Assessing the Role of Locus Coeruleus Degeneration in Essential Tremor and Parkinson’s Disease with Sleep Disorders
Abstract
Background:
Previous studies have demonstrated the importance of the locus coeruleus (LC) in sleep-wake regulation. Both essential tremor (ET) and Parkinson’s disease (PD) share common sleep disorders, such as poor quality of sleep (QoS). LC pathology is a feature of both diseases. A question arises regarding the contribution of LC degeneration to the occurrence of poor QoS.
Objective:
To evaluate the association between LC impairment and sleep disorders in ET and PD patients.
Methods:
A total of 83 patients with ET, 124 with PD, and 83 healthy individuals were recruited and divided into ET/PD with/without poor QoS (Sle/NorET and Sle/NorPD) subgroups according to individual Pittsburgh Sleep Quality Index (PSQI) score. Neuromelanin-sensitive magnetic resonance imaging (NM-MRI) and free-water imaging derived from diffusion MRI were performed. Subsequently, we evaluated the association between contrast-to-noise ratio of LC (CNRLC) and free-water value of LC (FWLC) with PSQI scores in ET and PD groups.
Results:
CNRLC was significantly lower in ET (p = 0.047) and PD (p = 0.018) than in healthy individuals, whereas no significant difference was found in FWLC among the groups. No significant differences were observed in CNR/FWLC between patients with/without sleep disorders after multiple comparison correction. No correlation was identified between CNR/FWLC and PSQI in ET and PD patients.
Conclusions:
LC degeneration was observed in both ET and PD patients, implicating its involvement in the pathophysiology of both diseases. Additionally, no significant association was observed between LC integrity and PSQI, suggesting that LC impairment might not directly relate to overall QoS.
Plain Language Summary
A brain nucleus named locus coeruleus (LC) is important in sleep-wake regulation, and poor quality of sleep (QoS) is common in essential tremor (ET) and Parkinson’s disease (PD) patients. Previous studies demonstrated the LC is impaired in ET and PD. We proposed that LC impairment might be related to LC degeneration. The contrast-to-noise ratio of LC (CNRLC) and free-water value of LC (FWLC) acquired from MRI scans, and Pittsburgh Sleep Quality Index (PSQI) score can reflect LC impairment and quality of sleep, respectively. To test this proposal, we recruited 83 patients with ET, 124 with PD, and 83 healthy individuals and divided them into ET/PD with/without poor QoS (Sle/NorET and Sle/NorPD) subgroups and MRI scans were performed. We found that CNRLC was lower in ET and PD than in healthy individuals, but nor was FWLC. The CNR/FWLC were equal between patients with/without poor QoS, and we found there were no correlation between CNR/FWLC and PSQI score in ET and PD patients. These results suggested that although the LC is impaired in ET and PD, it might not be directly linked to overall sleep quality.
INTRODUCTION
Essential tremor (ET) and Parkinson’s disease (PD) are the two most prevalent movement disorders, primarily characterized by their respective classical motor symptoms. Clinically, the former is defined as “isolated action tremor of bilateral upper limbs”, whereas the latter encompasses symptoms such as rest tremor, rigidity, bradykinesia, and postural and gait instability [1, 2]. Furthermore, comprehensive studies have indicated that individuals with ET or PD might also experience similar nonmotor symptoms, including sleep disorders [3–5], cognitive impairment [3, 6], and a spectrum of neuropsychiatric disorders [3, 7, 8]. Particularly in recent years, an increasing number of studies have shed light on sleep disorders in ET and PD, such as poor quality of sleep (QoS), rapid eye movement sleep behavior disorder (RBD), obstructive sleep apnea, and others. It has also been suggested that individuals with sleep disorders have an elevated risk of developing neurodegenerative disorders and that the progression of those disorders might be accelerated [9, 10]. This can be attributed to the impaired clearance of waste proteins during periods of inadequate sleep, potentially contributing to long-term neurodegenerative processes [11].
Of all the sleep problems mentioned above, poor QoS is the most frequent issue in ET patients. In PD, besides RBD, poor QoS is also associated with cognitive impairments, mobility issues, anxiety, and freezing of gait [12]. However, the neural mechanism underlying poor QoS in patients with ET and PD remains unknown. Sleep behavior is regulated by the ascending reticular activating system (ARAS), within which the locus coeruleus (LC) is a crucial nucleus [13]. The LC acts as the primary source of norepinephrine in the brain and plays a predominant role in sleep-wake regulation [14]. Findings from animal experiments and studies conducted on healthy subjects have revealed that the LC is involved in arousal [15, 16], sleep-wake transitions [15, 17], and emotional regulation [18]. Consequently, damage to the LC can disrupt these functions and impact QoS. Previous studies have already examined and found a strong association between LC degeneration and RBD in individuals with PD [19]. Furthermore, LC degeneration has also been associated with mild sleep disturbance in PD [20]. However, the impact of LC degeneration on sleep disorders in ET, specifically the decline in QoS, remains unknown. Therefore, the main objective of our research is to investigate whether LC degeneration is associated with the decline in QoS in PD or ET.
Due to the small size of the LC, conventional structural magnetic resonance imaging (sMRI) is unable to provide noninvasive imaging of the LC. However, advanced MRI techniques such as neuromelanin-sensitive MRI (NM-MRI) and free-water imaging have emerged as important tools for in vivo assessment of LC injury. Currently, NM-MRI is considered the optimal noninvasive in vivo imaging modality, with which our team and other researchers have detected LC degeneration in patients with ET and PD [19, 21]. Free-water imaging is a novel imaging technique for detecting LC degeneration with high sensitivity [22], and could therefore serve as a supplementary tool for evaluating LC injury.
Since LC degeneration occurs in both ET and PD patients, the objective of this study was to investigate and compare the relationship between overall sleep quality and LC in ET and PD patients, with and without impaired sleep quality. In addition, we examined the correlation between Pittsburgh Sleep Quality Index (PSQI) score and LC signal intensity (SI) in both ET and PD patients.
