Reference theories and future perspectives on robot-assisted rehabilitation in people with neurological conditions: A scoping review and recommendations from the Italian Consensus Conference on Robotics in Neurorehabilitation (CICERONE)
Abstract
BACKGROUND:
Robot-based treatments are developing in neurorehabilitation settings. Recently, the Italian National Health Systems recognized robot-based rehabilitation as a refundable service. Thus, the Italian neurorehabilitation community promoted a national consensus on this topic.
OBJECTIVE:
To conceptualize undisclosed perspectives for research and applications of robotics for neurorehabilitation, based on a qualitative synthesis of reference theoretical models.
METHODS:
A scoping review was carried out based on a specific question from the consensus Jury. A foreground search strategy was developed on theoretical models (context) of robot-based rehabilitation (exposure), in neurological patients (population). PubMed and EMBASE® databases were searched and studies on theoretical models of motor control, neurobiology of recovery, human-robot interaction and economic sustainability were included, while experimental studies not aimed to investigate theoretical frameworks, or considering prosthetics, were excluded.
RESULTS:
Overall, 3699 records were screened and finally 9 papers included according to inclusion and exclusion criteria. According to the population investigated, structured information on theoretical models and indications for future research was summarized in a synoptic table.
CONCLUSION:
The main indication from the Italian consensus on robotics in neurorehabilitation is the priority to design research studies aimed to investigate the role of robotic and electromechanical devices in promoting neuroplasticity.
1Introduction
Nowadays, robot-assisted rehabilitation is a growing part of the rehabilitation intervention for people who survived focal injuries, or live with degenerative diseases of the Central Nervous System (CNS) (Meng, Huang, Liu, & Qu, 2017; Weinrich, 2006). In this framework, electromechanical and robot-assisted rehabilitation devices complement the patient/therapist relationship, allowing to develop personalized approaches based on individual functional profile of each patient and aimed to promote the best recovery of sensorimotor, behavioral and motor functionalities (Turchetti et al., 2009). Among the advantages of using robotic equipment the following need to be acknowledged: boosting of frequency/intensity of stimulation, supporting voluntary intention to act, enriching the sensorimotor information, thus empowering the whole interaction with the external environment. Indeed, these are most of the main principles of the experience-dependent neural plasticity underpinning rehabilitation-induced recovery (Jones et al., 2009; Kleim & Jones, 2008; Reinkensmeyer, Wolbrecht, & Bobrow, 2007; Weinrich et al., 2004).
Recently, the Italian National Health System (IT-NHS) included in its latest Essential Levels of Healthcare (2018) (list of therapies financed by the IT-NHS) robot-assisted rehabilitation among the refundable services. This change of policy followed by many years the breaking change of rehabilitation paradigm in practice, due to large dissemination of robotic devices in rehabilitation services, regardless evidence of their efficacy, effectiveness and sustainability.
Based on those premises, the need emerged within the Italian neurorehabilitation community of a collegiate consensus involving all the stakeholders (e.g. National Health Institutions, Scientific Societies, Patients Associations, Medical, Research, Industry, Economic, Juridical and Ethical experts) in the field. Soon after the update of the Essential Levels of Healthcare in 2018 the Italian Society of Physical and Rehabilitation Medicine (SIMFER) and the Italian Society of Neurological Rehabilitation (SIRN), with the support of the Italian National Institute of Health (IT-NIH), have promoted the Italian Consensus Conference on Rehabilitation assisted by robotic and electromechanical devices for persons with neurological disabilities (Conferenza nazionale di consenso: la riabilitazione assistita da robot e dispositivi elettromeccanici per le persone con disabilitg di origine neurologica –CICERONE) (Boldrini, Bonaiuti, Mazzoleni, & Posteraro, 2019, 2021; Gimigliano et al., 2021).
The aim of the CICERONE Consensus was to provide recommendations on classification, endpoints, reference theories, model of management and delivery of care services, education and legislation dealing with the use of robotics and electromechanical devices for rehabilitation of people with neurological conditions. The consensus was managed according to the national standards published by the IT-NIH (Candiani et al., 2019), thus the Steering Committee (SC), Technical Scientific Committee (CTS), Jury Panel and Working Groups (WGs) were selected. From January to March 2019 the Steering Committee and the TSC, in charge of defining the key questions for the Jury, were nominated. Soon after, several WGs, responsible for the development of evidence-based documents for final recommendations, were nominated on May 2019. Finally, the Jury nominated on October 2019, was asked to answer with recommendations to six specific questions. In this regard, the WGs developed a first version of evidence from May 2019 to March 2020, then updated from November 2020 to March 2021, on the bases of the Panel review. The final document of the CICERONE Consensus was agreed on 15th September 2021 and officially released on December 2021.
The WG3 was in charge of developing evidence for the Jury on the question number 3: “Which are the most relevant reference theoretical models for the development of robotic and electromechanical devices for neurorehabilitation? Which are the most promising perspectives for the future? Which recommendations should be given for research and development?”. In this regard, rather than being asked to quantify results the WG3 was the only group within the CICERONE Consensus asked to conceptualize its findings, with the aim of suggesting undisclosed perspectives for reference theories to be applied in robotics for rehabilitation of people with neurological conditions.
