People with persistent postural-perceptual dizziness demonstrate altered postural strategies in complex visual and cognitive environments
Abstract
BACKGROUND:
People with PPPD report imbalance, increase in symptoms and impaired function within complex visual environments, but understanding of the mechanism for these behaviors is still lacking.
OBJECTIVE:
To investigate postural control in PPPD we compared changes in center of pressure (COP) and head kinematics of people with PPPD (N = 22) and healthy controls (N = 20) in response to different combinations of visual and cognitive perturbations during a challenging balance task.
METHODS:
Participants stood in a tandem position. Static or moving stars (0.2 Hz, 5 mm or 32 mm amplitude, anterior-posterior direction) were displayed through a head-mounted display (HTC Vive). On half the trials, participants performed a serial-3 subtraction task. We measured medio-lateral and anterior-posterior path and acceleration of COP and head.
RESULTS:
Controls significantly increased all COP and head parameters with the cognitive task whereas PPPD increased only COP ML path and acceleration. Only controls significantly increased head anterior-posterior & medio-lateral acceleration with moving visual load. Cognitive task performance was similar between groups.
CONCLUSIONS:
We observed altered postural strategies in people with PPPD, in the form of reduced movement with challenge, particularly around the head segment. The potential of this simple and portable head-mounted display setup for differential diagnosis of vestibular disorders should be further explored.
1Introduction
Persistent postural-perceptual dizziness (PPPD) has been defined by the Bárány Society [46] as having chronic symptoms (over 3 months) of dizziness, instability or both. In people with PPPD, laboratory tests are typically normal. Understanding the mechanism of instability in people with PPPD and, in particular, identifying objective markers of PPPD, could drive development of clinical balance assessments and effective balance rehabilitation programs. The diagnosis of PPPD replaced precursor diagnoses such as phobic postural vertigo (PPV) and chronic subjective dizziness. People with PPV, unlike healthy young adults, have been shown to reduce their movement in order to minimize postural instability under challenging balance conditions [35]. Indeed, Schniepp et al. [39], showed increased muscle co-contraction in individuals with PPV which they attributed to conscious movement processing, i.e., excessive internal focus of attention. On the other hand, people with chronic subjective dizziness sway more than healthy controls with sensory perturbations due to difficulty in sensory reweighting [10] and increased visual reliance for balance [14, 26, 31, 44]. People with PPPD report imbalance, increase in symptoms and impaired function within complex visual environments [26, 40], but the understanding of the mechanism for these behaviors is still lacking.
Cognitive-motor dual-tasking manifests in many balance activities of daily living. Postural sway has been shown to increase under dual-task conditions compared with single-task performance, particularly when either task (balance [1] or cognitive [13]) is more challenging, or when cognitive capacity is reduced due to age [48, 56] or neurological conditions [28, 33]. That is because the combination of increased balance challenge and cognitive load might lead to an attentional resource competition [51]. People with PPPD may need to allocate more attention to their balance. Thus, due to limited attentional resources, they may increase sway when balancing with an additional cognitive load. Nevertheless, mental distraction via a secondary cognitive task has also been shown to reduce sway in healthy adults [11, 30, 47] regardless of task type or difficulty, [8] as also been seen in people with PPV [52]. This was explained by the automaticity of postural control: since postural control is primarily controlled automatically, allocating excessive cognitive resources to control balance (i.e., internal focus of attention) may change with balance performance [15, 36]. Accordingly, if balance control in people with PPPD is governed by conscious movement processing and increased stiffening with challenge, performing a cognitive task may improve their balance performance because it will allow for a more automatic postural control.
Head-mounted displays provide diverse visual environments to study specific responses to mild visual perturbations [20, 21] in an ecologically valid and clinically applicable manner. Head-mounted displays also allow for a detailed analysis of head kinematics and was previously shown to be more sensitive to vestibular dysfunction [22, 23] or visual vertigo [17, 18] as compared with postural sway or EMG of the trunk or lower limbs during dynamic visual perturbations. Higher segments (such as center-of-mass) accelerations (rate of velocity change per unit of time) are known to be sensitive metrics of postural control [50]. Indeed, head acceleration is a critical element for the control of head movements [4]. Head-mounted display paradigms can therefore shed light on the mechanism governing balance deficits in people with PPPD and differences in stabilization of upper segments, such as the head, versus lower segments, such as the center of pressure, in these patients.
The current study aimed to identify determinants of postural and head control in people with PPPD, and the manner in which they vary with sensorimotor and cognitive load. The specific questions of this study were:
1) Evaluate postural sway in individuals with PPPD during a challenging balance task under complex visual and cognitive conditions as compared with healthy adults.
2) Evaluate head kinematics in individuals with PPPD during a challenging balance task under complex visual and cognitive conditions as compared with healthy adults.
3) Compare performance on a cognitive task between individuals with PPPD and healthy adults.
Based on related work [19], we expected healthy adults to demonstrate increased postural sway and head movement and acceleration when adding the cognitive task and increased head acceleration with increased visual load.
2Methods
2.1Sample
Participants were 18 or older with normal vision and peripheral sensation. Participants with PPPD were diagnosed according to the ICD-11/Bárány Society diagnostic criteria as confirmed on a phone interview [46]. They self-reported feelings of movement, dizziness, unsteadiness or light-headedness that lasted for hours or days for at least 3 months prior to the study. Their symptoms were exacerbated by self-upright movement and / or busy visual environments. All participants underwent the following diagnostic process: They were first seen by a primary care physician that performed routine blood tests to rule out anemia or low blood sugar, as well as electrocardiogram to rule out a cardiac problem. They were then seen by a neurologist and underwent brain and inner ear imaging (CT or MRI) with no clinical findings. Finally, they were seen by an Ear, Nose, And Throat (ENT) Specialist and were found to have negative Videonystagmography (VNG) and / or Video Head Impulse Test (vHIT), normal audiograms, and negative bed-side testing including Head Thrust; Head Shaking; and Gaze-evoked or Spontaneous Nystagmus. In addition, clinical examination confirmed normal smooth pursuit, saccades, sense of light touch and static visual acuity. While some participants had a history of migraines or Benign Paroxysmal Positional Vertigo, current other vestibular disorders, including migraine, were ruled out as a cause for the patients’ symptoms based on the clinical exam and interview. Participants with PPPD were excluded for active neuro-otologic disorders besides PPPD, and new medication use, or recent dosage change less than one month before participation (according to the interview findings). Partici-pants in both groups were excluded for pregnancy, uncorrected visual impairments (screened on Early Treatment Diabetic Retinopathy Study chart to confirm vision better than the cutoff 20/63, the NYS Department of Motor Vehicle cutoff for driving), history of drug/alcohol abuse, and all other conditions that may affect standing or walking including neurological conditions; use of an assistive device; active musculoskeletal pain; or peripheral neuropathy (screened for normal protective sensation at the bottom of the feet by the ability to detect 5.07 g monofilament) [37].