MATERIALS AND METHODS
Participants
A total of 290 participants, comprising 83 ET, 124 PD, and 83 healthy control (HC) individuals, were included in this study. All participants were enrolled in the Second Affiliated Hospital of Zhejiang University School of Medicine from March 2019 to June 2023. ET patients were diagnosed in accordance with the Consensus Statement on the classification of tremors [1], and PD diagnoses were confirmed by experienced neurologists based on MDS Clinical Diagnostic Criteria for Parkinson’s disease [23]. Participants who met the following criteria were excluded: (1) other neurodegenerative diseases, such as multiple system atrophy, progressive supranuclear palsy, dementia with Lewy bodies; (2) history of cerebrovascular disorders, craniocerebral trauma, neurological surgery, intracranial space-occupying lesions, and other psychiatric diseases; (3) low-quality MRI images containing artifacts; (4) left- or bilateral-handedness in participants; and (5) refusal to sign informed consent or presence of MRI contraindications. MRI scans and clinical evaluations were conducted on the same day for each participant. We further segregated the ET and PD groups into four subgroups for analysis across groups with/without sleep disorders: ET with poor QoS (SleET), ET with normal QoS (NorET), PD with poor QoS (SlePD), and PD with normal QoS (NorPD). Poor QoS was defined in those with a PSQI score > 5 [24].
Ethics statement
Ethical approval for this study was obtained from the ethics committee of the Second Affiliated Hospital of Zhejiang University School of Medicine, and informed consent forms were signed by all participants in accordance with the Declaration of Helsinki.
Clinical assessment
Demographic data of all patients were collected, including age, sex, education, and disease duration. Subsequently, clinical assessments were conducted, covering the following domains: (1) the severity of tremor and its impact on daily life for ET patients, assessed using The Essential Tremor Rating Assessment Scale (TETRAS); (2) PD severity, assessed using Unified Parkinson’s Disease Rating Scale-Part III (UPDRS-III); and (3) PSQI score to evaluate the overall sleep quality during the past month, comprising the following domains: sleep efficiency, sleep latency, sleep duration, sleep disturbance, sleep quality, use of sleep drugs, and daytime dysfunction.
Image acquisition and processing
All participants underwent a 3.0-Tesla MRI scan using a GE Discovery MR750 3.0T MRI scanner, earplugs and foam cushions were equipped to minimize noise and head motion. Three-dimensional T1-weighted structural MRI, NM-MRI, and diffusion tensor imaging (DTI) were performed.
Three-dimensional T1-weighted images were acquired using a fast spoiled gradient sequence: echo time (TE)=3.036 ms; repetition time (TR) = 7.336 ms; inversion time = 450 ms; flip angle (FA) = 11°; field of view (FOV) = 260×260 mm2; matrix = 256×256; slice thickness = 1.2 mm; number of slices = 196 (sagittal). NM-MRI images were obtained by applying a T1-weighted fast spin echo sequence: TE = 18.6 ms; TR = 600 ms; FA = 77°; FOV = 220×220 mm2; matrix = 512×512; slice thickness = 3 mm; slice gap = 0 mm; number of slices = 17 (axial). DTI images were obtained by applying a spin echo-echo planar imaging sequence: TR = 8000 ms; TE = 80 ms; FA = 90°; FOV = 256 × 256 mm2; matrix = 128×128; slice thickness = 2 mm; slice gap = 0 mm; number of slices = 67 (axial). Diffusion images were acquired from 30 gradient directions (b = 1000 s/mm2) and included five acquisitions without diffusion weighting (b = 0). An additional b = 0 acquisition with reverse phase-encode polarity was acquired for correcting distortion. The scanning coverage extended from the top of the basal ganglia to the bottom of the medulla oblongata, and the acquisition plane was positioned perpendicular to the brainstem.
CNRLC and CNRSN calculations
Two authors (SC.L. and YL.F.), who were blinded to the subjects’ information, conducted manual measurements of LC and substantia nigra (SN) independently. The intraclass correlation coefficients of the two measurements were 0.866 and 0.828, respectively. The measurements were performed using ITK-SNAP (https://sourceforge.net/projects/itk-snap/), an open-source software toolbox for MRI image segmentation. LC is an elongated subcortical nucleus situated bilaterally at the base of the fourth ventricle within the pontine tegmentum (PT). Therefore, we delineated circular areas as regions of interest (ROIs) in three continuous slices, either with the highest SI or corresponding to the approximate anatomical location of the LC. A larger ROI of PT was also selected on the same slice to calculate the contrast-to-noise ratio for the LC (CNRLC; Fig. 1). The methods for outlining the SN were described in our study [25]. The mean and standard deviation (SD) of the SI were calculated for LC, SN, PT, and cerebral peduncle (CP). The formula for calculating CNRLC and CNRSN was (SISN/LC –SICP/PT)/SDCP/PT and bilateral CNRLC/SN values of each sample were averaged for final evaluation.
Fig. 1
The anatomical location of the locus coeruleus (LC) and pontine tegmentum (PT). An NM-MRI slice of a healthy control (A) and the corresponding selected regions of interest (ROIs) of LC and PT (B).
