Relationship between inflammatory laboratory parameters and severity of adolescent idiopathic scoliosis: A pilot study
Abstract
BACKGROUND:
Adolescent idiopathic scoliosis is a complex condition whose pathogenesis may include inflammation and signs of joint and bone degeneration.
OBJECTIVE:
The main objective of this study is to evaluate the relationship between the severity of adolescent idiopathic scoliosis and inflammatory blood parameters.
METHODS:
The study recruited patients with adolescent idiopathic scoliosis who attended the Rehabilitation Center of the Apostolo Foundation in Merate (LC). The scoliosis curve (Cobb’s angle) was used as a severity index to compare with inflammatory blood parameters (white blood cells subpopulations, immunoglobulins, protein electrophoresis). In addition, the study used an overall severity grading called “Scoliosis Score” which includes all spine angles and Risser’s score (bone development index).
RESULTS:
Thirty-four subjects were recruited (mean age 14 years, 2 months), 30 females and 2 males. A significant correlation was found between Cobb’s angle and the percentage values of beta-2 globulins in a directly proportional manner (
CONCLUSION:
The correlation between beta-2 globulins and gamma globulins with Cobb’s angle and the Scoliosis Score suggests a link between spinal curvature and inflammation in scoliosis patients, This link may indicate the significance of these parameters for diagnosing, staging the disease, and monitoring therapies.
1.Introduction
Adolescent idiopathic scoliosis (AIS) is a spinal deformity that affects approximately 2% to 3% of the pediatric population, typically occurring between the ages of 11 and 18 [1]. AIS is a complex and highly diverse condition that remains incompletely understood, particularly with regard to its underlying causes and associated conditions. It is evident that AIS is a polygenic disorder, influenced by various genetic, epigenetic, neurological, hormonal/metabolic, and environmental factors, all contributing to its development [2].
Some studies have proposed a potential connection between the skeletal muscles and the immune system in AIS [3, 4]. This interplay between the musculoskeletal and immune system is notably pronounced in rheumatic diseases, such as rheumatoid arthritis and osteoarthritis [5], as well as in orthopedic disorders linked to intervertebral disc degeneration [6].
However, the relationship between the immune system and scoliosis remains relatively understudied. Recent investigations have explored the role of immune imbalances and inflammation in individuals with AIS. For instance, Rudapatna and colleagues observed macrophage infiltration and fibrosis in the paraspinal muscles of AIS patients [4], while Wang and colleagues detected several inflammatory markers in the plasma of this population [7]. Additionally, altered expression of dystrophin proteins and inflammatory changes, including T cell infiltration in the paraspinal muscles, has been noted [8].
In this study, we postulate that AIS is a multifactorial condition in which the interaction between the musculoskeletal and immune systems plays a significant role. Furthermore, we propose that the Cobb’s angle may not fully capture the severity of scoliosis, prompting us to develop an index that offers a more comprehensive assessment of clinical severity in affected individuals.
2.Methods
This is a retrospective observational study conducted at the Rehabilitation Center of the Apostolo Foundation in Merate (LC), Italy. This study included all consecutive patients with AIS who visited our outpatient clinic during a 7-year period. All tests were conducted on an outpatient basis at the University Hospital of Verona. Data were collected retrospectively and evaluated at the Department of Diagnostics and Public Health, University of Verona, specifically in the Section of Epidemiology and Medical Statistics. We reviewed the medical records of eligible patients to extract relevant laboratory data. The data evaluation and analysis were performed retrospectively at the time of subject enrollment, meaning that there was no ongoing monitoring or follow-up of patients over a specific timeframe. Inclusion criteria comprised a previous diagnosis of AIS, a Cobb’s angle of at least 10 degrees (ensured by correctly positioned anteroposterior X-rays), age between 10 and 18 years, and patient comprehension of the study’s methods and objectives. Exclusion criteria were: high-dose steroid therapy, anti-thrombotic drugs, immunosuppressive therapy, active infection within 15 days before participation, autoimmune diseases, and pregnancy. As a retrospective observational study, our research did not involve any procedures or interventions that deviated from the standard clinical routine analysis. Patients provided consent for data processing, with parental consent obtained for minors, ensuring anonymity. The study’s principal investigator and research team ensured participant confidentiality. Sensitive data of recruited subjects were processed in accordance with EU Regulation 2016/679 (GDPR) and Legislative Decree 30/6/2003, n. 196 (Code regarding the protection of personal data). Only researchers directly involved in the analysis had access to electronically stored data on an encrypted access computer throughout all study phases. The study adhered to the principles of the 1964 Declaration of Helsinki and its subsequent amendments.
To assess scoliosis severity, we employed the Cobb’s angle, representing the maximum angle recorded in any position. Cobb’s angle is measured by identifying the most tilted vertebrae in each curve. Lines are drawn along the tops and bottoms of these vertebrae, and two additional lines are drawn perpendicularly at a 90-degree angle to the first lines. The angle is measured at the intersection of these lines, expressed in degrees [9, 10].
Table 1
Characteristics of the sample (
mean | SD | median | 25th Perc. | 75th Perc. | Min | Max | |
Cobb’s (degrees) | 54.09 | 24.56 | 55 | 38 | 65.5 | 14 | 116 |
Scoliosis Score | 65.17 | 41.34 | 58.45 | 40.82 | 77.42 | 14.6 | 211.2 |
Age (years) | 14.26 | 3.74 | 14 | 13 | 16 | 5 | 23 |
WBC (103/ | 6.75 | 1.88 | 6.88 | 5.60 | 7.68 | 3.13 | 11.16 |
Neutrophils (%) | 49.87 | 11.51 | 49.15 | 41.85 | 60.18 | 28.50 | 69.80 |
Lymphocytes (%) | 38.45 | 10.56 | 34.95 | 29.13 | 45.88 | 22.80 | 58.60 |
Monocytes (%) | 5.94 | 2.03 | 5.35 | 4.68 | 6.93 | 3.90 | 13.40 |
Eosinophils (%) | 2.83 | 2.13 | 2.05 | 1.40 | 3.78 | 0.00 | 9.40 |
alfa1 globulins (%) | 3.78 | 0.50 | 3.7 | 3.4 | 4.1 | 2.7 | 4.9 |
alfa2 globulins (%) | 9.79 | 1.35 | 9.95 | 8.75 | 10.77 | 7.4 | 12.6 |
beta1 globulins (%) | 5.60 | 0.71 | 5.55 | 5.02 | 5.97 | 4.4 | 7.1 |
beta2 globulins (%) | 4.01 | 0.69 | 4 | 3.6 | 4.37 | 2.7 | 5.6 |
Gamma globulins (%) | 15.57 | 2.83 | 15.25 | 14.22 | 17.2 | 9.4 | 20.9 |
IgG (g/L) | 11.66 | 2.47 | 11.55 | 10.40 | 13.28 | 6.99 | 17.90 |
IgA (g/L) | 1.50 | 0.65 | 1.23 | 1.04 | 1.82 | 0.72 | 2.95 |
IgM (g/L) | 1.31 | 0.54 | 1.27 | 0.89 | 1.63 | 0.59 | 2.49 |
SD: Standard deviation. WBC: White blood cells.