2.2Procedure
This observational cross-sectional study took place at the university of Haifa, Department of Physical Therapy. The study was approved by the University of Haifa’s Institutional Review Board. Participants first signed informed consent. They then underwent a sensory systems screen including: Early Treatment Diabetic Retinopathy Study (ETDRS) chart confirming normal vision (cutoff 20/63, the NYS Department of Motor Vehicle cutoff for driving), a 5.07 monofilament screen confirming normal protective sensation at the bottom of their feet [37], smooth pursuit and saccades to rule out an oculomotor impairment. The participants performed the Timed Up and Go test (TUG) twice and we recorded the better performance out of the 2. For the TUG, a participant rises up from a chair, walks 10 feet in their comfortable speed, turns around a cone, walks back and sits back on the chair [42]. Participants completed the following questionnaires: A demographics questionnaire, The Montreal Cognitive Assessment (MoCA) [25], The Trail Making Test (TMT) A and B [38] and the State-Trait Anxiety Inventory [45]. The MoCA is a quick screening tool for mild cognitive impairments assessing attention and concentration, executive functions, memory, language, and calculations. The TMT evaluates attention, visual screening ability and processing speed. In section A, participants are required to trace sequential numbers, whereas in section B they are required to trace alternating numbers and letters. To evaluate potential cybersickness, participants were also interviewed on Kennedy’s Simulator Sickness Questionnaire [16] during the session.
For the balance protocol, participants were standing in a tandem (‘heel-to-toe’) position with the non-dominant leg in the back on an AMTI AccuSway (AMTI, Watertown, MA) force platform. A quad cane was placed on either side of the participant, and a researcher was guarding the participant from behind. Participants were wearing the HTC Vive (HTC Corporation, Taoyuan City, Taiwan) head-mounted display and observed 3 virtual environments, for 20 seconds each. For a detailed description of the scenes see Lubetzky et al [21]. Briefly, the virtual environment consisted of a 3-wall display (each wall 6.16 meters by 3.2 meters) of spheres. The walls were either static or moving in the anterior-posterior direction at a frequency of 0.2 Hz and an amplitude of either 5 mm or 32 mm, referred to as AP5 and AP32 respectively [29]. The instructions to the participants were: “Look straight ahead and try to stay steady. If you lose your balance go back to the position. Try not to use the canes but they are here if you need them.” On half of the trials, participants were asked to complete a serial-3 subtraction task [7] while maintaining the tandem position: “count backwards out loud in jumps of 3 from a number that I tell you. Start from the next number. For example, if I say 557, you start: 554, 551 and so on”. The serial subtraction task is an articulation cognitive task and as such, is particularly likely to increase postural sway [9] and even more likely to increase head movement because of the mobility of the jaw. All trials that included the serial subtraction task (dual-task) were audio recorded by a mobile phone for offline processing. The protocol began with performing each task separately: cognitive task in sitting and single-task balancing in a static visual environment (performed once). After that all scenes appeared twice in a randomized order (Static, AP5, AP32 single-task or dual-task). This protocol was part of larger protocol that included also a dynamic postural control task [3], which was always performed after the static task and the results of which are reported elsewhere [2]. See Fig. 1 for an illustration of the experimental setup.
Fig. 1
Experimental setup: A participant is standing on a force-platform in a tandem (heel-to-toes) position for 20 seconds while wearing the HTC Vive headset. He / she is asked to maintain their balance when observing spheres that are either static or moving anteroposterior at a frequency of 0.2 Hz and an amplitude of 5 or 32 mm. On half the trials, the participant, out loud, repeatedly subtracts 3 starting from a 3-digit number.
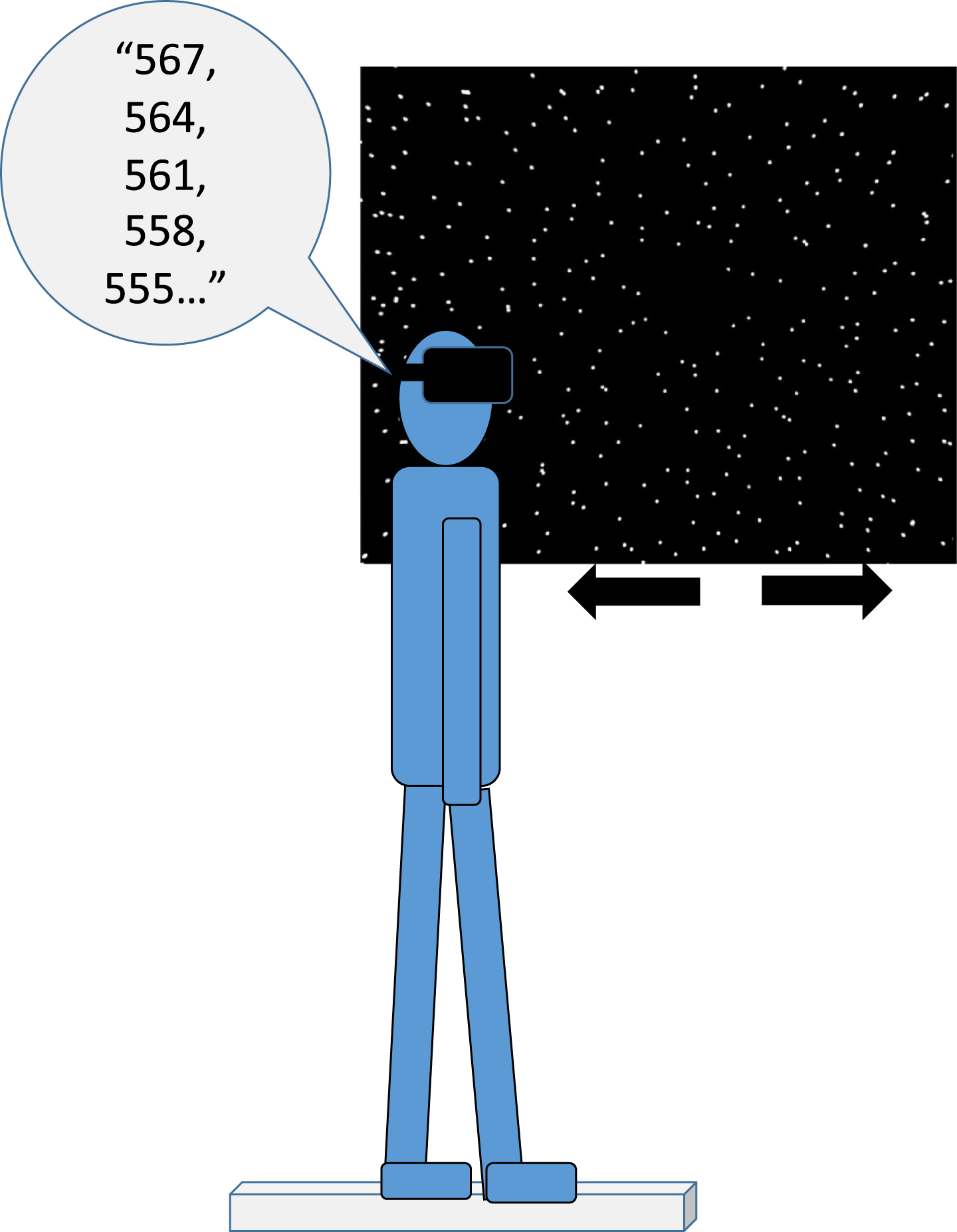
2.3Data reduction and outcome measures
Postural sway (center of pressure [COP] from the force-platform) was recorded at 100 Hz and head kinematics was recorded at 90 Hz using custom-written software for the HTC Vive headset. Subseq-uent analyses were performed in Matlab (Mathworks ltd., Natick, MA). We applied a low-pass 4th order Butterworth filter with a conservative cutoff frequency at 10 Hz [43]. We calculated the following outcome measures for 2 directions of force-platform and head data (anterior-posterior, medio-lateral):
Directional Path (Path, cm) [34]: the total path length of the position curve for a selected direction.