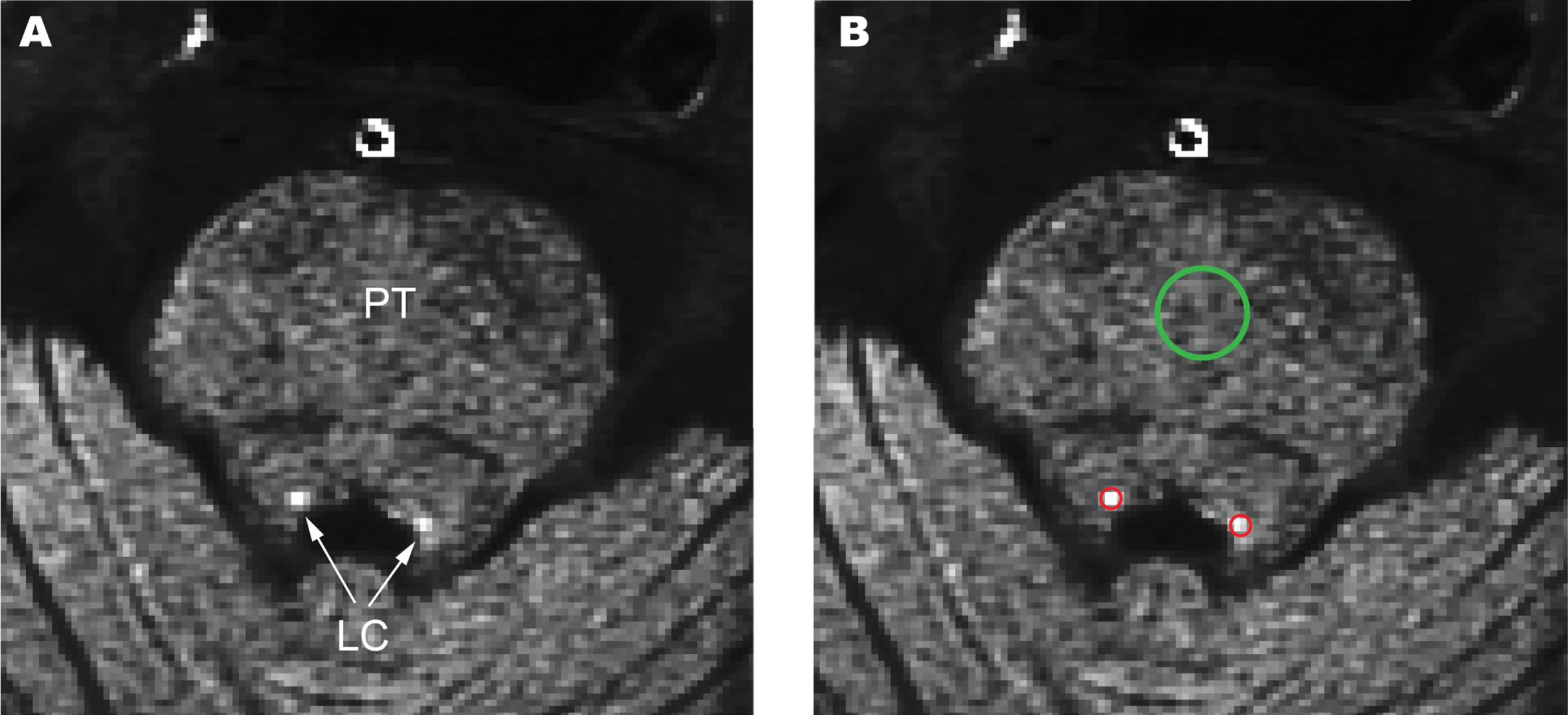
DTI data processing
MRtrix3 (www.mrtrix.org) and FMRIB Software Library (FSL, http://www.fmrib.ox.ac.uk./fsl) were used to process diffusion data. These data were first preprocessed by denoising and removing Gibbs ringing artifacts. Subsequently, topup and eddy in FSL were performed to correct susceptibility-induced and eddy-current-induced distortion, and motion effects in the diffusion data [26]. Next, the skulls were stripped from the DTI data for each participant. To acquire and analyze free-water data, a bi-tensor model from a previous study was applied for predicting intracellular and extracellular water components [27]. FA maps in native space were linearly and nonlinearly registered to the FA template in Montreal Neurological Institute (MNI) space using FSL. Subsequently, FW maps of the subjects were warped using the transformation matrix from the FA maps. Extraction of free-water data of LC (FWLC) based on a probabilistic atlas with peak signal coordinates observed at two SDs [28].
Statistical analysis
Statistical Package for the Social Sciences (SPSS), version 27, was used for statistical analysis. Differences in demographic and clinical variables among the ET, PD, and HC groups were analyzed using analysis of variance (ANOVA) for continuous variables and chi-squared testing for nominal variables. Differences in MRI variables (CNRLC and FWLC) were analyzed using a general linear model (GLM), with “sleep medication” set as a covariate. Post-hoc Bonferroni correction was applied to compare the differences between the two groups. Two-sample student’s t-tests were conducted to compare the differences between the SleET and NorET subgroups, as well as between the SlePD and NorPD subgroups. Partial correlation analysis was used to examine the correlation between CNRLC and FWLC and the total score and subscale PSQI scores (including seven domains) in ET, PD, SleET, and SlePD groups; age, sex, education, and disease duration were set as covariates, while UPRDRS-III was additionally included as a covariate in PD and SlePD groups. False discovery rate (FDR) correction was applied for multiple comparison correction. Statistical significance was defined as a p-value<0.05.
RESULTS
Demographics and clinical data
No significant differences in age, sex, and education were found among the ET, PD, and HC groups. Disease duration was significantly longer in the ET group than in the PD group (p<0.001). PSQI scores were significantly higher in both the ET and PD groups compared to the HC group (p<0.001), whereas no significant difference was found between the ET and PD groups. In addition, use of sleep medication was significantly different between ET and HC (p = 0.013), as well as between PD and HC (p = 0.008; Table 1).
Table 1
The demographic and clinical variables of ET, PD, and HC
Variables | ET | PD | HC | p | post-hoc tests p | ||
(n = 83) | (n = 124) | (n = 83) | HC vs. ET | HC vs. PD | ET vs. PD | ||
Age (y) | 60.76±8.45 | 60.06±8.43 | 61.58±7.97 | 0.435 | – | – | – |
Sex (M/F) | 43/40 | 63/61 | 38/45 | 0.699 | – | – | – |
Education (y) | 9.23±2.55 | 8.67±3.72 | 9.28±3.66 | 0.358 | – | – | – |
Disease duration (y) | 13.88±9.99 | 3.44±3.94 | – | <0.001*** | – | – | – |
TETRAS | 32.66±17.49 | – | – | – | – | – | – |
UPDRS-III | – | 22.71±14.09 | – | – | – | – | – |
PSQI | 4.92±4.04 | 4.62±3.57 | 2.75±1.34 | <0.001*** | <0.001*** | <0.001*** | 1.000 |
Sleep medication (Y/N) | 6/77 | 10/114 | 0/83 | 0.032* | 0.013* | 0.008** | 0.825 |
ET, essential tremor; PD, Parkinson’s disease; HC, healthy control; TETRAS, The Essential Tremor Rating Assessment Scale; UPDRS, Unified Parkinson’s Disease Rating Scale; PSQI, Pittsburgh Sleep Quality Index. *p<0.05, **p<0.01, ***p<0.001.
No significant differences in age, sex, education, and disease duration were found between the SleET and NorET groups, or between the SlePD and NorPD groups. Whereas the TETRAS score in the SleET and NorET groups did not differ, the UPDRS-III score of the SlePD group was significantly higher than that of the NorPD group (p = 0.009). The use of sleep medication was significantly higher in the SleET group than in NorET (p = 0.040), and also in SlePD compared to NorPD. (p<0.001; Table 2).