This paper reports the results corresponding to the research question the WG3 developed, designed in the form of a scoping review (Armstrong, Hall, Doyle, & Waters, 2011), with the aim of providing support for the Jury final recommendations.
2Material and methods
Given the theoretical framework of the SC question proposed by the SC and the TSC, the following fields of scientific literature were considered as reference:
• Motor control from the perspectives of mathematical modelling, neurobiology and engineering;
• The rationale underpinning the use of robots and electromechanical devices for neurorehabilitation:
• System dynamics, human-robot interaction and design;
• Engineering and economical theoretical models for measuring efficacy and effectiveness of robotic and electromechanical devices;
• Directions and trends for future development.
Such qualitative analysis of the literature was targeted to identify the potential of electromechanical and robotic devices for promoting the best functional reorganization after disruption of the CNS, to explore the capability of the best embodiment and empowerment of human-machine integration/interaction for both recovery and/or substitution of functions, to define technical limitations of devices currently available, to uncover the future opportunities of appropriateness, affordability, production and commercialization of devices for the largest fruition of technology in real clinical settings.
With the aim of developing a proper search strategy, the Panel question was translated in a foreground clinical query based on the PIC (Population, Intervention, Context) model (Munn, Stern, Aromataris, Lockwood, & Jordan, 2018). Thus, each concept of the query was defined through appropriate Medical Subject Headings (MeSH) terms and keywords, allowing to delimit the records retrieved in PubMed and EMBASE® medical databases (Table 1).
Table 1
Medical subject headings (MeSH) considered for the search strategy
Population | Intervention | Context |
Neurological rehabilitation [MeSH] | Robotics [MeSH] | Models, theoretical [MeSH] |
Neurology [MeSH] | Exercise therapy [MeSH] | Rehabilitation research [MeSH] |
Rehabilitation [MeSH] | Exoskeleton device [MeSH] | Models, neurological [MeSH] |
Physical and rehabilitation medicine [MeSH] | Disease models, animal [MeSH] | |
Patient-specific modelling [MeSH] | ||
Models, animal [MeSH] |
Following, a specific syntax for each database was developed and run (Tables 2 and 3).
Table 2
Exploded search strategies run in PubMed
No. | String |
#1 | (“Physical and Rehabilitation Medicine”[Mesh] OR “Neurological Rehabilitation”[Mesh] OR “Rehabilitation”[Mesh]) OR “Neurology”[Mesh] |
#2 | (“Robotics”[Mesh] OR “Exoskeleton Device”[Mesh]) OR “Exercise Therapy”[Mesh] |
#3 | ((“Models, Animal”[Mesh] OR “Models, Theoretical”[Mesh] OR “Models, Neurological”[Mesh]) OR (“Disease Models, Animal”[Mesh] OR “Patient-Specific Modeling”[Mesh])) OR “Rehabilitation Research”[Mesh] |
#4 | #1 AND #2 AND #3 |
Table 3
Exploded search strategies run in EMBASE®
No. | String |
#1 | ‘physical and rehabilitation medicine’/exp OR ‘physical and rehabilitation medicine’ OR ‘neurological rehabilitation’/exp OR ‘neurological rehabilitation’ OR ‘rehabilitation’/exp OR ‘rehabilitation’ OR ‘neurology’/exp OR ‘neurology’ |
#2 | ‘robotics’/exp OR ‘robotics’ OR ‘exoskeleton device’/exp OR ‘exoskeleton device’ OR ‘exercise therapy’/exp OR ‘exercise therapy’ |
#3 | ‘models, animal’/exp OR ‘models, animal’ OR ‘models, theoretical’/exp OR ‘models, theoretical’ OR ‘models, neurological’/exp OR ‘models, neurological’ OR ‘disease models, animal’/exp OR ‘disease models, animal’ OR ‘patient-specific modeling’/exp OR ‘patient-specific modeling’ OR ‘rehabilitation research’/exp OR ‘rehabilitation research’ |
#4 | #1 AND #2 AND #3 |
2.1Studies selection and synthesis
The records retrieved from each database were merged and duplicates removed using EndNote® citation management software (Team, 2013), then all the title and abstracts were screened by two independent reviewers on Rayyan (Ouzzani, Hammady, Fedorowicz, & Elmagarmid, 2016), with a third solving eventual disagreement. Full-text of selected abstracts were screened and finally included for qualitative synthesis, according to the following inclusion criteria:
• Discussing electromechanical and robotic devices considered as exoskeletons or end-effectors;
• Explaining computational, anatomical or neurophysiological theoretical models;
• Including neurological conditions.
Conversely, all the experimental studies (e.g. randomized controlled trials, clinical controlled trials, proof of concept) not aimed at investigating theoretical frameworks for research and development, and studies considering rehabilitation robotic devices for prosthetic or assistive use, were excluded.