Root Mean Square Acceleration (Acceleration, cm/s2) [23]: The square root of the average of the square of the acceleration over time.
Data from two trials in each condition were averaged. Concurrent validity of the HTC Vive as compared with a gold-standard motion capture system has been established for these head kinematics measures in healthy young adults [24].
For performance on the cognitive task, the numbers told correctly were processed from audio files.
2.4Statistical analysis
Demographic variables and dependent measures were described using descriptive statistics. Indepen-dent-sample T tests, Mann-Whitney U tests and χ2 tests were used to test for between-group differences on demographic variables and functional tests according to their distributions type. In order to evaluate changes in postural sway (question 1) and head kinematics (question 2) in people with PPPD and controls across visual and cognitive load conditions, for each outcome measure (COP and head: Path, Acceleration; anterior-posterior and medio-lateral) we ran a mixed model repeated-measures ANOVA with 2 within-subjects’ factors: visual (3 levels: Static, AP5, AP32) and task (2 levels: single, dual) separately per group. Between-group differences were explored using independent sample T tests. In order to compare performance on the cognitive task between groups (question 1), and since numbers told correctly were normally distributed both when standing (Standing Count) and seated (Seated Count), we ran a mixed model repeated measures analysis-of-variance (ANOVA) for standing count with 1 within-subjects factor: task (4 levels: sitting and the 3 visual conditions in standing) and 1 between-subjects factor: group (2 levels). When Mauchly’s test of sphericity was significant, we reported the P value of the Greenhouse-Geisser correction. The α level was set at 0.05 and Bonferroni corrections were applied to all within-subjects’ contrasts. We report the ANOVA’s Partial Eta Squared (PES) as an estimate of effect size. Data processing was done in Matlab R2019b (MathWorks, MA, USA). All statistical analysis was done in SPSS version 25 IBM (NY, USA). Figures created in SPSS and edited in Inkscape 0.92.4.
3Results
3.1Sample
Description of the sample (20 controls, 22 participants with PPPD) appears in Table 1. On average, participants with PPPD reported symptom duration of 5.6 years (SD = 7.8, min = 0.4, max = 30). Symptoms appeared suddenly in 16 participants, gradually in 2 and 4 did not report. Participants reported several different precipitating events to symptom onset including stress (N = 5), panic attack (N = 3), a flu (N = 3), an episode of BPPV (N = 2), a bumpy flight/bus ride (N = 3), giving birth (N = 2) and an episode of alcohol consumption (N = 1). Three participants reported migraine as a comorbidity. The groups were comparable in age, sex, weight and height. Performance on the TUG was similar between groups and better than the 11.1 seconds cutoff for increased fall risk in adults with vestibular dysfunction [49]. A small but significant between-group difference was observed on the MoCA (participants with PPPD scored on average 1.9 points lower than controls). In addition, a significantly faster performance by the control group was observed on the TMT B though the performance of neither group was considered poor [38]. The PPPD group also showed significantly higher trait and state anxiety and Simulator Sickness values before and after testing (Table 1).
Table 1
Description of the sample. Values in the table are Mean (SD) for normally distributed variables; Median [Minimum, Maximum] for skewed variables, and count (%) for sex
Variable | Controls (n = 20) | PPPD (n = 22) | P value |
Age | 39 [21, 66] | 32 [21, 66] | 0.35a |
Sex | 12 women (60%) | 10 women (47.6%) | 0.54b |
Weight (Kg) | 70.25 (14.87) | 68.48 (13.37) | 0.69c |
Height (cm) | 168.55 (8.79) | 169.62 (9.64) | 0.71c |
TUG (seconds, best out of 2 trials) | 7.6 (1.44) | 8.47 (1.32) | 0.053c |
MoCA | 27.8 (1.76) | 25.9 (1.61) | 0.001c |
TMT A | 26.9 [14.8, 61.3] | 27.3 [15.3, 66.8] | 0.68a |
TMT B | 50.7 [31.8, 140.6] | 68.3 [36.4, 122.9] | 0.03a |
Trait Anxiety | 36.1 (7.9) | 50.43 (11.67) | < 0.001c |
State Anxiety | 32.15 (8.91) | 41.14 (10.18) | 0.004c |
SSQ baseline* | 0 [0, 0] | 6 [1, 12] | < 0.001a |
SSQ post-testing | 3 [0, 12] | 7 [2, 26] | 0.006a |
aMann-Whitney U test. bχ2 test. cIndependent-sample T test. TUG: Timed-Up and Go; MoCA: Montreal Cognitive Assessment; TMT: Trail Making Test; SSQ: Simulator Sickness Questionnaire. *Note that the statistical comparison was made for the 9 participants with PPPD who were tested at baseline.
3.2Question 1: Postural sway
In the medio-lateral direction, COP path was larger when performing the cognitive task for both groups (control: F1,20 = 16.54, P = 0.001, PES = 0.45; PPPD: F1,20 = 6.59, P = 0.018, PES = 0.25) with larger increases with the cognitive task in the control group (See Fig. 2). Similarly, both groups significantly increased their COP acceleration in the medio-lateral direction when performing the cognitive task, with no effect of the visual scene (control: F1,20 = 8.44, P = 0.009, PES = 0.3; PPPD: F1,20 =4.41, P = 0.049, PES = 0.18). In the antero-posterior direction, differences in COP path were found only for controls (task: F1,20 = 7.76, P = 0.01, PES = 0.28; visual: F2,40 = 4.35, P = 0.02, PES = 0.18 with no interaction, Fig. 2). Specifically, higher COP antero-posterior path was found for AP32 vs. AP5 (P = 0.012), and a trend towards COP higher path for AP32 vs. Static (P = 0.059). In the antero-posterior direction, only controls increased COP acceleration with the cognitive task (F1,20 = 6.4, P = 0.02, PES = 0.24), but not with the visual load (Fig. 2).
Fig. 2
Box plots (median and inter-quartile range) of center of pressure (COP) Directional Path (cm, top) and Root Mean Square Acceleration (Acceleration, cm/s2, bottom) in the mediolateral direction (medio-lateral, left hand side) or the anteroposterior direction (anterior-posterior, right hand side) for the control (denoted in white) and PPPD (denoted in grey) groups given the visual load (Static, 5 mm amplitude [AP5], 32 mm amplitude [AP32]) and task (single-task, dual-task). * denotes a significant main effect at P < 0.05. ** denotes a significant main effect at P < 0.01. Dashed lines represent a significant effect for both groups and solid lines represent a significant effect in the control group only. Both groups significantly increased COP path and Acceleration medio-lateral with dual-task. The control group, but not the PPPD group, significantly increased COP path anterior-posterior with task and visual and Acceleration anterior-posterior with task.