Additionally, we introduced an overall severity grading, provisionally named the “Scoliosis Score,” which considers all spinal curvatures and the bone development index, offering a single numerical value suitable for statistical analysis. The severity of scoliosis is defined as follows: Scoliosis Score
2.1Statistical analysis
The data were summarized using mean, standard deviation, median, interquartile range and minimum and maximum. The Shapiro-Wilk test was used to test the normality of the distribution of variables.
Pearson’s correlation r was used to measure the degree of association between grade of scoliosis and the other variables. According to Colton [13], an r between 0–0.25 shows an absent or low correlation, 0.25–0.5 a decent one, 0.5–0.75 from moderate to good, 0.75–1 from very good to excellent correlation. A
Multiple linear regression models were used to further investigate the relation between the laboratory parameters, as dependent variables, and Cobb’s angle and Scoliosis Score each as independent variables in different models adjusting for age.
Statistical analyses were performed with Stata 16.1 (www.stata.com).
3.Results
We analyzed clinical and laboratory data from 34 subjects with AIS (Cobb’s angle
Figure 1.
A. Correlation between the Cobb’s angle and the Scoliosis Score. B. Correlation between Cobb’s angle and the Scoliosis Score, without males.
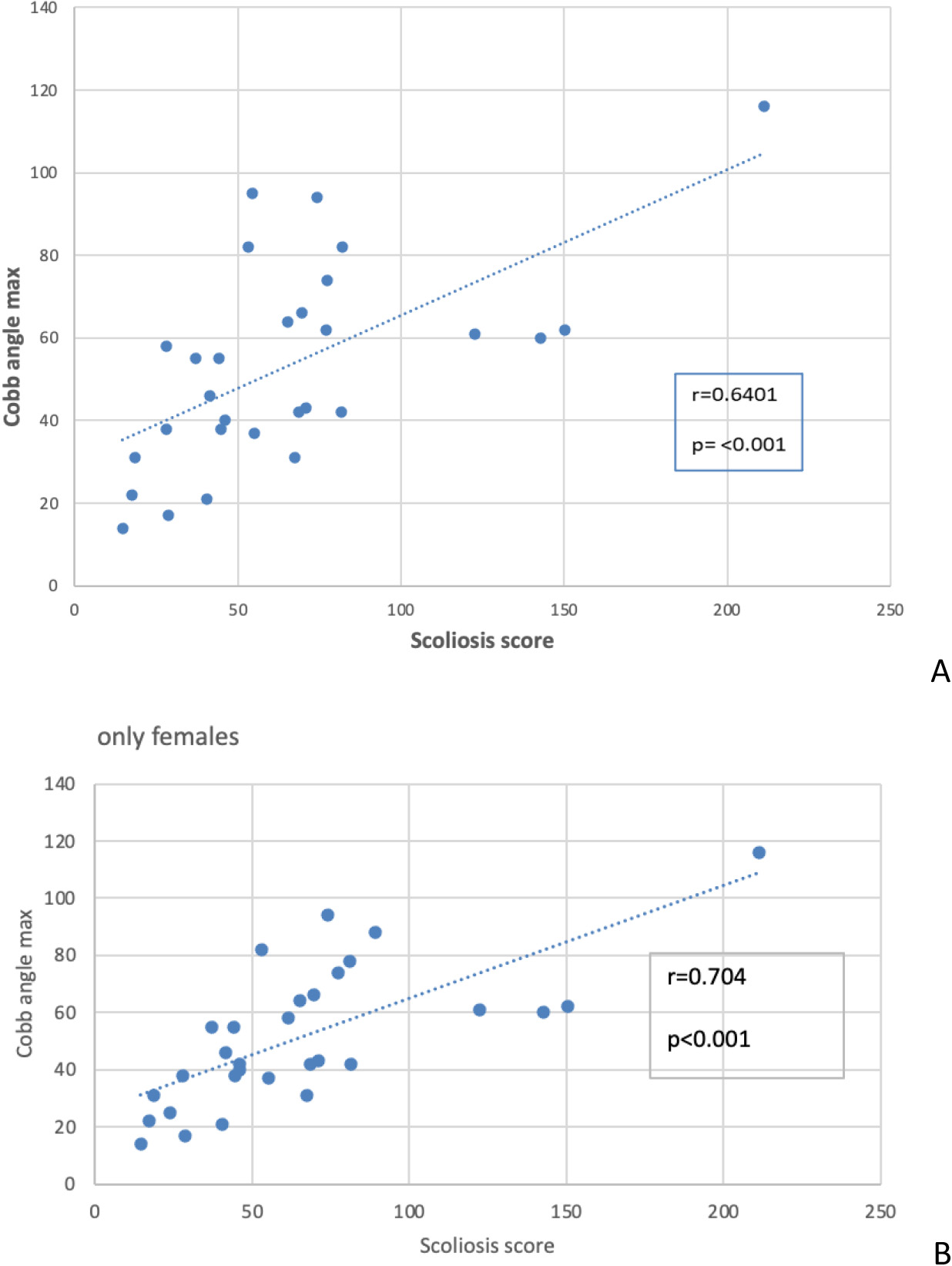
First, we assessed the correlation between the Cobb’s angle and the Scoliosis Score (Fig. 1A). While these two parameters showed a correlation it was not entirely definitive, suggesting that other factors or measurement errors might influence the test results. A sensitivity analysis on this correlation yielded consistent results, with only a slight reduction in the correlation coefficient (from 0.64 to 0.61) after removing outliers, maintaining statistical significance (data not shown). Notably, when considering only female participants (Fig. 1B), the correlation coefficient increased, both with and without outliers (data not shown).
Figure 2.
Scatter plot of the association of the angle of Cobb of scoliosis with blood WBC and subpopulations.
