Relationship Between Excessive Daytime Sleepiness and Caudate Nucleus Volume in Patients with Subjective Cognitive Decline: A Study from the SILCODE Using the Volbrain
Abstract
Background:
Excessive daytime sleepiness (EDS) and caudate nucleus volume alterations have been linked to Alzheimer’s disease (AD), but their relationship remains unclear under the context of subjective cognitive decline (SCD).
Objective:
This study aimed to investigate the relationship between EDS and caudate nucleus volume in patients with SCD.
Methods:
The volume of entire brain was measured in 170 patients with SCD, including 37 patients with EDS and 133 non-EDS, from the Sino Longitudinal Study on Cognitive Decline (SILCODE). Participants underwent a comprehensive assessment battery, including neuropsychological and clinical evaluations, blood tests, genetic analysis for APOE ɛ4, and structural MRI scans analyzed using the fully automated segmentation tool, volBrain.
Results:
Patients with EDS had significantly increased volume in the total and left caudate nucleus compared to non-EDS. The most significant cognitive behavioral factor associated with caudate nucleus volume in the EDS was the Auditory Verbal Learning Test-recognition.
Conclusions:
These findings suggest that EDS may be associated with alterations in caudate nucleus volume, particularly in the left hemisphere, in the context of SCD. Further research is necessary to understand the underlying mechanisms of this relationship and its implications for clinical management.
INTRODUCTION
Excessive daytime sleepiness (EDS) is a common symptom of sleep disorders, which may be an early marker and potentially a reversible risk factor of cognitive decline and onset of dementia, making it important to consider even during periods of unimpaired cognitive performance [1, 2]. Subjective cognitive decline (SCD) is a self-reported decline in cognitive function without evidence of objective cognitive impairment [3]. Individuals with SCD are biologically characterized by a higher risk of exhibiting a biomarker pattern that points to Alzheimer’s disease (AD), indicating that individuals with SCD may be more susceptible to progression to mild cognitive impairment (MCI) or AD [4, 5]. Furthermore, research have found that poor sleep quality was closely related to SCD [6–11]. However, limited literature reports on EDS symptoms in patients with SCD.
The caudate nucleus is a C-shaped structure located in the center of the cerebral hemispheres [12], playing a crucial role in regulating behavior by controlling functions such as memory and motivation [13]. This is because abnormal functioning of the caudate nucleus leads to reduced dopamine transporter uptake, which is associated with clinical EDS symptoms [14]. Research have reported that the caudate nucleus volume is larger in SCD patients than in healthy individuals [15]. Furthermore, greater caudate volume in elderly women with AD compared to non-dementia patients [16], and in addition, other studies have pointed to a reduction in caudate volume in AD patients [17] and an association with MCI to AD conversion [18]. An increasing body of evidence suggests that the sleep duration may be associated with the caudate nucleus volume [19, 20]. Therefore, the EDS symptoms combined with caudate nucleus volume changes could be high-risk factors for disease progression.
Structural magnetic resonance imaging (sMRI) is a widely accessible, non-invasive, and commonly used clinical method [21] that can track subcortical changes provoking disease progression [22]. A quantitative volumetric analysis of the caudate nucleus using sMRI can provide valuable information in early diagnosis and prognosis of patients with AD [17]. Furthermore, sMRI can be employed as an independent biomarker of neurodegeneration to understand relationships between cognition and neurodegeneration in AD, and atrophy measured on sMRI is a powerful biomarker for the stage and intensity of neurodegeneration in AD pathology [23].
For reasons above, our study aimed to investigate the characteristics of the caudate nucleus volume between the control and EDS groups in SCD patients, and whether behavioral and cognitive functions influenced the caudate nucleus volume, which may help improve the sensitivity of structural imaging studies to provide a separate morphologic index of SCD with sleep disorders risk factors. We used the fully automated segmentation method volBrain (http://volbrain upv.es) for volumetric analysis, which is the technique of choice for segmenting the caudate nucleus [24]. Respondents were measured on the Epworth Sleepiness Scale (ESS), with a score of ESS≥10 indicating EDS. We hypothesize that there was a specially changed caudate nucleus volume in patients with SCD with EDS compared with control subjects. We also estimated that subtle behavioral and cognitive functions would be associated with caudate nucleus volume alterations in SCD with EDS.
METHODS
Participants
We recruited 170 participants (EDS = 37 and NC = 133) from the Sino Longitudinal Study on Cognitive Decline (SILCODE) at the Xuanwu Hospital of Capital Medical University in China [25]. All participants from the community, and memory clinics and local clinicians referral recruiting mandarin-speaking right-handed and older Han Chinese living in Beijing for a long time [26], who were accepted a comprehensive set of assessments, including a standardized clinical physical examination and assessment, medical history review, detailed neuropsychological evaluations, and undergone blood tests and structural MRI scans [27]. The average age, education level, number of males and females in NC group were 66.22 years, 12.00 years, 52/81, respectively, and those in EDS group were 65.84 years, 12.14 years, 17/20, respectively. The definition criteria of SCD were as following [3]: 1) In the past five years, there has been a continuous decline in memory rather than other cognitive domains. 2) Concerns about memory decline have been expressed through structured interviews with doctors, and there has been a feeling of poor performance compared to the same age group. 3) Memory decline has been confirmed by another informed person. 4) Standardized neuropsychological tests within age, gender, and educational adaptation norms are met, which do not meet MCI or dementia criteria [28]. The research was registered on ClinicalTrials.gov (Identifier: NCT03370744). The Medical Ethics Committee of Xuanwu Hospital approved our study protocol [27], and all participants shall be completed before the study program written informed consent.
Exclusion criteria included respondents who could not comply with the study plan or were unwilling to undergo MRI, respondents with severe mental illness, subjects with other brain disorders that may cause cognitive impairment, participants with intellectual disabilities, and participants with systemic disorders [26, 29].
Behavioral and cognitive assessment
The staff was trained according to standard guidelines before participating in neuropsychological testing, which encompassed different domains, including cognitive function, sleep quality, and behavioral symptoms.