![Box plots (median and inter-quartile range) of center of pressure (COP) Directional Path (cm, top) and Root Mean Square Acceleration (Acceleration, cm/s2, bottom) in the mediolateral direction (medio-lateral, left hand side) or the anteroposterior direction (anterior-posterior, right hand side) for the control (denoted in white) and PPPD (denoted in grey) groups given the visual load (Static, 5 mm amplitude [AP5], 32 mm amplitude [AP32]) and task (single-task, dual-task). * denotes a significant main effect at P < 0.05. ** denotes a significant main effect at P < 0.01. Dashed lines represent a significant effect for both groups and solid lines represent a significant effect in the control group only. Both groups significantly increased COP path and Acceleration medio-lateral with dual-task. The control group, but not the PPPD group, significantly increased COP path anterior-posterior with task and visual and Acceleration anterior-posterior with task.](https://content.iospress.com:443/media/ves/2021/31-6/ves-31-6-ves201552/ves-31-ves201552-g002.jpg)
No between-group differences were found in the mediolateral direction for path or acceleration. (Fig. 2). In the antero-posterior direction, PPPD had a larger COP path in the static visual scene (single-task) condition (P = 0.03, mean difference 23.34 cm, 95% CI 2.1, 44.57). The PPPD group also had significantly higher COP acceleration in the static visual scene (single-task) (P = 0.03, mean difference 49.6, 95% CI 6.67, 92.53); AP5 single-task (P = 0.02, mean difference 33.82, 95% CI 5.7, 61.94) and AP32 dual-task (P = 0.02, mean difference 34.25, 95% CI 6.93, 61.57). Descriptive statistics for all outcomes appear in Appendices A & B.
3.3Question 2: Head kinematics
Head kinematics did not vary between conditions in the PPPD group. For controls, performance of the cognitive task was associated with increased head path movement in medio-lateral (F1,18 = 6.1, P = 0.024, PES = 0.25) and anterior-posterior (F1,18 =4.59, P = 0.046, PES = 0.2) directions, with no effect of visuals (Fig. 3). Only controls significantly increased head acceleration in both directions with the cognitive task (medio-lateral: F1,18 = 5.69, P =0.028, PES = 0.24; anterior-posterior: F1,18 = 4.78, P = 0.04, PES = 0.21), and with the moving visual scene (medio-lateral: F2,36 = 6.45, P = 0.005, PES =0.26, AP32 vs. Static P = 0.001; anterior-posterior: F1.3,23.28 = 7.3, P = 0.008, PES = 0.29, AP32 vs. Static P = 0.003) with no visual by task interaction.
Fig. 3
Box plots (median and inter-quartile range) of head Directional Path (cm, top) and Root Mean Square Acceleration (Acceleration, cm/s2, bottom) in the mediolateral direction (medio-lateral, left hand side) or the anteroposterior direction (anterior-posterior, right hand side) for the control and PPPD groups given the visual load (Static, 5 mm amplitude, 32 mm amplitude) and task (single task, dual task). * denotes a significant main effect at P < 0.05. ** denotes a significant main effect at P < 0.01. Dashed lines represent a significant effect for both groups and solid lines represents a significant effect in the control group only. Controls significantly increased head path medio-lateral and anterior-posterior with the task. Controls significantly increased head acceleration medio-lateral and anterior-posterior with the task and visual. No significant main effects were seen for the PPPD group.
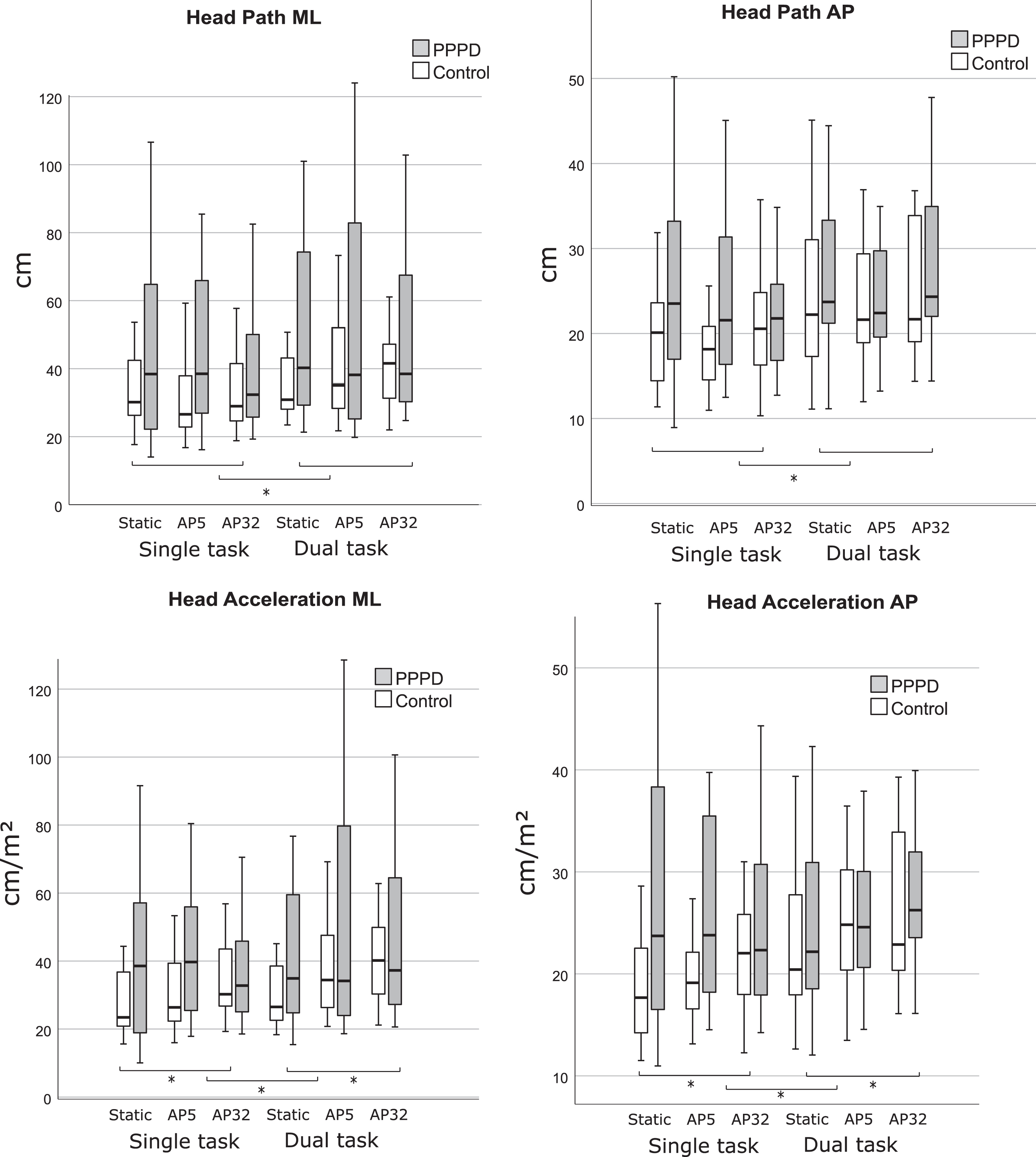
Between-group comparison showed that the groups were different on the least complex scenes only (Fig. 3). Namely, the PPPD group was significantly higher (P < 0.05) on all head outcome measures for the static single-task scene, and on all outcome measures but head acceleration medio-lateral on the AP5 single-task scene (See Fig. 3 and Appendix A & B).
3.4Question 3: Cognitive task performance
Numbers told correctly (Count) did not differ between groups on baseline or during the balance task. No main effect of task was observed for Count in either group. Both groups had an average of 10 Count in sitting (95% CI 8.3, 11.9). The PPPD group had an average count of 9.2 in all standing tasks (95% CI 7.5, 11).