The same information was extracted from all the studies included (i.e. first author, year of publication, population, type of study, aim of the study, results, theoretical models discussed, indications for future research) and summarized in a synoptic table.
Due to qualitative and explorative scope of the review no critical appraisal method was considered for grading the methodological quality of the included studies.
3Results
The search strategy was last updated on 5th February 2020 giving 1698 and 2001 results on PubMed and EMBASE®, respectively. Overall, 3699 records were retrieved and after duplicates removal (N = 144), application of inclusion and exclusion criteria to title and abstract (N = 3534) and full-text (N = 12), 9 records were finally included in the scoping review (Figure 1).
Fig. 1
PRISMA diagram of the review process.
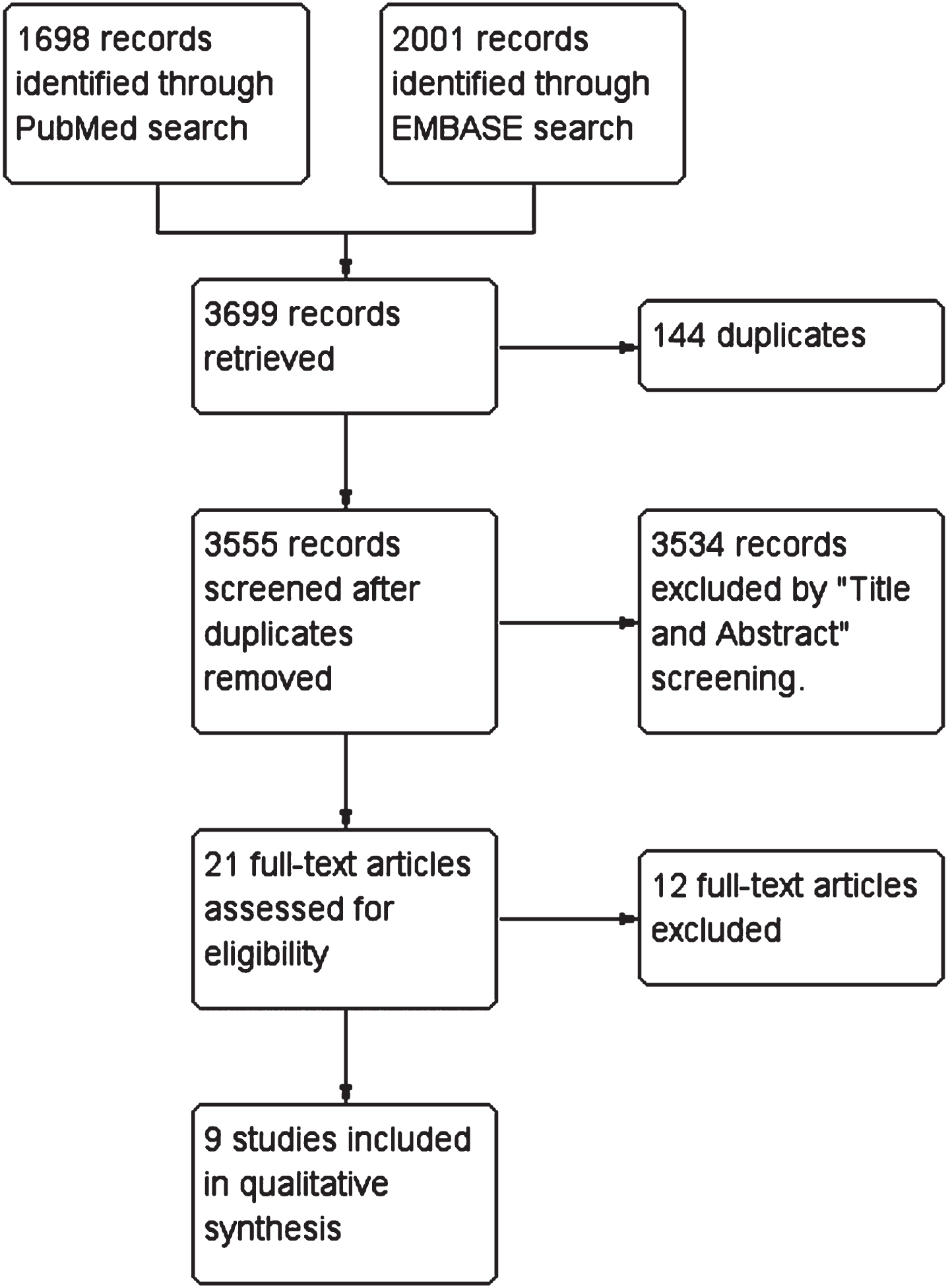
In the synoptic table synthesis of results from each study included is reported (Table 4). As shown in that Table, five of these nine papers are reviews, then a survey, a budget impact analysis, a qualitative thematic analysis and an editorial were included.
