Gaze shift dynamic visual acuity: A functional test of gaze stability that distinguishes unilateral vestibular hypofunction
Abstract
BACKGROUND:
Embedded within most rapid head rotations are gaze shifts, which is an initial eye rotation to a target of interest, followed by a head rotation towards the same target. Gaze shifts are used to acquire an image that initially is outside of the participant’s current field of vision. Currently, there are no tools available that evaluate the functional relevance of a gaze shift.
OBJECTIVE:
The purpose of our study was to measure dynamic visual acuity (DVA) while performing a gaze shift.
METHODS:
Seventy-one healthy participants (42.79±16.89 years) and 34 participants with unilateral vestibular hypofunction (UVH) (54.59±20.14 years) were tested while wearing an inertial measurement unit (IMU) sensor on the head and walking on a treadmill surrounded by three monitors. We measured visual acuity during three subcomponent tests: standing (static visual acuity), while performing an active head rotation gaze shift, and an active head rotation gaze shift while walking (gsDVAw).
RESULTS:
While doing gsDVAw, patients with Left UVH (n = 21) had scores worse (p = 0.023) for leftward (0.0446±0.0943 LogMAR) head rotation compared with the healthy controls (–0.0075±0.0410 LogMAR). Similarly, patients with right UVH (N = 13) had worse (p = 0.025) gsDVAw for rightward head motion (0.0307±0.0481 LogMAR) compared with healthy controls (–0.0047±0.0433 LogMAR). As a whole, gsDVAw scores were worse in UVH compared to the healthy controls when we included the ipsilesional head rotation on both sides gsDVAw (0.0061±0.0421 LogMAR healthy vs. 0.03926±0.0822 LogMAR UVH, p = 0.003). Controlling for age had no effect, the gsDVAw scores of the patients were always worse (p < 0.01).
CONCLUSION:
The gaze shift DVA test can distinguish gaze stability in patients with UVH from healthy controls. This test may be a useful measure of compensation for patients undergoing various therapies for their vestibular hypofunction.
1Introduction
The vestibular system plays an important role in the gaze stability during dynamic activities, such as walking or running. In activities of daily living, people must maintain gaze stability in order to prevent the movement of visual objects from the fovea of the retina (known as retinal slip), particularly during rapid head rotations that primarily rely on the vestibular ocular reflex (VOR) [29, 30]. Impairment of the VOR (high or low) causes the perception of visual targets moving off the fovea of the retina known as oscillopsia, but also causes reduced visual acuity during head motion, dizziness, and a sense of imbalance [3, 4, 16, 27]. When visually scanning for objects beyond the boundary of the individual’s field of view, the individual must rotate their head towards the direction of the target. Embedded within most large head rotations are gaze shifts, which is an initial eye rotation to a target of interest, followed by a head rotation towards the same target. Gaze shifts are used to acquire an image that initially is outside of the participant’s current field of vision. For example, a person walking along the street hears their name called from behind them and towards the right side. In this case, the participant initially uses a rightward directed saccade to the target of interest followed by a rightward head rotation. During the head rotation the VOR must be suppressed - otherwise it will move the eyes off the target. However, once the eyes acquire the target, the VOR must then engage in order to keep the eyes stable in space and fixated on the target [8, 17].
One of the most useful and functional measures of the VOR in patients with chronic vestibular loss or hypofunction is the computerized dynamic visual acuity test (cDVA). This test utilizes different font sizes of optotypes (i.e. letters) center-displayed on a computer monitor. During the test, participants typically use horizontal (or vertical) sinusoidal head rotations at a velocity threshold greater than the ocular following mechanism (i.e. pursuit, greater than 100°/s) while attempting to read the optotypes displayed on the screen. The total number of correctly identified optotypes are then converted to a LogMAR score (Logarithm of the Minimal Angle Resolvable) and compared against healthy controls. cDVA scores worsen with age and vestibular hypofunction [23]. The cDVA has been widely used in establishing recovery of gaze stability in patients with unilateral and bilateral vestibular hypofunction [20, 21, 46]. The cDVA test has been proven to be a reliable test in both normal participants and patients with vestibular hypofunction [23]. However, while the cDVA is a functional measure of the VOR, humans don’t typically engage in this behavior (repeated, active, sinusoidal head rotation while sitting).
The cDVA test was designed as a functional performance of the VOR. Currently, there are no tools available that evaluate the functional relevance of a gaze shift, hence, we have added a gaze shift component and modified the traditional cDVA. We believe this may provide a valuable new method to measure gaze stability given humans commonly make gaze shifts. We call this new test the gaze shift dynamic visual acuity test (gsDVA). The purpose of this study was to compare differences of the gsDVA between healthy controls (of different age groups) and patients with unilateral vestibular hypofunction. We hypothesized that healthy participants would have better visual acuity during gaze shifts than patients with vestibular hypofunction due to intact vestibular system and functional gaze control during locomotion.
2Methods
2.1Participants
Healthy individuals were recruited from the com-munity, and patients with unilateral vestibular hypofunction (UVH) were recruited from the clinics within the Department of Physical Medicine & Rehabilitation, Taipei Veterans General Hospital (CLK) and the Department of Otolaryngology, Cheng Hsin General Hospital (Lieber PH Li). All diagnoses were confirmed by otolaryngologists and based on results from bithermal caloric irrigations, which only assess function from each horizontal semicircular canal. Each participant agreed to participate and signed an informed consent form as approved by the Taipei Veterans General Hospital & National Yang-Ming University Institutional Review Board. Participants with normal or corrected-to-normal vision were enrolled with the following criteria: absent any previous physical, auditory, visual, or cognitive disorders, and not using a medication that may influence their walking abilities. Each participant was examined for the ability to conduct continual head turning for at least 60 degrees to either side during walking. Each participant was instructed in use of the equipment and practiced the experimental scenarios at their preferred speed on the treadmill for 5∼10 minutes. One physical therapist (PYC) accompanied the participants throughout the whole experiment to ensure their safety.