Table 2
The demographic and clinical variables of SleET, NorET, SlePD and NorPD
Variables | ET | p | PD | p | ||
SleET (n = 30) | NorET (n = 53) | SlePD (n = 40) | NorPD (n = 84) | |||
Age (y) | 61.64±9.02 | 60.26±8.15 | 0.479 | 60.77±8.23 | 59.72±8.55 | 0.519 |
Sex (M/F) | 16/14 | 27/26 | 0.834 | 17/23 | 46/38 | 0.202 |
Education (y) | 8.67±2.50 | 9.55±2.55 | 0.132 | 8.48±3.48 | 8.77±3.85 | 0.684 |
Disease duration (y) | 11.81±8.96 | 15.06±10.42 | 0.156 | 3.48±3.38 | 3.42±4.19 | 0.937 |
TETRAS | 30.58±16.58 | 33.83±18.04 | 0.420 | – | – | – |
UPDRS-III | – | – | – | 27.48±16.49 | 20.44±12.26 | 0.009** |
PSQI | 9.50±2.92 | 2.32±1.43 | <0.001*** | 8.95±2.71 | 2.56±1.47 | <0.001*** |
Sleep medication (Y/N) | 5/25 | 1/52 | 0.040 | 9/31 | 1/83 | <0.001*** |
SleET, essential tremor with poor QoS; NorET, essential tremor without poor QoS; SlePD, Parkinson’s disease with poor QoS; NorPD, Parkinson’s disease without poor QoS; TETRAS, The Essential Tremor Rating Assessment Scale; UPDRS, Unified Parkinson’s Disease Rating Scale; PSQI, Pittsburgh Sleep Quality Index. *p<0.05, **p<0.01, ***p<0.001.
CNRLC and FWLC data
Initially, the CNRLC and FWLC results were compared among the ET, PD, and HC groups. Post-hoc tests showed that both ET and PD groups exhibited significantly lower CNRLC (p = 0.047, p = 0.018; Fig. 2A) compared to the HC group, but no significant difference was found between ET and PD groups. The GLM values of FWLC were not significantly different between the ET, PD, and HC groups. (Fig. 2B).
Fig. 2
Comparison of CNRLC and FWLC in HC, ET, and PD groups. The result of ANOVA and post-hoc Bonferroni test of CNRLC (A) and FWLC (B) among these groups. CNRLC, contrast-to-noise ratio of locus coeruleus; FWLC, free-water value of locus coeruleus; HC, healthy control; ET, essential tremor; PD, Parkinson’s disease.
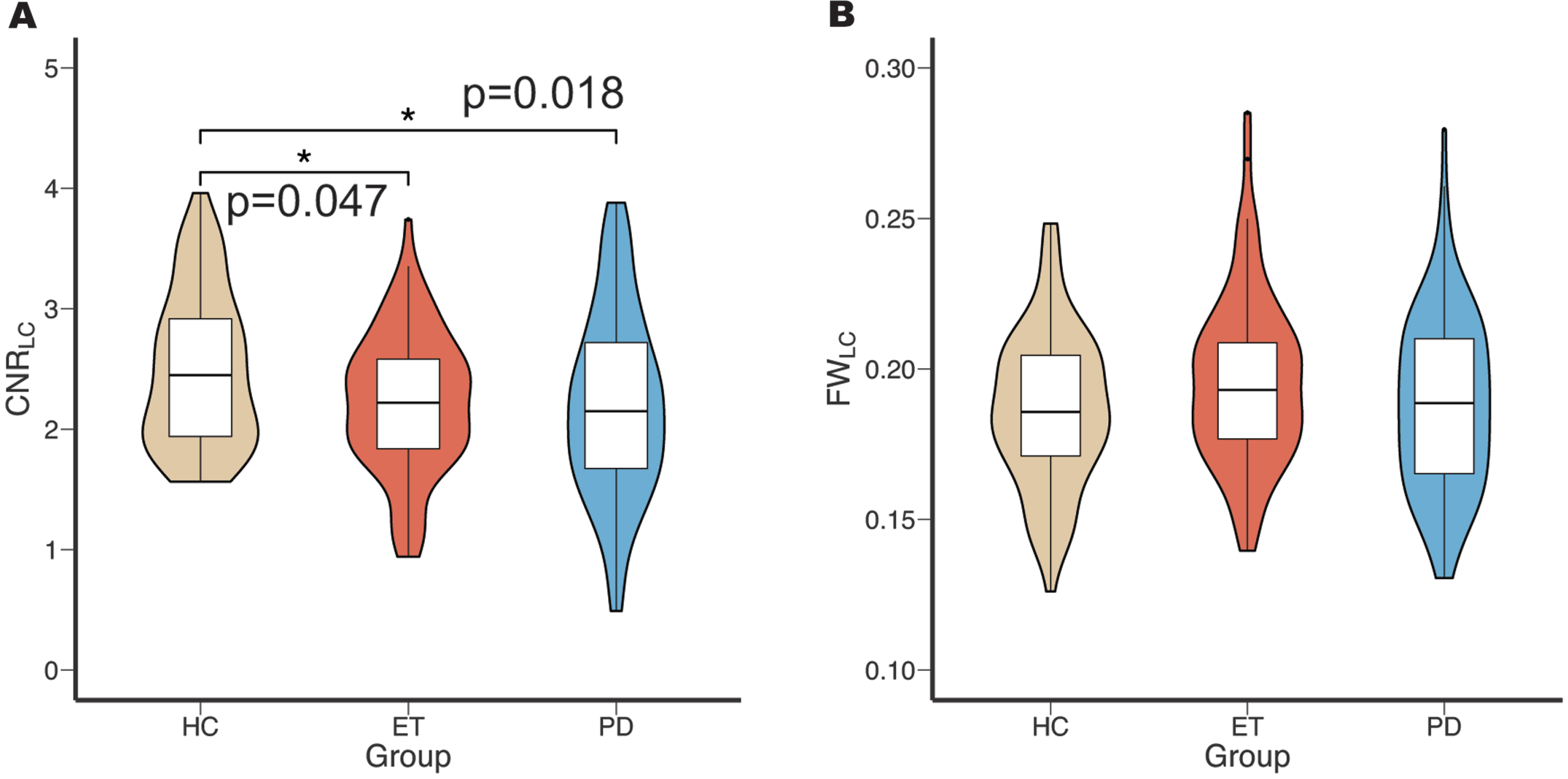
Subsequently, in the subgroup analysis, there was no significant difference in CNRLC and FWLC between either ET or PD subgroups (Fig. 3). Furthermore, considering the potential impact of both the different usage of sleep medication in subgroups and the difference in UPDRS-III score between SlePD and NorPD groups, an additional analysis of covariance (ANCOVA) was performed, with sleep medication and UPDRS-III score set as covariates. This analysis still did not reveal any difference between these groups.