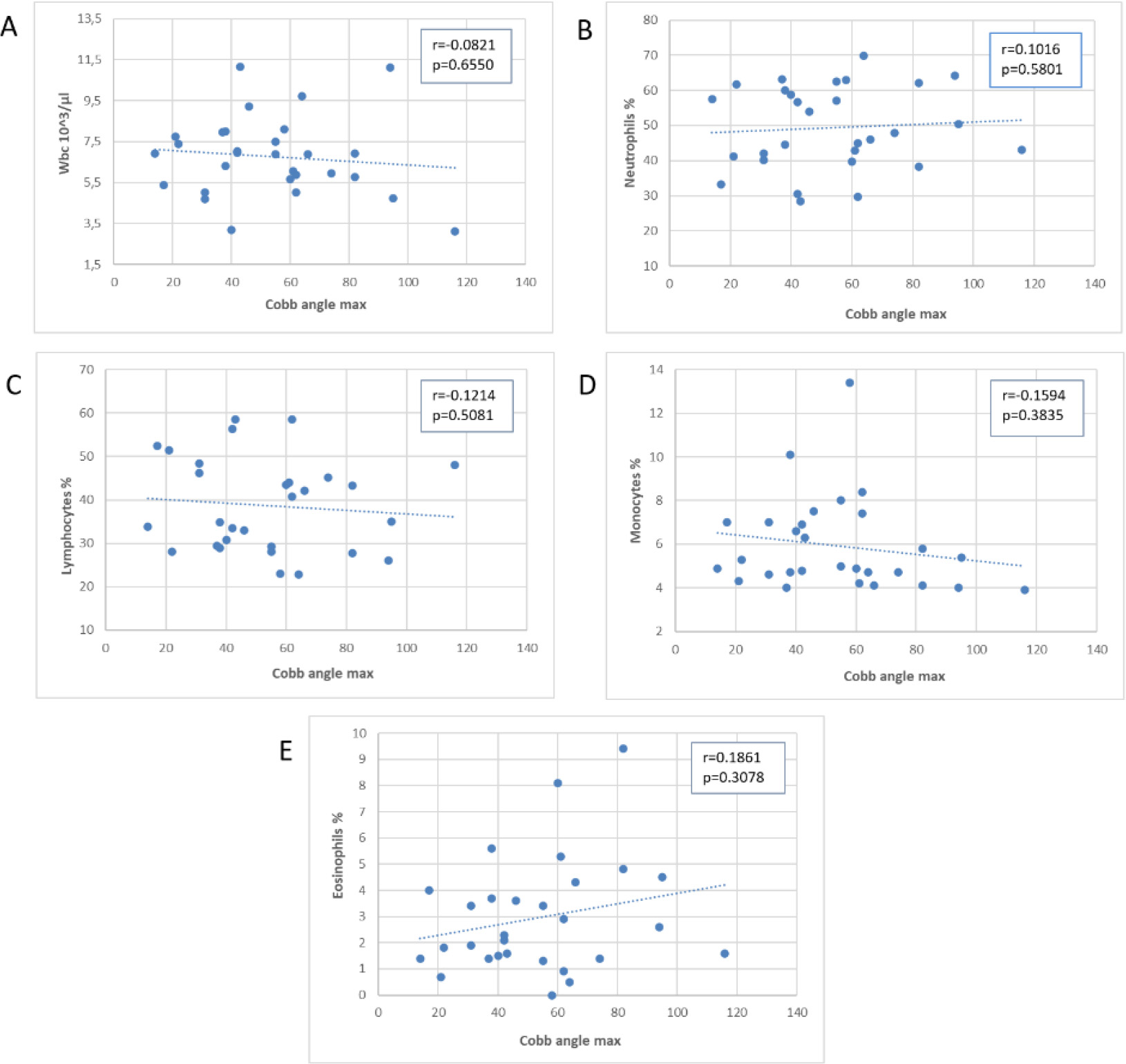
Figure 3.
Scatter plot of the association of the Cobb’s angle with plasma proteins.
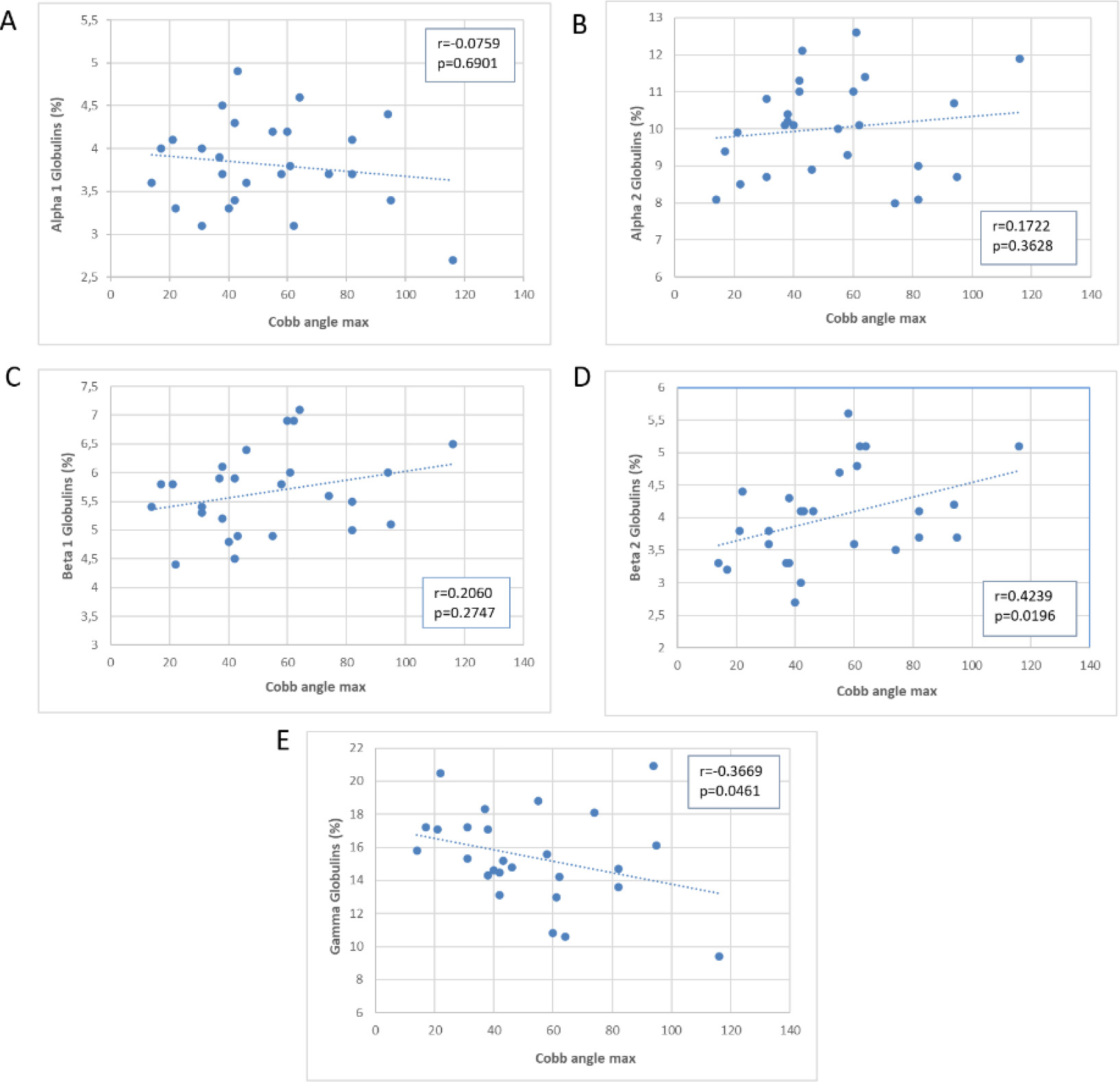
Figure 4.
Scatter plot of the association of Cobb’s angle with immunoglobulins. Values on the y axis are expressed in g/L.