4Discussion
We studied postural responses to combinations of visual and cognitive load in adults with PPPD and healthy controls via a virtual reality paradigm using a head-mounted display. Our purpose was to shed light on the balance controlling mechanisms in people with PPPD, a relatively new diagnosis derived from chronic subjective dizziness and PPV and explore the ability to quantify postural strategies in this population using both COP and head movement. Our findings show that people with PPPD moved their head and COP more than controls on easier tasks, but this difference decreased as the challenge increased. This is in agreement with previous studies showing altered performance (more sway) on the sensory organization test (SOT) for individuals with PPPD compared to individuals with other vestibular conditions, particularly on the simpler SOT conditions, such as standing with eyes closed on a stable surface [44]. At the same time, controls in the current study had larger responses to cognitive and visual perturbations than PPPD, particularly around the head segment and anterior-posterior postural sway. COP changes in controls in the anterior-posterior direction may reflect a normal response to the visual perturbations which were in this plane. In contrast, the similarity between groups on COP parameters in the medio-lateral direction may have been driven by the tandem standing position which reduces the base of support in this direction [6]. These findings support the assumption that postural strategy of people with PPPD is characterized by reduced movement in response to perturbations, particularly around the head segment.
Performance on the cognitive task was comparable between sitting and standing in both groups suggesting that both groups prioritized the cognitive task when standing. Wuehr et al., similarly found that cognitive performance (naming items from a given category) did not differ between healthy controls and patients with PPV or between conditions [52]. They also found that patients with PPV had significantly higher co-contraction around the ankle in eyes open or closed and larger postural sway during single-task which was reduced to a level similar to controls under dual-task. They thus suggested that performing a cognitive task ‘normalized’ postural control in people with PPV by taking away their conscious monitoring of postural adjustment, making the control of balance more automatic. In our work, while adding a cognitive task indeed reduced the differences between the groups, so did adding a visual load (AP32). In addition, and in contrast with the result of Wuehr et al., in our work, the reduced between-group difference was driven by increased postural sway and head kinematics with dual-task and visual load in controls with no such change in the PPPD group. Finally, the PPPD group remained higher on COP anterior-posterior acceleration on all conditions, particularly on AP32 dual-task. High COP anterior-posterior accelerations may reflect increased work of the muscles around the ankle (namely soleus, tibialis anterior) to avoid excessive movement of higher segments and maintain a stable position. Differences between our work and Wuehr may be explained by a more challenging balance task (tandem vs normal stance), and the immersive visual perturbations within a head-mounted display. First, tandem stance may require further adjustments around the ankle to avoid loss of balance, particularly among people with PPPD. Second, different levels of difficulty of the cognitive task in both studies may underscore the different effects of the cognitive dual-task on postural control. Specifically, the serial subtraction task is a mental tracking task [5] which is relatively challenging for multiple populations. According to model of dual-task prioritization [54], when faced with dual-task situations an individual is required to prioritize performance of one task over the other. When overall capacity for performance is limited, prioritizing one task (e.g., the cognitive task) may result in altered performance of the other task. Indeed, in the current work, scores of the PPPD group on the cognitive tests (MoCA, TMT) were lower than those of controls, alluding to potentially limited capacity for dual-task performance. While their performance on the cognitive task did not differ, it may be that this limited capacity is associated with the different effects of the added cognitive task on motor performance between groups. Third, the testing conditions in our study, i.e., immersive virtual environments, may have led to increased anxiety in people that was not fully reduced with the cognitive distraction. In related work [2], during a dynamic task, we observed reduced head movement with increased trait anxiety in individuals with PPPD but the opposite pattern in healthy controls. Anxiety is known to modify movement under dual-task conditions in other populations as well. For example, individuals with chronic low back pain and high pain-related anxiety demonstrated reduced postural sway under dual-task conditions compared with those without anxiety and healthy controls [41]. Finally, observing virtual environments via a head-mounted display has been shown to be more challenging than closing the eyes for people with vestibular dysfunction [22] and is more likely to evoke symptoms in people with motion sensitivity. It is therefore possible that individuals with PPPD attempted to reduce their head responses to perturbations to avoid worsening of symptoms within the virtual environment. Indeed, we observed higher Simulator Sickness scores in the PPPD group compared with controls. Alternatively, it may be that the lack of increase in head movement, which we observed both under visual and cognitive load conditions, is a strategy aimed to facilitate better performance of the motor task under potentially more challenging conditions. Explanatory factors of these altered postural strategies in the form of reduced response to perturbations and its implications to rehabilitation of people with PPPD should be investigated in future research.
While PPPD shares symptomatology with other vestibular disorders, individuals with vestibular dysfunction typically show increased postural sway with visual or somatosensory perturbations [27] due to inadequate sensory substitutions [21]. However, our work suggests that the postural behavior of people with PPPD is characterized by reduction of movement, like in PPV, rather than difficulty in sensory reweighting. Like other studies that showed high-risk postural control strategy of stiffening at the ankles [39, 53] we found increased COP anterior-posterior acceleration in people with PPPD without an increase in path, as well as overall reduced responsiveness to external perturbations, particularly around the head segment. These findings should be further contrasted with results from people with structural vestibular disorders. Lubetzky and Hujsak showed increased head movement in all directions in individuals with unilateral peripheral vestibular hypofunction in a similar visual environment (AP32) and the same balance position (tandem) in a single-task condition [22]. Thus, head movement during challenging postural tasks appears to differ between people with unilateral vestibular hypofunction and those with PPPD. Note that the people with PPPD also tended to be more symptomatic within the virtual environments than those in Lubetzky and Hujsak’s study [22].
Our findings indicate that for postural control, head kinematics provide complementary information to the laboratory forceplate. Young et al. observed that participants with compensated vestibular loss, as well as healthy controls, adopted a rigid stance when standing at height. This postural strategy was observed in higher segments, such as the head, more than via COP. It appears that participants stabilized their head over their feet, potentially to reduce their limits of stability [55]. It is possible that head patterns provide a more sensitive characteristic of vestibular dysfunction, either structural as in previous work or functional as in the current work, than postural sway [22, 23]. Young et al., recruited participants who compensated for their vestibular loss and indeed controlled their balance similar to healthy controls. In other studies, individuals with bilateral vestibular deficits showed different head rotation patterns than control while moving in darkness [32]. Keshner et al., [18] observed that head kinematics in response to visual perturbation could represent strategies of head and trunk stability in visually-sensitive individuals. In a study by Hoppes et al. [12], individuals with vestibular dysfunction showed significantly more head sway than controls when observing optic flow (measured by receivers placed on the head). Perhaps patients with PPPD chose a postural control strategy that minimizes head movement, to facilitate overall more stable performance via the COP. If that is the case, then altered sway patterns across different body segments may be a marker of PPPD. HMDs thus open the window to studying head movement in response to controlled manipulations of the environmental conditions in a systematic manner with potential direct clinical implications to differential diagnosis.
Limitations of this study include multiple statistical tests performed with a small sample and no a-priori power calculation; therefore, all findings need to be interpreted with caution. In addition to replication in a larger sample, future research should run a direct comparison between people with PPPD and other vestibular disorders to determine whether a dual-task HMD paradigm could be used clinically as a quick, non-invasive tool for differential diagnosis. Based on the current study and our previous work we expect more symptoms and reduced response to perturbations in the PPPD group compared to unilateral vestibular hypofunction.