Fig. 3
Comparison of CNRLC and FWLC values in ET and PD subgroups. The result showed no significant difference in CNRLC and FWLC between SleET and NorET (A, B), SlePD and NorPD (C, D). CNRLC, contrast-to-noise ratio of locus coeruleus, FWLC, free-water value of locus coeruleus, SleET, essential tremor with poor QoS; NorET, essential tremor without poor QoS; SlePD, Parkinson’s disease with poor QoS; NorPD, Parkinson’s disease without poor QoS.
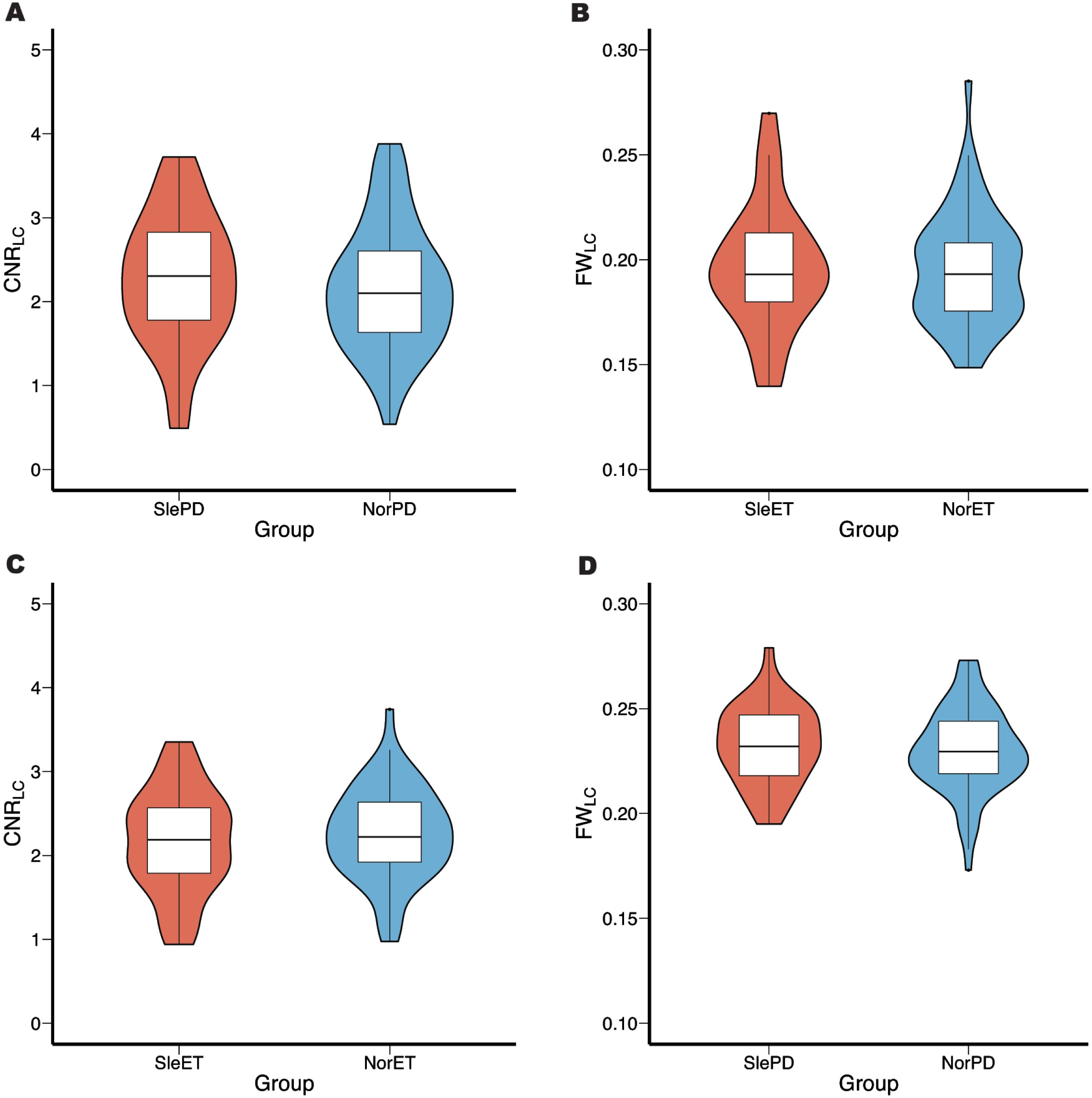
Correlation between CNR/FWLC and PSQI score
After adjustment of age, sex, education, disease duration, UPDRS-III score, use of sleep medication by FDR correction, no significant correlations were found between CNRLC and FWLC and total and subscale PSQI scores in ET, SleET, PD, and SlePD groups (Fig. 4).
Fig. 4
Correlations between CNRLC and FWLC with the total score and subscale scores of PSQI. No significant correlations were found between CNRLC (A, C) and FWLC (B, D) with the total score in ET, SleET, PD, and SlePD groups, nor were found between CNRLC and FWLC with the subscale scores (E). CNRLC, contrast-to-noise ratio of locus coeruleus, FWLC, free-water value of locus coeruleus, SleET, essential tremor with poor QoS; SlePD, Parkinson’s disease with poor QoS.