Table 4
Synoptic table of included studies
First author, year | Populations | Type of study | Type of robot | District/function | Reference theoretical model | Future research |
Lo, 2012(Lo & Xie, 2012) | Stroke | Review | Exoskeleton | Upper limb | Mechanical singularity Redundancy (DOF) HRI Actuation Control modelling Adaptive control | Integration with VR (virtual games) |
Louie, 2016(Louie & Eng, 2016) | Stroke | Scoping review | Actuated exoskeleton | Gait | Clinical efficacy | Efficacy in non-walking patients Impact of baseline function on the use of the exoskeleton Impact of exoskeleton mechanical configuration on efficacy Bilateral vs unilateral design Optimal dose of training for walking recovery Exoskeleton vs. BWS Safety for the therapist in the post-acute phase application |
Pavel, 2013 (Pavel et al., 2013) | Health systems | Review | Robot-assisted recovery and rehabilitation | / | HRI intuitive Automatic detection of human behavior Automatic detection of emotions and physiological states Long-term adaptation to user needs Quantitative diagnosis and assessment Manipulation dexterity Robot safety | / |
Pinto, 2020(Pinto et al., 2020) | SCI | BIA | Exoskeleton | Walking | Economic sustainability | |
Scott, 2011(Scott & Dukelow, 2011) | Stroke | Review | Exoskeleton | Upper limb | Motor, sensory and cognitive functions assessment | Economic sustainability Quantification of impairments, after stroke Assessment time needed Integration with other sensors (e.g. eye-tracking, blood pressure) Treatment plan Access to treatment |
Wade, 2011 (Wade & Winstein, 2011) | Stroke | Review | Exoskeleton End-effector Socially assistive robot | Upper limb | Automatic assessment of impairment Home-based functional recovery EBM assessment | Patient-based clinicians/engineers partnership Costs Augmenting technologies for rehabilitation |
Wang, 2017 (Wang, Sudhama, Begum, Huq, & Mihailidis, 2017) | AD and caregivers. | Qualitative thematic analysis | Assistive robot | ADL | Perception of assistive robot Social relationship | Consistency and variability of assistive robot perception Opportunities of assistive robots Reflections on implications in social relationships |
Whitall, 2004 (Whitall, 2004) | Stroke | Editorial | / | Motor function | Rehabilitation-induced neuroplasticity | Effect of combining rehabilitation plans |
Wolff, 2014 (Wolff, Parker, Borisoff, Mortenson, & Mattie, 2014) | Patients with wheelchairs, healthcare professionals | Survey | Exoskeleton | Rehabilitation | Design Psycho-social-motor wellness ADL Controlled by patient Functional effect size | Reliability Comfort Safety Easiness of use |
AD: Alzheimer’s disease; ADL: Activities of Daily Living; BIA: Budget Impact Analysis; BWS: Body Weight Support; DOF: Degrees Of Freedom; EBM: Evidence Based Medicine; HRI: Human-Robot Interface; SCI: Spinal Cord Injury; VR: Virtual Reality.
Results from included studies suggest that specific reference models and directions for research and development are needed for specific districts and functions. More in details, the upper limb requires high accuracy and carefulness in managing the mechanical design of the device (e.g. mechanical singularity, redundancy of degrees of freedom, actuation and control), integration with other non-robotic technologies (e.g. virtual reality) (Lo & Xie, 2012; Wade & Winstein, 2011) and fine assessment of residual functions (e.g. motor, sensory, cognitive) (Scott & Dukelow, 2011). On the other hand, regarding robotic devices for rehabilitation of the lower limb in gait and walking function, the main issues are clinical efficacy, optimal stratification of patients for referral to therapy, dose of training, safety and costs of use (Louie & Eng, 2016; Pinto et al., 2020). Finally, a third critical issue is the general applicability of robotics in neurorehabilitation meant as reliability, comfort, easiness of use, and (social) perception of devices. All those features, have to be considered as pivotal for determining whether the use of robotic devices will be integrated in standard rehabilitation settings, in the next future.
Our findings are coherent with fundamentals of motor neuroscience that need to define specific models for interpretation and explanation of real-time functioning of each body district and function (Cheung et al., 2009; Cheung et al., 2012; Ting et al., 2015), within a unique general theory of motor control (Poggio & Bizzi, 2004; Wolpert, Diedrichsen, & Flanagan, 2011).
4Conclusions
From the qualitative analysis of included studies, many challenges for the future of robots in neurorehabilitation emerged. As to research aims, the need of a better understanding of the mechanisms underpinning true and/or compensation recovery has clearly emerged. The presence of five reviews, two analyses, one editorial and one survey may suggest an a-posteriori attempt to define the reference theoretical model, instead of a research & development-based one. Thus, a first research aim is to investigate the effects of robot-based rehabilitation for both assessment and treatment of neurological conditions, especially in stroke, as it is the most prevalent neurological disease (Bressi et al., 2020). For this reason, it is mandatory to assess the specific effects of each new robotic and electromechanical device in all the clinical phases. Another important aim is to develop devices that are easy to use, wear and remove, to provide a relevant improvement of independent access to therapies. In this regard, it is paramount to enforce the synergy between rehabilitation professionals and engineers, by designing shared lines of research and development, both at national and international level. One of the main achievable results of such a collaboration is the possibility of embedding artificial intelligence into home assistant services, exoskeletons, virtual reality applications and also for the management of severe cognitive and mental diseases, such as Alzheimer’s disease (Battista, Salvatore, Berlingeri, Cerasa, & Castiglioni, 2020; Fabrizio, Termine, Caltagirone, & Sancesario, 2021; Liu et al., 2018; Seo, Jang, & Lee, 2022). Finally, the future generations of robots should be able to express empathy during the interaction with patients, which represents one of the most challenging edges of technological advancement.