In conclusion, balance responses to cognitive and visual load varied between people with PPPD and controls such that controls demonstrated increased movement with increased task difficulty and the PPPD group did not. These altered postural strategies were particularly observed around the head segment. Head-mounted displays thus appear to provide adequate input and output to study postural responses in people with PPPD. Once these findings are replicated in larger samples and extended to other vestibular disorders, the portability and simplicity of the HMD setup could allow for a simple clinical translation and could potentially serve for differential diagnosis of vestibular disorders.
Conflicts of interest and source of funding
The authors report no conflict of interest. Dr. Lubetzky was funded by grants from the National Institutes of Health National Rehabilitation Research Resource to Enhance Clinical Trials (REACT) pilot award and an Emerging Research Grant from Hearing Health Foundation. The sponsors had no role in the study design, collection, analysis and interpretation of data; in the writing of the manuscript; or in the decision to submit the manuscript for publication. The content is solely the responsibility of the authors and does not necessarily represent the official views of the National Institutes of Health.
Acknowledgments
We thank Zhu Wang, PhD candidate, NYU Courant Future Reality Lab and Tandon School of Engineering, for the development of the testing applications. We thank Maya Goldman and Nirit Di-Porto of the University of Haifa for their assistance with data collection. We thank the Edmond J. Safra Brain Research Center for the Study of Learning Disabilities for their support.
References
[1] | Aguiar S.A. , Gramani-Say K. , Lopes A.G. and Barela J.A. , Dual task interferes with sensorimotor coupling in postural control, Psych Neurosci 7: ((2014) ), 593–599. |
[2] | M.M.H. Aharoni, A.V. Lubetzky, L. Arie and T. Krasovsky, Factors associatedwith dynamic balance in people with Persistent Postural Perceptual Dizziness (PPPD): a crosssectional study using a virtual-reality Four Square Step Test, J NeuroengRehabil. To Appear. |
[3] | Aharoni M.M.H. , Lubetzky A.V. , Wang Z. , Goldman M. and Krasovsky T. , A Virtual Reality Four-Square Step Test for Quantifying Dynamic Balance Performance in People with Persistent Postural Perceptual Dizziness, in: 2019 International Conference on Virtual Rehabilitation (ICVR), 2019: pp. 1–6. |
[4] | Allum J.H. , Gresty M. , Keshner E. and Shupert C. , The control of head movements during human balance corrections, J Vestib Res 7: ((1997) ), 189–218. |
[5] | Al-Yahya E. , Dawes H. , Smith L. , Dennis A. , Howells K. and Cockburn J. , Cognitive motor interference while walking: a systematic review and meta-analysis, Neurosci Biobehav Rev 35: ((2011) ), 715–728. |
[6] | Black F.O. , Wall C. , Rockette H.E. and Kitch R. , Normal subject postural sway during the romberg test, American Journal of Otolaryngology 3: ((1982) ), 309–318. |
[7] | Danneels M. , Van Hecke R. , Keppler H. , Degeest S. , Cambier D. , van de Berg R. , Van Rompaey V. and Maes L. , Psychometric Properties of Cognitive-Motor Dual-Task Studies With the Aim of Developing a Test Protocol for Persons With Vestibular Disorders: A Systematic Review, Ear Hear 41: ((2020) ), 3–16. |
[8] | Dault M.C. , Frank J.S. and Allard F. , Influence of a visuo-spatial, verbal and central executive working memory task on postural control, Gait Posture 14: ((2001) ), 110–116. |
[9] | Dault M.C. , Yardley L. and Frank J.S. , Does articulation contribute to modifications of postural control during dual-task paradigms? Brain Res Cogn Brain Res 16: ((2003) ), 434–440. |
[10] | Fraizer E.V. and Mitra S. , Postural costs of performing cognitive tasks in non-coincident reference frames, Exp Brain Res 185: ((2008) ), 429–441. |
[11] | Gandemer L. , Parseihian G. , Kronland-Martinet R. and Bourdin C. , The influence of horizontally rotating sound on standing balance, Exp Brain Res 232: ((2014) ), 3813–3820. |
[12] | Hoppes C.W. , Sparto P.J. , Whitney S.L. , Furman J.M. and Huppert T.J. , Changes in cerebral activation in individuals with and without visual vertigo during optic flow: A functional near-infrared spectroscopy study, Neuroimage Clin 20: ((2018) ), 655–663. |
[13] | Huxhold O. , Li S.-C. , Schmiedek F. and Lindenberger U. , Dual-tasking postural control: Aging and the effects of cognitive demand in conjunction with focus of attention, Brain Res Bulletin 69: ((2006) ), 294–305. |
[14] | Jacob R.G. , Woody S.R. , Clark D.B. , Lilienfeld S.O. , Hirsch B.E. , Kucera G.D. , Furman J.M. and Durrant J.D. , Discomfort with space and motion: A possible marker of vestibular dysfunction assessed by the situational characteristics questionnaire, J Psychopathol Behav Assess 15: ((1993) ), 299–324. |
[15] | Johnson K.J. , Watson A.M. , Tokuno C.D. , Carpenter M.G. and Adkin A.L. , The effects of distraction on threat-related changes in standing balance control, Neurosci Lett 716: ((2020) ), 134635. |
[16] | Kennedy R.S. , Fowlkes J.E. , Berbaum K.S. and Lilienthal M.G. , Use of a motion sickness history questionnaire for prediction of simulator sickness, Aviat Space Environ Med 63: ((1992) ), 588–593. |
[17] | Keshner E.A. and Dhaher Y. , Characterizing Head Motion in 3 Planes during Combined Visual and Base of Support Disturbances in Healthy and Visually Sensitive Subjects, Gait Posture 28: ((2008) ), 127–134. |
[18] | Keshner E.A. , Streepey J. , Dhaher Y. and Hain T. , Pairing virtual reality with dynamic posturography serves to differentiate between patients experiencing visual vertigo, J Neuroeng Rehabil 4: ((2007) ), 24. |
[19] | Lubetzky A.V. , Coker E. , Arie L. , Aharoni M.M.H. and Krasovsky T. , Visual and Cognitive Interactions for Postural Control: Through the Head’s Magnifying Glass, (In preparation). |