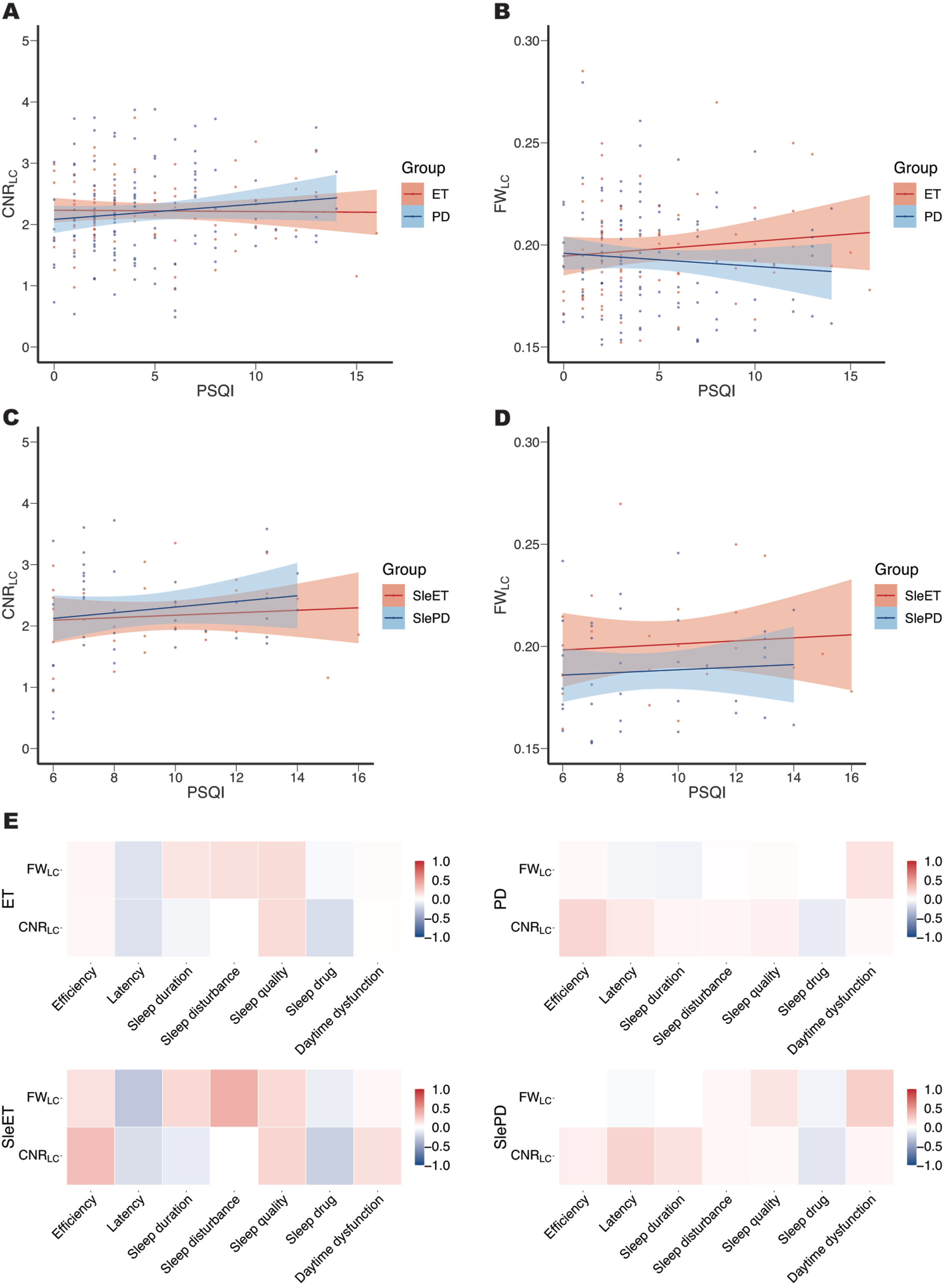
DISCUSSION
In this study, we used multi-modal MRI to assess LC integrity and investigate its relationship with overall sleep quality in ET and PD patients. A significant reduction of CNRLC was observed in both ET and PD patients compared to the HC group. FWLC values tended to be higher in ET and PD groups, although the differences were not statistically significant. However, no difference was found in CNRLC or FWLC between patients with and without poor QoS. The degree of LC impairment was not associated with the PSQI score.
It was demonstrated using NM-MRI that LC is impaired in both ET and PD patients. However, free-water analysis revealed no significant difference but only a tendency for LC impairment in ET and PD groups. This discrepancy could be attributed to the limited resolution of DTI, which might be inadequate for accurately resolving LC structure [29]. Compared to free-water imaging, NM-MRI is a preferable method for the assessment of LC impairment. Indeed, the aforementioned results are consistent with previous MRI studies [19, 21, 30] and post-mortem studies [31–33] demonstrating LC impairment in ET and PD. However, a smaller-scale study reported no significant difference in CNRLC between HC and ET patients, but a difference between ET and tremor-dominant PD (PDTD) patients was observed [34]. This might be attributed to the limited sample size, which did not account for the neuropathological heterogeneity of these diseases. Furthermore, the disease duration of ET patients in that study was shorter than in ours, which might explain the lesser extent of LC impairment.
The LC contains a number of norepinephrinergic neurons, the projections of which extensively reach the basal ganglia, cortex, and cerebellum, and participate in the regulation of cognition, emotion, and sleep [35]. The investigation of the association between LC impairment and overall sleep quality revealed no significant differences in CNR/FWLC between the subgroups of the two diseases, respectively. There were also no correlations between CNR/FWLC and PSQI scores in ET, PD, SleET, and SlePD groups. PSQI assesses seven aspects of sleep, encompassing sleep quality, sleep latency, nocturnal sleep duration, sleep efficiency, sleep disturbances, sleep medication use, and daytime dysfunction. Previous studies have demonstrated correlations between specific PSQI domains such as prolonged sleep latency or increased daytime sleepiness and neurodegeneration [36]. However, our analysis did not yield any significant correlations between the PSQI domains and LC impairment. Several explanations might account for these findings. Firstly, LC damage in either diseases might not substantially contribute to the deterioration of the overall sleep quality for these patients. Secondly, previous research on RBD in individuals with PD has demonstrated an association between RBD and impairment of the LC [37, 38]. However, it is important to note that PSQI total score and subscale scores, which cover seven domains of sleep quality, might not provide a complete understanding of the intricate relationship between the LC and sleep. This might be because the LC specifically influences certain aspects of sleep that PSQI does not assess, such as RBD and arousal, while other factors such as functional brain networks and lower cerebellar volume might also impair sleep quality [39, 40].
Limitations
This study has several limitations. Firstly, as PSQI is a self-reported questionnaire, polysomnography offers greater advantages in providing a more objective description of sleep patterns. Given that our MRI scan resolution is limited to a slice thickness of 3 mm, which is prone to partial-volume effects, further studies with higher resolution and isotropic LC imaging are needed to confirm the present findings [41]. Also, a more direct method, such as positron emission tomography to measure noradrenergic transporter levels, might provide valuable insights.
Conclusion
We observed significant LC impairment in both ET and PD individuals, which might be involved in the pathophysiology of both diseases. In addition, there was no difference in LC integrity between groups with/without poor QoS, nor was there a correlation with PSQI score. Our findings suggest that LC impairment might not be directly linked to overall sleep quality. More experiments should be conducted to further support this conclusion.
