobutyric acid (L-BAIBA) (Colaianni et al., 2017) have been demonstrated to reduce muscle atrophy in population with chronic SCI. In this context, rehabilitation might play a key role in the clinical management of these subjects to improve muscle mass and function (Beckwée et al., 2019).
The inactivity-related VML, as in subjects suffering from SCI, might take advantage of physical exercise, isometric or cycle-ergometry functional electrical stimulation (FES), neuromuscular electrical stimulation (NMES), treadmill step-trained (Stp-T) or stand-trained (Std-T), body weight supported treadmill training (BWSTT), spinal magnetic stimulation, acrobatic exercises, testosterone replacement therapy, exoskeletal body-powered gait orthosis (EBPGO), and epidural spinal cord stimulation (eSCS) (Bustamante et al., 2016; Choi et al., 2020; Durán et al., 2001; Gao et al., 2017; Maher et al., 2017; Marquez-Chin & Popovic, 2020; McHugh et al., 2021; Mehrholz et al., 2017; Nightingale et al., 2018). The physical exercise efficacy seems to be related to its action on skeletal muscle tissue structure and neuroplasticity by the motor cortex activation (Fu et al., 2016; Jurkiewicz et al., 2007), and may result in resistance and cardiopulmonary conditioning improvement due to its neuroprotective and regenerative properties in people with SCI (Durán et al., 2001). FES and NMES are clinically applied as both assistive devices and therapeutic interventions to facilitate functioning restoration through short electrical pulses to generate muscle contractions, resulting in physical health benefits (Bochkezanian et al., 2018; Kapadia et al., 2014). Treadmill training, typically assisted with sensory stimulation, with or without body weight support, may recover some locomotor functions (R. V. Ung et al., 2010; R.-V. Ung et al., 2012). rTMS central nervous system stimulation occurs according to Faraday’s law of electromagnetic induction and seems to improve muscle strength and locomotor function in mice affected by SCI (Ahmed & Wieraszko, 2008). Testosterone Replacement Therapy (TRT) has been identified as an effective strategy for restoring lean body mass and decreasing fat mass in different clinical populations (Bassil et al., 2009), and may be a potential approach to manage body composition changes and improve metabolism in persons with SCI (Nightingale et al., 2018). In individuals with SCI, long-term sitting may result in secondary complications including pressure ulcers, muscle-tendon retractions, and osteoporosis (Karimi, 2011). Thus, the EBPGO training program was effective in preventing VML and in reducing body fat (Choi et al., 2020). eSCS has been identified as able to elicit motor function and to reestablish volitional movement in patients affected by SCI (Megía García et al., 2020) due to its action on some supraspinal control over motor function below the level of injury (Darrow et al., 2019).
To date, there are no clear indications in the literature concerning the type of intervention, timing, and duration to reduce muscle loss in subjects suffering from traumatic spinal cord injury outcomes. Indeed, studies on VML on SCI are often based on animal models, for ethical reasons. Including these papers in the analysis might aid to understand from a different point of view the more effective techniques to restore muscle mass.
Therefore, the present systematic review aims to investigate the role of rehabilitation in the reduction of volume muscle loss, to provide useful data for clinical practice concerning the timing, duration, and treatment parameters in subjects (humans and animals) with traumatic SCI.
2Methods
2.1Search strategy
PubMed, Scopus, and Web of Science databases were systematically searched for articles published from the inception until September 26th, 2022, according to each specific thesaurus, following the strategy described in Table 1. Furthermore, a manual search of the references of previous systematic reviews on a similar topic was conducted as well.
Table 1
Search strategy
PubMed |
(“spinal cord injury”) AND (“sarcopenia” OR “muscle loss” OR “muscle atrophy”) AND (“physiotherapy” OR “physical therapy” OR “rehabilitation” OR “exercise” OR “electrical stimulation”) |
Scopus |
TITLE-ABS-KEY(((“spinal cord injury”) AND (“sarcopenia” OR “muscle loss” OR “muscle atrophy”) AND (“physiotherapy” OR “physical therapy” OR “rehabilitation” OR “exercise” OR “electrical stimulation”))) |
Web of Science |
((“spinal cord injury”) AND (“sarcopenia” OR “muscle loss” OR “muscle atrophy”) AND (“physiotherapy” OR “physical therapy” OR “rehabilitation” OR “exercise” OR “electrical stimulation”)) |
This systematic review was conducted according to the guidance of Preferred Reporting Items for Systematic Reviews and Meta-Analyses (PRISMA). The systematic review protocol was registered in the International Prospective Register of Systematic Reviews (PROSPERO) (number: CRD42022313117).
2.2Eligibility criteria
All observational longitudinal studies were assessed for eligibility according to the following population, intervention, comparison, and outcomes (PICO) model:
P) Participants consisted of humans and animals with a diagnosis of traumatic SCI.
I) Intervention consisted of rehabilitative approaches aimed to reduce volumetric muscle loss (e.g., physical therapy, FES, NMES, body weight supported or not supported treadmill training, spinal magnetic stimulation, weight lift exercises, testosterone replacement therapy, EBPGO, and eSCS).
C) Comparison: no control group, healthy controls, SCI subjects that did not undergo any treatment, individually tailored exercise program, sham electrical stimulation.
O) Outcome measures consisted of total body lean body mass, lower limb lean body mass, cross-sectional area (CSA), fiber type composition, isometric muscle contraction strength, functional gait recovery, such as Basso-Beattie-Bresnahan locomotor rating scale or Irvine, Beattie and Bresnahan Forelimb recovery scale, and muscle thickness and echo intensity.
Two reviewers independently screened all potential articles for eligibility after duplication removal. Any disagreement has been resolved through discussion or, if necessary, by a consultation with a third reviewer.
Only prospective studies were included. Exclusion criteria were: 1) studies involving non traumatic SCI subjects; 2) studies without assessment of muscle atrophy by specific evaluation tools; 2) studies on children (age < 18 years); 3) studies on patients with previous psychological disorders; 4) studies written in a language different from English; 5) full-text unavailability (i.e. posters and conference abstracts).
2.3Data extraction and data synthesis
Data from included studies were extracted by two reviewers independently through a customized data extraction on a Microsoft Excel sheet. In case of disagreement, a third reviewer was asked for a consensus. The following data were extracted: 1) First author; 2) Journal; 3) Publication year; 4) Nationality; 5) Study design; 4) Age of study participants; 5) Sex of study participants; 6) Body mass index (BMI) of study participants; 6) Anamnestic characteristics of study participants; 7) Comorbidities of study participants; 8) Time from the acute event; 9) Neurological level according to ASIA Scale; 10) Extent of SCI defined by ASIA Impairments Scale (AIS); 11) Rehabilitative intervention; 12) Muscle atrophy assessment at the baseline and at the end of treatment (T1); 13) Other clinical functional assessments.
The data have been synthesized by two authors from full-text articles included. Any disagreement between the two reviewers has been solved by collegial discussion among the authors. In case of disagreement, a third author was asked. Text and tables were used to provide a narrative synthesis and explanation of both study characteristics and findings.
Due to the heterogeneity of study participants and intervention assessed, a meta-analysis was not possible in accordance with the Cochrane Handbook for Systematic Reviews of Interventions (version 6.2; Higgins J.P. et al., 2021).
2.4Quality assessment
We evaluated the risk of bias by Joanna Briggs Institute Critical Appraisal Checklist for Quasi-Experimental Studies (non-randomized experimental studies) checklist to estimate the included studies’ methodological quality. Each article was evaluated by two authors separately; a third author was involved to solve any disagreements. The JBI-QES tool consists of nine domains that allow for finding any study bias. Options for each judgment are: 1) low risk of bias, 2) moderate risk of bias/some concerns, 3) serious risk of bias, 4) critical risk of bias, and 5) no information. Domain-level reports providing the basis for an overall risk of-bias judgment.
3Results
3.1Study characteristics
Altogether, 658 studies were identified at the end of the database search. After duplication removal, 407 were considered suitable for the title and abstract screening. As a result, 328 were excluded and 81 articles were screened in full text. Lastly, 24 studies (Adams et al., 2011; Ahmed & Wieraszko, 2008; Baldi et al., 1998; Battistuzzo et al., 2017; Choi et al., 2020; Crameri et al., 2002; de Freitas et al., 2018; Demchak et al., 2005; Ditterline et al., 2020; Dolbow & Credeur, 2018; Everaert et al., 2021; Galea et al., 2017; L. Giangregorio et al., 2012; L. M. Giangregorio et al., 2005, 2006; Gorgey & Dudley, 2007a; Holman & Gorgey, 2019; Karelis et al., 2017; M. Liu et al., 2008; Roy et al., 1998; Singh et al., 2011; Skiba et al., 2021; Sutor et al., 2022; R. V. Ung et al., 2010) were included in this systematic review, as illustrated by the PRISMA flowchart in Fig. 2. The included studies (Adams et al., 2011; Ahmed & Wieraszko, 2008; Baldi et al., 1998; Battistuzzo et al., 2017; Choi et al., 2020; Crameri et al., 2002; de Freitas et al., 2018; Demchak et al., 2005; Ditterline et al., 2020; Dolbow & Credeur, 2018; Everaert et al., 2021; Galea et al., 2017; L. Giangregorio et al., 2012; L. M. Giangregorio et al., 2005, 2006; Gorgey & Dudley, 2007a; Holman & Gorgey, 2019; Karelis et al., 2017; M. Liu et al., 2008; Roy et al., 1998; Singh et al., 2011; Skiba et al., 2021; Sutor et al., 2022; R. V. Ung et al., 2010) have been published from 1998 to 2022. Twelve (50%) were conducted in the USA (Adams et al., 2011; Ahmed & Wieraszko, 2008; Baldi et al., 1998; Demchak et al., 2005; Ditterline et al., 2020; Dolbow & Credeur, 2018; Gorgey & Shepherd, 2010; Holman & Gorgey, 2019; M. Liu et al., 2008; Roy et al., 1998; Singh et al., 2011; Sutor et al., 2022), two in Brazil (8,3%) (de Freitas et al., 2018; Skiba et al., 2021), six in Canada (25%) (Everaert et al., 2021; L. Giangregorio et al., 2012; L. M. Giangregorio et al., 2005, 2006; Karelis et al., 2017; R. V. Ung et al., 2010), two in Australia (8.3%) (Battistuzzo et al., 2017; Galea et al., 2017), one in Denmark (4.16%) (Crameri et al., 2002), and one in Korea (4.16%) (Choi et al., 2020).
Fig. 2
Volume muscle loss’ progression in subjects affected by spinal cord injury.