2.2Instrumentation
The experiments were conducted in an open space (Height 4 x Length 6 x Width 3 m3) using an inertial measurement unit (IMU) sensor (PNI SpacePoint SCOUT, USA) that included a built-in 3-axis accelerometer, a 3-axis gyroscope, and PNI’s Geomagnetic Sensor Suite with a sampling rate of 100 Hz. Participants walked on an automated rehabilitation treadmill (SUNPRO GZ-8643E, Taiwan) surrounded by three monitors connected to a desktop PC. One monitor was placed in front of the participants and two additional monitors were positioned 60 degrees to each side of the patient when facing the center monitor (Fig. 1). Custom software to execute the experiments was written using NI LabVIEW 2013 (National Instruments, USA).
Fig. 1
Gaze Shift DVA Test. A). Rear view of the test. B). A leftward arrow displayed on the center monitor instructs the participant to turn to their head from the initial head position (center, green arrow) towards the left. Once the head position is greater than 15 degrees (moving from green to red zone), the arrow disappears. C). As the head position moves inside the red zone, the left monitor displays the optotype (letter E) and the participant reports the direction the opening of the letter is facing (right is illustrated). The examiner records the participants’ response and the optotype is removed. The participant then returns to the original head position (green zone) and waits 2 seconds for the next random presentation of the direction of head rotation (and random optotype display).
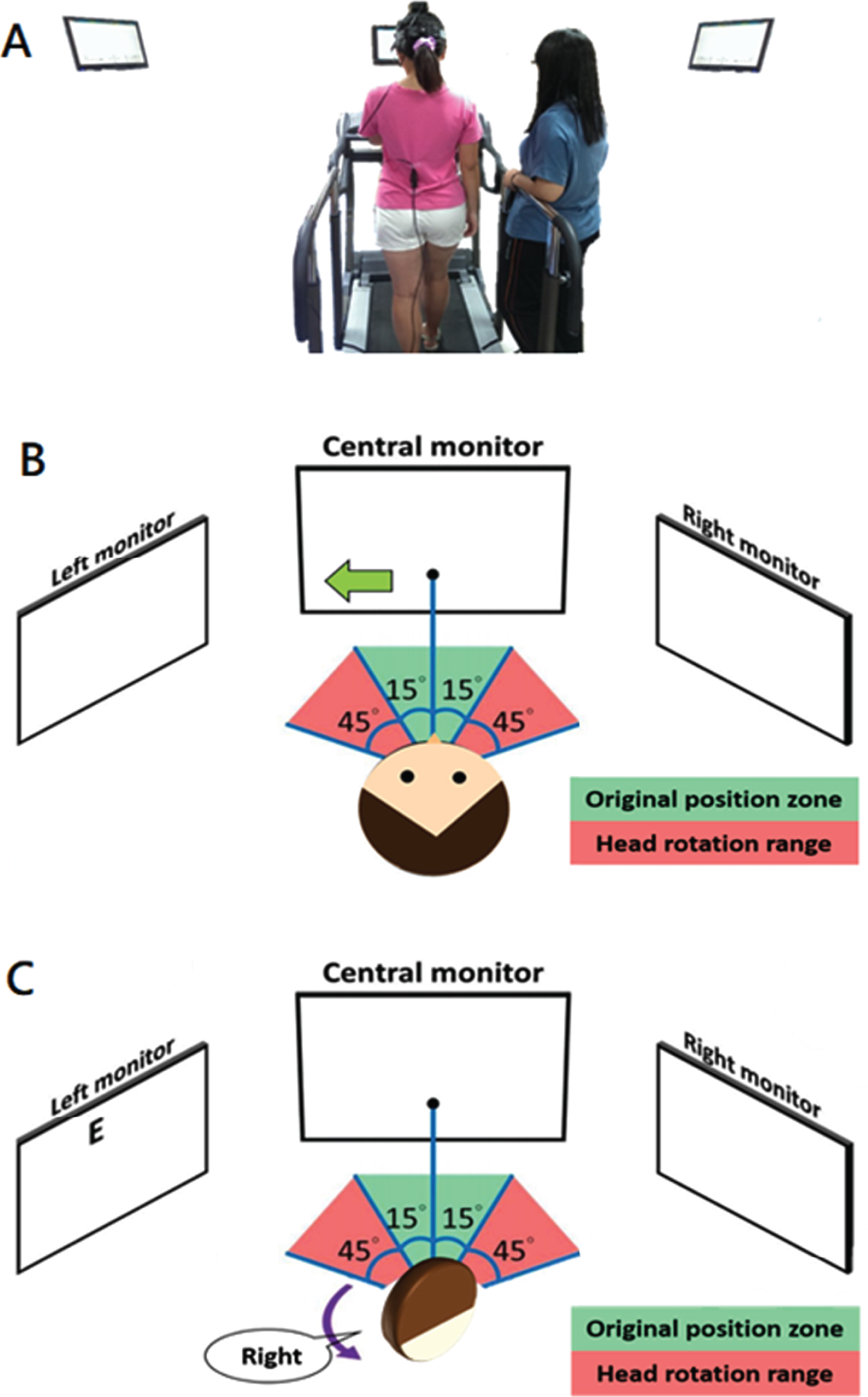
2.3Experimental procedures
Participants were first introduced to the method of the study and ample time was provided to ensure understanding. Three major components were then collected: static visual acuity, gsDVA in stance (gsDVAs), and gsDVA while walking (gsDVAw). The gsDVA test were modified based on the traditional cDVA. Once the headband and attached IMU sensor was placed, static visual acuity (SVA) was measured by having participants stand facing the center monitor and verbally reporting the direction the ‘legs’ of the optotype (letter E) (e.g. ‘E’ –rightward facing). All participants were asked to identify the “E” letter (the letter E, randomly rotated each trial by 0°, 90°, 180°, or 270°) openings shown on the monitor 2 meters in front of them. There are 5 trials for each acuity level. The optotype size reduced in size as the test progressed; changes in visual acuity from line to line are equivalent to 0.1 logarithm of the minimal angle of resolution (LogMAR). If the participant missed all of 5 optotypes of the same size or provided responses for all 55 optotypes in our test, the test terminated. We provided a 2-second interval between each presentation of the letter orientation after patients’ responses entered by the investigator. Any participants who could not read the largest size of optotypes correctly (Snellen visual acuity worse than 20/200), were excluded. Next, we measured gsDVA with the participants standing still (gsDVAs). At the start of the test, the center monitor illustrated an arrow that randomly pointed to the either the left or to the right. The participant was instructed to turn their head ∼ 60 degrees as fast as possible towards the direction of the arrow and look at that 2nd monitor (positioned 60 deg on that side). Next, the letter E was presented on that 2nd monitor, having been triggered by the participant’s head rotation range ≧60 degrees to either side. The participant then declared the orientation of the ‘legs’ of the letter as fast as possible. The orientation of the letter stayed on the screen until the investigator recorded the response. Once the response was recorded, the letter disappeared on the side screen, which was the cue for the participant to return their gaze (eye and head) back to the center monitor and wait 2 seconds before the arrow randomly directed the next head rotation. The process of the optotype display in gsDVA test is similar to SVA test as stated above. The changing of the optotype size and the timing of termination are the same as in the SVA test. The final test used the same method to collect gsDVA as described but the participants self-selected a comfortable pace for walking on a treadmill (gsDVAw). The entire duration of tests required about 40 minutes to complete.