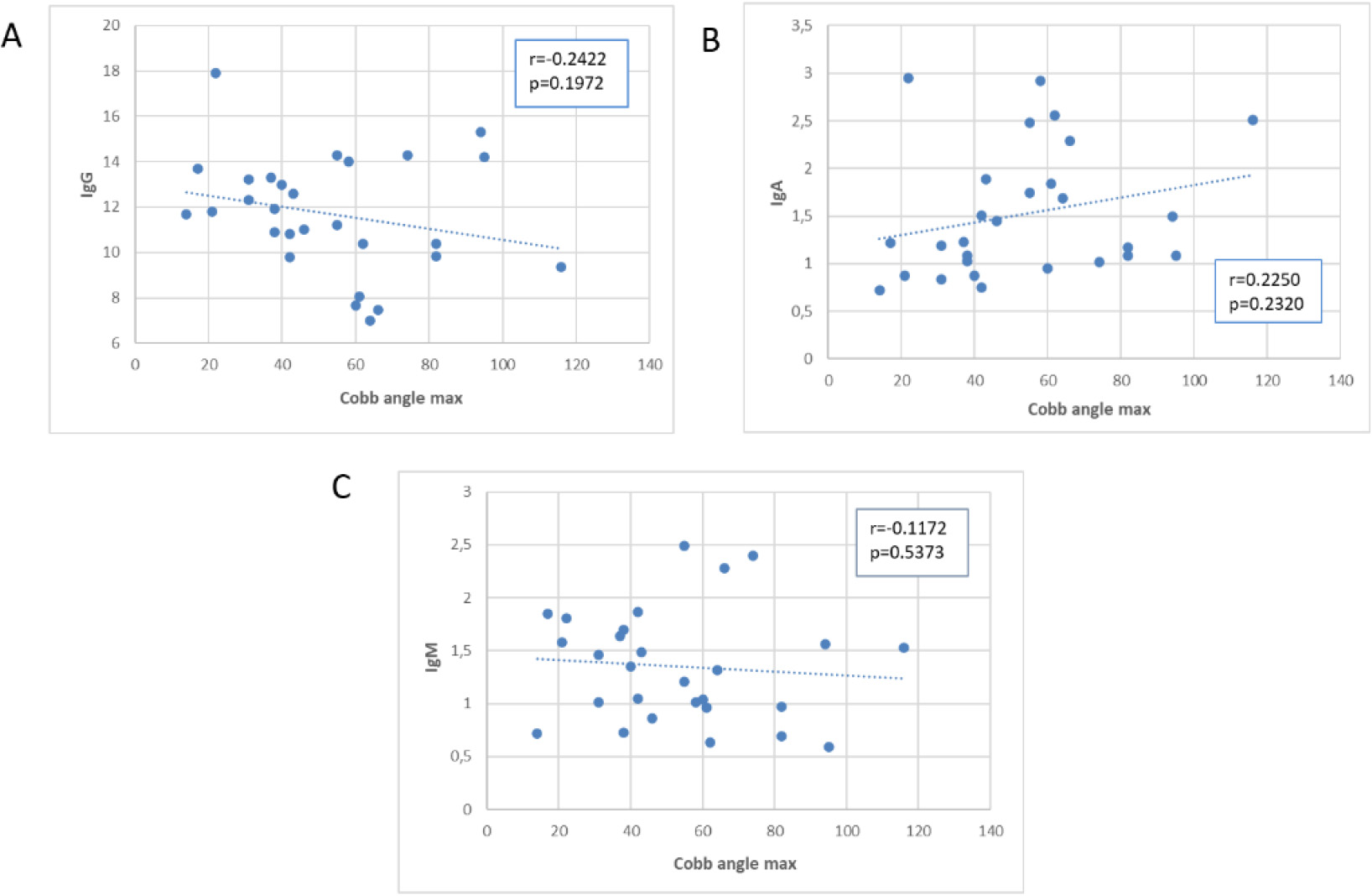
Next, we examined the correlation between Cobb’s angle and the laboratory test values, as shown in scatter plots in Figs 2–4, depicting the correlation with leukocytes (Fig. 2A–E), plasma proteins (Fig. 3A–E), and immunoglobulins (Fig. 4A–C). Pearson’s correlation coefficient (r) was used to calculate correlation values, and statistical significance of the correlation is represented by the
Figure 2 displays the relationship between Cobb’s angle (x-axis) and different white blood cell subpopulations (y-axis). We did not observe a significant correlation between Cobb’s angle and the absolute values of WBC, neutrophils %, lymphocytes %, or monocytes %. However, there was a non-statistically significant increase in eosinophil percentage values, partially correlated with an increase in Cobb’s angle, although removing outliers weakened this correlation (data not shown).
Figure 3 shows a significant correlation between Cobb’s angle and the percentage values of beta 2 globulins (directly proportional,
Concerning immunoglobulins (Fig. 4), we observed a negative correlation with IgGs and a positive correlation with IgAs in absolute values, although statistical significance was not reached (
Figure 5.
Scatter plots of the association of the Scoliosis Score with blood WBC and subpopulations.
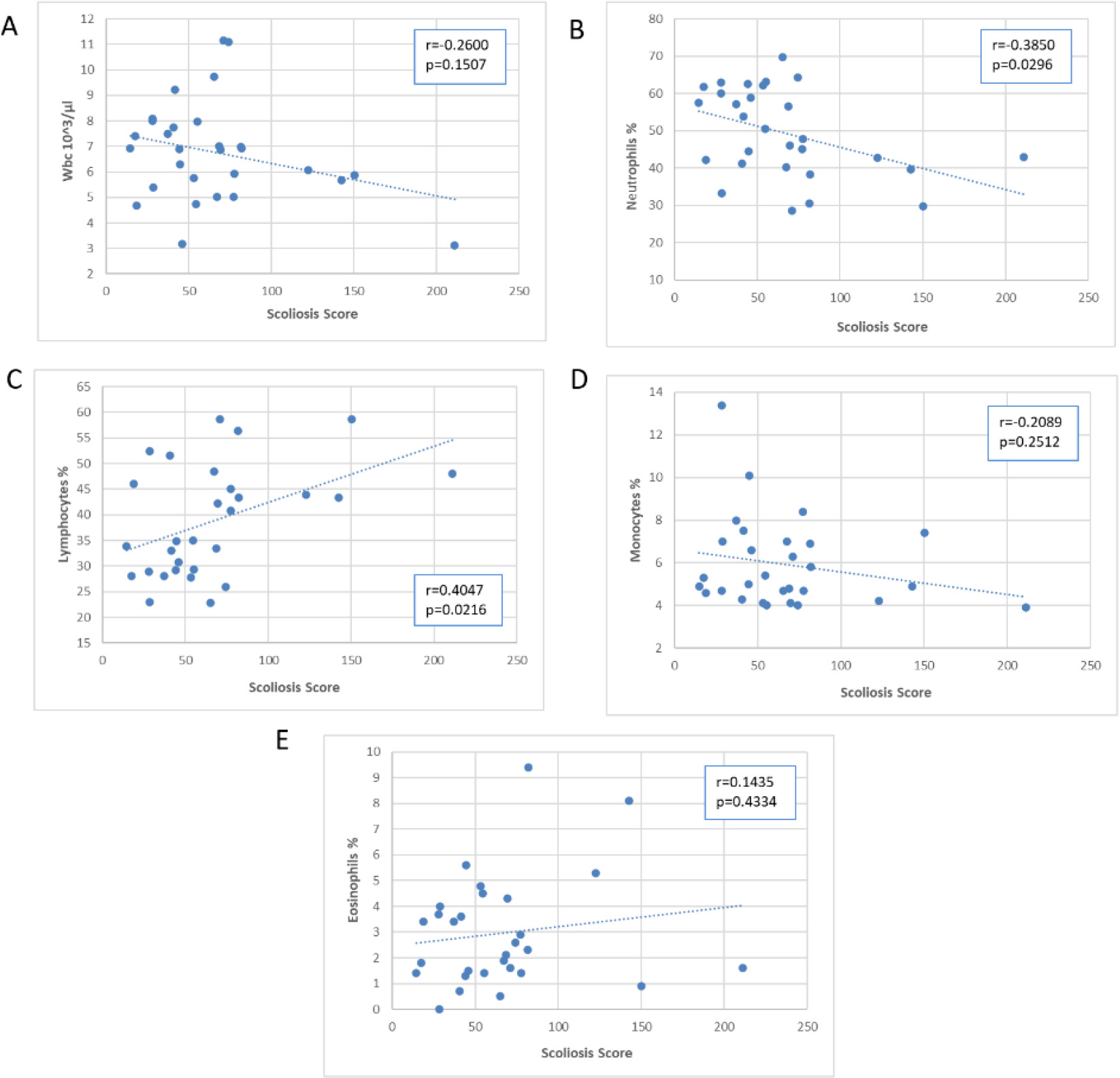
Figure 6.