The cognitive domain assessment included the auditory verbal learning test-Huashan version (AVLT-H) for memory function, semantic verbal fluency of animals (AFT) and Boston naming test (BNT) for language function, and shape testing sections A and B (STT-A & B) for executive function evaluation. Self-perceived cognitive function was assessed using a subjective cognitive decline questionnaire-item 9 (SCD-Q9). The mini-mental state examination (MMSE), the Montreal cognitive assessment-basic (MoCA-B), and Memory and executive screening (MES) were conducted for overall cognitive function assessment. For sleep quality evaluation, the Pittsburgh sleep quality index (PSQI), rapid-eye-movement sleep behavior disorder screening questionnaire (RBDSQ), and Epworth sleepiness scale (ESS) were used. The Hamilton depression scale (HAMD), the Hamilton anxiety scale (HAMA) and the Geriatric depression scale (GDS) were used for the assessment of psych behavioral symptoms [27, 30, 31].
EDS characterization
The ESS is a self-administered questionnaire that consists of eight questions asking to rate how likely it is to fall asleep in everyday situations. Responses are rated on a range from 0 to 3, with 0 representing no likelihood of nodding off, 1 meaning a very slight probability of nodding off, 2 representing a medium likelihood of nodding off, and 3 meaning dozing [32, 33]. A higher score indicates that the respondent is more likely to snooze [34], with a score of ≥10 indicating EDS.
APOE genotyping
Clinical laboratory staff followed standard procedures when doing apolipoprotein E (APOE) genotyping work. Two single nucleotide polymorphisms (SNPs), rs7412 and rs429358, were analyzed to determine the apolipoprotein ɛ2/ɛ3/ɛ4 haplotype [26]. Standard Sanger sequencing methods were used to genotype lipoproteins using the following primers: 5′-ACGCGGGCACGGCTGTCCAAGG-3′ (forward) and 5′-GGCGCTCGCGGATGGCGCTGA-3′ (reverse) [27]. The amplification of APOE conditions were as following: 1 cycle at 98°C for 10 s, 35 cycles at 72°C for 5 s, 1 cycle at 72°C for 5 min.
MRI acquisition
Before the actual entire brain imaging scan, the participants may undergo a simulation session to familiarize themselves with the scanning process to minimize any potential bias that may arise due to anxiety or discomfort during the actual scan. The actual scan lasted approximately one hour, and T1-weighted structural images of entire brain were acquired using SPGR sequences. Respondents were scanned on an integrated simultaneous 3.0T TOF PET/MR (Signa PET/MR, GE Healthcare, WI, USA) [27]. The parameters of 3D BRAVO T1-weighted sagittal images were as following: repetition time/echo time (TR/TE) = 6.9 ms/2.98 ms, flip angle = 12°, inversion time (TI) = 450 ms, the field of view (FOV)=256×256 mm 2, matrix = 256×256, slices = 192, slice thickness = 1 mm and voxel size = 1×1×1 mm3.
Imaging analysis
VolBrain is a fully automated segmentation tool that is commonly used for segmenting caudate nuclei due to its high level of anatomical precision and minimal labor requirements. The method employs a multi-tuple patch-based label fusion segmentation technique to accurately segment anatomical structures [24, 35, 36]. The process of obtaining the required data involves several steps. First, the patient’s images were exported from the local image archive. Next, the scanned images in DICOM format were converted to NIFTI format using MRIcron software. After that, an account was created and registered on the volBrain website, where individual scanned images were uploaded in NIFTI format. VolBrain software performs automatic quantitative analysis of the MR Data to improve the quality of the input image and is set in geometric space and intensity to segment and distinguish various structures and tissues. The segmentation process consists of the following steps: spatially adaptive non-local mean denoising, coarse non-uniformity correction, affine registration to MNI space, fine SPM-based non-uniformity correction, intensity normalization, non-local intracranial cavity extraction, tissue classification, non-local hemisphere segmentation and non-local subcortical structure segmentation [35]. After completing the above process, a split PDF report was automatically generated and provided via email. Tissue volume is reported as measured in cubic centimeters, and the ratio of the volume of a given structure to the total volume is presented as a percentage. We assessed the reports substantively and performed statistical analyses using percentage values from the volBrain procedure. In this study, we focused on the uncorrected volumes of the left and right caudate nucleus, the total volume of the caudate nucleus, and brain tissue [37].
Statistical analysis
Statistical analysis was performed using IBM SPSS version 26.0. Between-group differences in demographics, sleep, cognitive, and mental-behavioral variables were analyzed using t-tests and Mann-Whitney tests for continuous variables and chi-squared statistics for categorical variables. The independent samples t-test and Bonferroni corrected were used to compare brain tissue volume, total caudate nucleus volume, and the left and right caudate nucleus volumes between the EDS and non-EDS groups. Two-way ANOVA was used to analyze the association between the ApoE ɛ4 gene and brain tissue and caudate nucleus volume. The EDS group was also examined for its correlation with behavioral factors, caudate nucleus volume, and APOE ɛ4 gene (ɛ4 gene carriers versus non-carriers).
Stepwise regression analysis was conducted in the EDS group to assess whether cognitive-behavioral factors were correlated with brain tissue volume and caudate nucleus volume. Four domains of independent variables were entered in the model: sleep quality score, demographic score, cognitive domain score, and psychosomatic symptom score. The dependent variables were total caudate nucleus volume, left caudate nucleus volume, and right caudate nucleus volume. The covariates for all statistical analyses in this paper included age, gender, years of education, and only results with a two-tailed p value less than 0.05 were considered significant and reported.
RESULTS
Subject characteristics
Table 1 summarizes the results for the demographic and behavioral parameters, sleep quality and neuropsychological scores, and the corresponding between-group differences. No significant differences between EDS versus non-EDS groups in age, gender, years of education, BMI, APOE ɛ4 carriers/non-carriers. Significant between group-differences (p < 0.05) were found for PSQI (p = 0.013), AVLT-recognition (p = 0.005), and GDS (p = 0.047).