2.4Data analysis and experimental parameters
We collected five variables from each participant during each of the three subcomponent tests (SVA, gsDVAs, gsDVAw);
1. Gait speed - the preferred gait velocity of the participant walking on the treadmill. Healthy participants were binned into three unique decades (N = 38, participants aged 20–40 years; N = 16, participants aged 41–60 years and N = 14, participants aged 61–80 years).
2. Head velocity - velocity of yaw head rotation during both rightward and leftward testing of the gsDVAs and gsDVAw tests.
3. LogMAR static visual acuity (SVA): Participants viewed 5 optotypes per acuity level, with optotype size decreasing in steps equivalent to a visual acuity change of 0.1 logMAR. A LogMAR of 0 indicates a Snellen visual acuity of 20/20 (6/6 m), which means an observer can resolve details as small as 1 minute of visual angle a distance of 6m. SVA was scored when a participant failed to correctly identify 5 optotypes on an acuity level or reached the 55th optotype (logMAR score of 0.000; Snellen equivalency of 20/20 acuity). The number of incorrect responses out of 55 were converted to LogMAR values.
4. LogMAR gsDVA: The Participants turned their heads 60 degrees to one side and stopped (instead of rotated continually as in cDVA). The gsDVA performance was calculated by subtracting the numbers of incorrect responses in SVA from the numbers of incorrect response in gsDVA tests. Then, the scores were converted to logMAR. The leftward and rightward gsDVA were scored separately. Additional information about the computation of visual acuity has been published previously [24].
5. Response time - the time in seconds from the presentation of the letter E on the monitor to the examiner entering the subjects’ response.
In this study, we estimated sample size using the G*Power 3.13 software (Franz Faul, University Kiel, Germany), and based on data published in patients with UVH performing dynamic visual acuity testing [22]. Accordingly, we achieved 80% statistical power using N = 13 healthy controls and N = 7 patients with UVH. For the analysis of age differences, we recruited additional healthy participants to be able to have good sample sizes in the unique age bins.
2.5Statistical analysis
The visual acuity, average response time, and head velocity while turning were exported and processed in LabVIEW2013 (National Instruments, USA). With unequal sample sizes between the two groups, independent t-test compared the difference between the healthy participants and patients. The level of significance was set at α<0.05. Statistical analyses were performed using the SPSS 20.0 analysis software (Armonk, NY: IBM Corp.).
3.Results
We recruited a total of N = 105 participants. Among them, N = 34 patients had UVH (M:F=12 : 22, mean age 54.6±20.1 years); N = 21 left and N = 13 right UVH. The remaining N = 71 (M:F=31 : 40, mean age=42.8±16.9 years) were healthy individuals (Table 1). Forty of the 71 healthy controls were selected randomly based on mean age of patients by a customized program of LabVIEW to the age-matched control (M:F=16 : 24, mean age=53.9±13.0 years). The average duration of disease onset was within one year. There were no differences in average response time between healthy participants and UVH patients. During the period of experiments, some participants required more time to learn the task. Occasionally, those participants that used glasses needed to affix them tighter to their head.
Table 1
Demographicsand Static Visual Acuity
UVH Patients | HC Age-matched | All HC | |
Age | 54.6±20.1 | 53.9±13.0 | 42.8±16.9 |
Male: Female | 12:22 | 16:24 | 31:40 |
Sample Size | 34 | 40 | 71 |
SVA | 0.0889±0.1094 | 0.0647±0.1002 | 0.0458±0.0811 |
UVH = unilateral vestibular hypofunction, HC –healthy controls; SVA –static visual acuity (LogMAR); M = male, and F = female.
3.1The performances of age-matched healthy participant v.s. UVH patients
3.1.1Gait speed
There was no difference in mean gait speed between the age-matched healthy controls (N = 40, mean 2.87±1.10 km/h) and patient participants with UVH (N = 34, 2.67±1.12 km/h).(Fig. 2A, p = 0.45).
Fig. 2
Gait speed as a function during the gsDVAw test. A). Age-matching revealed no difference with UVH patients in gait speed completing the gsDVAw test. B). Gait speed reduced as age increased among different age groups. * represents p < 0.01.