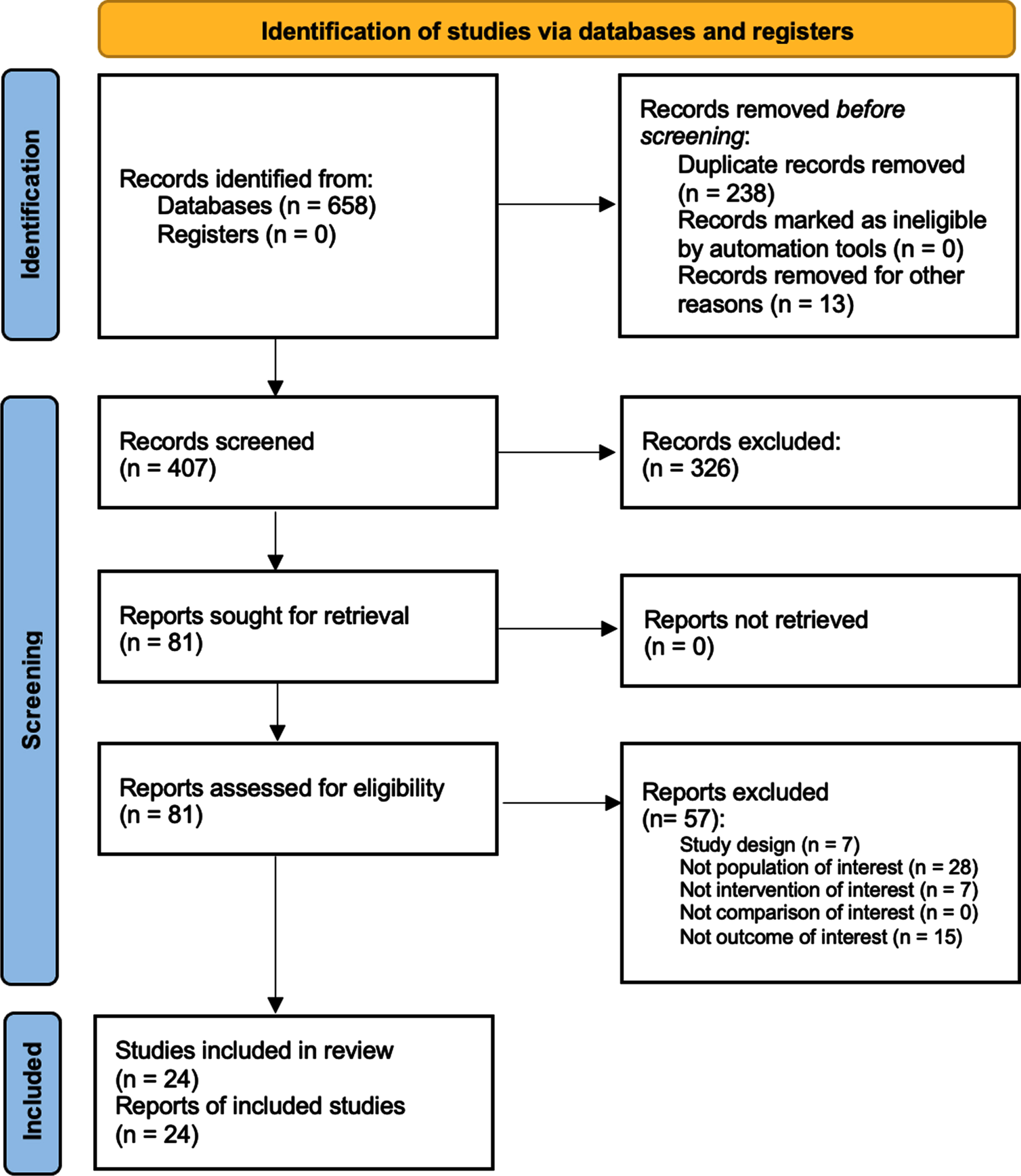
A total of 370 subjects were analyzed, of whom 203 were adult humans (Adams et al., 2011; Baldi et al., 1998; Choi et al., 2020; Crameri et al., 2002; Demchak et al., 2005; Ditterline et al., 2020; Dolbow & Credeur, 2018; Everaert et al., 2021; Galea et al., 2017; L. Giangregorio et al., 2012; L. M. Giangregorio et al., 2005, 2006; Gorgey & Shepherd, 2010; Holman & Gorgey, 2019; Karelis et al., 2017; Skiba et al., 2021; Sutor et al., 2022), 20 Mongrel cats (Roy et al., 1998), and 177 mice (13 CD1 female rats (Ahmed & Wieraszko, 2008), 22 CD1 male rats (R. V. Ung et al., 2010), 72 Sprague-Dawley female rats (M. Liu et al., 2008; Singh et al., 2011), 49 C57Bl/6 male rats (Battistuzzo et al., 2017), and 21 Wistar female rats (de Freitas et al., 2018)). Study cohorts ranged from 1 (Dolbow & Credeur, 2018; Gorgey & Shepherd, 2010) to 49 subjects (human and animals) (Battistuzzo et al., 2017). About intervention, twelve (Adams et al., 2011; Baldi et al., 1998; Crameri et al., 2002; de Freitas et al., 2018; Demchak et al., 2005; Dolbow & Credeur, 2018; Everaert et al., 2021; Galea et al., 2017; L. Giangregorio et al., 2012; Gorgey & Shepherd, 2010; Holman & Gorgey, 2019; Skiba et al., 2021) studies provided several types of electrical stimulation, seven administered body weight supported or not supported treadmill training (Battistuzzo et al., 2017; L. M. Giangregorio et al., 2005, 2006; M. Liu et al., 2008; Roy et al., 1998; Singh et al., 2011; R. V. Ung et al., 2010), and five performed other approaches (Ahmed & Wieraszko, 2008; Choi et al., 2020; Ditterline et al., 2020; Karelis et al., 2017; Sutor et al., 2022). Concerning the outcome measures, most of the studies evaluated the lower-limb muscles’ cross-sectional area (CSA) (Adams et al., 2011; Ahmed & Wieraszko, 2008; Battistuzzo et al., 2017; Crameri et al., 2002; Demchak et al., 2005; Everaert et al., 2021; Galea et al., 2017; L. Giangregorio et al., 2012; L. M. Giangregorio et al., 2005, 2006; Gorgey & Shepherd, 2010; Holman & Gorgey, 2019; M. Liu et al., 2008; R. V. Ung et al., 2010) and the muscle function (Ahmed & Wieraszko, 2008; Crameri et al., 2002; Demchak et al., 2005; Ditterline et al., 2020; Everaert et al., 2021; Holman & Gorgey, 2019; R. V. Ung et al., 2010), while nine studies (Ahmed & Wieraszko, 2008; Baldi et al., 1998; Choi et al., 2020; Ditterline et al., 2020; Galea et al., 2017; L. Giangregorio et al., 2012; Karelis et al., 2017; Singh et al., 2011; Sutor et al., 2022) analyzed the muscle features. The main characteristics of the studies included in this systematic review are summarized in Table 2.
Table 2
Main characteristics and findings of the included studies
Authors | Journal | Type of population | Type of lesion | Intervention | Control | Outcomes | Main findings |
Baldi JC et al. 1998, USA [62] | Spinal Cord | Adult humans (n = 26) | Traumatic complete thoracic or cervical SCI (lesser than 3 months post-injury) | 1. Isometric FES contractions (FES-IC), 60 min/day, 5 days/week; 2. FES-cycle ergometry (FES-CE), 30 min/day, 3 days/week | No FES training (n = 9) | TB-LBM, LL-LBM, and G-LBM | Subjects in the FES-IC group consistently lost less lean body mass than controls, however, only 6-month G-LBM loss was significantly attenuated in this group relative to the controls. FES-CE significantly increased G-LBM and LL-LBM after 6 months of training relative to pre-training levels. |
Roy RR et al. 1998, USA [76] | Muscle &Nerve | Adult mongrel cats (n = 20) | Complete transection at the level of T12–13 (1 month posttransection) | Step-trained on a treadmill (Stp-T) or stand-trained (Std-T), 30 min/day, 5 days/week for 5 months | No step or stand training (n = 8) | Soleus architectural features, mechanical properties, fiber type and size properties, and MHC and biochemical properties | Training ameliorated soleus atrophy and enhanced maximum force capability compared to nontrained spinal cats, with Stp-T being. Thus, rhythmical activity involving muscle length and force changes (stepping) was more effective than a similar amount of a more static activity (standing), and significantly different from nontrained. |
Crameri RM et al. 2002, Denmark [64] | Scandinavian Journal of Medicine and Science in Sport | Adult humans (n = 6) | Traumatic complete SCI at T4-T12 (at least 8 years post-injury) | FES cycle ergometry leg training, 30 min/day, 3 days/week for 10 weeks | No control group | Muscle function, fiber CSA, fiber type composition, myosine heavy chain analysis, capillarization, citrate synthase and hexokinase activity | The paralyzed vastus lateralis muscle showed significant alterations in skeletal muscle characteristics after training, indicated by an improvement in total work output, and an increase in fiber CSA. |
Demchak TJ et al. 2005, USA [66] | Journal of Sports Science and Medicine | Adult humans (n = 10) | Traumatic complete cervical or thoracic SCI (4–6 weeks post-injury) | FES cycle ergometry leg training (FES-CE), 30 min/days, 3 days/week for 13 weeks | No FES training (n = 5) and healthy controls (n = 5) | CSA, average muscle power output, and MHC composition | In the FES-CE trained group, power output increased from 2.4±.88 Watts to 24.5±3.2 Watts. Following 13 weeks of FES-CE training, CSA increase was 63% greater when compared to the IC group. |
Giangregorio LM et al. 2005, Canada [72] | Spinal Cord | Adult humans (n = 5) | Traumatic SCI (2–6 months post-injury) | BWSTT, 5–15 min/days, 2 days/week for 12 weeks | No control group | CSA | BWSTT increased the walking speed and the walking duration. The average increase in lean mass was of 3.8%, whereas the average increase in fat mass was 31%. All participants experienced an increase in thigh and calf muscle and fat CSA after BWSTT. |
Giangregorio LM et al. 2006, Canada [73] | Applied Physiology, Nutrition, and Metabolism | Adult humans (n = 14) | Traumatic incomplete SCI (AIS C) (at least 12 months post-injury) | BWSTT, 5–15 min/days, 3 days/week for 12 weeks. | No control group | CSA | Participants experienced significant increases in whole-body lean mass, from 45.9±8.7 kg to 47.8±8.9 kg (mean±SD; p < 0.003). Muscle CSA increased by an average of 4.9% and 8.2% at the thigh and lower leg sites, respectively. |
Ahmed Z and Wierasko A 2008, USA [55] | Journal of Neurotrauma | CD-1 35-day-old female mice (n = 13) | Incomplete spinal cord transection (2 days after transection) | 1. Spinal magnetic stimulation followed by acrobatic exercises; 2. Acrobatic exercise only; 3. Magnetic stimulation only. MS ad acrobatic exercises were administered daily for 24 days. | Injured control, with neither acrobatic exercise nor MS (n = 3); healthy subjects (n = 3). | CSA of individual muscle fibers, and void area of muscle tissue, isometric muscle contraction, BMS, horizontal-ladder test | CSA of the muscle was reduced (to approximately 30% of control) in controls. While the muscles from exercising and magnetic field exposed animals had the area reduced approximately by half, the muscles from exercising/exposed animals were practically not changed. |
Gorgey AS and Sheperd C 2010, USA [81] | The Journal of Spinal Cord Medicine | Adult human (n = 1) | Traumatic complete cervical SCI (5 years prior to the study) | Surface NMES and ankle weights exercises, 2 days/week for 12 weeks. | No control group | Whole thigh, knee extensor, hamstrings, hip adductors, sartorius, and gracilis skeletal muscle CSA | Skeletal muscle hypertrophy was detected across multiaxial slices in both knee extensor and adjacent muscle groups. The ratio of subcutaneous fat CSA to whole thigh skeletal muscle decreased, from 0.85±0.2 to 0.81±0.2; this suggests that skeletal muscle CSA increased at a greater rate when compared to subcutaneous fat after training. |
Liu M et al. 2008, USA [75] | Spinal Cord | Sprague–Dawley female rats (n = 24) | Moderate midthoracic contusion SCI (8 days after transection) | 1. Treadmill training; 2. Cycle training. Both protocols were administered 20 min/day, 2 times/day, 5 days/week for 3 months. | No treadmill training | CSA of hindlimbs muscles | Significant atrophy in all rat hindlimb muscles with the posterior muscles (triceps surae and flexordigitorum) were observed, showing greater atrophy than the anterior muscles (tibialis anterior and extensor digitorum). The greatest amount of atrophy was measured at 2-week post injury (range from 11 to 26%), and spontaneous recovery in muscle size was observed by 4 weeks post-SCI. |
Ung RV et al. 2010, Canada [54] | Spinal Cord | Male CD1 mice (n = 22) | Complete low-thoracic (T9-T10) spinal transection (3 days after transection) | Motorized treadmill training, 15 min/days, 5 days/week for 5 weeks. | No treadmill training (n = 11) | Individual fiber CSA, locomotor performances, and muscle fiber type conversion | body weight, adipose tissue, whole muscle, and individual fiber CSA values were significantly lower in trained compared with untrained animals. In contrast, no significant difference in locomotor performances or in muscle fiber type conversion was found between trained and untrained mice. |
Adams CM et al. 2011, USA [61] | Muscle Nerve | Adult humans (n = 2) | Traumatic T4 complete paraplegia (at least 6 years before) | Electrical stimulation of right soleus, 30 min/day, 5 days/week for > 6 years. | No control group | Plantar flexor CSA | The untrained left soleus muscles possessed the atrophy and fast twitch properties characteristic of chronic SCI. |
Singh A et al. 2011, USA [77] | Journal of Neurotrauma | Female Sprague-Dawley rats (n = 48) | Thoracic contusion injury or a thoracic transection, or a contusion followed at 9 weeks by a second transection injury | BWSTT for 500 steps/day, 5 days/week for 8 weeks. Participants were divided into 6 groups: 1. Contusion injury with 8 weeks of BWSTT; 2. Contusion injury with no BWSTT; 3. Contusion injury with 8 weeks of BWSTT followed by a transection injury at week 9; 4. Contusion injury with no BWSTT followed by a transection injury at week 9; 5. Transection injury with 8 weeks of BWSTT; 6. Transection injury with no BWSTT. | No BWSTT (n = 4) | Soleus muscle weight, Basso-Beattie-Bresnahan locomotor rating scale, kinematic analysis | A protective effect of BWSTT on the soleus muscle weight was observed in trained animals with contusion or transection or both injuries with a significantly higher wet muscle weight:body weight ratio in trained than untrained animals with similar injuries (p < 0.01by ANOVA). Additionally, the soleus muscle mass of trained contused animals (group 2) and unoperated controls (group 1) did not differ from each other. |