ACKNOWLEDGMENTS
We thank all the patients, healthy controls, and their families for their participation in data acquisition.
FUNDING
This work was supported by the Major Health Science and Technology Program of Zhejiang Province (No. WKJ-ZJ-2208) and the Medical and Health Research Project of Zhejiang Province (No. 2021RC065).
CONFLICT OF INTEREST
The authors have no conflict of interest to report.
DATA AVAILABILITY
The data supporting the findings of this study are available on request from the correspondence authors. Detailed original data are not publicly available due to privacy and ethical issues.
REFERENCES
[1] | Bhatia KP , Bain P , Bajaj N , Elble RJ , Hallett M Louis ED , Raethjen J , Stamelou M , Testa CM , Deuschl G ((2018) )Tremor Task Force of the International Parkinson and Movement Disorder Society Consensus Statement on the Classification of Tremors. From the Task Force on Tremor of the International Parkinson and Movement Disorder Society. Mov Disord. 33: , 75–87. |
[2] | Jankovic J ,((2008) )Parkinson’s disease: Clinical features and diagnosis. J Neurol Neurosurg Psychiatry. 79: , 368–376. |
[3] | Lee SM , Kim M , Lee HM , Kwon KY , Koh SB ((2015) )Nonmotor symptoms in essential tremor: Comparison with Parkinson’s disease and normal control. J Neurol Sci. 349: , 168–173. |
[4] | Gerbin M , Viner AS , Louis ED , ((2012) )Sleep in essential tremor: Acomparison with normal controls and Parkinson’s disease patients. Parkinsonism Relat Disord. 18: , 279–284. |
[5] | Barut BO , Tascilar N , Varo A ,((2015) ) Sleep disturbances in essential tremor and Parkinson disease: A polysomnographic study. J Clin Sleep Med. 11: , 655–662. |
[6] | Aarsland D , Batzu L , Halliday GM , Geurtsen GJ , Ballard C Chaudhuri KR , Weintraub D ,((2021) )Parkinson disease-associated cognitive impairment. Nat Rev Dis Primers. 7: , 47. |
[7] | Miller KM , Okun MS , Fernandez HF , Jacobson CE , Rodriguez RL , Bowers D ((2007) ) Depression symptoms in movement disorders: Comparing Parkinson’s disease, dystonia, and essential tremor. Mov Disord. 22: , 666–672. |
[8] | Smeltere L , Kuznecovs V , Erts R ,((2017) ) Depression and social phobia in essential tremor and Parkinson’s disease. Brain Behav. 7: , e00781. |
[9] | Lin W , Lin YK , Yang FC , Chung CH , Hu JM Tsao CH , Weng ZX , Ko CA , Chien WC , xxx xxx ((2023) ) Risk of neurodegenerative diseases in patients with sleep disorders: A nationwide population-based case-control study. Sleep Med. 107: , 289–299. |
[10] | Malhotra RK ,((2022) ) Neurodegenerative disorders and sleep. Sleep Med Clin. 17: , 307–314. |
[11] | Husain M ,((2021) ) Sleep and neurodegenerative diseases. Brain. 144: , 695–696. |
[12] | de Almeida FO , Ugrinowitsch C , Brito LC , Milliato A , Marquesini R Moreira-Neto A , Barbosa ER , Horak FB , Mancini M , Silva-Batista C ((2021) ) Poor sleep quality is associated with cognitive, mobility, and anxiety disability that underlie freezing of gait in Parkinson’s disease. Gait Posture. 85: , 157–163. |
[13] | Saper CB , Scammell TE , Lu J ,((2005) )Hypothalamic regulation of sleep and circadian rhythms. Nature. 437: 1257–1263. |
[14] | Van Egroo M , Koshmanova E , Vandewalle G , Jacobs HIL ,((2022) ) Importance of the locus coeruleus-norepinephrine system in sleep-wake regulation: Implications for aging and Alzheimer’s disease. Sleep Med Rev. 62: 101592. |
[15] | Hayat H , Regev N , Matosevich N , Sales A , Paredes-Rodriguez E Krom AJ , Berg L , Li Y , Lavigne M , Kremer EJ Yizhar O , Pickering AE , Nirl Y ,((2020) ) Locus coeruleus norepinephrine activity mediates sensory-evoked awakenings from sleep. Sci Adv. 6: , eaaz4232. |
[16] | Steriade M ,((1996) ) Arousal: Revisiting the reticular activating system. Science. 272: , 225–226. |
[17] | Scammell TE , Arrigoni E , Lipton JO ,((2017) )Neural circuitry of wakefulness and sleep. Neuron. 93: 747–765. |
[18] | Zerbi V , Floriou-Servou A , Markicevic M , Vermeiren Y , Sturman O Privitera M , von Ziegler L , Ferrari KD , Weber B , De Deyn PP Wenderoth N , Bohacek J ,((2019) ) Rapid reconfiguration of the functional connectome after chemogenetic locus coeruleus activation. Neuron. 103: , 702–108. |
[19] | Nobileau A , Gaurav R , Chougar L , Faucher A , Valabregue R Mangone G , Leu-Semenescu S , Lejeune FX , Corvol JC , Arnulf I Vidailhet M , Grabli D , Degos B , Lehericy S ,((2023) ) Neuromelanin-sensitive magnetic resonance imaging changes in the locus coeruleus/subcoeruleus complex in patients with typical and atypical parkinsonism. Mov Disord. 38: , 479–484. |
[20] | Doppler CEJ , Smit JAM , Hommelsen M , Seger A , Horsager J Kinnerup MB , Hansen AK , Fedorova TD , Knudsen K , Otto M Nahimi A , Borghammer P , Sommerauer M ,((2021) )Microsleep disturbances are associated with noradrenergic dysfunction in Parkinson’s disease. Sleep. 44: , zsab040. |
[21] | Lv DY , Zhou C , Pu JL , Lu JY , Zhao GH Gu LY , Guan XJ , Guo T , Xu XJ , Zhang MM Tian J , Yin XZ , Zhang BR , Zhao GH , Yan YP ((2023) ) Locus coeruleus degeneration and cerebellar gray matter changes in essential tremor. J Neurol. 270: , 780–787. |