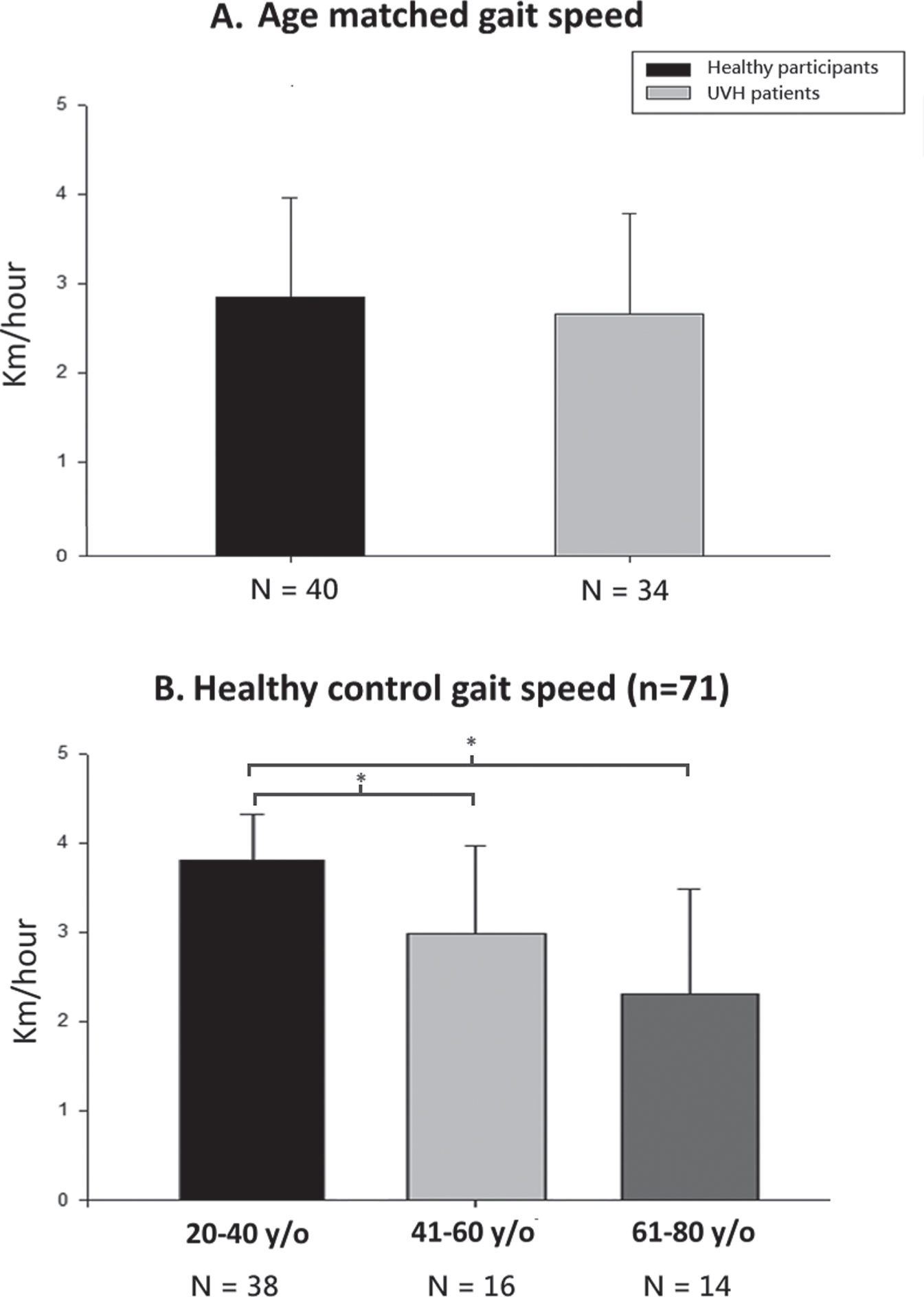
3.1.2Head velocity during the gsDVAs and gsDVAw
We found no difference in head velocity across patient or healthy controls while doing the gsDVAs (p > 0.26) in Table 2. While walking and completing the gsDVAw test, patients with UVH moved their head (combined right and left) slower than healthy controls (p = 0.02). Interestingly, both healthy control and patient participants made faster velocity head rotations toward the right in Table 2. As a result, only ipsilesional head rotations directed towards the left were significantly slower than healthy controls, ipsilesional rightward head rotations were similar in velocity to controls. When combined leftward and rightward ipsilesional rotation, we found significantly lower head velocity compared with the age matched controls.
Table 2
Head Velocity while performing the Gaze Shift DVA while Standing and Walking
Ispilesional Head Velocity | Significance | |
Participants while Standing | ||
Left UVH Patients (N = 21) | 97.26±65.8°/ s | p = 0.49 |
Age Matched (N = 40) | 109.0±61.7°/ s | |
Right UVH Patients (N = 13) | 120.91±94.1°/ s | p = 0.27 |
Age-matched (N = 40) | 153.6±75.1°/ s | |
Participants while Walking | ||
Left UVH Patients (N = 21) | 101.1±82.2°/ s | p = 0.037^* |
Age Matched (N = 40) | 147.0±71.1°/ s | |
Right UVH Patients (N = 13) | 156.6±98.4°/ s | p = 0.42 |
Age-matched (N = 40) | 180.2±89.2°/ s | |
All UVH Patients (N = 34) | 122.4±91.4 °/ s | p = 0.02^* |
Age-matched (N = 40) | 163.6±81.9 °/ s |
UVH=unilateral vestibular hypofunction.
3.1.3Scores of the gaze shift dynamic visual acuity (gsDVA) while standing (gsDVAs)
Patients with UVH displayed worse gsDVAs scores during ipsilesional head rotation compared with the age-matched healthy controls. Patients with right UVH (N = 13) had worse gsDVAs (p = 0.050) for rightward compared with healthy controls. Similarly, left UVH (n = 21) had gsDVAs scores worse (p = 0.041) for leftward rotation compared with the healthy controls (Fig. 3A).
Fig. 3
Gaze shift DVA by lesion of UVH. Regardless of controlling for static or dynamic conditions, individuals with UVH have worse gsDVA scores during A). standing and B). walking than do healthy controls (combined). a represents p = 0.041, & represents p = 0.05, and b represents p < 0.01.