Giangregorio LM et al. 2012, Canada [71] | The Journal of Spinal Cord Medicine | Adult humans (n = 27) | Traumatic incomplete cervical or thoracic SCI (at least 18 months prior to study) | FES stimulation while ambulating on a body weight support treadmill and harness system, 45 min/day, 3 days/week for 4 months. | Individually tailored exercise program consisting of 20–25 minutes of resistance training (using hand weights, cables, and uppertone) and 20–25 minutes of aerobic training (arm cycling, leg cycling, and walking in parallel bars or on treadmill), 45 min/day, 3 days/week for 4 months (n = 17). | Total body fat-free mass, leg lean mass, and calf muscle CSA, and fat mass (total body fat mass and calf fat CSA) | FES-assisted walking had no significant effect on lower-limb muscle CSA; there was no significant change over time and there were no differences between the groups. For total body lean mass, leg lean mass and total body fat mass changes over time were not significant in intention-to-treat analyses. |
Battistuzzo CR et al. 2016, Australia [63] | Muscle Nerve | Male C57BL/6 mice (n = 49) | Complete low-thoracic (T10) spinal transection (1 week after transection) | Treadmill training 10 m/min for 10 min, twice per day, 5 days/week for 3, 6, or 9 weeks. | No treadmill training | CSA and muscle fiber type composition of medial gastrocnemius, soleus, and tibialis anterior | Fiber CSA from the affected and non-affected hindlimbs of trained SCI animals differed with the training period, muscle groups, and fiber types. The results from the comparisons of muscle fiber type composition between the affected and non-affected sides (internal control) of trained animals also did not show a statistically significant difference in any muscle. |
de Freitas GR et al. 2017, Brazil [65] | Physical Therapy | Female Wistar rats (n = 21) | Cervical (C3-C7) spinal hemisection (3 days after surgery) | Brachii biceps NMES, 10 min/day, 5 days/week for 5 weeks. Rats were randomly divided into 3 groups: 1. Control (sham); 2. SCI; 3. SCI plus NMES. | Sham (n = 8) or no NMES (n = 7) | Biceps muscle tropism, Irvine, Beattie and Bresnahan Forelimb Recovery Scale, grip test, and analysis of the phosphorylated Akt, p70S6K and GSK-3ß cellular anabolic pathway markers | The animals that underwent cyclical-NMES had higher muscle tropism values when compared to the SCI group (0.061±0.005 g and 0.051±0.006 g, p = 0.005, respectively). Furthermore, the NMES group showed no difference in muscle tropism values when compared to the sham group (0.06±0.005 g and 0.06±0.005 g, p = 0.99, respectively). It’s noteworthy to say that there is no difference between body weight of the of the animals in experimental groups (SCI and NMES, p > 0.05). |
Galea MP et al. 2017, Australia [70] | Neurorehabilitation and Neural Repair | Adult humans (n = 24) | Traumatic complete or incomplete SCI above the neurological level of T12 (at least 4 weeks post-injury) | FES cycle ergometry leg training, 60 min, 4 days/week for 12 weeks. | Personalized physiotherapy provided by registered physiotherapists, as appropriate for the stage and according to the participants’ impairments and goals for 12 weeks (n = 12). | CSA of the thigh and calf, girth measurements of the chest and waist, appendicular lean mass, assessment of Quality of Life 8, Health Utilities Index 3, Hospital Anxiety and Depression Scale, and World Health Organisation Quality of Life-Australian Version 2000 | No statistically significant between-group differences were found for the primary outcome measures (mean maximum CSA of thigh and calf), nor for any other tissue, anthropometric, or behavioral parameter. |
Karelis AD et al. 2017, Canada [80] | Journal of Rehabilitation Medicine | Adult humans (n = 5) | Non-progressive traumatic complete sensorimotor spinal cord injury, using a wheelchair as a primary mode of mobility (7 years post-injury) | Personalized 6-week progressive locomotor training program using a robotic exoskeleton 3 times/week for up to 60 min. | No control group | Total body weight, percentage fat mass (FM) and lean body mass (LBM in kg) of the arms, legs and trunk | A significant increase in CSA, leg and appendicular lean body mass and a decrease in total, leg and appendicular fat mass were observed after the intervention. |
Dolbow DR and Credeur DP 2018, USA [68] | The Journal of Spinal Cord Medicine | Adult humans (n = 1) | Traumatic complete thoracic (T10) SCI (11 years post-injury) | Resistance-guided, high intensity interval training FES cycling program, 30 min/day, 3days/week for 10 weeks. | No control group | Measures of body composition and cardio-metabolic health (vascular endothelial function of the brachial artery via flow mediated dilation) and HbA1c blood values | TB-LBM and LL-LBM increased by 2.8% and 5.3% respectively while vastus lateralis thickness increased by 59.5%. |
Holman ME and Gorgey AS 2019, USA [74] | Medicine &Science in Sports &Exercise | Adult humans (n = 22) | Traumatic complete cervical or thoracic (C5-L2) SCI (at least 1-year postinjury) | 1. Testosterone replacement therapy; 2. NMES resistance training along with testosterone replacement therapy. Both interventions were administered for 16 weeks. | No control group | CSA and isokinetic dynamometry and knee extensor of both lower extremities | Main effects between testosterone replacement therapy + NMES and testosterone replacement therapy were identified as not significant, F(1, 17.98) = 2.72, P = 0.12, η2partial = 0.13, while time was found to be significant, F(1, 18.10) = 29.95, P < 0.0001, η2partial = 0.62. A significant interaction was also observed between the conditions and time, F(1, 18.10) = 9.36, P = 0.007, η2partial = 0.34. |
Choi HJ et al. 2020, Korea [44] | Journal of Multidisciplinary Healthcare | Adult humans (n = 11) | Traumatic chronic complete or incomplete thoracic SCI (more than 2 years before the study) | EBPGO, 120 min/days, for 2 or 3 days/week for 52 weeks. | No EBPGO (n = 5). | Lean body mass, soft muscle mass, fat-free mass, fat mass, and percentage body fat | The whole-body lean body mass of the intervention group was maintained, but the whole-body lean body mass of the non-exercise group was significantly reduced by –6.4% after 52-weeks (p = 0.022). In the non-exercise group, the reduction rate of segmental lean body mass was higher in the upper limb (31%) than in the lower limb (8.6%). |
Ditterline B et al. 2020, USA [67] | Experimental Physiology | Adult humans (n = 6) | Traumatic chronic complete or incomplete cervical SCI (from 3 to 10 years before the study) | scES, 2 hours/day in 4 individuals and 5 hours/day in 2 individuals, 7 days/week for 6 months) and NMES of knee extensors, knee flexors, and ankle plantar flexors of the left and right leg. | No control group | Whole-body percent lean mass and fat mass, and lower limb (regional) lean mass, muscle strength and fatigability | Spinal cord epidural stimulation significantly increased (0.67±0.39 kg, or 9.4±8.1%; p < 0.001) lower limb lean mass. On the other hand, whole-body mass and composition did not change significantly. Additionally, muscle strength and fatigability data elicited by NMES demonstrated a general increase (57±117%) in maximum torque output (between 2 Nm and 44 Nm in 14 of the 17 muscle groups tested overall) and torque time integral during intermittent, fatiguing contractions (63±71%; between 7% and 230% in 16 of the 17 muscle groups tested overall). |
Everaert DG et al. 2021, Canada [69] | The Journal of Spinal Cord Medicine | Adult humans (n = 3) | Traumatic acute complete or incomplete cervical or thoracic SCI (25 days after injury) | FES cycle ergometry leg training, supine or sitting, 15–45 min/day, 3 days/week for 8–13 weeks. | No control group | Muscle CSA in thighs and calves cycling performance | Initially, muscle CSA decreased in all participants (up to 16% after 6 weeks) and recovered later after a variable period of FES cycling (up to 16% at 13.3 weeks). |
Skiba et al. 2021, Brazil [78] | Neurological Sciences | Adult humans (n = 32) | Complete SCI (from 1 to 26 years before the study) | Low-intensity FES with blood flow restriction, 2 days/week for 8 weeks. | Low-intensity FES, 2 days/week for 8 weeks (n = 14). | Muscle thickness and echo intensity | The effects of exercise on muscle thickness immediately before and 5 min after the end of the exercises indicated acute increases of 19.7% in the FES + blood flow restriction (p < 0.05) and 7.6% in the FES (p < 0.05). The muscle thickness increases in the FES + blood flow restriction was greater than in the FES (U = 29, p < 0.05), r = 0.65. There was a chronic increase in the muscle thickness in the FES + blood flow restriction, especially between PRE and INT (p < 0.05; r = 0.61) and PREPOS (p < 0.05; r = 0.60). |
Sutor TW et al., 2022 [79] | Frontiers in Rehabilitation Sciences | Adult humans (n = 8) | Chronic SCI (at least 1 year post- injury) | Exoskeleton assisted-walking program 2-3 times per week for 12 weeks | No control group | Lean mass and total mass for the total body, legs, and trunk. | Percent fat was reduced from baseline for the total body (–1.4%, P = 0.018), leg (–1.3%, P = 0.018), and trunk (–2%, P = 0.036) regions. |
Legend: AIS = ASIA impairments scale; BMS = Basso mouse scale; BWSTT = body weight supported treadmill training; CSA = cross-sectional area; EBPGO = exoskeletal body-powered gait orthosis; FES = functional electrical stimulation; FI = fatigue index; G-LBM = gluteal lean body mass; LL-LBM = lower limb lean body mass; MHC = major histocompatibility complex; NMES = neuromuscular electrical stimulation; scES = spinal cord epidural stimulation; SCI = spinal cord injury; TB-LBM = Total body lean body mass.