Table 1
Subjects Characteristics and Between-Group Differences
NC (n = 133) | EDS (n = 37) | p | |
Demographic parameters | |||
Age (y) | 66.22 (5.40) | 65.84 (5.77) | 0.725 |
Education (y) | 12.00 (3.03) | 12.14 (2.85) | 0.948 |
Gender (male/female) | 52/81 | 17/20 | 0.453# |
BMI | 24.28 (2.70) | 23.52 (2.78) | 0.145♦ |
APOE ɛ4 (+/–) | 40/93 | 8/29 | 0.312# |
Sleep assessments | |||
ESS | 5.03 (2.72) | 13.46 (2.94) | <0.001 |
RBDSQ | 1.51 (1.69) | 1.70 (2.17) | 0.832 |
PSQI | 4.04 (2.25) | 5.19 (2.56) | 0.013 |
Cognitive assessments | |||
MMSE | 28.89 (1.13) | 28.86 (1.20) | 0.945 |
MoCA-B | 26.08 (1.86) | 26.30 (1.71) | 0.467 |
AVLT-immediate recall | 6.78 (1.52) | 7.06 (1.21) | 0.295 |
AVLT-long-term delayed recall | 7.15 (2.13) | 7.59 (2.14) | 0.258 |
AVLT-Recognition | 22.20 (1.87) | 22.97 (1.40) | 0.005 |
STT-A (s) | 59.01 (19.13) | 64.70 (21.11) | 0.079 |
STT-B (s) | 132.49 (35.32) | 135.81 (28.67) | 0.600♦ |
AFT | 19.39 (4.11) | 19.19 (5.04) | 0.492 |
BNT | 25.52 (3.00) | 25.78 (2.38) | 0.988 |
Mental-behavioral assessments | |||
HAMD | 4.11 (4.10) | 3.97 (4.69) | 0.525 |
HAMA | 4.47 (4.23) | 3.78 (3.44) | 0.434 |
GDS | 2.29 (2.27) | 3.41 (3.18) | 0.047 |
#Chi-Square Test, ♦ T-Test, others use the Mann Whitney test. BMI, body mass index (calculated as weight in kilograms divided by height in meters squared); APOE, apolipoprotein E; SCD, subject cognitive decline; ESS, Epworth Sleepiness Scale; RBDSQ, REM Sleep Behavior Disorder Screening Questionnaire; PSQI, Pittsburgh sleep quality index; MMSE, Mini-Mental State; MOCA-B, Montreal Cognitive Assessment Basic Version; AVLT, Auditory Verbal Learning Test; STT-A, Shape Trails Test Part A, STT-B, Shape Trails Test Parts B; AFT, animal fluency tests; BNT, Boston Naming Test; HAMD, Hamilton Depression Rating Scale; HAMA, Hamilton Anxiety Scale; GDS, Geriatric Depression Scale.
Caudate nucleus and brain tissue volumes
Table 2 shows the t-test statistics regarding brain tissue and caudate nucleus volumes, with significant results for total caudate nucleus volume and left caudate nucleus volume. Additionally, the EDS group exhibited increased volumes of all caudate nuclei compared to the non-EDS group.
Table 2
Between-group differences in Caudate nucleus and Brain tissue features
NC | EDS | T | p | |
Tissue Brain (cm3) | 1082.36±9.78 | 1104.81±15.92 | 1.10 | 0.272 |
Caudate Total (cm3) | 6.44±0.08 | 6.88±0.26 | 2.09 | 0.038 |
Caudate Right (cm3) | 3.23±0.04 | 3.42±0.12 | 1.89 | 0.061 |
Caudate Left (cm3) | 3.21±0.04 | 3.45±0.15 | 2.23 | 0.027 |
Data is expressed as the means±SEM; covariates include gender, age, and years of education.
Table 3 and Fig. 1 present the comparison of APOE ɛ4 carriers and non-carriers in the EDS and 11 non-EDS groups, revealing a decrease in brain tissue and caudate nucleus volumes in APOE ɛ4 carriers compared to non-carriers in the non-EDS group, but not in the EDS group. Therefore, our findings suggest that in the non-EDS group, APOE ɛ4 carriers have reduced brain tissue and caudate volume compared with non-APOE ɛ4 carriers, whereas non-APOE ɛ4 carriers in EDS patients have increased brain tissue and caudate volume.
Table 3
The estimated value of interaction effect between APOE and diagnosis in Brain tissue and caudate nucleus features
GROUPS | APOE4 | Estimation | 95% CI | p |
Tissue Brain cm3 | ||||
EDS | ɛ4+ | 1089.04±27.86 | 1034.03–1144.06 | 0.158 |
ɛ4– | 1096.83±14.59 | 1068.02–1125.65 | ||
NC | ɛ4+ | 1045.07±12.47 | 1020.44–1069.69 | |
ɛ4– | 1102.23±8.13 | 1086.16–1118.30 | ||
Caudate Total cm3 | ||||
EDS | ɛ4+ | 6.73±0.38 | 5.98–7.48 | 0.498 |
ɛ4– | 6.86±0.20 | 6.46–7.25 | ||
NC | ɛ4+ | 6.14±0.17 | 5.80–6.47 | |
ɛ4– | 6.58±0.11 | 6.36–6.80 | ||
Caudate Right cm3 | ||||
EDS | ɛ4+ | 3.36±0.18 | 3.00–3.71 | 0.422 |
ɛ4– | 3.41±0.09 | 3.22–3.59 | ||
NC | ɛ4+ | 3.08±0.08 | 2.92–3.23 | |
ɛ4– | 3.31±0.05 | 3.20–3.41 | ||
Caudate Left cm3 | ||||
EDS | ɛ4+ | 3.37±0.20 | 2.96–3.78 | 0.578 |
ɛ4– | 3.45±0.10 | 3.23–3.66 | ||
NC | ɛ4+ | 3.06±0.09 | 2.87–3.24 | |
ɛ4– | 3.27±0.06 | 3.16–3.39 |
Data are presented as mean±SD; covariates include gender, age, and years of education; ɛ4+ was APOE ɛ4 carriers while ɛ4– was non-carriers.