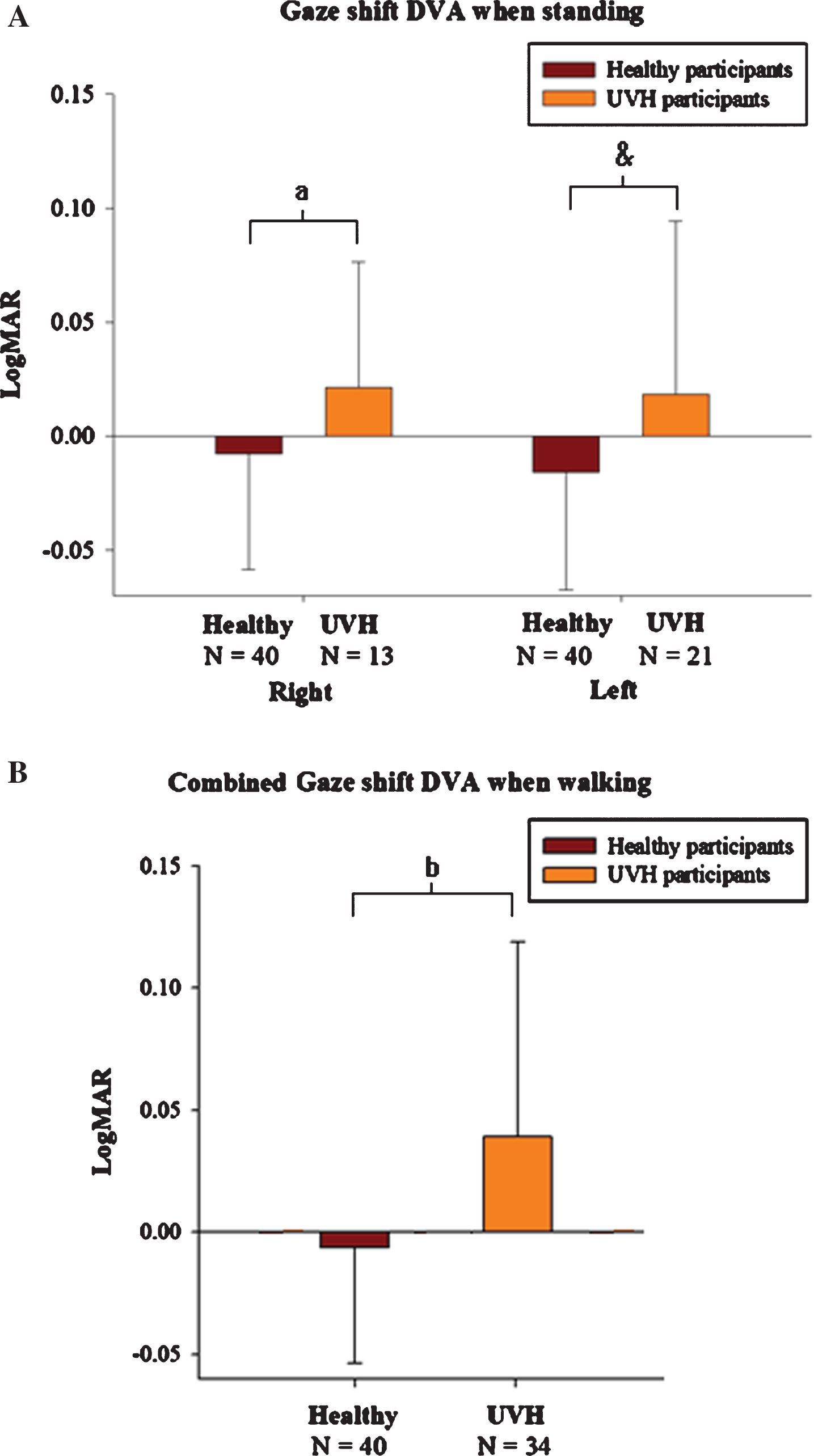
3.1.4Scores of the gaze shift dynamic visual acuity (gsDVA) while walking (gsDVAw)
Next, we combined the right and left gsDVAw for each UVH participant and compared this against the healthy participant scores. Compared with age-matched healthy controls (N = 40), the gsDVAw scores were worse in the patients subject even when we included the ipsilesional head rotation score from both sides (–0.0062±0.0476 LogMAR healthy vs. 0.0393±0.0822 LogMAR UVH, p = 0.003) (Fig. 3B). Additionally, gsDVAw scores were worse in UVH when compared against the entire healthy participants (N = 71).
Next, we analyzed the gsDVAw scores for ipsilesional only rotation (Left N = 21/Right N = 13, mean age 54.6±20.1 years) with the gsDVAw scores of healthy participants (N = 40, mean age 53.9±13.0 years). When ipsilateral rotations in UVH were compared against age-matched healthy controls, gsDVAw was worse in the participants with right UVH, the ipsilesional gsDVAw also was worse (p = 0.028) than healthy controls (Rt gsDVAw =–0.0042±0.0482 LogMAR vs. Rt UVHs: Rt gsD-VAw = 0.0307±0.0481 LogMAR). Similarly, comparing gsDVAw in patients with Left UVH (N = 21) for leftward rotation (healthy participants: Lt gsDVAw = –0.0083±0.0476 LogMAR vs. Lt UVH: Lt gsDVAw = 0.0446±0.0943 LogMAR, P = 0.024) (Table 3).
Table 3
Scores of Gaze shift DVA while standing and walking in UVH and healthy controls
Healthy Control | Ipsilesional UVH | P value | |
Age-Matched | N = 13 right; | ||
N = 40 | N = 21 left | ||
gsDVAs Rt | –0.0079±0.0505 | 0.0211±0.0551 | 0.041* |
gsDVAs Lt | –0.0159±0.0515 | 0.0183±0.0758 | 0.050 |
gsDVAw Rt | –0.0042±0.0482 | 0.0307±0.0481 | 0.028* |
gsDVAw Lt | –0.0083±0.0476 | 0.0446±0.0943 | 0.024* |
gsDVAs = dynamic visual acuity with active head rotation gaze shift while standing; gsDVAw = dynamic visual acuity with active head rotation gaze shift while walking; Rt = right head turn; Lt = left head turn. * represents p < 0.05.
3.2The performances of all the healthy participants
The mean self-selected walking velocity of the healthy controls reduced as age of the group (N =38 participants aged 20–40 years; N = 16 participants aged 41–60 years; N = 14 participants aged 61–80 years) increased (3.80±0.52 km/h; 2.98±0.99 km/h; 2.31±1.16 km/h) (Fig. 2B).
The gsDVAw scores during walking was similar across the healthy participants for both leftward and rightward head rotation.