3.2Studies on animal model
3.2.1Electrical stimulation
de Freitas and colleagues (de Freitas et al., 2018) analyzed the effects of biceps brachii NMES on muscle function, tropism, and the Akt pathway signaling involved in muscular plasticity in rats after C5-C7 level surgical induced hemisection, observing an increase in bicep muscle strength in the NMES group when compared with the untreated SCI group.
3.2.2Treadmill on sub-acute phase SCI
In 1998, Roy et al. (1998) trained twenty adult cats to determine the role of short periods of daily stepping on a treadmill (rhythmic activity) or standing (continuous activity) in ameliorating the adaptations in the muscle properties, demonstrating that training ameliorated soleus atrophy and enhanced maximum force capability.
Liu et al. (2008) concluded that both cycling and treadmill training on rats paused the atrophic process and improved the recovery rate, with a positive correlation between locomotor functional scores and hindlimb muscle size following SCI.
Ung et al. (2012), through experiments in a mouse model of complete paraplegia which underwent non-assisted treadmill training, stated that body weight, adipose tissue, whole muscle, and individual fiber CSA values were significantly lower in trained compared with untrained animals.
Singh and colleagues (2011) found that BWSTT-contused animals showed accelerated locomotor recovery and reduced muscle atrophy.
Battistuzzo et al. (2017) also demonstrated that 9 weeks of training after incomplete SCI was effective in preventing fast-twitch muscles atrophy, but there were limited effects on slow-twitch muscles and muscle fiber type composition.
3.2.3Spinal magnetic stimulation on sub-acute phase SCI)
Ahmed and Wierasko (2008) provided preliminary data about the efficacy in the maintenance of CSA in epidural spinal cord magnetic stimulation and acrobatic exercise on exercising/exposed animals.
3.3Studies on humans
3.3.1Electrical stimulation
3.3.1.1. Sub-acute phase SCI. Baldi et al. (1998) and Demchak et al. (2005) determined that FES cycle ergometry (FES-CE) or non-loaded FES isometric contractions (FES-IC) could prevent disuse muscle atrophy after SCI, with a less loss of lean body mass and CSA than controls.
In 2017, the Switch-On study (Galea et al., 2017), examined the efficacy, safety, feasibility, and tolerability of FES-assisted cycling (FESC) and passive cycling 4-week post-traumatic complete or incomplete SCI, stating that no significant between-group difference in post-intervention muscle CSA was found. Furthermore, Everaert et al. (2021) reported that muscle CSA decreased and recovered after a variable period of FES cycling, concluding that changes in muscle CSA did not always correspond with the dose of FES cycling.
3.3.1.2. Humans (chronic phase SCI). In Crameri and colleagues’ study (2002), the paralyzed vastus lateralis muscle showed significant alterations in skeletal muscle characteristics after FES cycle ergometry leg training.
Four studies (Adams et al., 2011; Dolbow & Credeur, 2018; L. Giangregorio et al., 2012; Gorgey & Shepherd, 2010) demonstrated that, after the electrical stimulation training, the average skeletal muscle CSA increased and torque, fatigue index, contractile speed, and cross-sectional area were preserved.
In 2019, Holman and Gorgey (2019) concluded that 16 weeks of combined TRT and NMES-RT improved muscle quality in men with motor-complete SCI.
Skiba and colleagues (2021), identified a chronic increase in muscle thickness in the subjects treated with FES + blood flow restriction (BFR) in thirty-two adult humans affected by complete SCI.
3.3.2Treadmill training
3.3.2.1 Sub-acute phase SCI. In 2005, Giangregorio and colleagues (2005) demonstrated an increased muscle CSAs and a small reduction in lower limb bone mineral density (BMD), after BWSTT training in the sub-acute phase of SCI, and, in 2006 (L. M. Giangregorio et al., 2006), the same results were obtained in subjects at least 12 months after injury.
3.3.3Exoskeletal body-powered gait orthosis or exoskeleton-assisted walking
3.3.3.1. Humans (chronic phase SCI). Karelis et al. (2017), after a personalized locomotor training program using a robotic exoskeleton, found a significant increase in CSA, leg and appendicular lean body mass, while Choi et al. (2020) did not identify significant changes in weight, fat-free mass, lean body mass, and percent fat mass in the exercise group.
Sutor and colleagues (Sutor et al., 2022) performed an exploratory study to investigate the efficacy of exoskeleton training and trans-spinal stimulation in 8 subjects after spinal cord injury (EXTra-SCI), concluding that percent fat was reduced.
3.3.4Spinal cord epidural stimulation
3.3.4.1. Humans (chronic phase SCI). Ditterline and colleagues (Ditterline et al., 2020) stated that implanted eSCS significantly increased LL-LBM, muscle strength, and fatigability.
3.4Study quality
To evaluate the quality of evidence of this systematic review, we adopted the Joanna Briggs Institute Critical Appraisal Checklist for Quasi-Experimental Studies (non-randomized experimental studies). As depicted in Table 3, we assessed the 9-question risk-of-bias domains. All the included articles presented full-text availability. Most of the studies (n = 19; 79.16%) were judged with at least one serious risk of bias, which translated into an overall serious risk of bias for that study. Fourteen (58.33%) included studies reported a lack of data on follow-up outcomes.
Table 3
Joanna briggs institute critical appraisal checklist for quasi-experimental studies (non-randomized experimental studies).
Author and year | Q1 | Q2 | Q3 | Q4 | Q5 | Q6 | Q7 | Q8 | Q9 |
Baldi 1998 [61] | Y | Y | Y | Y | Y | Y | Y | Y | Y |
Roy 1998 [75] | Y | Y | Y | Y | Y | N | Y | Y | N/A |
Crameri 2002 [63] | Y | Y | Y | N | Y | Y | Y | Y | N/A |
Demchak 2005 [65] | Y | Y | Y | Y | Y | Y | Y | Y | Y |
Giangregorio 2005 [71] | Y | Y | Y | N | Y | Y | Y | Y | N/A |
Giangregorio 2006 [72] | Y | Y | Y | N | Y | Y | Y | Y | N/A |
Ahmed and Wierasko 2008 [55] | Y | Y | Y | Y | Y | N | Y | Y | N/A |
Gorgey and Sheperd 2008 [78] | N/A | Y | Y | N | Y | Y | Y | Y | N/A |
Liu 2008 [74] | Y | Y | Y | Y | Y | N | Y | Y | N/A |
Ung 2010 [53] | Y | Y | Y | Y | Y | N | Y | Y | N/A |
Adams 2011 [60] | Y | Y | Y | N | Y | Y | Y | Y | N/A |
Singh 2011 [76] | Y | Y | Y | Y | Y | Y | Y | Y | Y |
Giangregorio 2012 [70] | Y | Y | Y | Y | Y | Y | Y | Y | Y |
Battistuzzo 2016 [62] | Y | Y | Y | Y | Y | N | Y | Y | N/A |
de Freitas 2017 [64] | Y | Y | Y | Y | Y | N | Y | Y | N/A |
Galea 2017 [69] | Y | Y | Y | Y | Y | N | Y | Y | N/A |
Karelis 2017 [79] | Y | Y | Y | N | Y | N | Y | Y | Y |
Dolbow and Credeur 2018 [67] | N/A | Y | Y | N | Y | N | Y | Y | N/A |
Holman and Gorgey 2019 [73] | Y | Y | Y | N | Y | N | Y | Y | N/A |
Choi 2020 [44] | Y | Y | Y | Y | Y | N | Y | Y | Y |
Ditterline 2020 [66] | Y | Y | Y | N | Y | N | Y | Y | N/A |
Everaert 2021 [68] | Y | Y | Y | N | Y | N | Y | Y | N/A |
Skiba 2021 [77] | Y | Y | Y | Y | Y | Y | Y | Y | Y |
Sutor 2022 [78] | Y | Y | Y | N | Y | N | Y | Y | Y |
Legend: Q1 = Is it clear in the study what is the ‘cause’ and what is the ‘effect’ (i.e. there is no confusion about which variable comes first)?; Q2 = Were the participants included in any comparisons similar?; Q3 = Were the participants included in any comparisons receiving similar treatment/care, other than the exposure or intervention of interest?; Q4 = Was there a control group?; Q5 = Were there multiple measurements of the outcome both pre and post the intervention/exposure?; Q6 = Was follow up complete and if not, were differences between groups in terms of their follow up adequately described and analyzed?; Q7 = Were the outcomes of participants included in any comparisons measured in the same way?; Q8 = Were outcomes measured in a reliable way?; Q9 = Was appropriate statistical analysis used?; N = no, Y = yes; N/A = not applicable.