Scatter plot of the association of Scoliosis Score with plasma proteins.
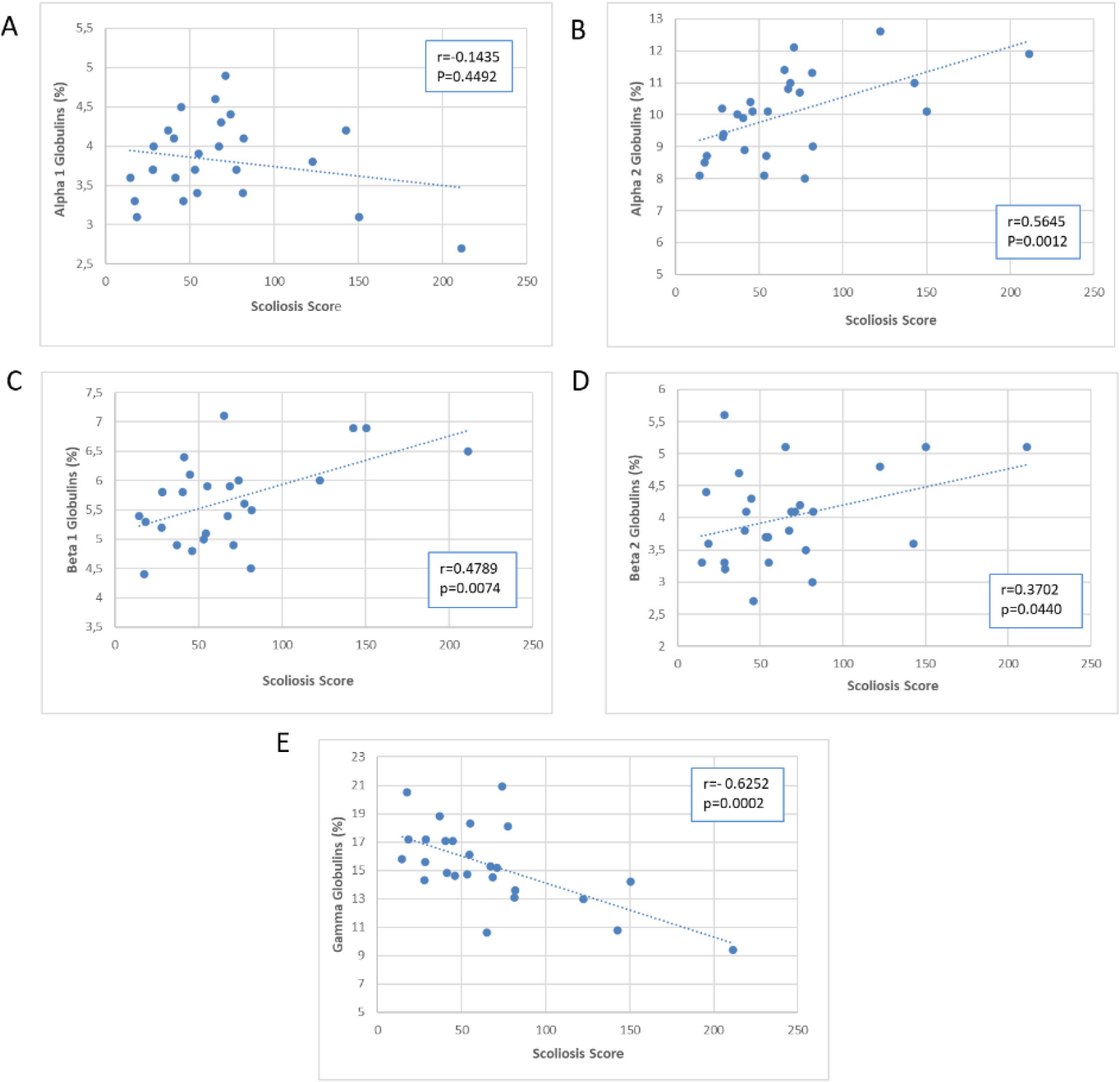
Figures 5–7 presented scatter plots illustrating the correlation between the Scoliosis Score and leukocytes (Fig. 5A–E), plasma proteins (Fig. 6A–E), and immunoglobulins (Fig. 7A–C). Using the Scoliosis Score resulted in a stronger correlation. We found a robust, inverse correlation between the score and the percentage of neutrophils (
The use of the Scoliosis Score confirmed the correlation with beta 2 globulins observed with the Cobb’s angle. Furthermore, there was a strong correlation between the Scoliosis Score and alpha 2 globulins (
A correlation between IgG (absolute number) and Scoliosis Score (
Table 2 displays the regression analyses performed for each scoliosis severity index, with age corrections applied. The results from regression analysis were consistent with those from correlation analysis, with the Scoliosis Score yielding a greater number of significant results.
4.Discussion
Our data suggests a potential correlation between blood protein composition and the severity of AIS, as quantified by Cobb’s angle. Cobb’s angle is a widely accepted metric for spinal curvature, providing an objective measure of deformity. In our study, we explored the relationship between Cobb’s angle and various laboratory parameters, including WBC subgroups, plasma proteins, and immunoglobulin types.
Our findings show a significant correlation between Cobb’s angle and the percentage values of beta-2 globulins and gamma-globulins. The correlation between beta-2 globulins and Cobb’s angle suggests that scoliosis severity may be associated to higher levels of inflammatory markers in scoliosis patients. This aligns with the findings of Wang et al. [7], who found a correlation between scoliosis grade and plasma fibrinogen, a type of beta-2 globulin. Conversely, the negative correlation between gamma-globulins and Cobb’s angle suggests that more severe spinal curvature may be linked to decreased production of immunoglobulins. Around 10% of severe scoliosis cases showed significant hypogammaglobulinemia. Additionally, some severe scoliosis cases had high concentrations of alpha-2 globulins. Since alpha-2 and gamma globulins have an inverse correlation, subjects with elevated alpha-2 concentrations also experienced hypogammaglobulinemia compared to the rest of the group. This finding supports the idea that dysregulated immune function may play a role in scoliosis.