4Discussion
4.1Impaired visual acuity during head motion in vestibular hypofunction
Our study reveals that patients with UVH have worse dynamic visual acuity (DVA) during walking and making head turns than do healthy controls. As to the typical function of the vestibular system, the VOR is responsible for generating rapid eye movements that match head velocity to ensure images remain stable on the fovea of the retina. Patients with UVH have a reduced VOR gain that results in poor vision during head motion and typically poor DVA scores [24, 41, 42]. We now show that patients with UVH also have reduced visual acuity when searching a visual target in the same direction of head rotation, as occurs during a gaze shift while walking. To date, we are unaware of any other study examining the consequence of performing a gaze shift on visual acuity in patients with vestibular hypofunction. Recently, Anastasopoulos et al (2019) measured eye-head coordination and postural stability during whole-body gaze shifts (45, 90, 135, 180deg) in seven patients with absent VOR function [1]. They report a number of significant performance decrements relative to healthy controls including reduced trunk and head velocity, increased sway path after target acquisition, increased latency of target acquisition, and reduced amplitude of the gaze shift as the target amplitude increased. However, visual acuity during the whole-body gaze shift was not measured.
There do exist a few studies examining the role of active or passive head rotation on gaze stability in seated position for patients with vestibular hypofunction, for which cDVA test is abnormal [13, 18, 19, 25]. However, we believe the gaze shift DVA is a more practical measure of gaze stability than the DVA test given how frequently humans make gaze shifts (i.e. looking around the oncoming vehicles at a road intersection). At the beginning of a gaze shift, a saccade is generated first followed by the head rotating towards the same direction. As the head rotation begins, the VOR must be suppressed to enable the eye velocity to reach the target of interest. Once the target is acquired however, the VOR must be engaged to ensure the eyes stay on the target by rotating opposite the head motion. Kasai et al. found unique gaze shift strategies in patients with bilateral vestibular hypofunction. For example, some patients generated a hypometric saccade, followed by the eyes drifting with the head during rotation towards the target to then acquire the target of interest [28]. The ‘drifting’ of the eyes with the head was possible because the VOR did not move the eyes opposite the head. Others have shown that the VOR is modifiable and dependent on amplitude of the gaze shift. Pelisson et al. concluded that the VOR is inhibited when the range of gaze shift was greater than 30 degrees in human participants [33]. Studying the gaze shift in the rhesus monkey, Tomlinson found that the VOR function is normal when the gaze shifts are small (<10 degrees). If, however, the amplitude of the gaze shift increases, the gain of the VOR diminishes to a point that it turns off completely for very large gaze shifts [44]. It also appears that gaze shifting is influenced by cognition, not surprising given the well-known effects of cognition on VOR testing [7, 10]. The gain of the VOR is suppressed when making gaze shifts with active (predictable) head perturbation, yet engaged during passive (unpredictable) head perturbations [9]. Given the unique influences of amplitude and cognition on gaze shifting, knowing its functional relevance (i.e. gsDVAw) is useful. Future studies have to be done to discover the roles of VOR and gaze shift in stabilization of DVA in both healthy and participants with vestibular hypofunction.
4.2Differences between the gsDVAw and cDVA tests
The gsDVAw has some significant advantages to the conventional measure of cDVA. First, the gsDVAw is able to distinguish the influence of age given we show that gsDVAw scores differ significantly (p < 0.05) between UVH and healthy participants regardless of age-matching. Next, the gsDVAw is not dependent on a minimum head rotation velocity neither is it dependent on walking speed (participants walk at selected pace), which means the test can be performed at the patients’ current abilities. Additionally, this suggests the gsDVAw test can be performed without participants’ being required to meet an artificially imposed head or gait velocity that is often limited by symptoms of dizziness or others. Clearly, the gsDVAw is challenging given the testing is done while walking. It is essential to maintain stability and clear vision when walking with head turning. Therefore, the quality of gsDVA may play an important role in clearly identifying targets during dynamic activities and reflect a more realistic challenge patients with vestibular hypofunction encounter during their daily life. Although the difference of scores in gsDVA were only one-third of the changes from cDVA test (0.14–0.24 LogMAR),[45] the head rotation velocity was relatively slow during gsDVA compared with traditional cDVA tests (120–180d/s range), and thus is likely to be more comfortable to complete.
The cDVA has been studied more extensively (i.e. vertical head rotation, linear head motion) and after rehabilitation [5, 34, 38]. Herdman et al. observed that patients with vestibular hypofunction do show marked improvement in cDVA after an average of 4 weeks of vestibular rehabilitation. This improved cDVA showed a greater response during active (predictable) head rotations, suggesting that central preprogramming plays a role in the recovery of cDVA in patients with UVH [11, 21, 43]. It remains unknown if the gsDVAs can be similarly improved.
The mechanism of compensation from a vestibular hypofunction is complex and includes recruitment of a compensatory saccade during both predictable and unpredictable head rotation [39, 40]. Prior research suggests compensatory saccades have an important role stabilizing gaze in both healthy and vestibular hypofunction patients [31, 35, 36]. Recently, Colagiorgio et al utilized the video head impulse EyeSeeCam (Interacoustics, Denmark) to measure head and eye trajectory during passive head rotation. They revealed that patients with vestibular hypofunction generate eye trajectories much less smoothly than healthy controls do, and postulated this further contributes to their gaze instability [6].
4.3Impact of age on gait speed, yaw head velocity, and DVA
In healthy function, the human vestibular system integrates head motion signals in order to stabilize the eyes and body position in space. Patients with vestibular hypofunction typically experience dizziness performing their daily activities, especially when walking and making head rotations. The dizziness may lead to a greater risk for fall [37]. Advanced age is correlated with impaired VOR gain in both healthy and vestibular hypofunction [2, 26]. Additionally, several studies indicate age impairs DVA [14, 15, 23]. Herdman et al showed an inverse correlation of DVA scores as age of participants with bilateral vestibular loss (BVL) increased [23]. Paige et al (1992) showed a more impaired VOR gain during high head velocity in older participants [32]. Deshpande et al similarly showed that DVA worsens in older participants during faster walking speeds [12]. We speculate older participants require more musculoskeletal and neurological adaptation when making faster head rotation and walking speed. In our study, vestibular hypofunction did not have a significant impact on gait speed, suggesting that age alone is not a significant influence.