[22] | Chu WT , Wang WE , Zaborszky L , Golde TE , DeKosky S Duara R , Loewenstein DA , Adjouadi M , Coombes SA , Vaillancourt DE ((2022) ) Association of cognitive impairment with free water in the nucleus basalis of meynert and locus coeruleus to transentorhinal cortex tract. Neurology. 98: , E700–E710. |
[23] | Postuma RB , Berg D , Stern M , Poewe W , Olanow CW Oertel W , Obeso J , Marek K , Litvan I , Lang AE Halliday G , Goetz CG , Gasser T , Dubois B , Chan P Bloem BR , Adler CH , Deuschl G ,((2015) ) MDS clinical diagnostic criteria for Parkinson’s disease. Mov Disord. 30: , 1591–1599. |
[24] | Mollayeva T , Thurairajah P , Burton K , Mollayeva S , Shapiro CM ((2016) )Colantonio A The Pittsburgh sleep quality index as a screening tool for sleep dysfunction in clinical and non-clinical samples: A systematic review and meta-analysis. Sleep Med Rev. 25: , 52–73. |
[25] | Zhou C , Wang LB , Cheng W , Lv JC , Guan XUJ , Guo T , Wu JJ , Zhang W , Gao T , Liu XC , Bai XQ , Wu HT , Cao ZY , Gu LY , Chen JW , Wen JQ , Huang PY , Xu XJ , Zhang BR , Feng XUJ , Zhang MM ,((2023) ) Two distinct trajectories of clinical and neurodegeneration events in Parkinson’s disease. NPJ Parkinsons Dis. 9: , 111. |
[26] | Andersson JLR , Sotiropoulos SN ,((2016) )An integrated approach to correction for off-resonance effects and subject movement in diffusion MR imaging. Neuroimage. 125: , 1063–1078. |
[27] | Zhou LC , Li GL , Zhang YY , Zhang M , Chen ZC Zhang LN , Wang XJ , Zhang M , Ye GY , Li YY Chen SD , Li BA , Wei HJ , Liu J ,((2021) )Increased free water in the substantia nigra in idiopathic REM sleep behaviour disorder. Brain. 144: , 1488–1497. |
[28] | Sun W , Tang YC , Qiao YC , Ge XT , Mather M Ringman JM , Shi YG ,((2020) ) A probabilistic atlas of locus coeruleus pathways to transentorhinal cortex for connectome imaging in Alzheimer’s disease. Neuroimage. 223: , 117301. |
[29] | Langley J , Hussain S , Flores JJ , Bennett IJ , Hu XP Characterization of age-related microstructural changes in locus coeruleus and substantia nigra pars compacta. Neurobiol Aging. 87: ((2020) )89–97. |
[30] | Wang J , Li Y , Huang Z , Wan W , Zhang Y Wang C , Cheng X , Ye F , Liu K , Fei G Zeng M , Jin L ,((2018) )Neuromelanin-sensitive magnetic resonance imaging features of the substantia nigra and locus coeruleus in de novo Parkinson’s disease and its phenotypes. Eur J Neurol. 25: , 949. |
[31] | Louis ED , Faust PL , Vonsattel JPG , Honig LS , Rajput A Robinson CA , Rajput A , Pahwa R , Lyons KE , Ross GW Borden S , Moskowitz CB , Lawton A , Hernandez N ,((2007) )Neuropathological changes in essential tremor: 33 cases compared with 21 controls. Brain. 130: , 3297–3307. |
[32] | Shill HA , Adler CH , Sabbagh MN , Connor DJ , Caviness JN Hentz JG , Beach TG ,((2008) )Pathologic findings in prospectively ascertained essential tremor subjects. Neurology. 70: , 1452–1455. |
[33] | Seidel K , Mahlke J , Siswanto S , Krüger R , Heinsen H Auburger G , Bouzrou M , Grinberg LT , Wicht H , Korf HW den Dunnen W , Rüb U ,((2015) )The brainstem pathologies of Parkinson’s disease and dementia with Lewy bodies. Brain Pathol. 25: , 121–135. |
[34] | Wang XH , Huang P , Haacke EM , Liu Y , Zhang YM Jin ZJ , Li Y , Xu QY , Liu P , Chen SD He NY , Yan FH ,((2023) ) Locus coeruleus and substantia nigra neuromelanin magnetic resonance imaging differentiates Parkinson’s disease and essential tremor. Neuroimage Clin. 38: , 103420. |
[35] | Tsukahara JS , Engle RW ,((2021) )Fluid intelligence and the locus coeruleus-norepinephrine system. Proc Natl Acad Sci U S A. 118: , e2110630118. |
[36] | Xu W , Tan L , Su BJ , Yu H , Bi YL Yue XF , Dong Q , Yu JT ,((2020) )Sleep characteristics and cerebrospinal fluid biomarkers of Alzheimer’s disease pathology in cognitively intact older adults: The CABLE study. Alzheimers Dement. 16: , 1146–1152. |
[37] | García-Lorenzo D , Longo-Dos Santos C , Ewenczyk C , Leu-Semenescu S , Gallea C Quattrocchi G , Lobo PP , Poupon C , Benali H , Arnulf I Vidailhet M , Lehericy S ,((2013) )The coeruleus/subcoeruleus complex in rapid eye movement sleep behaviour disorders in Parkinson’s disease. Brain. 136: , 2120–2129. |
[38] | Tan SJ , Zhou C , Wen JQ , Duanmu X , Guo T Wu HT , Wu JJ , Cao ZY , Liu XC , Chen JW Wu CQ , Qin JM , Xu JJ , Gu LY , Yan YP Zhang BR , Zhang MM , Guan XJ , Xu XJ , ((2023) )Presence but not the timing of onset of REM sleep behavior disorder distinguishes evolution patterns in Parkinson’s disease. Neurobiol Dis. 180: 106084. |
[39] | Peng JX , Yang J , Li JY , Lei D , Li NN Suo XL , Duan LR , Chen CL , Zeng Y , Xi J Jiang Y , Gong QY , Peng R , ((2022) )Disrupted brain functional network topology in essential tremor patients with poor sleep quality. Front Neurosci. 16: , 814745. |
[40] | Yang J , Lei D , Peng JX , Suo XL , Pinaya WHL Li WB , Kemp GJ , Peng R , Gong QY ,((2021) ) Disrupted brain gray matter networks in drug-naive participants with essential tremor. Neuroradiology. 63: , 1501–1510. |
[41] | Priovoulos N , Jacobs HIL , Ivanov D , Uludag K , Verhey FRJ , Poser BA ,((2018) ) High-resolution imaging of human locus coeruleus by magnetization transfer MRI at 3T and 7T. Neuroimage. 168: , 427–436. |