However, the exact causal relationship between scoliosis and these immunological changes remains unclear. AIS is a complex condition in which multiple biochemical and molecular mechanisms may play a role [14]. A case-control study found a link between a genetic polymorphism of IL-6 and the severity of idiopathic scoliosis, indicating a possible genetic susceptibility to inflammatory mechanisms [15]. Indeed, existing literature suggests associations between scoliosis and infections, autoimmune diseases, and rare inflammatory conditions [16, 17], such as rheumatoid arthritis (RA) [18]. Particularly, the prevalence of scoliosis in RA exceeds that in the general population (16–32%) [19], with its incidence correlated with RA disease activity and corticosteroid use [20]. Genetic susceptibility to RA is associated with inflammation and immunity-related genes, so it’s plausible that inflammation underlies both conditions. However, the correlation between scoliosis and corticosteroid use is not straightforward. It may indicate increased inflammation in high-activity RA patients, but it can also reduce bone density and increase the risk of scoliosis. Indeed, the link between inflammation and AIS may involve alterations in bone calcification and density. AIS patients have been found to have altered bone mineral status [21, 22, 23]. The presence of RANKL, an important pro-inflammatory modulator [24], has been found to be increased in the serum of idiopathic scoliosis patients, leading to loss of bone density [25, 26]. Decreased bone density is a common factor in RA and scoliosis comorbidity [27], and RA’s chronic inflammation is a risk factor for osteoporosis [28]. Therefore, altered bone mineral status in AIS may help explain the connection between inflammation and spinal curvature. Additionally, beta2-microglobulin, a key beta-2 globulin, has been identified as a biological marker of bone remodeling, stimulating osteoclastic and osteoblastic activity [29, 30, 31]. The role of immunoglobulins warrants further exploration. It’s conceivable that immunocompromised patients (with lower immunoglobulin levels) may be more susceptible to infections, including viral ones, leading to increased inflammation and rapid bone damage. However, additional research is needed to substantiate this hypothesis.
Our assessment of scoliosis severity was improved by introducing a new index that considers multiple parameters, including the Risser index. Since the progression of curvature in AIS heavily depends on skeletal age [32], the correlation between this index and certain laboratory values related to the immune and inflammatory systems highlights its potential significance in case collections and clinical practice. There is a correlation between the two scoliosis indexes, although it is not perfect. In extreme cases, the correlation may be weak. This can be explained by the non-uniform distribution of ages in our sample. The Scoliosis Score takes into account the Risser index, which assigns different weights to degrees of curvature based on skeletal development. Therefore, a patient in the early stages of skeletal development may be classified as more severe than one whose development is already complete, even if they have the same degree of curvature. This shows that clinical severity is influenced not only by scoliosis itself but also by individual variations in developmental stages [11, 33]. This approach could provide a more precise understanding of clinical severity and age-related variations in scoliosis patients.
Future research in scoliosis may lead to the discovery that specific protein species are responsible for the observed increase in globulins. For instance, alpha-2-macroglobulin, representative of alpha-2 globulins in serum, decreases during Deer antler treatment, which promotes bone and cartilage development [34]. It remains an underexplored area to identify proteins as indicators of AIS severity and progression risk [35]. Notably, calmodulin, a regulator of skeletal muscle and platelet systems, holds promise in predicting severity and therapeutic response. Platelet calmodulin levels are abnormal in scoliosis and higher in patients with progressive curves [36, 37]; in addition, it showed potential as an independent predictor of severity and therapy response [38]. Beta-2 microglobulin, linked to inflammatory processes [39, 40], could be valuable in evaluating disease activity. Furthermore, exploring the potential benefits of dietary flavonoids with osteoprotective and mild anti-inflammatory properties for this population is also worth considering [41, 42, 43].
Table 2
Regression analysis for each scoliosis index
Cobb’s angle | Age (per Cobb’s angle) | Scol. Score | Age (per Scol. Score) | |||||||||
---|---|---|---|---|---|---|---|---|---|---|---|---|
Coeff | st.err |
| Coeff | st.err |
| Coeff | st.err |
| Coeff | st.err |
| |
alpha1 | 0.096 | 0.738 | 0.021 | 0.093 | 0.825 | 0.107 | 0.490 | 0.107 | 0.907 | |||
alpha2 | 0.113 | 0.234 | 0.633 | 0.504 | 0.228 | 0.036 | 0.614 | 0.238 | 0.015 | 0.238 | 0.413 | |
beta1 | 0.131 | 0.133 | 0.331 | 0.129 | 0.712 | 0.394 | 0.132 | 0.006 | 0.136 | 0.132 | 0.310 | |
beta2 | 0.308 | 0.117 | 0.014 | 0.112 | 0.114 | 0.336 | 0.374 | 0,129 | 0.007 | 0.248 | 0.129 | 0.066 |
gamma | 0.494 | 0.083 | 0.553 | 0.480 | 0.260 | 0.476 | 0.001 | 0.476 | 0.637 | |||
IgG | 0.447 | 0.261 | 0.637 | 0.472 | 0.188 | 0.456 | 0,014 | 0.040 | 0.498 | 0.937 | ||
IgA | 0.168 | 0.119 | 0.171 | 0.169 | 0.126 | 0.191 | 0.291 | 0.126 | 0.029 | 0.307 | 0.137 | 0.034 |
IgM | 0.103 | 0.579 | 0.038 | 0.109 | 0.727 | 0.115 | 0.602 | 0.013 | 0.125 | 0.921 | ||
WBC | 0.346 | 0.790 | 0.279 | 0.343 | 0.422 | 0.386 | 0.262 | 0.065 | 0.387 | 0.869 | ||
neutro | 2,313 | 1.875 | 0.227 | 5.511 | 1.856 | 0.006 | 2.106 | 0.294 | 3.814 | 2.153 | 0.087 | |
lympho | 1.627 | 0.137 | 1.611 | 0.001 | 1.879 | 1.891 | 0.329 | 1.898 | 0.031 | |||
mono (log) | 0.052 | 0.371 | 0.065 | 0.052 | 0.220 | 0.060 | 0.587 | 0.057 | 0.060 | 0.350 | ||
eos (log) | 0.113 | 0.131 | 0.396 | 0.147 | 0.136 | 0.290 | 0.074 | 0.149 | 0.622 | 0.159 | 0.156 | 0.314 |
Figure 7.
Scatter plot of the association of the Scoliosis Score with immunoglobulins.