4.1Indications from the CICERONE Consensus Conference
Based on these results, the CICERONE Consensus Jury has decided to provide indications, rather than recommendations, for each question raised from the SC and the TSC. Thus, in the following statements the indications for the question number 3 are reported:
• It is a priority to design research studies with the aim of investigating the role of robotic and electromechanical devices in promoting neuroplasticity.
• Research lines should be directed to the development and optimization of available prototypes demonstrating clinical efficacy, with the aim of boosting the certification process in all the international markets.
• The available devices should be compared according to their technical characteristics (e.g. exoskeletons vs. end-effector robots) (Gandolfi et al., 2021).
• A new generation of neurorehabilitation robots should be developed, by collaborative teams of engineers, clinicians and researchers, to improve usability and to better investigate the rationale of their effects.
• New exoskeletons fully adaptable to user morphology and anthropometry, for optimizing their size, encumbrance, workspace, (body-machine) interaction, comfort, should be developed, with the aim of having all-in-one devices.
• New software should be developed to detect voluntary intentions both at cognitive and physical levels, aimed to making the robots as embodied, as possible.
The indications for contents of future research from the CICERONE Consensus Jury are as follows:
• To investigate the combination of different rehabilitation plans to profile the characteristics of patients better responding to robot-based treatment.
• To integrate different technologies (e.g. virtual reality, augmented reality, artificial intelligence) to improve robot usability and adaptability.
• To quantify doses of robot-based therapies, to be tested by significant improvement of learning curves and clinically important difference at outcome measures.
• To predict trajectories of recovery, also by big-data mining, with the aim to customize robot-based treatment to each individual patient.
Finally, need to be acknowledged that indications on research and development from the CICERONE Consensus Conference result also from actual limitations of current application of robotic systems, in real clinical settings. The main issue is the lack of high-quality evidence that the use of robotics is related to better clinical outcomes, than the same intensity of usual rehabilitation care, in neurological conditions. To date, Cochrane evidence are available only for arm recovery (Mehrholz, Pohl, Platz, Kugler, & Elsner, 2018) and walking training after stroke (Mehrholz et al., 2017) and for walking training after spinal cord injury (Mehrholz, Kugler, & Pohl, 2012), but not for neurological conditions as a whole. Other main issues needing new reference frameworks are: the lack of adaptability of robot-based therapy, as compared to manual approach; the lack of accuracy on automatic detection of human behavior and emotions; the lack of integration of other functions than the motor one both for assessment and treatment and the difficulty in quantifying impairments to support patient profiling. All that given, CICERONE indications for future theoretical perspectives would be a paramount contribution for the next generations of robots and users.
The CICERONE Consensus Conference provided a unique opportunity of debating within the Italian neurorehabilitation community, involving the full set of stakeholders in the field. Results from the Consensus has promoted a large exchange of views, fostering all the people involved to share different perspectives and needs. To date, the CICERONE Consensus promoted important evidence on the impact of robotics in stroke (Calabrò, Sorrentino, et al., 2021; Morone, Palomba, et al., 2021), multiple sclerosis (Bowman et al., 2021; Calabrò, Cassio, et al., 2021), Parkinson’s disease (Carmignano et al., 2022; Picelli et al., 2021) and spinal cord injury (Morone, de Sire, et al., 2021). The dissemination of the CICERONE Consensus results is a contribute to national and international perspectives on how to improve functional outcomes of patients living with neurological conditions.
Conflict of interest
The authors have nothing to disclose.
Acknowledgments
Andrea Turolla, Pawel Kiper and Deborah Mazzarotto equally contributed as first authors. All authors, as well as Emanuele Gruppioni, Andrea Giovanni Cutti, Francesco Draicchio, and Rinaldo Sacchetti, are official members of Working Group 3 (“Reference theoretical models, future perspectives, development, and research”) of the Italian “Consensus Conference on Robot-Assisted Rehabilitation for People with Disabilities in Neurological Conditions,” chaired by Andrea Turolla and Franco Molteni (La riabilitazione assistita da robot e dispositivi elettromeccanici per le persone con disabilità di origine neurologica – CICERONE). The authors acknowledge the Italian Society of Physical Medicine and Rehabilitation (Società Italiana di Medicina Fisica e Riabilitazione – SIMFER) and the Italian Society of Neurological Rehabilitation (Società Italiana di Riabilitazione Neurologica – SIRN) for promoting the CICERONE Consensus Conference. Then, the authors acknowledge the Steering Committee, Technical Scientific Committee, Jury and all the Working Groups of the CICERONE Consensus for their valuable discussions and collaboration throughout the whole project.