Fig. 1
Between-group difference in EDS and control with APOE ɛ4 carriers and non-carriers at total, right and left caudate nucleus volume.
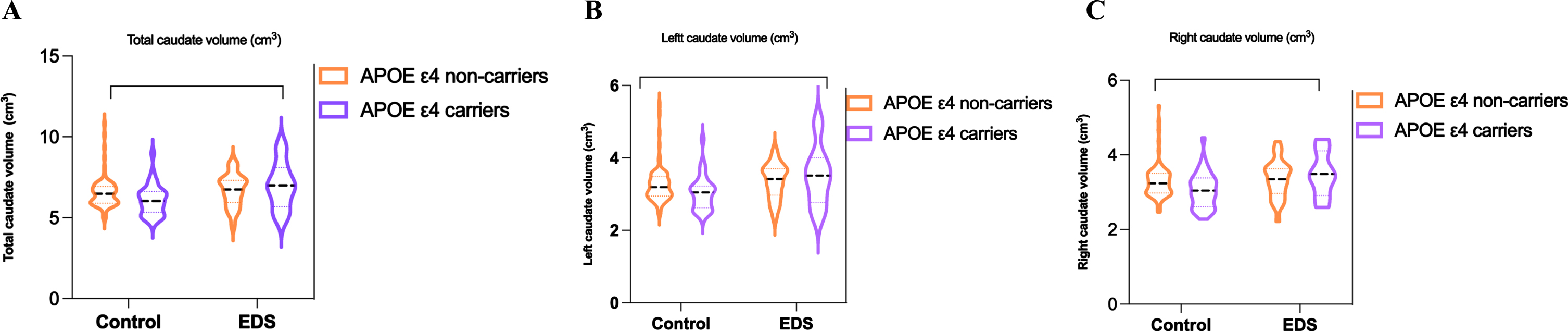
LINKS between EDS-associated caudate nucleus changes with cognitive-behavioral factors
Stepwise regression was conducted to determine the association between cognitive-behavioral factors and changes in caudate nucleus volume in the EDS group. AVLT-recognition was the variable most strongly correlated with increased caudate nucleus volume, especially for increased left caudate nucleus volume, accounting for 25% of the variance (p = 0.001) and 19.4% of the variance for right caudate nucleus volume (p = 0.006). Years of education also associated with total caudate nucleus volume, accounting for 9% of the variance (p = 0.03), and with right caudate nucleus volume, accounting for 11.7% of the variance (p = 0.022).
A simple linear analysis was used to investigate whether APOE ɛ4 plays a significant role in the increase in left caudate nucleus volume and AVLT-recognition scores in the EDS group. However, no significant association was found between the increase in left caudate nucleus volume and AVLT-recognition scores in the APOE ɛ4 EDS group. It is interesting to note that in the non-APOE ɛ4 EDS group, found that there was significantly negative correlation between the two (Fig. 2).
Fig. 2
Relationship between recognition recall score and left caudate nucleus volume in the EDS group. A) There is a significant negative correlation between recognition recall score and left caudate nucleus volume in the EDS. B) The significant negative correlation between recognition recall score and left caudate nucleus volume in the EDS with APOE ɛ4 non-carriers.
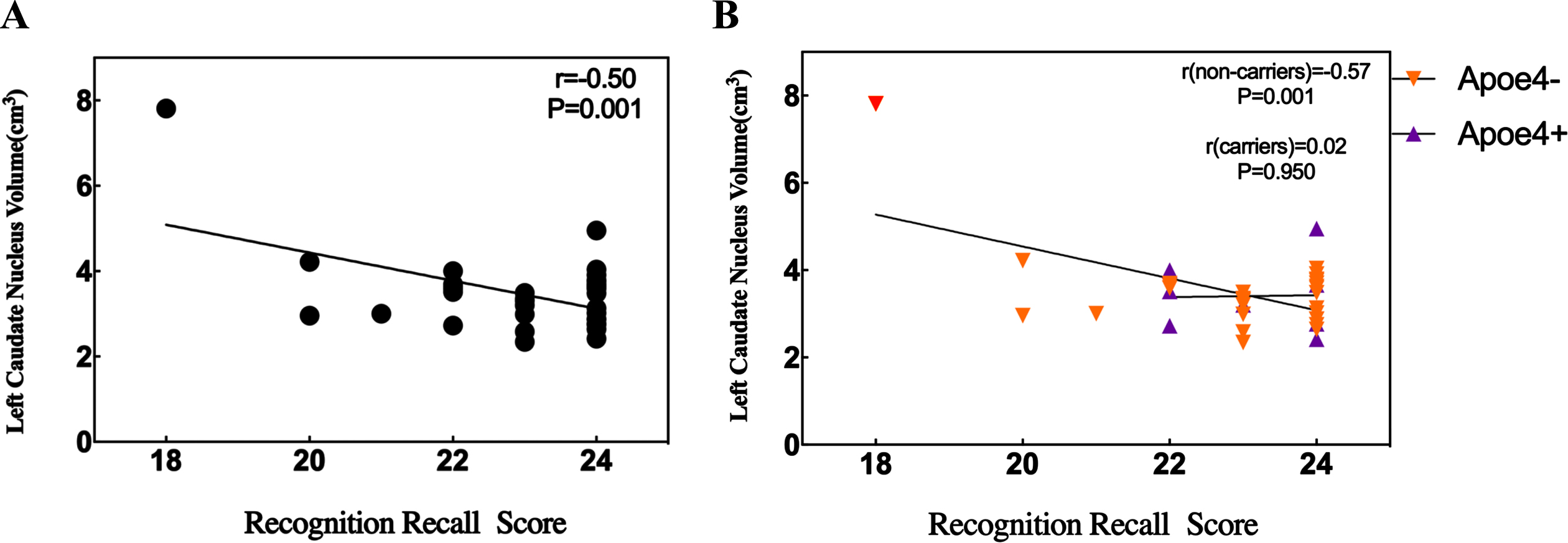
DISCUSSION
This study utilized sMRI scans and volBrain to investigate the relationship between EDS and caudate nucleus volume in patients with SCD. The results revealed significantly increased volume in both the total and left caudate nucleus in EDS group compared to the non-EDS group. The most significant cognitive behavioral factor associated with caudate nucleus volume in the EDS group was AVLT-recognition. Additionally, a significant negative correlation was found between the AVLT-recognition and caudate nucleus volume in ApoE ɛ4 non-carriers within the EDS group.