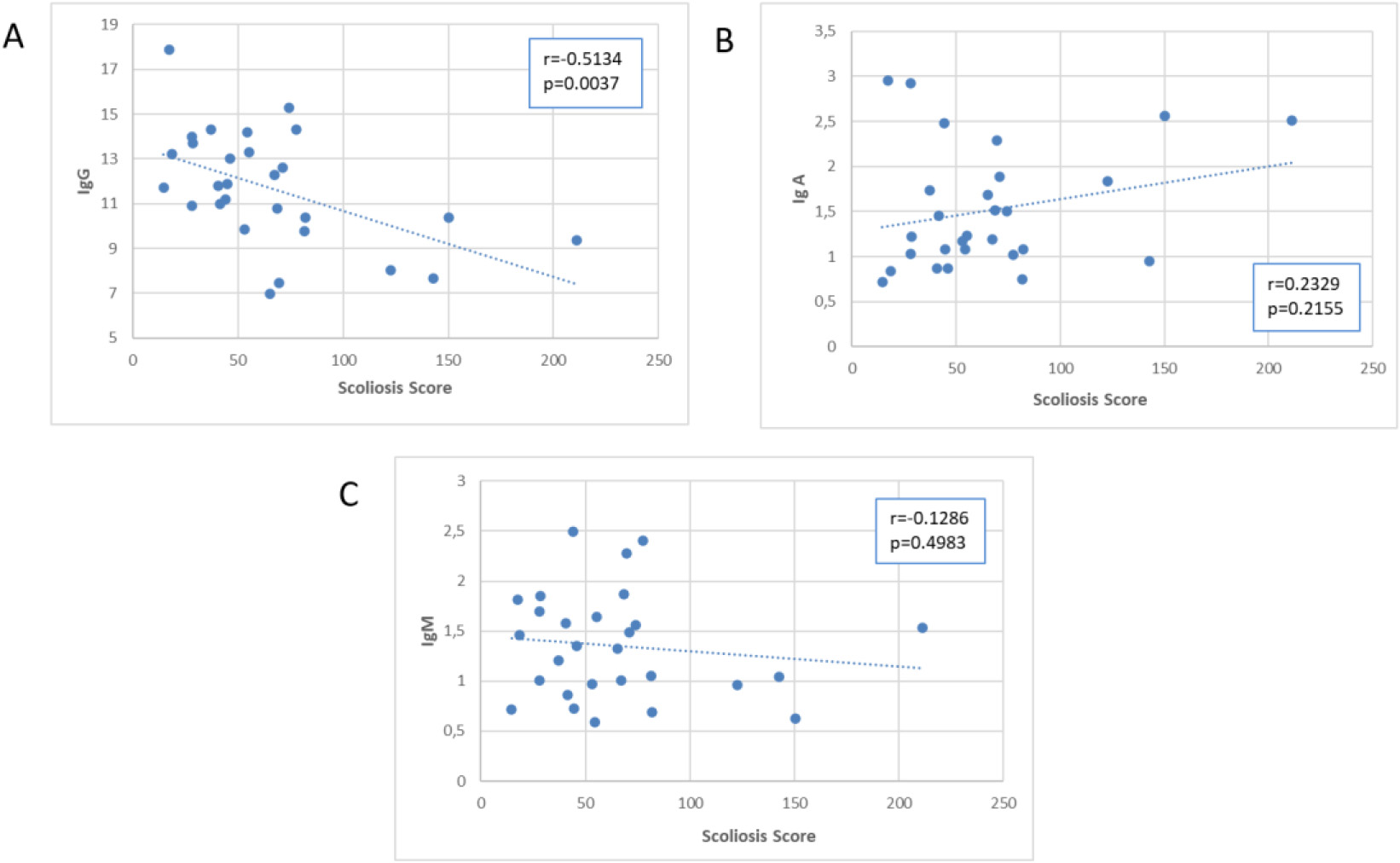
Our study has certain limitations. Our results are preliminary and therefore should be interpreted with caution. Our sample size is relatively small, with only 4 male participants, which may introduce a potential confounding variable. This, combined with the low mean and median age of our sample, raises considerations about the generalizability of our findings, although we added a partial analysis excluding the male population. To gain a comprehensive understanding, further research with larger and more diverse populations is necessary. In addition, our proposed Scoliosis Score also requires validation through additional studies.
5.Conclusion
In conclusion, our pilot study supports the idea that scoliosis involves systemic inflammation and immune system dysregulation in some patients. It highlights differences between Cobb’s angle and the Scoliosis Score index, emphasizing the need for considering various clinical factors in the scoliosis population. The intricate relationship between blood proteins and scoliosis severity suggests potential for novel therapies. Further research is crucial to validate and explore these insights.
Funding
The authors report no funding.
Ethics statement
This research did not involve any procedures or interventions that deviated from the standard clinical routine analysis. Patients provided consent for data processing, with parental consent obtained for minors, ensuring anonymity. The study’s principal investigator and research team ensured participant confidentiality. Sensitive data of recruited subjects were processed in accordance with EU Regulation 2016/679 (GDPR) and Legislative Decree 30/6/2003, n. 196 (Code regarding the protection of personal data). Only researchers directly involved in the analysis had access to electronically stored data on an encrypted access computer throughout all study phases. The study adhered to the principles of the 1964 Declaration of Helsinki and its subsequent amendments.
Acknowledgments
The authors would like to thank the staff of the Apostolo Foundation for their support during this research.
Conflict of interest
The authors declare that they have no conflict of interest.
References
[1] | Negrini S, de Mauroy JC, Grivas TB, Knott P, Kotwicki T, Maruyama T, et al. Actual evidence in the medical approach to adolescents with idiopathic scoliosis. Eur J Phys Rehabil Med. (2014) ; 50: : 87-92. |
[2] | Peng Y, Wang SR, Qiu GX, Zhang JG, Zhuang QY. Research progress on the etiology and pathogenesis of adolescent idiopathic scoliosis. Chin Med J (Engl). (2020) ; 133: : 483-493. |
[3] | Samaan MC, Missiuna P, Peterson D, Thabane L. Understanding the role of the immune system in adolescent idiopathic scoliosis: Immunometabolic CONnections to Scoliosis (ICONS) study protocol. BMJ Open. (2016) ; 6: : e011812. |
[4] | Rudrapatna S, Peterson D, Missiuna P, Aditya I, Drew B, Sahar N, et al. Understanding muscle-immune interactions in adolescent idiopathic scoliosis: a feasibility study. Pilot Feasibility Stud. (2017) ; 3: : 50. |
[5] | Lee AS, Ellman MB, Yan D, Kroin JS, Cole BJ, van Wijnen AJ, et al. A current review of molecular mechanisms regarding osteoarthritis and pain. Gene. (2013) ; 527: : 440-447. |
[6] | Johnson ZI, Schoepflin ZR, Choi H, Shapiro IM, Risbud MV. Disc in flames: Roles of TNF-alpha and IL-1beta in intervertebral disc degeneration. Eur Cell Mater. (2015) ; 30: : 104-116. |
[7] | Wang Q, Wang C, Liu J, Sun J, Wang C, Zhang X. Plasma proteomics analysis of adolescent idiopathic scoliosis patients revealed by Quadrupole-Orbitrap mass spectrometry. Proteomics Clin Appl. (2021) ; 15: : e2100002. |
[8] | Zheng D, Li J, Li J, Zhang Y, Yu M. Special pathologic features of adolescent idiopathic scoliosis: Could there be a new type of muscular dystrophinopathy? Research Square. (2020) ; 2020. |
[9] | Cobb J. Outline for the study of scoliosis. Instructional Course Lectures. (1948) ; 5: : 261-275. |
[10] | Horng MH, Kuok CP, Fu MJ, Lin CJ, Sun YN. Cobb angle measurement of spine from x-ray images using convolutional neural network. Comput Math Methods Med. (2019) ; 2019: : 6357171. |
[11] | Poncet P, Dansereau J, Labelle H. Geometric torsion in idiopathic scoliosis: three-dimensional analysis and proposal for a new classification. Spine (Phila Pa 1976). (2001) ; 26: : 2235-43. |
[12] | Risser JC. The classic: The iliac apophysis: an invaluable sign in the management of scoliosis. 1958. Clin Orthop Relat Res. (2010) ; 468: : 643-53. |
[13] | Colton T. Statistics in Medicine. (1974) . Little, Brown and Company, Boston (Mass). |
[14] | Newton Ede MM, Jones SW. Adolescent idiopathic scoliosis: evidence for intrinsic factors driving aetiology and progression. Int Orthop. (2016) ; 40: : 2075-2080. |
[15] | Nikolova ST, Yablanski VT, Vlaev EN, Stokov LD, Savov AS, Kremensky IM, et al. Association between IL-6 and MMP3 common genetic polymorphisms and idiopathic scoliosis in bulgarian patients: A case-control study. Spine (Phila Pa 1976). (2016) ; 41: : 785-791. |
[16] | D’Amico S, Pavone P, Testa G, Greco F, Marino L, Smilari P, et al. Secondary scoliosis as a complication of acute transverse myelitis in a child. J Funct Morphol Kinesiol. (2020) ; 5. |