4.4Limitations of this study
Our study is limited in part based on the age of the participants given none were over 80 years old. Additionally, it’s hard to know how scores on the gsDVAw test will generalize to real world visual acuity during gaze shifting given walking over ground is inherently uneven. The gsDVA tests in the current study was modified based on the cDVA test, but we have not determined the psychometric properties at this time [24]. Finally, we did not record the eye and head instantaneous angular velocity during the gsDVAw and thus are uncertain what oculomotor strategies may be influencing the scores.
5Conclusion
The gsDVAw represents the first functional measure of a gaze shift during walking. The test of gsDVAw is similar to tasks encountered in daily life. In addition, the test device could be rapidly set up and modified to use with a treadmill in local communities or clinical institutions. The protocol is easily to follow and execute in individuals with varied gait speeds. Furthermore, the gsDVAw can distinguish patients with UVH from healthy controls, which we believe is a more realistic measure of gaze stability than cDVA test. Future studies are warranted to investigate if the gsDVAw can show change with intervention.
Conflicts of interest
The authors declare that they have no conflicts of interest related to the subject matter or materials discussed in this article.
Acknowledgments
This study was funded by the Ministry of Science and Technology (NSC 101-2627-B-075-001- 102-2314-B-010 -061-MY3, 107-2314-B-010-010, 108-2314-B-010-042-MY3), Taipei Veterans General Hospital (V108C-152, V105C-077, and V104E14-001-MY3-2), Taipei Veterans General Hospital (VN109-11). This work was financially supported by the “ Center For Intelligent Drug Systems and Smart Bio-devices (IDS2B) “ from The Featured Areas Research Center Program within the framework of the Higher Education Sprout Project by the Ministry of Education (MOE) in Taiwan.
References
[1] | Anastasopoulos D. , Ziavra N. , Bronstein A.M. , Large gaze shift generation whilst standing up-the role of the vestibular system, J Neurophysiol ((2019) ). |
[2] | Baloh R.W. , Jacobson K.M. , Socotch T.M. , The effect of aging on visual-vestibuloocular responses, Experimental Brain Research 95: ((1993) ), 509–516. |
[3] | Bhansali S.A. , Stockwell C.W. , Bojrab D.I. , Oscillopsia in patients with loss of vestibular function, Otolaryn-gology–Head and Neck Surgery 109: ((1993) ), 120–125. |
[4] | Chambers B.R. , Mai M. , Barber H.O. , Bilateral vestibular loss, oscillopsia, and the cervico-ocular reflex, Otolaryn-gology–Head and Neck Surgery 93: ((1985) ), 403–407. |
[5] | Cheng R.C. , Walker M.F. , Vertical head translation impairs dynamic visual acuity during near viewing, Journal of Vestibular Research 26: ((2016) ), 417–423. |
[6] | Colagiorgio P. , Colnaghi S. , Versino M. , Ramat S. , A New Tool for Investigating the Functional Testing of the VOR, Frontiers in Neurology 4: ((2013) ), 1–9. |
[7] | Collins W.E. , Crampton G.H. , Posner J.B. , Effects of mental activity on vestibular nystagmus and the electroencephalogram, Nature 190: ((1961) ), 194. |
[8] | Cullen K.E. , Guitton D. , Analysis of primate IBN spike trains using system identification techniques. III. Relationship To motor error during head-fixed saccades and head-free gaze shifts, J Neurophysiol 78: ((1997) ), 3307–3322. |
[9] | Cullen K.E. , Huterer M. , Braidwood D.A. , Sylvestre P.A. , Time course of vestibuloocular reflex suppression during gaze shifts, J Neurophysiol 92: ((2004) ), 3408–3422. |
[10] | Davis R.I. , Mann R.C. , The effects of alerting tasks on caloric induced vestibular nystagmus, Ear and hearing 8: ((1987) ), 58–60. |
[11] | Della Santina C.C. , Cremer P.D. , Carey J.P. , Minor L.B. , Comparison of head thrust test with head autorotation test reveals that the vestibulo-ocular reflex is enhanced during voluntary head movements, Archives of Otolaryngology - Head and Neck Surgery 129: ((2002) ), 1044–1054. |
[12] | Deshpande N. , Tourtillott B.M. , Peters B.T. , Bloomberg J.J. , Dynamic visual acuity (DVA) during locomotion for targets at near and far distances: effects of aging, walking speed and head-trunk coupling, Journal of Vestibular Research 23: ((2013) ), 195–201. |
[13] | Dooren T.V. , Lucieer F. , Duijn S. , Janssen M. , Guinand N. , Perez Fornos A. , Van Rompaey V. , Kingma H. , Ramat S. , van de Berg R. , The Functional Head Impulse Test to Assess Oscillopsia in Bilateral Vestibulopathy, Frontiers in Neurology 10: ((2019) ), 365. |
[14] | Freedman E.G. , Coordination of the eyes and head during visual orienting, Experimental Brain Research 190: ((2008) ), 369–387. |
[15] | Gauthier G.M. , Robinson D.A. , Adaptation of the human vestibuloocular reflex to magnifying lenses, Brain Research 92: ((1975) ), 331–335. |
[16] | Gresty M.A. , Hess K. , Leech J. , Disorders of the vestibulo-ocular reflex producing oscillopsia and mechanisms compensating for loss of labyrinthine function, Brain 100: ((1977) ), 693–716. |
[17] | Guitton D. , Volle M. , Gaze control in humans: eye-head coordination during orienting movements to targets within and beyond the oculomotor range, J Neurophysiol 58: ((1987) ), 427–459. |
[18] | Herdman S.J. , Hall C.D. , Delaune W. , Variables associated with outcome in patients with unilateral vestibular hypofunction, Neurorehabilitation and Neural Repair 26: ((2012) ), 151–162. |