4Discussion
The present systematic review describes the state-of-art of rehabilitation effects on volume muscle loss in subjects (humans and animals) with SCI. Our results underlined that electrical stimulation, whether functional or neuromuscular and exercise on a treadmill with or without load relief seem to be effective in the VML reduction in subjects suffering from SCI. Moreover, we highlighted that most of the studies in the literature presented a relevant risk of bias, as more rigorous trials are needed to conclude on best practices. As previously stated, VML in individuals with SCI may significantly impair the patient’s functional independence. Due to this, it is mandatory to monitor changes in body composition over time, detecting early muscle volume reduction and the most effective type of intervention, also considering the social and health implications of this condition. Several authors evaluated the effects of different rehabilitative approaches in VML management, without providing reliable data about the optimal treatment parameters and the intervention timing. The mechanisms of VML, which occurs for 40–60% in the first two weeks after SCI, are not fully understood but seem primarily supported by an upregulation of the proteolytic degradation of muscle proteins, such as muscle-restricted E3 ubiquitin ligases muscle atrophy F-box (MAFBx, atrogin-1) and muscle ring finger-1 (MuRF1, Trim63) (Gorgey et al., 2019; Qin et al., 2010). Both functional and neuromuscular electrical stimulation is the most commonly used approaches in addition to conventional rehabilitation treatment. In 2020, Zhao et al. (2021) evaluated the efficacy of surface or implantable ES to elicit muscle contraction and prevent muscle atrophy. In this study, the authors found a significant reduction of the mass of soleus, plantaris and extensor digitorum longus muscles in a rat model 6 weeks post-SCI treated with prolonged electrical stimulation and /or testosterone enanthate compared to sham-transected animals. Zhang and colleagues (Zhang et al., 2017) examined the effects of tail nerve electrical stimulation (TANES) 5 times/week and electroacupuncture (EA) 1 time/week on lumbar motor neurons and hindlimb muscle in spinal transected rats. Both TANES and EA showed a significant impact in ameliorating the atrophy of hindlimb muscle after SCI four weeks after the beginning of treatment, suggesting that these approaches seem to protect the motor neurons as well as alleviate muscle atrophy. An experimental prospective study with four parallel treatment groups (Butezloff et al., 2015) analyzed the skeletal muscle changes after a complete SCI in rats and aimed to compare the ES and whole-body vibration’s effects on the prevention of muscle hypotrophy in these animals. After 30 days of treatment with ES (3 days/week, 20 mins per day) the Authors obtained a significant partial muscle hypotrophy prevention. As stated before, insightful changes in skeletal muscle size and strength might occur after injury, often in conjunction with increased intramuscular fat (Elder et al., 2004; Gorgey & Dudley, 2007b). This condition is also associated with increased muscle fatigue in response to the ES, as well as the reduced oxidative capacity of the skeletal muscle (Mahoney et al., 2007; Olive et al., 2003). Erickson et al. (2017) demonstrated that the endurance training elicited with NMES could reverse these changes and result in muscle metabolic health improvement. They recruited 14 subjects affected by SCI undergoing a 16-week home-based endurance NMES training of knee extensors muscles; after this rehabilitation protocol, an increased number of contractions (performed throughout training) was observed, with a significant improvement in skeletal muscle oxidative capacity of 119% (p = 0.019).
As underlined by the results of our systematic review, treadmill training with or without weight relief also appears to significantly reduce VML. More in detail, incomplete SCI animal models, and quadrupedal bodyweight-supported treadmill training could reduce muscle atrophy and improve muscle force production and recovery of voluntary locomotion (M. Liu et al., 2008, 2010; Stevens et al., 2006); these results might be obtained through better afferent neuromodulation, the prevention of axonal degradation, and the normalization of the spinal reflex pathways that regulate spasticity and motoneuron excitability (Hou et al., 2014). Moreover, several systemic hormonal irregularities occur secondary to SCI and may worsen musculoskeletal deficits, such as low serum testosterone (Qin et al., 2010). Yarrow et al. (2020) concluded that short-term bodyweight-supported treadmill training alone did not improve bone, muscle, or locomotor recovery in adult rats after severe SCI. In contrast, longer-term bodyweight-supported treadmill training associated with adjuvant testosterone-enanthate more comprehensive musculoskeletal benefit than adjuvant testosterone-enanthate alone, suggesting that adjuvant testosterone-enanthate influenced locomotor recovery. Marques et al. (2018) randomly divided 95 Wistar rats into four groups: control, SCI (rats with spinal cord contusion), and SCI groups exposed to locomotor training starting 7, 14 or 28 days after the injury (SCI-T7, SCI-T14 and SCI-T28), performed on a treadmill, five days a week, 20 minutes per day, for ten weeks. Interestingly, the authors reported that late training groups had preservation of the motoneurons in the spinal cord and larger muscle fibers area in tibialis anterior muscle; in contrast, the SCI-T7 group had higher lesion volume after locomotor training in comparison with the SCI group, demonstrating that identify a locomotor training starting time after the injury is essential to define the best therapeutic window for rehabilitation. Santo et al. (2018) investigated the effects of locomotor treadmill training on muscle tropism mediated by protein kinase B (Akt)/mammalian target of rapamycin (mTOR)/p70 ribosomal protein S6 kinase (p70S6K) in paraplegic adult female Wistar rats underwent an incomplete thoracic SCI induced by compression using an aneurysm clip, and, after 7 days, a 3-week locomotor treadmill training with body weight-support. In particular, trained animals did not show locomotor improvement, but presented an increase in muscle weight and myofiber CSA, confirming the locomotor treadmill training’s role in the prevention of soleus muscle hypotrophy in rats with SCI. To date, we found only a single recent systematic review in the literature investigating the strategies to increase skeletal muscle mass in individuals with SCI (Santos et al., 2022); however, the Authors focused on the chronic phases (after at least one year from the event) and considered only human studies.
Concerning the risk of bias in the studies included in the present systematic review, we performed an evaluation through the Joanna Briggs Institute Critical Appraisal Checklist for Quasi-Experimental Studies (non-randomized experimental studies). It was interesting to be noted that only 5 papers (20.83%) did not present any risk of bias. Furthermore, 10 studies (41.66%) showed a lack of data on follow-up outcomes. Finally, 13 (54.16%) of the 24 papers included in our systematic review did not provide data from a control group.
To the best of our knowledge, this is the first systematic review providing a broad overview of the currently available rehabilitation options to prevent VML in patients with SCI to promote an early therapeutic intervention targeting the multilevel mechanisms underpinning this disabling condition. However, we are aware that this paper is not free from limitations. First, the studies included in this systematic review administered the treatments to both humans and animals (i.e., cats and mice), resulting in complicated comparability of the obtained data. However, we believe that the inclusion of animal studies might be beneficial in the SCI field, to better understand the mechanisms underpinning muscle mass restoration. In addition, the subjects presented a very different AIS classification, with consequent different findings; lastly, we should take into consideration the high heterogeneity of the rehabilitation approaches administered, and the outcome measures assessed.
5Conclusion
Taking together the findings of the present systematic review showed that the electrical stimulation and treadmill exercises might be effective to counteract the VML in both the sub-acute and the chronic individuals with SCI. In the recent scientific literature, several rehabilitative treatments have been investigated, albeit there is no agreement on the duration and the modality of the intervention. The data obtained in the present systematic review confirm the role of approaches widely used in clinical practice as effective for the prevention of VML; this allows rehabilitators to customize the treatment for each patient while maintaining the methods investigated as a gold standard.
In this context, considering the key role that a personalized medicine might have in these subjects, further observational studies are still needed to overcome the limitations of a standardize rehabilitation in patients with SCI affected by VML. Moreover, future studies should focus on the definition of clear protocols, with timing, duration, and parameters that might be a benchmark for the treatment of a such disabling condition.
Author contributions
Conceptualization: AdS; Methodology: AdS, LM, and KM; Investigation: LM, NM, and CC; Formal analysis: AdS, LM, and NM; Data curation: AdS, KM, and AB; Writing—original draft preparation, AdS and LM; Writing—review and editing: KM and AA; Visualization: NM, CC, LL, and MI; Supervision: AdS, KM, and AA. All authors read and approved the final version of the manuscript.
Conflict of interest
None of the authors declare any conflict of interests, funding sources or consultant relationships with any organizations involved in this research.
Acknowledgments
The authors would like to thank Martina Ragona for her contribution to the work.