Increased volume of the caudate nucleus in patients with EDS
Neuroimaging studies have identified alterations in brain structure, function, and metabolic levels in patients with EDS and cognitive dysfunction [14]. EDS is associated with marked increases in daytime sleepiness due to the degeneration of the sleep and wakes regulatory system, which may interfere with neuronal metabolism and lead to enhanced and accelerated Aβ aggregation [1]. The amyloid cascade hypothesis suggests that amyloid aggregation and deposition is the initiating event for cognitive impairment [38]. Consistently, amyloid imaging studies in asymptomatic individuals have shown that the caudate nucleus may be the earliest site of amyloid deposition [39]. The increased volume of the caudate nucleus in EDS with SCD may be attributed to the elevated Aβ deposition, suggesting that the hypertrophy of the caudate nucleus could be due to a reactive neuronal response or an inflammatory reaction to Aβ, or both.
The caudate nucleus has a high density of dopaminergic terminals, and animal studies have shown that the caudate nucleus region is most affected by dopamine neurotoxicity. Aβ aggregation can cause changes in neuron shapes, increase the distance between neurons, and ultimately prevent communication between neurons [40]. Overwhelming evidence substantiates that the reduction in dopaminergic transmission mediates the increase in caudate nucleus volume [41]. Reduced dopamine transporter uptake in the presynaptic membrane of caudate dopamine neurons causes clinical EDS symptoms [14].
In our study, the caudate nucleus volume was increased in patients with EDS and SCD as compared to controls. The increased volume of the caudate nucleus in patients may reflect neurotrophic effects and microglial or inflammation involvement, partially compensating for the already impaired cognitive function [42]. Studies have shown that increased caudate nucleus volume impairs memory for attentional control and navigation tasks [43, 44]. Furthermore, EDS has been reported to be inextricably associated with MCI and AD [2, 45, 46]. In elderly women with dementia, their caudate nucleus volume was larger than that of non-dementia patients [16]. During the SCD stage, increased caudate nucleus volume was greater compared with healthy individuals, and was associated with global cognitive dysfunction [15]. Neuronal hypertrophy and inflammatory processes may be responsible for the initial increase in the volume of structures in the caudate nucleus, which gradually shrinks as the disease progresses [39]. Many studies have focused on the correlation between caudate nucleus volume and cognitive impairment, however, to our knowledge, few studies have reported the correlation of caudate volume in SCD and EDSpopulations.
The left caudate nucleus is vulnerable
In patients with EDS and SCD, the left caudate nucleus volume showed a more prominent increase in volume than the right, indicating that the left caudate nucleus is initially changed. This finding contrast with the SCD with non-EDS group. MRI studies have shown that changes in the brain’s left hemisphere occur earlier and progress more rapidly in patients with cognitive impairment than in the right hemisphere [47]. Similarly, Gunning-Dixon et al. observed that the volume of the left caudate nucleus was more significant than the right [48]. The enlargement of the caudate nucleus may be pathological, suggesting that the left caudate nucleus plays a more critical role in maintaining cognitive function [49]. Conversely, its atrophy may lead to more cognitive impairment [50]. Cortical atrophy in the left hemisphere occurs earlier and progresses faster in AD patients on MRI [47]. This asymmetry progresses more rapidly in those with mild cognitiveimpairment [51].
Memory associated with changes in caudate nucleus volume
The AVLT is possibility an effective representative test for assessing situational memory capable in early detection, which might indicate the early stage of MCI [52]. Situational memory belongs to the category of distant memory, which is the most advanced and late-maturing human memory system and the one most affected by aging [53]. In our study, the stepwise regression analysis showed that AVLT-recognition is a significantly associated with EDS-related changes in left caudate nucleus volume, particularly in APOE ɛ4 non-carriers. The larger the left caudate nucleus volume, the poorer the ability at recognition, which may suggest that changes in caudate nucleus volume may be an early sign of AD, in line with previous literature [54].
Studies suggest that the caudate nucleus plays a role in slower learning and memory, which is enhanced by repetition over time [55, 56], and it is related to memory, cognitive and delayed logical memory functions [18, 57], an increase in the caudate nucleus volume improves cognitive function and compensates for the neurological damage suffered [42, 58]. Emerging evidence suggests that EDS is positively associated with the severity of cognitive impairment [59]. In subjects with symptoms of EDS, changes in caudate nucleus volume may be an early sig [54].
However, this study has several limitations and shortcomings. Firstly, the cross-sectional design of the study limited its ability to comprehensively assess changes in neuroimaging parameters and their potential impact on cognitive function. Secondly, the association between caudate nucleus volume and EDS symptoms cannot be explained as a causal relationship. Thirdly, hippocampal volume is closely related to episodic memory function in SCD individuals, a direct comparison between the caudate nucleus and the hippocampus was not performed in present research. In future studies, adjust to a prospective study and compare changes in the caudate nucleus, the hippocampus and other brain structures horizontally, and follow up longitudinally to observe changes in brain structures as the diseaseprogresses.