Funding
The authors report no funding.
References
1 | Armstrong, R. , Hall, B. J. , Doyle, J. , & Waters, E. ((2011) ). Scoping the scope’ of a cochrane review. Journal of Public Health, 33: (1), 147–150. doi: 10.1093/pubmed/fdr015 |
2 | Battista, P. , Salvatore, C. , Berlingeri, M. , Cerasa, A. , & Castiglioni, I. ((2020) ). Artificial intelligence and neuropsychological measures: The case of Alzheimer’s disease. Neurosci Biobehav Rev, 114: , 211–228. doi: 10.1016/j.neubiorev.2020.04.026 |
3 | Boldrini, P. , Bonaiuti, D. , Mazzoleni, S. , & Posteraro, F. ((2019) ). Rehabilitation assisted by robotic and electromechanical devices for persons with neurological disabilities: An Italian consensus conference. Functional Neurology, 34: (2), 123–124. |
4 | Boldrini, P. , Bonaiuti, D. , Mazzoleni, S. , & Posteraro, F. ((2021) ). Rehabilitation assisted by robotic and electromechanical devices for people with neurological disabilities: contributions for the preparation of a national conference in Italy. Eur J Phys Rehabil Med, 57: (3), 458–459. doi: 10.23736/s1973-9087.21.07084-2 |
5 | Bowman, T. , Gervasoni, E. , Amico, A. P. , Antenucci, R. , Benanti, P. , Boldrini, P. , ... Carrozza, M. C. ((2021) ). What is the impact of robotic rehabilitation on balance and gait outcomes in people with multiple sclerosis? A systematic review of randomized control trials. Eur J Phys Rehabil Med, 57: (2), 246–253. doi: 10.23736/s1973-9087.21.06692-2 |
6 | Bressi, F. , Bravi, M. , Campagnola, B. , Bruno, D. , Marzolla, A. , Santacaterina, F. , ... Sterzi, S. ((2020) ). Robotic treatment of the upper limb in chronic stroke and cerebral neuroplasticity: a systematic review. J Biol Regul Homeost Agents, 34: (5 Suppl. 3), 11–44 Technology in Medicine. |
7 | Calabrò, R. S. , Cassio, A. , Mazzoli, D. , Andrenelli, E. , Bizzarini, E. , Campanini, I. , ... Bonaiuti, D. ((2021) ). What does evidence tell us about the use of gait robotic devices in patients with multiple sclerosis? A comprehensive systematic review on functional outcomes and clinical recommendations. Eur J Phys Rehabil Med, 57: (5), 841–849. doi: 10.23736/s1973-9087.21.06915-x |
8 | Calabrò, R. S. , Sorrentino, G. , Cassio, A. , Mazzoli, D. , Andrenelli, E. , Bizzarini, E. , ... Bonaiuti, D. ((2021) ). Robotic-assisted gait rehabilitation following stroke: a systematic review of current guidelines and practical clinical recommendations. Eur J Phys Rehabil Med, 57: (3), 460–471. doi: 10.23736/s1973-9087.21.06887-8 |
9 | Candiani, G. , Colombo, C. , Daghini, R. , Magrini, N. , Mosconi, P. , Nonino, F. , & Satolli, R. (2019). Come organizzare una conferenza di consenso. Manuale metodologico Sistema nazionale per le Linee Guida. |
10 | Carmignano, S. M. , Fundaro, C. , Bonaiuti, D. , Calabro, R. S. , Cassio, A. , Mazzoli, D. , ... Andrenelli, E. (2022). Robot-assisted gait training in patients with Parkinson’s disease: Implications for clinical practice.Asystematic review. NeuroRehabilitation. doi: 10.3233/nre-220026 |
11 | Cheung, V. C. , Piron, L. , Agostini, M. , Silvoni, S. , Turolla, A. , & Bizzi, E. ((2009) ). Stability of musclesynergies for voluntary actions after cortical stroke in humans. Proc Natl Acad Sci U S A, 106: (46), 19563–19568. doi: 10.1073/pnas.0910114106 |
12 | Cheung, V. C. , Turolla, A. , Agostini, M. , Silvoni, S. , Bennis, C. , Kasi, P. , ... Bizzi, E. ((2012) ). Muscle synergy patterns as physiological markers of motor cortical damage. Proc Natl Acad Sci U S A, 109: (36), 14652–14656. doi: 10.1073/pnas.1212056109 |
13 | Fabrizio, C. , Termine, A. , Caltagirone, C. , & Sancesario, G. ((2021) ). Artificial Intelligence for Alzheimer’s Disease: Promise or Challenge? Diagnostics (Basel), 11: (8). 10.3390/diagnostics11081473 |
14 | Gandolfi, M. , Vale, N. , Posteraro, F. , Morone, G. , Dell’orco, A. , Botticelli, A. , ...Italian Consensus Conference on Robotics in, N. ((2021) ). State of the art and challenges for the classification of studies on electromechanical and robotic devices in neurorehabilitation: a scoping review. Eur J Phys Rehabil Med, 57: (5), 831–840. doi: 10.23736/S1973-9087.21.06922-7 |