[19] | Herdman S.J. , Hall C.D. , Maloney B. , Knight S. , Ebert M. , Lowe J. , Variables associated with outcome in patients with bilateral vestibular hypofunction: preliminary study, Journal of Vestibular Research 25: ((2015) ), 185–194. |
[20] | Herdman S.J. , Hall C.D. , Schubert M.C. , Das V.E. , Tusa R.J. , Recovery of dynamic visual acuity in bilateral vestibular hypofunction, Arch Otolaryngol Head Neck Surg 133: ((2007) ), 383–389. |
[21] | Herdman S.J. , Schubert M.C. , Das V.E. , Tusa R.J. , Recovery of dynamic visual acuity in unilateral vestibular hypofunction, Arch Otolaryngol Head Neck Surg 129: ((2003) ), 819–824. |
[22] | Herdman S.J. , Schubert M.C. , Tusa R.J. , Role of central preprogramming in dynamic visual acuity with vestibular loss, Archives of Otolaryngology–Head & Neck Surgery 127: ((2001) ), 1205–1210. |
[23] | Herdman S.J. , Tusa R.J. , Blatt P. , Suzuki A. , Venuto P.J. , Roberts D. , Computerized dynamic visual acuity test in the assessment of vestibular deficits, American Journal of Otolaryngology 19: ((1998) ), 790–796. |
[24] | Herdman S.J. , Tusa R.J. , Blatt P. , Suzuki A. , Venuto P.J. , Roberts D. , Computerized dynamic visual acuity test in the assessment of vestibular deficits, Otology & Neurotology 19: ((1998) ), 790–796. |
[25] | Hermann R. , Ionescu E.C. , Dumas O. , Tringali S. , Truy E. , Tilikete C. , Bilateral Vestibulopathy: Vestibular Function, Dynamic Visual Acuity and Functional Impact, Frontiers in neurology 9: ((2018) ), 555. |
[26] | Huang Y. , Liu X. , Improvement of balance control ability and flexibility in the elderly Tai Chi Chuan (TCC) practitioners: a systematic review and meta-analysis, Archives of gerontology and geriatrics 60: ((2015) ), 233–238. |
[27] | Kao C.L. , Hsieh W.L. , Wang S.J. , Chen S.J. , Wei S.H. , Chan R.C. , Efficacy of a Computerized Sensor System for Evaluation and Training of Dizzy Patients, Sensors (Basel, Switzerland) 10: ((2010) ), 7602–7620. |
[28] | Kasai T. , Zee D.S. , Eye-head coordination in labyrinthine-defective human beings, Brain Research 144: ((1978) ), 123–141. |
[29] | Leigh R.J. , Brandt T. , A reevaluation of the vestibulo-ocular reflex: new ideas of its purpose, properties, neural substrate, and disorders, Neurology 43: ((1993) ), 1288–1295. |
[30] | Leigh R.J. , Sawyer R.N. , Grant M.P. , Seidman S.H. , High-frequency vestibuloocular reflex as a diagnostic tool, Annals of the New York Academy of Sciences 656: ((1992) ), 305–314. |
[31] | Macdougall H.G. , Curthoys I.S. , Plasticity during Vestibular Compensation: The Role of Saccades, Frontiers in Neurology 3: ((2012) ), 21–21. |
[32] | Paige G.D. , Senescence of human visual-vestibular interactions. 1. Vestibulo-ocular reflex and adaptive plasticity with aging, Journal of Vestibular Research 2: ((1992) ), 133–151. |
[33] | Pelisson D. , Prablanc C. , Urquizar C. , Vestibuloocular reflex inhibition and gaze saccade control characteristics during eye-head orientation in humans, J Neurophysiol 59: ((1988) ), 997–1013. |
[34] | Peters B.T. , Mulavara A.P. , Cohen H.S. , Sangi-Haghpeykar H. , Bloomberg J.J. , Dynamic visual acuity testing for screening patients with vestibular impairments, Journal of Vestibular Research 22: ((2012) ), 145–151. |
[35] | Ramat S. , Straumann D. , Zee D.S. , Interaural translational VOR: suppression, enhancement, and cognitive control, J Neurophysiol 94: ((2005) ), 2397–2402. |
[36] | Ramat S. , Zee D.S. , Ocular motor responses to abrupt interaural head translation in normal humans, J Neurophysiol 90: ((2003) ), 887–902. |
[37] | Schlick C. , Schniepp R. , Loidl V. , Wuehr M. , Hesselbarth K. , Jahn K. , Falls and fear of falling in vertigo and balance disorders: A controlled cross-sectional study, Journal of Vestibular Research 25: ((2016) ), 241–251. |
[38] | Schubert M.C. , Herdman S.J. , Tusa R.J. , Vertical dynamic visual acuity in normal subjects and patients with vestibular hypofunction, Otology & Neurotology 23: ((2002) ), 372–377. |
[39] | Schubert M.C. , Migliaccio A.A. , Santina C.C.D. , Modification of compensatory saccades after aVOR gain recovery, Journal of Vestibular Research 16: ((2006) ), 285–291. |
[40] | Schubert M.C. , Zee D.S. , Saccade and vestibular ocular motor adaptation, Restorative neurology and neuroscience 28: ((2010) ), 9–18. |
[41] | Tian J.-R. , Shubayev I. , Demer J.L. , Dynamic visual acuity during transient and sinusoidal yaw rotation in normal and unilaterally vestibulopathic humans, Experimental brain research 137: ((2001) ), 12–25. |
[42] | Tian J.-R. , Shubayev I. , Demer J.L. , Dynamic visual acuity during passive and self-generated transient head rotation in normal and unilaterally vestibulopathic humans, Experimental Brain Research 142: ((2002) ), 486–495. |
[43] | Tian J.R. , Shubayev I. , Demer J.L. , Dynamic visual acuity during passive and self-generated transient head rotation in normal and unilaterally vestibulopathic humans, Experimental Brain Research 142: ((2002) ), 486–495. |
[44] | Tomlinson R.D. , Bahra P.S. , Combined eye-head gaze shifts in the primate. II. Interactions between saccades and the vestibuloocular reflex, J Neurophysiol 56: ((1986) ), 1558–1570. |
[45] | Ward B.K. , Mohammad M.T. , Whitney S.L. , Marchetti G.F. , Furman J.M. , The reliability, stability, and concurrent validity of a test of gaze stabilization, Journal of Vestibular Research 20: ((2010) ), 363–372. |
[46] | Yen M.T. , Herdman S.J. , Tusa R.J. , Oscillopsia and pseudonystagmus in kidney transplant patients, Am J Ophthalmol 128: ((1999) ), 768–770. |