Conclusions
In conclusion, our findings suggest that volume changes in the total and left caudate nucleus are pronounced in patients with SCD and EDS, the Auditory Verbal Learning Test-recognition is associate with left caudate nucleus volume in the SCD and EDS. The result of study provides evidence of the relationship between EDS, caudate nucleus volume and memory function in SCD patients, which may serve as a potential neurophysiological marker for the early detection of AD. Further research is needed to explore the causal relationship between EDS and caudate nucleus volume and to investigate the potential of the caudate nucleus as a therapeutic target for cognitive impairment.
AUTHOR CONTRIBUTIONS
Ziqian Feng (Writing – original draft); Jiayu Wang (Writing – original draft; Writing – review & editing); Li-Si Xu (Data curation); Jiajing Wu (Conceptualization); Hongyi Li (Conceptualization); Mingjun Duan (Writing – review & editing); Ziqi Wang (Data curation; Funding acquisition; Methodology; Resources; Supervision; Writing – review & editing).
ACKNOWLEDGMENTS
The authors would like to thank all colleagues involved in SILCODE for their dedication and the interviewees for their strong support of the research.
FUNDING
This study was supported by the National Natural Science Foundation of China (No. 82201628) and the Chengdu Municipal Bureau of Science and Technology Innovation R&D Project (No.2022-YF05-01883-SN).
CONFLICT OF INTEREST
The authors have no conflict of interest to report.
DATA AVAILABILITY
The clinical data supporting the findings of this study are available on request from the corresponding author. The data are not publicly available due to privacy or ethical restrictions.
REFERENCES
[1] | Dauvilliers Y ((2021) ) Hypocretin/orexin, sleep and Alzheimer’s disease. Front Neurol Neurosci 45: , 139–149. |
[2] | Jaussent I , Bouyer J , Ancelin ML , Berr C , Foubert-Samier A , Ritchie K , Ohayon MM , Besset A , Dauvilliers Y ((2012) ) Excessive sleepiness is predictive of cognitive decline in the elderly. Sleep 35: , 1201–1207. |
[3] | Jessen F , Amariglio RE , van Boxtel M , Breteler M , Ceccaldi M , Chetelat G , Dubois B , Dufouil C , Ellis KA , van der Flier WM , Glodzik L , van Harten AC , de Leon MJ , McHugh P , Mielke MM , Molinuevo JL , Mosconi L , Osorio RS , Perrotin A , Petersen RC , Rabin LA , Rami L , Reisberg B , Rentz DM , Sachdev PS , de la Sayette V , Saykin AJ , Scheltens P , Shulman MB , Slavin MJ , Sperling RA , Stewart R , Uspenskaya O , Vellas B , Visser PJ , Wagner M , Subjective Cognitive Decline Initiative Working G ((2014) ) A conceptual framework for research on subjective cognitive decline in preclinical Alzheimer’s disease. Alzheimers Dement 10: , 844–852. |
[4] | Amariglio RE , Mormino EC , Pietras AC , Marshall GA , Vannini P , Johnson KA , Sperling RA , Rentz DM ((2015) ) Subjective cognitive concerns, amyloid-beta, and neurodegeneration in clinically normal elderly. Neurology 85: , 56–62. |
[5] | Kielb S , Rogalski E , Weintraub S , Rademaker A ((2017) ) Objective features of subjective cognitive decline in a United States national database. Alzheimers Dement 13: , 1337–1344. |
[6] | Kim JH , Ahn JH , Min CY , Yoo DM , Choi HG ((2021) ) Association between sleep quality and subjective cognitive decline: Evidence from a community health survey. Sleep Med 83: , 123–131. |
[7] | André C , Tomadesso C , de Flores R , Branger P , Rehel S , Mézenge F , Landeau B , Sayette V , Eustache F , Chételat G , Rauchs G ((2019) ) Brain and cognitive correlates of sleep fragmentation in elderly subjects with and without cognitive deficits. Alzheimers Dement (Amst) 11: , 142–150. |
[8] | Sun Y , Yang FC , Lin CP , Han Y ((2015) ) Biochemical and neuroimaging studies in subjective cognitive decline: Progress and perspectives. CNS Neurosci Ther 21: , 768–775. |
[9] | Marcotte C , Potvin O , Collins DL , Rheault S , Duchesne S ((2019) ) Brain atrophy and patch-based grading in individuals from the CIMA-Q study: A progressive continuum from subjective cognitive decline to AD. Sci Rep 9: , 13532. |
[10] | Lee JE , Ju YJ , Park EC , Lee SY ((2020) ) Effect of poor sleep quality on subjective cognitive decline (SCD) or SCD-related functional difficulties: Results from 220,000 nationwide general populations without dementia. J Affect Disord 260: , 32–37. |
[11] | Joo HJ , Joo JH , Kwon J , Jang BN , Park EC ((2021) ) Association between quality and duration of sleep and subjective cognitive decline: A cross-sectional study in South Korea. Sci Rep 11: , 16989. |
[12] | Matz OC , Spocter M ((2022) ) The effect of Huntington’s disease on the basal nuclei: A review. Cureus 14: , e24473. |
[13] | Drori E , Berman S , Mezer AA ((2022) ) Mapping microstructural gradients of the human striatum in normal aging and Parkinson’s disease. Sci Adv 8: , eabm1971. |
[14] | Gong L , Li H , Yang D , Peng Y , Liu D , Zhong M , Zhang B , Xu R , Kang J ((2019) ) Striatum shape hypertrophy in early stage Parkinson’s disease with excessive daytime sleepiness. Front Neurosci 13: , 1353. |