15 | Gimigliano, F. , Palomba, A. , Arienti, C. , Morone, G. , Perrero, L. , Agostini, M. , ...Italian Consensus Conference on Robotics in, N. ((2021) ). Robot-assisted arm therapy in neurological health conditions: rationale and methodology for the evidence synthesis in the CICERONE Italian Consensus Conference. Eur J Phys Rehabil Med, 57: (5), 824–830. doi: 10.23736/S1973-9087.21.07011-8 |
16 | Jones, T. A. , Allred, R. P. , Adkins, D. L. , Hsu, J. E. , O’Bryant, A. , & Maldonado, M. A. ((2009) ). Remodeling the brain with behavioral experience after stroke. Stroke, 40: (3 Suppl), S136–138. doi: 10.1161/STROKEAHA.108.533653 |
17 | Kleim, J. A. , & Jones, T. A. ((2008) ). Principles of experience-dependent neural plasticity: implications for rehabilitation after brain damage. J Speech Lang Hear Res, 51: (1), S225–239. doi: 10.1044/1092-4388(2008/018) |
18 | Liu, X. , Chen, K. , Wu, T. , Weidman, D. , Lure, F. , & Li, J. ((2018) ). Use of multimodality imaging and artificial intelligence for diagnosis and prognosis of early stages of Alzheimer’s disease. Transl Res, 194: , 56–67. doi: 10.1016/j.trsl.2018.01.001 |
19 | Lo, H. S. , & Xie, S. Q. ((2012) ). Exoskeleton robots for upper-limb rehabilitation: state of the art and future prospects. Med Eng Phys, 34: (3), 261–268. doi: 10.1016/j.medengphy.2011.10.004 |
20 | Louie, D. R. , & Eng, J. J. ((2016) ). Powered robotic exoskeletons in post-stroke rehabilitation of gait: A scoping review. Journal of Neuro Engineering and Rehabilitation, 13: (1). doi: 10.1186/s12984-016-0162-5 |
21 | Mehrholz, J. , Kugler, J. , & Pohl, M. ((2012) ). Locomotor training for walking after spinal cord injury. Cochrane Database Syst Rev, 11: , CD006676. doi: 10.1002/14651858.CD006676.pub3 |
22 | Mehrholz, J. , Pohl, M. , Platz, T. , Kugler, J. , & Elsner, B. ((2018) ). Electromechanical and robot-assisted arm training for improving activities of daily living, arm function, and arm muscle strength after stroke. Cochrane Database Syst Rev, 9: , CD006876. doi: 10.1002/14651858.CD006876.pub5 |
23 | Mehrholz, J. , Thomas, S. , Werner, C. , Kugler, J. , Pohl, M. , & Elsner, B. ((2017) ). Electromechanical-assisted training for walking after stroke., . Cochrane Database Syst Rev, 5: , CD006185. doi: 10.1002/14651858.CD006185.pub4 |
24 | Meng, L. , Huang, D. , Liu, H. H. , & Qu, Y. ((2017) ). Research progress of new technologies in stroke rehabilitation. Chinese Journal of Contemporary Neurology and Neurosurgery, 17: (3), 171–175. doi: 10.3969/j.issn.1672-6731.2017.03.003 |
25 | Morone, G. , de Sire, A. , Martino Cinnera, A. , Paci, M. , Perrero, L. , Invernizzi, M. , ... Straudi, S. ((2021) ). Upper Limb Robotic Rehabilitation for Patients with Cervical Spinal Cord Injury: A Comprehensive Review. Brain Sci, 11: (12). doi: 10.3390/brainsci11121630 |
26 | Morone, G. , Palomba, A. , Martino Cinnera, A. , Agostini, M. , Aprile, I. , Arienti, C. , ... Straudi, S. ((2021) ). Systematic review of guidelines to identify recommendations for upper limb robotic rehabilitation after stroke. Eur J Phys Rehabil Med, 57: (2), 238–245. doi: 10.23736/s1973-9087.21.06625-9 |
27 | Munn, Z. , Stern, C. , Aromataris, E. , Lockwood, C. , & Jordan, Z. ((2018) ). What kind of systematic review should I conduct? A proposed typology and guidance for systematic reviewers in the medical and health sciences. BMC medical research methodology, 18: (1), 5–5. doi: 10.1186/s12874-017-0468-4 |
28 | Ouzzani, M. , Hammady, H. , Fedorowicz, Z. , & Elmagarmid, A. ((2016) ). Rayyan-a web and mobile app for systematic reviews. Syst Rev, 5: (1), 210. doi: 10.1186/s13643-016-0384-4 |
29 | Pavel, M. , Jimison, H. B. , Wactlar, H. D. , Hayes, T. L. , Barkis, W. , Skapik, J. , & Kaye, J. ((2013) ). The role of technology and engineering models in transforming healthcare. IEEE Rev Biomed Eng, 6: , 156–177. doi: 10.1109/rbme.2012.2222636 |