[17] | Li Z, Shen J, Liang J, Feng F. Successful surgical treatment of scoliosis secondary to Guillain-Barre syndrome: Case report. Medicine (Baltimore). (2016) ; 95: : e3775. |
[18] | Mochizuki T, Yano K, Ikari K, Hiroshima R, Takaoka H, Kawakami K, et al. Scoliosis-related factors in patients with rheumatoid arthritis: A cross-sectional study. Mod Rheumatol. (2016) ; 26: : 352-7. |
[19] | Makino T, Kaito T, Fujiwara H, Yonenobu K. Lumbar scoliosis in rheumatoid arthritis: epidemiological research with a DXA cohort. Spine (Phila Pa 1976). (2013) ; 38: : E339-43. |
[20] | Yamada K, Suzuki A, Takahashi S, Inui K, Koike T, Okano T, et al. Incidence of and risk factors for spondylolisthesis, scoliosis, and vertebral fracture in rheumatoid arthritis. J Bone Miner Metab. (2022) ; 40: : 120-131. |
[21] | Li XF, Li H, Liu ZD, Dai LY. Low bone mineral status in adolescent idiopathic scoliosis. Eur Spine J. (2008) ; 17: : 1431-40. |
[22] | Lam TP, Hung VW, Yeung HY, Tse YK, Chu WC, Ng BK, et al. Abnormal bone quality in adolescent idiopathic scoliosis: a case-control study on 635 subjects and 269 normal controls with bone densitometry and quantitative ultrasound. Spine (Phila Pa 1976). (2011) ; 36: : 1211-7. |
[23] | Pourabbas Tahvildari B, Erfani MA, Nouraei H, Sadeghian M. Evaluation of bone mineral status in adolescent idiopathic scoliosis. Clin Orthop Surg. (2014) ; 6: : 180-4. |
[24] | Papadaki M, Rinotas V, Violitzi F, Thireou T, Panayotou G, Samiotaki M, et al. New Insights for RANKL as a Proinflammatory Modulator in Modeled Inflammatory Arthritis. Front Immunol. (2019) ; 10: : 97. |
[25] | Zhou S, Wang W, Zhu Z, Sun X, Zhu F, Yu Y, et al. Increased expression of receptor activator of nuclear factor-kappaB ligand in osteoblasts from adolescent idiopathic scoliosis patients with low bone mineral density. J Huazhong Univ Sci Technolog Med Sci. (2012) ; 32: : 686-690. |
[26] | Suh KT, Lee SS, Hwang SH, Kim SJ, Lee JS. Elevated soluble receptor activator of nuclear factor-kappaB ligand and reduced bone mineral density in patients with adolescent idiopathic scoliosis. Eur Spine J. (2007) ; 16: : 1563-1569. |
[27] | Ohishi M, Miyahara H, Kondo M, Nakashima Y, Terada K, Esaki Y, et al. Characteristics of lumbar scoliosis in patients with rheumatoid arthritis. J Orthop Surg Res. (2014) ; 9: : 30. |
[28] | Baker R, Narla R, Baker JF, Wysham KD. Risk factors for osteoporosis and fractures in rheumatoid arthritis. Best Pract Res Clin Rheumatol. (2022) ; 36: : 101773. |
[29] | Ripoll E, Arribas I, Relea P, Varela L, Villa LF, Revilla M, et al. Beta-2-microglobulin in diseases with high bone remodeling. Calcif Tissue Int. (1995) ; 57: : 272-6. |
[30] | Balint E, Marshall CF, Sprague SM. Role of interleukin-6 in beta2-microglobulin-induced bone mineral dissolution. Kidney Int. (2000) ; 57: : 1599-607. |
[31] | Menaa C, Esser E, Sprague SM. Beta2-microglobulin stimulates osteoclast formation. Kidney Int. (2008) ; 73: : 1275-81. |
[32] | Winiarski A, Zarzycki D, Koniarski A, Kalicinski M. The natural history of idiopathic scoliosis. Ortop Traumatol Rehabil. (2005) ; 7: : 1-7. |
[33] | Asher MA, Burton DC. A concept of idiopathic scoliosis deformities as imperfect torsion(s). Clin Orthop Relat Res. (1999) . doi: 10.1097/00003086-199907000-0000311-25. |
[34] | Yao B, Gao H, Liu J, Zhang M, Leng X, Zhao D. Identification of potential therapeutic targets of deer antler extract on bone regulation based on serum proteomic analysis. Mol Biol Rep. (2019) ; 46: : 4861-4872. |
[35] | Kuznia AL, Hernandez AK, Lee LU. adolescent idiopathic scoliosis: Common questions and answers. Am Fam Physician. (2020) ; 101: : 19-23. |
[36] | Kindsfater K, Lowe T, Lawellin D, Weinstein D, Akmakjian J. Levels of platelet calmodulin for the prediction of progression and severity of adolescent idiopathic scoliosis. J Bone Joint Surg Am. (1994) ; 76: : 1186-92. |
[37] | Acaroglu E, Akel I, Alanay A, Yazici M, Marcucio R. Comparison of the melatonin and calmodulin in paravertebral muscle and platelets of patients with or without adolescent idiopathic scoliosis. Spine (Phila Pa 1976). (2009) ; 34: : E659-63. |
[38] | Lowe T, Lawellin D, Smith D, Price C, Haher T, Merola A, et al. Platelet calmodulin levels in adolescent idiopathic scoliosis: do the levels correlate with curve progression and severity? Spine (Phila Pa 1976). (2002) ; 27: : 768-75. |
[39] | Crisp AJ, Coughlan RJ, Mackintosh D, Clark B, Panayi GS. beta 2 microglobulin plasma levels reflect disease activity in rheumatoid arthritis. J Rheumatol. (1983) ; 10: : 954-6. |
[40] | Zychowska I, Suszek D, Dryglewska M, Majdan M. beta2-microglobulin as a marker of systemic lupus erythematosus activity. Adv Clin Exp Med. (2018) ; 27: : 379-382. |
[41] | Martiniakova M, Babikova M, Mondockova V, Blahova J, Kovacova V, Omelka R. The Role of Macronutrients, Micronutrients and Flavonoid Polyphenols in the Prevention and Treatment of Osteoporosis. Nutrients. (2022) ; 14. |
[42] | Gao G, Ding H, Zhuang C, Fan W. Effects of hesperidin on H2O2-treated chondrocytes and cartilage in a rat osteoarthritis model. Med Sci Monit. (2018) ; 24: : 9177-9186. |
[43] | Goyal A, Agrawal N. Quercetin: A Potential Candidate For The Treatment Of Arthritis. Curr Mol Med. (2021) . CMM-EPUB-114889 [pii]; doi: 10.2174/1566524021666210315125330. |