[15] | Chen B , Wang Q , Zhong X , Mai N , Zhang M , Zhou H , Haehner A , Chen X , Wu Z , Auber LA , Rao D , Liu W , Zheng J , Lin L , Li N , Chen S , Chen B , Hummel T , Ning Y ((2022) ) Structural and functional abnormalities of olfactory-related regions in subjective cognitive decline, mild cognitive impairment, and Alzheimer’s disease. Int J Neuropsychopharmacol 25: , 361–374. |
[16] | Persson K , Bohbot VD , Bogdanovic N , Selbaek G , Braekhus A , Engedal K ((2018) ) Finding of increased caudate nucleus in patients with Alzheimer’s disease. Acta Neurol Scand 137: , 224–232. |
[17] | Jiji S , Smitha KA , Gupta AK , Pillai VP , Jayasree RS ((2013) ) Segmentation and volumetric analysis of the caudate nucleus in Alzheimer’s disease. Eur J Radiol 82: , 1525–1530. |
[18] | Madsen SK , Ho AJ , Hua X , Saharan PS , Toga AW , Jack CR Jr. , Weiner MW , Thompson PM ((2010) ) 3D maps localize caudate nucleus atrophy in 400 Alzheimer’s disease, mild cognitive impairment, and healthy elderly subjects. Neurobiol Aging 31: , 1312–1325. |
[19] | Jones NF , Ikuta T ((2022) ) Sleep duration is associated with Caudate volume and executive function. Brain Imaging Behav 16: , 2601–2607. |
[20] | Won J , Alfini AJ , Weiss LR , Nyhuis CC , Spira AP , Callow DD , Carson Smith J ((2019) ) Caudate volume mediates the interaction between total sleep time and executive function after acute exercise in healthy older adults. Brain Plast 5: , 69–82. |
[21] | Pan D , Zeng A , Yang B , Lai G , Hu B , Song X , Jiang T ((2023) ) Deep learning for brain MRI confirms patterned pathological progression in Alzheimer’s disease. Adv Sci (Weinh) 10: , e2204717. |
[22] | Dougherty RJ , Jonaitis EM , Gaitán JM , Lose SR , Mergen BM , Johnson SC , Okonkwo OC , Cook DB ((2021) ) Cardiorespiratory fitness mitigates brain atrophy and cognitive decline in adults at risk for Alzheimer’s disease. Alzheimers Dement (Amst) 13: , e12212. |
[23] | Vemuri P , Jack CR Jr. , ((2010) ) Role of structural MRI in Alzheimer’s disease. Alzheimers Res Ther 2: , 23. |
[24] | Akudjedu TN , Nabulsi L , Makelyte M , Scanlon C , Hehir S , Casey H , Ambati S , Kenney J , O’Donoghue S , McDermott E , Kilmartin L , Dockery P , McDonald C , Hallahan B , Cannon DM ((2018) ) A comparative study of segmentation techniques for the quantification of brain subcortical volume. Brain Imaging Behav 12: , 1678–1695. |
[25] | Lin L , Sun Y , Wang X , Su L , Wang X , Han Y ((2021) ) Resilience to plasma and cerebrospinal fluid amyloid-β in cognitively normal individuals: Findings from two cohort studies. Front Aging Neurosci 13: , 610755. |
[26] | Li X , Wang X , Su L , Hu X , Han Y ((2019) ) Sino Longitudinal Study on Cognitive Decline (SILCODE): Protocol for a Chinese longitudinal observational study to develop risk prediction models of conversion to mild cognitive impairment in individuals with subjective cognitive decline. BMJ Open 9: , e028188. |
[27] | Sun Y , Wang X , Wang Y , Dong H , Lu J , Scheininger T , Ewers M , Jessen F , Zuo XN , Han Y ((2019) ) Anxiety correlates with cortical surface area in subjective cognitive decline: APOE ɛ4 carriers versus APOE ɛ4 non-carriers. Alzheimers Res Ther 11: , 50. |
[28] | Bondi MW , Edmonds EC , Jak AJ , Clark LR , Delano-Wood L , McDonald CR , Nation DA , Libon DJ , Au R , Galasko D , Salmon DP ((2014) ) Neuropsychological criteria for mild cognitive impairment improves diagnostic precision, biomarker associations, and progression rates. J Alzheimers Dis 42: , 275–289. |
[29] | Sheng C , Yang K , He B , Du W , Cai Y , Han Y ((2022) ) Combination of gut microbiota and plasma amyloid-β as a potential index for identifying preclinical Alzheimer’s disease: A cross-sectional analysis from the SILCODE study. Alzheimers Res Ther 14: , 35. |
[30] | Alzheimer’s disease specialist in China (2018) Expert consensus on preclinical SCD diagnosis process and specification of Alzheimer’s disease in China. |
[31] | Chen G , Yang K , Du W , Hu X , Han Y ((2019) ) Clinical characteristics in subjective cognitive decline with and without worry: Baseline investigation of the SILCODE study. J Alzheimers Dis 72: , 443–454. |
[32] | Gandhi KD , Mansukhani MP , Silber MH , Kolla BP ((2021) ) Excessive daytime sleepiness: A clinical review. Mayo Clin Proc 96: , 1288–1301. |
[33] | Ahmed AE , Fatani A , Al-Harbi A , Al-Shimemeri A , Ali YZ , Baharoon S , Al-Jahdali H ((2014) ) Validation of the Arabic version of the Epworth sleepiness scale. J Epidemiol Glob Health 4: , 297–302. |
[34] | Boeve A , Ferman TJ , Aakre J , St Louis E , Silber M , Machulda M , Fields J , Graff-Radford N , Mielke M , Geda Y , Jones D , Graff-Radford J , Knopman D , Petersen R , Boeve B ((2019) ) Excessive daytime sleepiness in major dementia syndromes. Am J Alzheimers Dis Other Demen 34: , 261–264. |
[35] | Manjon JV , Coupe P ((2016) ) volBrain: An online MRI brain volumetry system Front Neuroinform 10: , 30. |
[36] | Kim S , Greene DJ , D’Andrea CB , Bihun EC , Koller JM , O’Reilly B , Schlaggar BL , Black KJ ((2020) ) Hippocampal volume in provisional tic disorder predicts tic severity at 12-month follow-up. J Clin Med 9: , 1715. |