References
1 | Ackery, A. , Tator, C. , & Krassioukov, A. ((2004) ). A global perspective on spinal cord injury epidemiology. Journal of Neurotrauma, 21: (10), 1355–1370. https://doi.org/10.1089/neu.2004.21.1355 |
2 | Adams, C. M. , Suneja, M. , Dudley-Javoroski, S. , & Shields, R. K. ((2011) ). Altered mRNA expression after long-term soleus electrical stimulation training in humans with paralysis. Muscle & Nerve, 43: (1), 65–75. https://doi.org/10.1002/mus.21831 |
3 | Ahmed, Z. , & Wieraszko, A. ((2008) ). Combined effects of acrobatic exercise and magnetic stimulation on the functional recovery after spinal cord lesions. Journal of Neurotrauma, 25: (10), 1257–1269. https://doi.org/10.1089/neu.2008.0626 |
4 | Algahtany, M. , McFaull, S. , Chen, L. , Zhang, S. , Saarela, O. , Alqahtani, F. , & Cusimano, M. D. ((2021) ). The Changing Etiology and Epidemiology of Traumatic Spinal Injury: A Population-Based Study. World Neurosurgery, 149: , e116–e127. https://doi.org/10.1016/j.wneu.2021.02.066 |
5 | An, Y. H. , Kim, J. , Kim, H.-J. , & Lim, K. ((2020) ). Effects of leucine-enriched essential amino acid supplementation on muscular fatigue and inflammatory cytokines in wheelchair basketball players. Physical Activity and Nutrition, 24: (2), 38–46. https://doi.org/10.20463/pan.2020.0013 |
6 | ASIA and ISCoS International Standards Committee. ((2019) ). The 2019 revision of the International Standards for Neurological Classification of Spinal Cord Injury (ISNCSCI)-What’s new? Spinal Cord, 57: (10), 815–817. https://doi.org/10.1038/s41393-019-0350-9 |
7 | Baldi, J. C. , Jackson, R. D. , Moraille, R. , & Mysiw, W. J. ((1998) ). Muscle atrophy is prevented in patients with acute spinal cord injury using functional electrical stimulation. Spinal Cord, 36: (7), 463–469. https://doi.org/10.1038/sj.sc.3100679 |
8 | Bárbara-Bataller, E. , Méndez-Suárez, J. L. , Alemán-Sánchez, C. , Sánchez-Enríquez, J. , & Sosa-Henríquez, M. ((2018) ). Change in the profile of traumatic spinal cord injury over 15 years in Spain. Scandinavian Journal of Trauma, Resuscitation and EmergencyMedicine, 26: (1), 27. https://doi.org/10.1186/s13049-018-0491-4 |
9 | Barbiellini Amidei, C. , Salmaso, L. , Bellio, S. , & Saia, M. ((2022) ). Epidemiology of traumatic spinal cord injury: A large population-based study. Spinal Cord, 60: (9), Art. 9. https://doi.org/10.1038/s41393-022-00795-w |
10 | Bassil, N. , Alkaade, S. , & Morley, J. E. ((2009) ). The benefits and risks of testosterone replacement therapy: A review. Therapeutics and Clinical Risk Management, 5: , 427–448. |
11 | Battistuzzo, C. R. , Rank, M. M. , Flynn, J. R. , Morgan, D. L. , Callister, R. , Callister, R. J. , & Galea, M. P. ((2017) ). Effects Of treadmill training on hindlimb muscles of spinal cord-injured mice. Muscle & Nerve, 55: (2), 232–242. https://doi.org/10.1002/mus.25211 |
12 | Bauman, W. A. , & Cardozo, C. P. ((2015) ). Osteoporosis in Individuals with Spinal Cord Injury. PM&R, 7: (2), 188–201. https://doi.org/10.1016/j.pmrj.2014.08.948 |
13 | Beckwée, D. , Delaere, A. , Aelbrecht, S. , Baert, V. , Beaudart, C. , Bruyere, O. , de Saint-Hubert, M. , & Bautmans, I. ((2019) ). Exercise Interventions for the Prevention and Treatment of Sarcopenia. A Systematic Umbrella Review. The Journal of Nutrition, Health & Aging, 23: (6), 494–502. https://doi.org/10.1007/s12603-019-1196-8 |
14 | Bochkezanian, V. , Newton, R. U. , Trajano, G. S. , & Blazevich, A. J. ((2018) ). Effects of Neuromuscular Electrical Stimulation in People with Spinal Cord Injury. Medicine and Science in Sports and Exercise, 50: (9), 1733–1739. https://doi.org/10.1249/MSS.000000000000163 |
15 | Bustamante, C. , Brevis, F. , Canales, S. , Millón, S. , & Pascual, R. ((2016) ). Effect of functional electrical stimulation on the proprioception, motor function of the paretic upper limb, and patient quality of life: A case report.. Scopus. Journal of Hand Therapy, 29: (4), 507–514. https://doi.org/10.1016/j.jht.2016.06.01 |
16 | Butezloff, M. M. , Zamarioli, A. , Maranho, D. A. , & Shimano, A. C. ((2015) ). Effect of electrical stimulation and vibration therapy on skeletal muscle trophism in rats with complete spinal cord injury. American Journal of Physical Medicine and Rehabilitation, 94: (11), 950–957. https://doi.org/10.1097/PHM.000000000000027 |
17 | Carda, S. , Cisari, C. , & Invernizzi, M. ((2013) ). Sarcopenia or muscle modifications in neurologic diseases: A lexical or patophysiological difference? European Journal of Physical and Rehabilitation Medicine, 49: (1), 119–130. |
18 | Choi, H.-J. , Kim, G.-S. , Chai, J. H. , & Ko, C.-Y. ((2020) ). Effect of Gait Training Program with Mechanical Exoskeleton on Body Composition of Paraplegics. Journal of Multi disciplinary Healthcare, 13: , 1879–1886. https://doi.org/10.2147/JMDH.S285682 |
19 | Clark, J. M. , & Findlay, D. M. ((2017) ). Musculoskeletal Health in the Context of Spinal Cord Injury. Current Osteoporosis Reports, 15: (5), 433–442. https://doi.org/10.1007/s11914-017-0400-1 |
20 | Colaianni, G. , Mongelli, T. , Cuscito, C. , Pignataro, P. , Lippo, L. , Spiro, G. , Notarnicola, A. , Severi, I. , Passeri, G. , Mori, G. , Brunetti, G. , Moretti, B. , Tarantino, U. , Colucci, S. C. , Reseland, J. E. , Vettor, R. , Cinti, S. , & Grano, M. ((2017) ). Irisin preventsand restores bone loss and muscle atrophy in hind-limb suspendedmice. Scientific Reports, 7: (1), 2811. https://doi.org/10.1038/s41598-017-02557-1 |
21 | Corona, B. T. , Rivera, J. C. , & Greising, S. M. ((2018) ) Inflammatory and Physiological Consequences of Debridement of Fibrous Tissue after Volumetric Muscle Loss Injury. Clinical and Translational Science, 11: (2), 208–217. https://doi.org/10.1111/cts.12519 |
22 | Crameri, R. M. , Weston, A. , Climstein, M. , Davis, G. M. , & Sutton, J. R. ((2002) ) Effects of electrical stimulation-induced leg training on skeletal muscle adaptability in spinal cord injury. Scandinavian Journal of Medicine and Science in Sports, 12: (5), 316–322. https://doi.org/10.1034/j.1600-0838.2002.20106.x |
23 | Darrow, D. , Balser, D. , Netoff, T. I. , Krassioukov, A. , Phillips, A. , Parr, A. , & Samadani, U. ((2019) ) Epidural Spinal Cord Stimulation Facilitates Immediate Restoration of Dormant Motor and Autonomic Supraspinal Pathways after Chronic Neurologically Complete Spinal Cord Injury. Journal of Neurotrauma, 36: (15), 2325–2336. https://doi.org/10.1089/neu.2018.6006 |
24 | de Freitas, G. R. , Santo, C. C. E. , de Machado-Pereira, N. A. M. M. , Bobinski, F. , dos Santos, A. R. S. , & Ilha, J. ((2018) ) Early cyclical neuromuscular electrical stimulation improves strength and trophism by akt pathway signaling in partially paralyzed biceps muscle after spinal cord injury in rats. Physical Therapy, 98: (3), 172–181. https://doi.org/10.1093/ptj/pzx116 |
25 | Demchak, T. , Linderman, J. , Mysiw, W. , Jackson, R. , Suun, J. , & Devor, S. ((2005) ) Effects of functional electric stimulation cycle ergometry training on lower limb musculature in acute SCI individuals. Journal of Sports Science and Medicine, 4: (3), 263–271. |
26 | Devivo, M. J. ((2012) ) Epidemiology of traumatic spinal cord injury: Trends and future implications. Spinal Cord, 50: (5), 365–372. https://doi.org/10.1038/sc.2011.178 |
27 | Ding, W. , Hu, S. , Wang, P. , Kang, H. , Peng, R. , Dong, Y. , & Li, F. ((2022) ) Spinal Cord Injury: The Global Incidence, Prevalence, and Disability From the Global Burden of Disease Study 2019. Spine, 47: (21), 1532–1540. https://doi.org/10.1097/BRS.0000000000004417 |
28 | Ditterline, B. L. , Harkema, S. J. , Willhite, A. , Stills, S. , Ugiliweneza, B. , & Rejc, E. ((2020) ) Epidural stimulation for cardiovascular function increases lower limb lean mass in individuals with chronic motor complete spinal cord injury. Experimental Physiology, 105: (10), 1684–1691. https://doi.org/10.1113/EP088876 |
29 | Dolbow, D. R. , & Credeur, D. P. ((2018) ) Effects of resistance-guided high intensity interval functional electrical stimulation cycling on an individual with paraplegia: A case report. The Journal of Spinal Cord Medicine, 41: (2), 248–252. https://doi.org/10.1080/10790268.2017.1367358 |
30 | Downing, K. , Prisby, R. , Varanasi, V. , Zhou, J. , Pan, Z. , & Brotto, M. ((2021) ) Old and new biomarkers for volumetric muscle loss. Current Opinion in Pharmacology, 59: , 61–69. https://doi.org/10.1016/j.coph.2021.05.001 |
31 | Durán, F. S. , Lugo, L. , Ramírez, L. , & Eusse, E. ((2001) ) Effects of an exercise program on the rehabilitation ofpatients with spinal cord injury. Archives of Physical Medicineand Rehabilitation, 82: (10), 1349–1354. https://doi.org/10.1053/apmr.2001.26066 |
32 | Elder, C. P. , Apple, D. F. , Bickel, C. S. , Meyer, R. A. , & Dudley, G. A. ((2004) ) Intramuscular fat and glucose tolerance after spinalcord injury—A cross-sectional study. Spinal Cord, 42: (12), 711–716. https://doi.org/10.1038/sj.sc.3101652 |
33 | Erickson, M. L. , Ryan, T. E. , Backus, D. , & McCully, K. K. ((2017) ) Endurance neuromuscular electrical stimulation training improves skeletal muscle oxidative capacity in individuals with motor-complete spinal cord injury. Muscle & Nerve, 55: (5), 669–675. https://doi.org/10.1002/mus.25393 |
34 | Everaert, D. G. , Okuma, Y. , Abdollah, V. , & Ho, C. ((2021) ) Timing and dosage of FES cycling early after acute spinal cord injury: A case series report. The Journal of Spinal Cord Medicine, 44: (sup1), S250–S255. https://doi.org/10.1080/10790268.2021.1953323 |
35 | Farkas, G. J. , Pitot, M. A. , Berg, A. S. , & Gater, D. R. ((2019) ) Nutritional status in chronic spinal cord injury: A systematic review and meta-analysis. Spinal Cord, 57: (1), 3–17. https://doi.org/10.1038/s41393-018-0218-4 |