30 | Picelli, A. , Capecci, M. , Filippetti, M. , Varalta, V. , Fonte, C. , R, D. I. C. , ... Nardone, A. ((2021) ). Effects of robot-assisted gait training on postural instability in Parkinson’s disease: a systematic review. Eur J Phys Rehabil Med, 57: (3), 472–477. doi: 10.23736/s1973-9087.21.06939-2 |
31 | Pinto, D. , Garnier, M. , Barbas, J. , Chang, S. H. , Charlifue, S. , Field-Fote, E. , ... Heinemann, A. W. ((2020) ). Budget impact analysis of robotic exoskeleton use for locomotor training following spinal cord injury in four SCI Model Systems. Journal of NeuroEngineering and Rehabilitation, 17: (1). doi: 10.1186/s12984-019-0639-0 |
32 | Poggio, T. , & Bizzi, E. ((2004) ). Generalization in vision and motor control. Nature, 431: (7010), 768–774. doi: 10.1038/nature03014 |
33 | Reinkensmeyer, D. J. , Wolbrecht, E. , & Bobrow, J. (2007). A computational model of human-robot load sharing during robot-assisted arm movement training after stroke. Conference proceedings :... Annual International Conference of the IEEE Engineering in Medicine and Biology Society. IEEE Engineering in Medicine and Biology Society. Conference, 2007, 4019-4023. |
34 | Scott, S. H. , & Dukelow, S. P. ((2011) ). Potential of robots asnext-generation technology for clinical assessment of neurologicaldisorders and upper-limb therapy. Journal of RehabilitationResearch and Development, 48: (4), 335–354. doi: 10.1682/JRRD.2010.04.0057 |
35 | Seo, Y. , Jang, H. , & Lee, H. ((2022) ). Potential Applications of Artificial Intelligence in Clinical Trials for Alzheimer’s Disease. Life (Basel), 12: (2). doi: 10.3390/life12020275 |
36 | Team, T. E. ((2013) ). EndNote (Version EndNote X8) [64 bit]. Philadelphia, PA: Clarivate. |
37 | Ting, L. H. , Chiel, H. J. , Trumbower, R. D. , Allen, J. L. , McKay, J. L. , Hackney, M. E. , & Kesar, T. M. ((2015) ). Neuromechanical principles underlying movement modularity and their implications for rehabilitation. Neuron, 86: (1), 38–54. doi: 10.1016/j.neuron.2015.02.042 |
38 | Turchetti, G. , Labella, B. , Bellelli, S. , Cannizzo, S. , Palla, I. , Mazzoleni, S. , ... Guglielmelli, E. ((2009) ). Innovation in rehabilitation technology: Technological opportunities and socioeconomic implications –A theoretical model. International Journal of Healthcare Technology and Management, 10: (4-5), 245–261. doi: 10.1504/IJHTM.2009.030449 |
39 | Wade, E. , & Winstein, C. J. ((2011) ). Virtual reality and robotics for stroke rehabilitation: Where do we go from here? Topics in Stroke Rehabilitation, 18: (6), 685–700. doi: 10.1310/tsr1806-685 |
40 | Wang, R. H. , Sudhama, A. , Begum, M. , Huq, R. , & Mihailidis, A. ((2017) ). Robots to assist daily activities: Views of older adults with Alzheimer’s disease and their caregivers. International Psychogeriatrics, 29: (1), 67–79. doi: 10.1017/S1041610216001435 |
41 | Weinrich, M. ((2006) ). National Institutes of Health support of rehabilitation robotics research. Journal of Rehabilitation Research and Development, 43: (5), 5, xxi–xxii. doi: 10.1682/JRRD.2006.06.0073 |
42 | Weinrich, M. , Good, D. C. , Reding, M. , Roth, E. J. , Cifu, D. X. , Silver, K. H. , ... Flax, H. J. ((2004) ). Timing, Intensity, and Duration of Rehabilitation for Hip Fracture and Stroke: Report of a Workshop at the National Center for Medical Rehabilitation Research. Neurorehabilitation and Neural Repair, 18: (1), 12–28. doi: 10.1177/0888439003262041 |
43 | Whitall, J. ((2004) ). Stroke Rehabilitation Research: Time to Answer More Specific Questions? Neurorehabilitation and Neural Repair, 18: (1), 3–11. |
44 | Wolff, J. , Parker, C. , Borisoff, J. , Mortenson, W. B. , & Mattie, J. ((2014) ). A survey of stakeholder perspectives on exoskeleton technology. Journal of NeuroEngineering and Rehabilitation, 11: (1). doi: 10.1186/1743-0003-11-169 |
45 | Wolpert, D. M. , Diedrichsen, J. , & Flanagan, J. R. ((2011) ). Principles of sensorimotor learning. Nat Rev Neurosci, 12: (12), 739–751. doi: 10.1038/nrn3112 |