[20] | Lubetzky AV. , Harel D. , Darmanin H. and Perlin K. , Assessment via the Oculus of Visual “Weighting” and “Re-weighting” in Young Adults, Motor Control 21: ((2017) ), 468–482. |
[21] | Lubetzky A.V. , Harel D. , Kelly J. , Hujsak B.D. and Perlin K. , Weighting and Reweighting of Visual Input via Head Mounted Display given Unilateral Peripheral Vestibular Dysfunction, Hum Mov Sci 68: ((2019) ), 102526. |
[22] | Lubetzky A.V. and Hujsak B.D. , A virtual reality head stability test for patients with vestibular dysfunction, J Vestib Res 28: ((2018) ), 393–400. |
[23] | Lubetzky A.V. , Hujsak B.D. , Fu G. and Perlin K. , An Oculus Rift Assessment of Dynamic Balance by Head Mobility in a Virtual Park Scene: A Pilot Study, Motor Control 23: ((2019) ), 127–142. |
[24] | Lubetzky A.V. , Wang Z. and Krasovsky T. , Head mounted displays for capturing head kinematics in postural tasks, J Biomech 27: ((2019) ), 175–182. |
[25] | Nasreddine Z.S. , Phillips N.A. , Bédirian V. , Charbonneau S. , Whitehead V. , Collin I. , Cummings J.L. and Chertkow H. , The Montreal Cognitive Assessment, MoCA: a brief screening tool for mild cognitive impairment, J Am Geriatr Soc 53: ((2005) ), 695–699. |
[26] | Pavlou M. , Davies R.A. and Bronstein A.M. , The assessment of increased sensitivity to visual stimuli in patients with chronic dizziness,, J Vestib Res 16: ((2006) ), 223–231. |
[27] | Pedalini M.E.B. , Cruz O.L.M. , Bittar R.S.M. , Lorenzi M.C. and Grasel S.S. , Sensory organization test in elderly patients with and without vestibular dysfunction, Acta Otolaryngol 129: ((2009) ), 962–965. |
[28] | Pellecchia G.L. , Dual-Task Training Reduces Impact of Cognitive Task on Postural Sway, J Mot Behav 37: ((2005) ), 239–246. |
[29] | Polastri P.F. and Barela J.A. , Adaptive visual re-weighting in children’s postural control, PLoS ONE 8: ((2013) ), e82215. |
[30] | Polskaia N. and Lajoie Y. , Reducing postural sway by concurrently performing challenging cognitive tasks, Hum Mov Sci 46: ((2016) ), 177–183. |
[31] | Popkirov S. , Staab J.P. and Stone J. , Persistent postural-perceptual dizziness (PPPD): a common, characteristic and treatable cause of chronic dizziness, Pract Neurol 18: ((2018) ), 5–13. |
[32] | Pozzo T. , Berthoz A. , Lefort L. and Vitte E. , Head stabilization during various locomotor tasks in humans. II. Patients with bilateral peripheral vestibular deficits, Exp Brain Res 85: ((1991) ), 208–217. |
[33] | Prosperini L. , Castelli L. , Sellitto G. , De Luca F. , De Giglio L. , Gurreri F. and Pozzilli C. , Investigating the phenomenon of “cognitive-motor interference” in multiple sclerosis by means of dual-task posturography, Gait Posture 41: ((2015) ), 780–785. |
[34] | Quatman-Yates C.C. , Lee A. , Hugentobler J.A. , Kurowski B.G. , Myer G.D. and Riley M.A. , Test-retest consistency of a postural sway assessment protocol for adolescent athletes measured with a force plate, Int J Sports Phys Ther 8: ((2013) ), 741–748. |
[35] | Querner V. , Krafczyk S. , Dieterich M. and Brandt T. , Patients with somatoform phobic postural vertigo: the more difficult the balance task, the better the balance performance, Neurosci Lett 285: ((2000) ), 21–24. |
[36] | Richer N. , Saunders D. , Polskaia N. and Lajoie Y. , The effects of attentional focus and cognitive tasks on postural sway may be the result of automaticity, Gait Posture 54: ((2017) ), 45–49. |
[37] | Rith-Najarian S.J. , Stolusky T. and Gohdes D.M. , Identifying diabetic patients at high risk for lower-extremity amputation in a primary health care setting. A prospective evaluation of simple screening criteria, Diabetes Care 15: ((1992) ), 1386–1389. |
[38] | Salthouse T.A. , What cognitive abilities are involved in trail-making performance? Intelligence 39: ((2011) ), 222–232. |
[39] | Schniepp R. , Wuehr M. , Pradhan C. , Novozhilov S. , Krafczyk S. , Brandt T. and Jahn K. , Nonlinear variability of body sway in patients with phobic postural vertigo, Front Neurol 4: ((2013) ), 115. |
[40] | Seemungal B.M. and Passamonti L. , Persistent postural-perceptual dizziness: a useful new syndrome, Pract Neurol 18: ((2018) ), 3–4. |
[41] | Shanbehzadeh S. , Salavati M. , Talebian S. , Khademi-Kalantari K. and Tavahomi M. , Attention demands of postural control in non-specific chronic low back pain subjects with low and high pain-related anxiety, Exp Brain Res 236: ((2018) ), 1927–1938. |
[42] | Shumway-Cook A. , Brauer S. and Woollacott M. , Predicting the probability for falls in community-dwelling older adults using the Timed Up & Go Test, Phys Ther 80: ((2000) ), 896–903. |
[43] | Soames R.W. and Atha J. , The spectral characteristics of postural sway behaviour, Eur J Appl Physiol Occup Physiol 49: ((1982) ), 169–177. |
[44] | Söhsten E. , Bittar R.S.M. and Staab J.P. , Posturographic profile of patients with persistent postural-perceptual dizziness on the sensory organization test, J Vestib Res 26: ((2016) ), 319–326. |
[45] | Spielberger C. , Gorsuch R. , Lushene R. , Vagg P. and Jacobs G. , Manual for the State-Trait Anxiety Inventory (Form Y1 – Y2), 1983. |
[46] | Staab J.P. , Eckhardt-Henn A. , Horii A. , Jacob R. , Strupp M. , Brandt T. and Bronstein A. , Diagnostic criteria for persistent postural-perceptual dizziness (PPPD): Consensus document of the committee for the Classification of Vestibular Disorders of the Barany Society,, J Vestib Res 27: ((2017) ), 191–208. |
[47] | Swan L. , Otani H. and Loubert P.V. , Reducing postural sway by manipulating the difficulty levels of a cognitive task and a balance task, Gait Posture 26: ((2007) ), 470–474. |
[48] | Swanenburg J. , de Bruin E.D. , Favero K. , Uebelhart D. and Mulder T. , The reliability of postural balance measures in single and dual tasking in elderly fallers and non-fallers, BMC Musculoskelet Disord 9: ((2008) ), 162. |