36 | Fu, J. , Wang, H. , Deng, L. , & Li, J. ((2016) ) Exercise Training Promotes Functional Recovery after Spinal Cord Injury. Neural Plasticity, 2016: , 4039580. https://doi.org/10.1155/2016/4039580 |
37 | Galea, M. P. , Panisset, M. G. , El-Ansary, D. , Dunlop, S. A. , Marshall, R. , Clark, J. M. , Churilov, L. , & Trial, S. S.-O. ((2017) ) SCIPA Switch-On: A Randomized Controlled Trial Investigating the Efficacy and Safety of Functional Electrical Stimulation-Assisted Cycling and Passive Cycling Initiated Early After Traumatic Spinal Cord Injury. Neurorehabilitation and Neural Repair, 31: (6), 540–551. https://doi.org/10.1177/1545968317697035 |
38 | Gao, F. , Chu, H. , Li, J. , Yang, M. , Du, L. , Li, J. , Chen, L. , Yang, D. , Zhang, H. , & Chan, C. ((2017) ) Repetitive transcranial magnetic stimulation for pain after spinal cord injury: A systematic review and meta-analysis. Journal of Neurosurgical Sciences, 61: (5), 514–522. https://doi.org/10.23736/S0390-5616.16.03809-1 |
39 | Ge, L. , Arul, K. , Ikpeze, T. , Baldwin, A. , Nickels, J. L. , & Mesfin, A. ((2018) ) Traumatic and Nontraumatic Spinal Cord Injuries. World Neurosurgery, 111: , e142–e148. https://doi.org/10.1016/j.wneu.2017.12.008 |
40 | Giangregorio, L. , Craven, C. , Richards, K. , Kapadia, N. , Hitzig, S. L. , Masani, K. , & Popovic, M. R. ((2012) ) A randomized trial of functional electrical stimulation for walking in incomplete spinal cord injury: Effects on body composition. Journal of Spinal Cord Medicine, 35: (5), 351–360. https://doi.org/10.1179/2045772312Y.0000000041 |
41 | Giangregorio, L. M. , Hicks, A. L. , Webber, C. E. , Phillips, S. M. , Craven, B. C. , Bugaresti, J. M. , & McCartney, N. ((2005) ) Body weight supported treadmill training in acute spinal cord injury: Impact on muscle and bone. Spinal Cord, 43: (11), 649–657. https://doi.org/10.1038/sj.sc.3101774 |
42 | Giangregorio, L. M. , Webber, C. E. , Phillips, S. M. , Hicks, A. L. , Craven, B. C. , Bugaresti, J. M. , & McCartney, N. ((2006) ) Can body weight supported treadmill training increase bone mass and reverse muscle atrophy in individuals with chronic incomplete spinal cord injury? Applied Physiology, Nutrition, and Metabolism= Physiologie Appliquee, Nutrition et Metabolisme, 31: (3), 283–291. https://doi.org/10.1139/h05-036 |
43 | Gorgey, A. S. , & Dudley, G. A. , ((2007) a). Skeletal muscle atrophy and increased intramuscular fat after incomplete spinal cord injury. Spinal Cord, 45: (4), 304–309. https://doi.org/10.1038/sj.sc.3101968 |
44 | Gorgey, A. S. , & Dudley, G. A. , ((2007) b). Skeletal muscle atrophy and increased intramuscular fat after incomplete spinal cord injury. Spinal Cord, 45: (4), 304–309. https://doi.org/10.1038/sj.sc.3101968 |
45 | Gorgey, A. S. , & Shepherd, C. ((2010) ) Skeletal muscle hypertrophy and decreased intramuscular fat after unilateral resistance training in spinal cord injury: Case report. The Journal of Spinal Cord Medicine, 33: (1), 90–95. https://doi.org/10.1080/10790268.2010.11689681 |
46 | Gorgey, A. S. , Witt, O. , O’Brien, L. , Cardozo, C. , Chen, Q. , Lesnefsky, E. J. , & Graham, Z. A. ((2019) ) Mitochondrial health and muscle plasticity after spinal cord injury. European Journal of Applied Physiology, 119: (2), 315–331. https://doi.org/10.1007/s00421-018-4039-0 |
47 | Hachem, L. D. , Ahuja, C. S. , & Fehlings, M. G. ((2017) ) Assessment and management of acute spinal cord injury: From point of injury to rehabilitation. The Journal of Spinal Cord Medicine, 40: (6), 665–675. https://doi.org/10.1080/10790268.2017.1329076 |
48 | Higgins, J.P. , Chandler, J. , Cumpston, J. , Li, M. , Page, T. , & Welch, M.J. , V.A. (Eds.) Cochrane Handbook for Systematic Reviews of Interventions, Version 6.2; John Wiley & Sons: Hoboken, NJ, USA, 2021. (s.d.). |
49 | Holman, M. E. , & Gorgey, A. S. ((2019) ) Testosterone and Resistance Training Improve Muscle Quality in Spinal Cord Injury. Medicine and Science in Sports and Exercise, 51: (8), 1591–1598. https://doi.org/10.1249/MSS.0000000000001975 |
50 | Hou, J. , Nelson, R. , Nissim, N. , Parmer, R. , Thompson, F. J. , & Bose, P. ((2014) ) Effect of combined treadmill training and magnetic stimulation on spasticity and gait impairments after cervical spinal cord injury. Journal of Neurotrauma, 31: (12), 1088–1106. https://doi.org/10.1089/neu.2013.3096 |
51 | Invernizzi, M. , Carda, S. , Rizzi, M. , Grana, E. , Squarzanti, D. F. , Cisari, C. , Molinari, C. , & Renò, F. ((2015) ) Evaluation of serum myostatin and sclerostin levels in chronic spinal cord injured patients. Spinal Cord, 53: (8), 615–620. https://doi.org/10.1038/sc.2015.61 |
52 | Invernizzi, M. , de Sire, A. , Carda, S. , Venetis, K. , Renò, F. , Cisari, C. , & Fusco, N. ((2020) ) Bone Muscle Crosstalk in Spinal Cord Injuries: Pathophysiology and Implications for Patients’ Quality of Life. Current Osteoporosis Reports, 18: (4), 422–431. https://doi.org/10.1007/s11914-020-00601-7 |
53 | Invernizzi, M. , de Sire, A. , & Fusco, N. ((2021) ) Rethinking the clinical management of volumetric muscle loss in patients with spinal cord injury: Synergy among nutritional supplementation, pharmacotherapy, and rehabilitation. Current Opinion in Pharmacology, 57: , 132–139. https://doi.org/10.1016/j.coph.2021.02.003 |
54 | Invernizzi, M. , de Sire, A. , Renò, F. , Cisari, C. , Runza, L. , Baricich, A. , Carda, S. , & Fusco, N. ((2020) ) Spinal Cord Injury as a Model of Bone-Muscle Interactions: Therapeutic Implications From in vitro and in vivo Studies. Frontiers in Endocrinology, 11: , 204. https://doi.org/10.3389/fendo.2020.00204 |
55 | Iolascon, G. , Gimigliano, R. , Bianco, M. , De Sire, A. , Moretti, A. , Giusti, A. , Malavolta, N. , Migliaccio, S. , Migliore, A. , Napoli, N. , Piscitelli, P. , Resmini, G. , Tarantino, U. , & Gimigliano, F. ((2017) ) Are Dietary Supplements and Nutraceuticals Effective for Musculoskeletal Health and Cognitive Function? A Scoping Review. The Journal of Nutrition, Health & Aging, 21: (5), 527–538. https://doi.org/10.1007/s12603-016-0823-x |
56 | Jacobs, P. L. , Mahoney, E. T. , Cohn, K. A. , Sheradsky, L. F. , & Green, B. A. ((2002) ) Oral creatine supplementation enhances upper extremity work capacity in persons with cervical-level spinal cord injury. Archives of Physical Medicine and Rehabilitation, 83: (1), 19–23. https://doi.org/10.1053/apmr.2002.26829 |
57 | Jurkiewicz, M. T. , Mikulis, D. J. , McIlroy, W. E. , Fehlings, M. G. , & Verrier, M. C. ((2007) ) Sensorimotor cortical plasticity during recovery following spinal cord injury: A longitudinal fMRI study. Neurorehabilitation and Neural Repair, 21: (6), 527–538. https://doi.org/10.1177/1545968307301872 |
58 | Kapadia, N. , Masani, K. , Catharine Craven, B. , Giangregorio, L. M. , Hitzig, S. L. , Richards, K. , & Popovic, M. R. ((2014) ) A randomized trial of functional electrical stimulation for walking in incomplete spinal cord injury: Effects on walking competency. The Journal of Spinal Cord Medicine, 37: (5), 511–524. https://doi.org/10.1179/2045772314Y.0000000263 |
59 | Karelis, A. D. , Carvalho, L. P. , Castillo, M. J. , Gagnon, D. H. , & Aubertin-Leheudre, M. ((2017) ) Effect on body composition and bone mineral density of walking with a robotic exoskeleton in adults with chronic spinal cord injury. Journal of Rehabilitation Medicine, 49: (1), 84–87. https://doi.org/10.2340/16501977-2173 |
60 | Karimi, M. T. ((2011) ) Evidence-based evaluation of physiological effects of standing and walking in individuals with spinal cord injury. Iranian Journal of Medical Sciences, 36: (4), 242–253. |
61 | Kirshblum, S. , Botticello, A. , Benedetto, J. , Donovan, J. , Marino, R. , Hsieh, S. , & Wagaman, N. ((2020) ) A Comparison of Diagnostic Stability of the ASIA Impairment Scale Versus Frankel Classification Systems for Traumatic Spinal Cord Injury. Archives of Physical Medicine and Rehabilitation, 101: (9), 1556–1562. https://doi.org/10.1016/j.apmr.2020.05.016 |
62 | Kirshblum, S. , Snider, B. , Rupp, R. , & Read, M. S. , International Standards Committee of ASIA and ISCoS. ((2020) ) Updates of the International Standards for Neurologic Classification of Spinal Cord Injury: 2015 and 2019. Physical Medicine and Rehabilitation Clinics of North America, 31: (3), 319–330. https://doi.org/10.1016/j.pmr.2020.03.005 |
63 | Kojouharov, H. V. , Chen-Charpentier, B. M. , Solis, F. J. , Biguetti, C. , & Brotto, M. ((2021) ) A simple model of immune and muscle cell crosstalk during muscle regeneration. Mathematical Biosciences, 333: , 108543. https://doi.org/10.1016/j.mbs.2021.108543 |
64 | Liu, H. , Angert, M. , Nishihara, T. , Shubayev, I. , Dolkas, J. , & Shubayev, V. I. ((2015) ) Spinal Glia Division Contributes to Conditioning Lesion-Induced Axon Regeneration Into the Injured Spinal Cord: Potential Role of Cyclic AMP-Induced Tissue Inhibitor of Metalloproteinase-1. Journal of Neuropathology and Experimental Neurology, 74: (6), 500–511. https://doi.org/10.1097/NEN.0000000000000192 |
65 | Liu, M. , Bose, P. , Walter, G. A. , Thompson, F. J. , & Vandenborne, K. ((2008) ) A longitudinal study of skeletal muscle following spinal cord injury and locomotor training. Spinal Cord, 46: (7), 488–493. https://doi.org/10.1038/sj.sc.3102169 |
66 | Liu, M. , Stevens-Lapsley, J. E. , Jayaraman, A. , Ye, F. , Conover, C. , Walter, G. A. , Bose, P. , Thompson, F. J. , Borst, S. E. , & Vandenborne, K. ((2010) ) Impact of treadmill locomotor training onskeletal muscle IGF and myogenic regulatory factors in spinal cordinjured rats. European Journal of Applied Physiology, 109: (4), 709–720. https://doi.org/10.1007/s00421-010-1392-z |
67 | Maher, J. L. , McMillan, D. W. , & Nash, M. S. ((2017) ) Exercise and Health-Related Risks of Physical Deconditioning After Spinal Cord Injury. Topics in Spinal Cord Injury Rehabilitation, 23: (3), 175–187. https://doi.org/10.1310/sci2303-175 |