[37] | Acosta H , Jansen A , Kircher T ((2021) ) Larger bilateral amygdalar volumes are associated with affective loss experiences. J Neurosci Res 99: , 1763–1779. |
[38] | Klunk WE , Price JC , Mathis CA , Tsopelas ND , Lopresti BJ , Ziolko SK , Bi W , Hoge JA , Cohen AD , Ikonomovic MD , Saxton JA , Snitz BE , Pollen DA , Moonis M , Lippa CF , Swearer JM , Johnson KA , Rentz DM , Fischman AJ , Aizenstein HJ , DeKosky ST ((2007) ) Amyloid deposition begins in the striatum of presenilin-1 mutation carriers from two unrelated pedigrees. J Neurosci 27: , 6174–6184. |
[39] | Ryan NS , Keihaninejad S , Shakespeare TJ , Lehmann M , Crutch SJ , Malone IB , Thornton JS , Mancini L , Hyare H , Yousry T , Ridgway GR , Zhang H , Modat M , Alexander DC , Rossor MN , Ourselin S , Fox NC ((2013) ) Magnetic resonance imaging evidence for presymptomatic change in thalamus and caudate in familial Alzheimer’s disease. Brain 136: , 1399–1414. |
[40] | Zhang Y , Xiao Z , He Z , Chen J , Wang X , Jiang L ((2020) ) Dendritic complexity change in the triple transgenic mouse model of Alzheimer’s disease. PeerJ 8: , e8178. |
[41] | Jacobsen LK , Giedd JN , Gottschalk C , Kosten TR , Krystal JH ((2001) ) Quantitative morphology of the caudate and putamen in patients with cocaine dependence. Am J Psychiatry 158: , 486–489. |
[42] | Jan RK , Lin JC , Miles SW , Kydd RR , Russell BR ((2012) ) Striatal volume increases in active methamphetamine-dependent individuals and correlation with cognitive performance. Brain Sci 2: , 553–572. |
[43] | Ersche KD , Barnes A , Jones PS , Morein-Zamir S , Robbins TW , Bullmore ET ((2011) ) Abnormal structure of frontostriatal brain systems is associated with aspects of impulsivity and compulsivity in cocaine dependence. Brain 134: , 2013–2024. |
[44] | Hartley T , Harlow R ((2012) ) An association between human hippocampal volume and topographical memory in healthy young adults. Front Hum Neurosci 6: , 338. |
[45] | Merlino G , Piani A , Gigli GL , Cancelli I , Rinaldi A , Baroselli A , Serafini A , Zanchettin B , Valente M ((2010) ) Daytime sleepiness is associated with dementia and cognitive decline in older Italian adults: A population-based study. Sleep Med 11: , 372–377. |
[46] | Nakakubo S , Doi T , Makizako H , Tsutsumimoto K , Hotta R , Kurita S , Kim M , Suzuki T , Shimada H ((2019) ) Sleep condition and cognitive decline in Japanese community-dwelling older people: Data from a 4-year longitudinal study. J Sleep Res 28: , e12803. |
[47] | Wachinger C , Salat DH , Weiner M , Reuter M , Alzheimer’s Disease Neuroimaging Initiative ((2016) ) Whole-brain analysis reveals increased neuroanatomical asymmetries in dementia for hippocampus and amygdala. Brain 139: , 3253–3266. |
[48] | Gunning-Dixon FM , Head D , McQuain J , Acker JD , Raz N ((1998) ) Differential aging of the human striatum: A prospective MR imaging study. AJNR Am J Neuroradiol 19: , 1501–1507. |
[49] | Beauchamp MH , Dagher A , Aston JA , Doyon J ((2003) ) Dynamic functional changes associated with cognitive skill learning of an adapted version of the Tower of London task. Neuroimage 20: , 1649–1660. |
[50] | Bartrés-Faz D , Junqué C , Serra-Grabulosa JM , López-Alomar A , Moya A , Bargalló N , Mercader JM , Moral P , Clemente IC ((2002) ) Dopamine DRD2 Taq I polymorphism associates with caudate nucleus volume and cognitive performance in memory impaired subjects. Neuroreport 13: , 1121–1125. |
[51] | Liu C , Lee SH , Hernandez-Cardenache R , Loewenstein D , Kather J , Alperin N ((2021) ) Poor sleep is associated with small hippocampal subfields in cognitively normal elderly individuals. J Sleep Res 30: , e13362. |
[52] | Guo Q , Zhao Q , Chen M , Ding D , Hong Z ((2009) ) A comparison study of mild cognitive impairment with 3 memory tests among Chinese individuals. Alzheimer Dis Assoc Disord 23: , 253–259. |
[53] | Yu S , Xu H , Wu C , Jiang X , Sun R , Sun L ((2023) ) Bionic path planning fusing episodic memory based on RatSLAM. Biomimetics (Basel) 8: , 59. |
[54] | Mijalkov M , Veréb D , Canal-Garcia A , Volpe G , Pereira JB ((2023) ) Directed functional brain connectivity is altered in sub-threshold amyloid-β accumulation in cognitively normal individuals. Neurosci Insights 18: , 26331055231161625. |
[55] | Seger CA , Cincotta CM ((2005) ) The roles of the caudate nucleus in human classification learning. J Neurosci 25: , 2941–2951. |
[56] | Strafella AP , Paus T , Fraraccio M , Dagher A ((2003) ) Striatal dopamine release induced by repetitive transcranial magnetic stimulation of the human motor cortex. Brain 126: , 2609–2615. |
[57] | Bauer E , Toepper M , Gebhardt H , Gallhofer B , Sammer G ((2015) ) The significance of caudate volume for age-related associative memory decline. Brain Res 1622: , 137–148. |
[58] | Penalba-Sánchez L , Oliveira-Silva P , Sumich AL , Cifre I ((2022) ) Increased functional connectivity patterns in mild Alzheimer’s disease: A rsfMRI study. Front Aging Neurosci 14: , 1037347. |
[59] | Luyster FS , Choi J , Yeh CH , Imes CC , Johansson AE , Chasens ER ((2015) ) Screening and evaluation tools for sleep disorders in older adults. Appl Nurs Res 28: , 334–340. |