[49] | Whitney S.L. , Marchetti G.F. , Schade A. and Wrisley D.M. , The sensitivity and specificity of the Timed “Up & Go” and the Dynamic Gait Index for self-reported falls in persons with vestibular disorders,, J Vestib Res 14: ((2004) ), 397–409. |
[50] | Whitney S.L. , Roche J.L. , Marchetti G.F. , Lin C.-C. , Steed D.P. , Furman G.R. , Musolino M.C. and Redfern M.S. , A comparison of accelerometry and center of pressure measures during computerized dynamic posturography: a measure of balance, Gait Posture 33: ((2011) ), 594–599. |
[51] | Woollacott M. and Shumway-Cook A. , Attention and the control of posture and gait: a review of an emerging area of research, Gait Posture 16: ((2002) ), 1–14. |
[52] | Wuehr M. , Brandt T. and Schniepp R. , Distracting attention in phobic postural vertigo normalizes leg muscle activity and balance, Neurology 88: ((2017) ), 284–288. |
[53] | Wuehr M. , Pradhan C. , Novozhilov S. , Krafczyk S. , Brandt T. , Jahn K. and Schniepp R. , Inadequate interaction between open- and closed-loop postural control in phobic postural vertigo, J Neurol 260: ((2013) ), 1314–1323. |
[54] | Yogev-Seligmann G. , Hausdorff J.M. and Giladi N. , Do we always prioritize balance when walking? Towards an integrated model of task prioritization, Mov Disord 27: ((2012) ), 765–770. |
[55] | Young L.R. , Bernard-Demanze L. , Dumitrescu M. , Magnan J. , Borel L. and Lacour M. , Postural performance of vestibular loss patients under increased postural threat, J Vestib Res 22: ((2012) ), 129–138. |
[56] | Zeynalzadeh Ghoochani B. , Hosseini S.A. , Talebian S. , Biglarian A. , Zeinalzadeh A. , Nazary-Moghadam S. and Derakhshanrad S.A. , Healthy older adults balance pattern under dual task conditions: exploring the strategy and trend, Health Promot Perspect 6: ((2016) ), 207–212. |
Appendices
Appendix A
Descriptive Statistics for the Single Task conditions
Control | PPPD | ||||||
Static | AP5 | AP32 | Static | AP5 | AP32 | ||
COP Path ML (cm) | Mean (SD) | 77.24 (27.43) | 68.48 (25.18) | 73.74 (23.57) | 75.15 (28.41) | 67.47 (22.26) | 69.07 (20.56) |
Median | 74 | 64.75 | 64.99 | 77.07 | 64.79 | 65.48 | |
Range | 108.98 | 90.24 | 87.75 | 101.75 | 95.13 | 79 | |
COP Path AP (cm) | Mean (SD) | 58.84 (18.99) | 56.02 (19.49) | 63.53 (19.63) | 82.18 (44.61) | 68.3 (25.8) | 67.76 (21.11) |
Median | 59.21 | 51.02 | 60.28 | 72.79 | 62.29 | 61.6 | |
Range | 68.04 | 81.76 | 70.96 | 185.98 | 85.65 | 62.16 | |
COP RMSA ML (cm/s2) | Mean (SD) | 89.46 (36.8) | 80.78 (36.09) | 88 (34.2) | 99.03 (46.17) | 86.91 (37.18) | 87.02 (35) |
Median | 84.13 | 77.38 | 78.4 | 94.44 | 78.5 | 78.37 | |
Range | 139.17 | 140.47 | 125.13 | 149.4 | 149.67 | 128.32 | |
COP RMSA AP (cm/s2) | Mean (SD) | 99.66 (37.77) | 90.93 (36.13) | 105.60 (40.13) | 149.26 (90.45) | 124.75 (52.25) | 119.82 (40.16) |
Median | 93.86 | 85.84 | 98.21 | 130.24 | 114.46 | 108.14 | |
Range | 149.09 | 138.52 | 122.90 | 328.1 | 177.12 | 129.52 | |
Head Path ML (cm) | Mean (SD) | 33.25 (11.13) | 32.22 (12.47) | 35.24 (12.67) | 48.05 (29.03) | 43.37 (21.21) | 41.97 (23.74) |
Median | 30.16 | 27.57 | 31.23 | 38.42 | 38.5 | 32.37 | |
Range | 35.97 | 42.49 | 38.92 | 92.6 | 69.28 | 90.25 | |
Head Path AP (cm) | Mean (SD) | 19.8 (6.19) | 18.71 (5.67) | 20.87 (6.17) | 26.06 (11.8) | 23.66 (8.87) | 22.01 (6.86) |
Median | 20.1 | 18.24 | 20.37 | 23.52 | 21.56 | 21.77 | |
Range | 20.49 | 22.43 | 25.42 | 41.27 | 32.57 | 27.25 | |
Head RMSA ML (cm/s2) | Mean (SD) | 27.33 (9.24) | 32.37 (12.69) | 35.51 (12.67) | 40.98 (24.66) | 42.45 (20.95) | 40.49 (23.18) |
Median | 23.44 | 27.20 | 30.8 | 38.55 | 39.72 | 32.79 | |
Range | 28.73 | 37.36 | 37.79 | 81.53 | 62.56 | 80.17 | |
Head RMSA AP (cm/s2) | Mean (SD) | 18.49 (5.07) | 20.12 (5.12) | 23.39 (8.53) | 27.74 (13.47) | 27.32 (12.12) | 24.46 (7.56) |
Median | 17.67 | 19.26 | 22.21 | 23.72 | 23.8 | 22.33 | |
Range | 17.11 | 19.01 | 40.58 | 45.33 | 51.90 | 30.08 |
Appendix B
Descriptive Statistics for the Dual Task conditions
Control | PPPD | ||||||
Static | AP5 | AP32 | Static | AP5 | AP32 | ||
COP Path ML (cm) | Mean (SD) | 78.66 (26.1) | 79.25 (26.78) | 85.4 (25.67) | 76.82 (26.66) | 75.91 (20.51) | 80.24 (20.31) |
Median | 71.97 | 72.71 | 80.53 | 78.62 | 76.82 | 76.74 | |
Range | 98.13 | 89.22 | 104.88 | 118.88 | 84.29 | 76.64 | |
COP Path AP (cm) | Mean (SD) | 66.40 (27.19) | 66.89 (25.57) | 70.21 (24.48) | 72.74 (31.45) | 76.03 (31.38) | 80.23 (26.14) |
Median | 58.89 | 62.98 | 65.84 | 61.77 | 64.11 | 67.64 | |
Range | 109.33 | 98.7 | 76.15 | 116.79 | 116.19 | 85.26 | |
COP RMSA ML (cm/s2) | Mean (SD) | 92.27 (38.38) | 91.6 (38.5) | 99.76 (35.3) | 97.63 (41.19) | 96.07 (32.42) | 104.36 (33.56) |
Median | 82.32 | 82.89 | 95.98 | 82.48 | 97.74 | 101.22 | |
Range | 157.66 | 146.73 | 139.11 | 174.4 | 128.77 | 122.27 | |
COP RMSA AP (cm/s2) | Mean (SD) | 112.55 (58.37) | 104.43 (43.34) | 111.17 (37.17) | 129.29 (54.88) | 133.27 (56.64) | 145.42 (49.37) |
Median | 95.5 | 99.6 | 102.13 | 116.41 | 125.49 | 128.82 | |
Range | 222.74 | 172.92 | 123.55 | 215.48 | 230.39 | 167.04 | |
Head Path ML (cm) | Mean (SD) | 40.7 (23.22) | 40.77 (16.38) | 45.18 (20.96) | 58.6 (43.48) | 53.2 (33.02) | 55.43 (45.21) |
Median | 32.4 | 35.26 | 41.77 | 40.23 | 38.17 | 38.48 | |
Range | 98.07 | 51.59 | 94.57 | 176.07 | 104.25 | 203.42 | |
Head Path AP (cm) | Mean (SD) | 27.21 (17.76) | 27.91 (19.46) | 27.14 (15.61) | 27.26 (12.06) | 27.03 (14.07) | 29.85 (13.31) |
Median | 22.01 | 21.5 | 21.34 | 23.71 | 22.41 | 24.33 | |
Range | 81.41 | 90.34 | 71.06 | 53.14 | 59.11 | 57.88 | |
Head RMSA ML (cm/s2) | Mean (SD) | 33.97 (18.4) | 38.8 (15.72) | 43.11 (18.18) | 48.30 (35.73) | 50.41 (31.99) | 51.7 (40.3) |
Median | 26.82 | 34.46 | 40.54 | 34.91 | 34.17 | 37.26 | |
Range | 77.24 | 48.42 | 79.87 | 140.17 | 109.91 | 182.48 | |
Head RMSA AP (cm/s2) | Mean (SD) | 25.09 (13.97) | 28.18 (15.83) | 28.05 (14.08) | 25.37 (9.8) | 28.78 (13.96) | 30.71 (12.07) |
Median | 20.21 | 23.78 | 22.68 | 22.16 | 24.58 | 26.25 | |
Range | 63.56 | 71.88 | 63.04 | 41.24 | 52.49 | 48.01 |