68 | Mahoney, E. , Puetz, T. W. , Dudley, G. A. , & McCully, K. K. ((2007) ) Low-Frequency Fatigue in Individuals With Spinal Cord Injury. The Journal of Spinal Cord Medicine, 30: (5), 458–466. |
69 | Mailhot, G. , Lamarche, J. , & Gagnon, D. H. ((2018) ) Effectiveness oftwo vitamin D3 repletion protocols on the vitamin D status of adultswith a recent spinal cord injury undergoing inpatientrehabilitation: A prospective case series. Spinal Cord Seriesand Cases, 4: , 96. https://doi.org/10.1038/s41394-018-0129-9 |
70 | Marques, M. R. , Nicola, F. C. , Sanches, E. F. , Arcego, D. M. , Durán-Carabali, L. E. , Aristimunha, D. , Dalmaz, C. , & Netto, C. A. ((2018) ) Locomotor Training Promotes Time-dependent Functional Recovery after Experimental Spinal Cord Contusion. Neuroscience, 392: , 258–269. https://doi.org/10.1016/j.neuroscience.2018.08.033 |
71 | Marquez-Chin, C. , & Popovic, M. R. ((2020) ) Functional electrical stimulation therapy for restoration of motor function after spinal cord injury and stroke: A review. Biomedical Engineering Online, 19: (1), 34. https://doi.org/10.1186/s12938-020-00773-4 |
72 | McDonald, J. W. , & Sadowsky, C. ((2002) ) Spinal-cord injury. Lancet (London England), 359: (9304), 417–425. https://doi.org/10.1016/S0140-6736(02)07603-1 |
73 | McHugh, C. , Taylor, C. , Mockler, D. , & Fleming, N. ((2021) ) Epidural spinal cord stimulation for motor recovery in spinal cord injury: A systematic review. Neurorehabilitation, 49: (1), 1–22. https://doi.org/10.3233/NRE-210093 |
74 | Megía García, A. , Serrano-Muñoz, D. , Taylor, J. , Avendaño-Coy, J. , & Gómez-Soriano, J. ((2020) ) Transcutaneous Spinal Cord Stimulation and Motor Rehabilitation in Spinal Cord Injury: A Systematic Review. Neurorehabilitationand Neural Repair, 34: (1), 3–12. https://doi.org/10.1177/1545968319893298 |
75 | Mehrholz, J. , Harvey, L. A. , Thomas, S. , & Elsner, B. ((2017) ) Is body-weight-supported treadmill training or robotic-assisted gait training superior to overground gait training and other forms of physiotherapy in people with spinal cord injury? A systematic review. Spinal Cord, 55: (8), 722–729. https://doi.org/10.1038/sc.2017.31 |
76 | Nakamura, M. , Houghtling, R. A. , MacArthur, L. , Bayer, B. M. , & Bregman, B. S. ((2003) ) Differences in cytokine gene expression profile between acute and secondary injury in adult rat spinal cord. Experimental Neurology, 184: (1), 313–325. https://doi.org/10.1016/s0014-4886(03)00361-3 |
77 | Nightingale, T. E. , Moore, P. , Harman, J. , Khalil, R. , Gill, R. S. , Castillo, T. , Adler, R. A. , & Gorgey, A. S. ((2018) ) Body composition changes with testosterone replacement therapy following spinal cord injury and aging: A mini review. The Journal of Spinal Cord Medicine, 41: (6), 624–636. https://doi.org/10.1080/10790268.2017.1357917 |
78 | Olive, J. L. , Slade, J. M. , Dudley, G. A. , & McCully, K. K. ((2003) ) Blood flow and muscle fatigue in SCI individuals during electrical stimulation. Journal of Applied Physiology, 94: (2), 701–708. https://doi.org/10.1152/japplphysiol.00736.2002 |
79 | Panisset, M. G. , Galea, M. P. , & El-Ansary, D. ((2016) ) Does early exercise attenuate muscle atrophy or bone loss after spinal cord injury? Spinal Cord, 54: (2), 84–92. https://doi.org/10.1038/sc.2015.150 |
80 | Qin, W. , Bauman, W. A. , & Cardozo, C. ((2010) ) Bone and muscle loss after spinal cord injury: Organ interactions. Annals of the New York Academy of Sciences, 1211: , 66–84. https://doi.org/10.1111/j.1749-6632.2010.05806.x |
81 | Roy, R. R. , Talmadge, R. J. , Hodgson, J. A. , Zhong, H. , Baldwin, K. M. , & Edgerton, V. R. ((1998) ) Training effects on soleus of cats spinal cord transected (T12-13) as adults. Muscle and Nerve, 21: (1), 63–71. https://doi.org/10.1002/(SICI)1097-4598(199801)21:1<63::AID-MUS9>3.0.CO;2-E |
82 | Rupp, R. ((2020) ) Spinal cord lesions. Handbook of Clinical Neurology, 168: , 51–65. https://doi.org/10.1016/B978-0-444-63934-9.00006-8 |
83 | Sandrow-Feinberg, H. R. , & Houlé, J. D. ((2015) ) Exercise after Spinal Cord Injury as an Agent for Neuroprotection, Regeneration and Rehabilitation. Brain Research, 1619: , 12–21. https://doi.org/10.1016/j.brainres.2015.03.052 |
84 | Santo, C. C. D. E. , Secco, D. D. , Meireles, A. , De Freitas, G. R. , Bobinski, F. , Cunha, M. P. , Rodrigues, A. L. S. , Swarowsky, A. , Santos, A. R. S. , & Ilha, J. ((2018) ) Locomotor treadmill training promotes soleus trophism by mammalian target of rapamycin pathway in paraplegic rats. Neurochemical Research, 43: (6), 1258–1268. https://doi.org/10.1007/s11064-018-2543-6 |
85 | Santos, L. V. , Pereira, E. T. , Reguera-García, M. M. , Oliveira, C. E. P. , & de, Moreira, O. C. ((2022) ) Resistance Training and Muscle Strength in people with Spinal cord injury: A systematic review and meta-analysis. Journal of Bodywork and Movement Therapies, 29: , 154–160. https://doi.org/10.1016/j.jbmt.2021.09.031 |
86 | Schopp, L. H. , Clark, M. , Mazurek, M. O. , Hagglund, K. J. , Acuff, M. E. , Sherman, A. K. , & Childers, M. K. ((2006) ) Testosterone levels among men with spinal cord injury admitted to inpatient rehabilitation. American Journal of Physical Medicine & Rehabilitation, 85: (8), 685–687. https://doi.org/10.1097/01.phm.0000228617.94079.4a |
87 | Singh, A. , Balasubramanian, S. , Murray, M. , Lemay, M. , & Houle, J. ((2011) ) Role of spared pathways in locomotor recovery after body-weight-supported treadmill training in contused rats. Journal of Neurotrauma, 28: (12), 2405–2416. https://doi.org/10.1089/neu.2010.1660 |
88 | Singh, A. , Tetreault, L. , Kalsi-Ryan, S. , Nouri, A. , & Fehlings, M. G. ((2014) ) Global prevalence and incidence of traumatic spinal cord injury. Clinical Epidemiology, 6: , 309–331. https://doi.org/10.2147/CLEP.S68889 |
89 | Skiba, G. H. , Andrade, S. F. , & Rodacki, A. F. (2021). Effects of functional electro-stimulation combined with blood flow restriction in affected muscles by spinal cord injury. Neurological Sciences: Official Journal of the Italian Neurological Society and of the Italian Society of Clinical Neurophysiology. https://doi.org/10.1007/s10072-021-05307-x |
90 | Spangenburg, E. E. , Le Roith, D. , Ward, C. W. , & Bodine, S. C. ((2008) ) A functional insulin-like growth factor receptor is not necessary for load-induced skeletal muscle hypertrophy. The Journal of Physiology, 586: (1), 283–291. https://doi.org/10.1113/jphysiol.2007.141507 |
91 | Stevens, J. E. , Liu, M. , Bose, P. , O’Steen, W. A. , Thompson, F. J. , Anderson, D. K. , & Vandenborne, K. ((2006) ) Changes in soleus muscle function and fiber morphology with one week of locomotor training in spinal cord contusion injured rats. Journal of Neurotrauma, 23: (11), 1671–1681. https://doi.org/10.1089/neu.2006.23.1671 |
92 | Sutor, T. , Kura, J. , Mattingly, A. , Otzel, D. , & Yarrow, J. ((2022) ) The Effects of Exercise and Activity-Based Physical Therapy on Bone after Spinal Cord Injury. International Journal of Molecular Sciences, 23: (2). https://doi.org/10.3390/ijms23020608 |
93 | Tator, C. H. ((1995) ) Update on the pathophysiology and pathology of acute spinal cord injury. Brain Pathology (Zurich, Switzerland), 5: (4), 407–413. https://doi.org/10.1111/j.1750-3639.1995.tb00619.x |
94 | Ung, R. V. , Lapointe, N. P. , Rouleau, P. , & Guertin, P. A. ((2010) ) Non-assisted treadmill training does not improve motor recovery and body composition in spinal cord-transected mice. Spinal Cord, 48: (10), 750–755. https://doi.org/10.1038/sc.2010.19 |
95 | Ung, R.-V. , Rouleau, P. , & Guertin, P. A. ((2012) ) Functional and physiological effects of treadmill training induced by buspirone, carbidopa, and L-DOPA in clenbuterol-treated paraplegic mice. Neurorehabilitation and Neural Repair, 26: (4), 385–394. https://doi.org/10.1177/1545968311427042 |
96 | van Middendorp, J. J. , Hosman, A. J. F. , Donders, A. R. T. , Pouw, M. H. , Ditunno, J. F. , Curt, A. , Geurts, A. C. H. , & Van de Meent, H. , EM-SCI Study Group. ((2011) ) A clinical prediction rule for ambulation outcomes after traumatic spinal cord injury: A longitudinal cohort study. Lancet (London England), 377: (9770), 1004–1010. https://doi.org/10.1016/S0140-6736(10)62276-3 |
97 | Yarrow, J. F. , Kok, H. J. , Phillips, E. G. , Conover, C. F. , Lee, J. , Bassett, T. E. , Buckley, K. H. , Reynolds, M. C. , Wnek, R. D. , Otzel, D. M. , Chen, C. , Jiron, J. M. , Graham, Z. A. , Cardozo, C. , Vandenborne, K. , Bose, P. K. , Aguirre, J. I. , Borst, S. E. , & Ye, F. ((2020) ) Locomotor training with adjuvanttestosterone preserves cancellous bone and promotes muscleplasticity in male rats after severe spinal cord injury. Journal of Neuroscience Research, 98: (5), 843–868. https://doi.org/10.1002/jnr.24564 |
98 | Yip, P. K. , & Malaspina, A. ((2012) ) Spinal cord trauma and the molecular point of no return. Molecular Neurodegeneration, 7: , 6. https://doi.org/10.1186/1750-1326-7-6 |
99 | Zhang, Y.-T. , Jin, H. , Wang, J.-H. , Wen, L.-Y. , Yang, Y. , Ruan, J.-W. , Zhang, S.-X. , Ling, E.-A. , Ding, Y. , & Zeng, Y.-S. ((2017) ) Tail Nerve Electrical Stimulation and Electro-Acupuncture Can Protect Spinal Motor Neurons and Alleviate Muscle Atrophy after Spinal Cord Transection in Rats. Neural Plasticity, 2017: , 7351238. https://doi.org/10.1155/2017/7351238 |
100 | Zhao, W. , Peng, Y. , Hu, Y. , Guo, X. E. , Li, J. , Cao, J. , Pan, J. , Feng, J. Q. , Cardozo, C. , Jarvis, J. , Bauman, W. A. , & Qin, W. ((2021) ) Electrical stimulation of hindlimb skeletal muscle has beneficial effects on sublesional bone in a rat model of spinal cord injury. Bone, 144: , 115825. https://doi.org/10.1016/j.bone.2020.115825 |