PREHAB vs. REHAB – presurgical treatment in vestibular schwannoma surgery enhances recovery of postural control better than postoperative rehabilitation: Retrospective case series
Abstract
OBJECT:
To evaluate post-surgical postural stability when treating patients with remaining vestibular function with intratympanic gentamicin (PREHAB) prior to schwannoma surgery.
METHOD:
44 consecutive patients with some form remaining vestibular function scheduled for vestibular schwannoma surgery. 20 were medically deafferented with intratympanic gentamicin before surgery and 24 were not. Both groups were of the same age, had the same tumor size, same type of surgery, and same perioperative sensory rehabilitation (training exercises), and no surgical complications. Postural stability measured as energy expenditure while standing on a force platform during vibratory stimulation of the calf muscles, performed prior to surgery (or gentamicin treatment) and 6 months after surgery.
RESULTS:
Patients pretreated with gentamicin had significantly better postural stability at the time for follow-up (p < 0.05) and displayed a better adaptive capacity when faced with a postural challenge (p < 0.01). They were also able to use vision more efficiently to control their stability (p < 0.05).
CONCLUSIONS:
By separating the sensory loss (through intratympanic gentamicin, that ablates the remaining vestibular function) from the intracranial surgical trauma, the postural control system benefited from a better short-term (adaptation) and long-term (habituation) recovery, when experiencing a postural challenge or resolving a sensory conflict. The benefits could be attributed to; active and continuous motor learning as the vestibular function slowly attenuates; no concomitant central nervous dysfunction due to effects from neurosurgery, thus allowing time for a separate unimpeded recovery process with more limited challenges and objectives; and the initiation and certain progression of sensory reweighting processes allowed prior to surgery. In contrast, worse compensation could be due to; immobilization from nausea after surgery, harmful amount of stress and cognitive dysfunction from the combination of surgical and sensory trauma and an abrupt vestibular deafferentation and its consequences on sensory reweighting.
1Introduction
Vestibular schwannoma surgery is unique in the aspect that a specific date can be set for a complete unilateral vestibular deafferentation (uVD). As such, it offers both exceptional opportunities to make an intervention, as well as studying the effects from uVD with different time-courses. At our hospital, we have since 2004 treated patients with gentamicin in order to reduce the vestibular function prior to surgery. The results immediately postoperatively are most satisfying [37]. Patients are mobilized quickly and do not suffer from vertigo. However, disequilibrium after uVD in vestibular schwannoma surgery is the most significant problem patients experience in quality of life surveys [18], and although the postural problems most often subside, they can be protracted and restrict daily life activities long after surgery [35, 42]. The importance of compensation after a vestibular deficit cannot be underestimated, since it directly correlates to the risk for an injurious fall that might seriously hurt an individual as well as lead to high healthcare costs [12, 22, 31]. This paper specifically analyzes the effect of gentamicin treatment (vestibular PREHAB) on postural control dynamics.
The residual function of the vestibular system before surgery differs considerably between patients, due to individual extent and influence of tumor growth, resulting in varying spectra of post-surgery illness as well as vestibular symptoms. An acute uVD, if significant vestibular function remains prior to surgery, invariably results in spontaneous nystagmus, head and ocular tilt, postural disequilibrium, altered vestibulo-ocular and spinal reflexes [8], and of course severe nausea and vertigo. Many symptoms resolve within the first week after uVD, however, the dynamic loss of reflexes persists and remain functionally inadequate and asymmetric [9]. The vestibular compensation process ultimately results in a restoration of the intrinsic activity of the vestibular nuclei in the brainstem [10, 58]. Immediately following an uVD there is asymmetric nuclei activity where the intact side becomes initially increased [30, 34], after which cerebellar inhibition depresses the activity from the vestibular nuclei bilaterally [39]. In the last and longest compensatory stage of vestibular compensation, the symptoms gradually resolve and the spinal imbalance normalizes and behavioral recovery is initiated [8, 33]. In this process plastic changes of the activity of the vestibulocerebellum are essential [7, 30]. Following an uVD, rehabilitation exercises have been proved to be of utmost importance [13, 21, 23], and indeed also an early start of training promotes rehabilitation [53]. Presurgical physical activity is also beneficial to postsurgical postural stability [17] and in the PREHAB concept both these important aspects of physical activity are considered.
The nausea/vertigo induced by surgery and sudden uVD can impede the vestibular compensation process and in extension, also increase the need for more extensive rehabilitation. The vestibular PREHAB protocol (Fig. 1) was developed in order to address this problem and to ensure an enhanced and sufficient rehabilitation [37]. The protocol encompass treating patients that have measurable vestibular function before surgery with intratympanic gentamicin injections, thus producing gradually a uVD over a period of 3-4 weeks, whilst performing daily vestibular exercises before and after the gentamicin treatment [37]. Through this procedure the sensory trauma is separated from the surgical trauma, making it possible for vestibular compensation to ensue as the vestibular function gradually decline from gentamicin toxicity [38], unimpeded by any depression of central nervous function that might result from schwannoma surgery or the acute stages of an uVD (Fig. 2). During the acute stage after schwannoma surgery the cerebellar inhibition of the intact vestibular function (though essential from a coping perspective of the individual) might impede the following compensatory stage. Furthermore, surgery in itself leads to an increase of cytokines and other neurotransmitters within the spinal fluid and cerebral circulation that can cross the blood-brain barrier and affect important structures like the hippocampus and cerebellum, causing a decrease in functional plasticity, cognitive dysfunction and memory formation [49, 57]. Although static symptoms like spontaneous nystagmus are supposed to disappear during the first days after uVD, such symptoms can persist after schwannoma surgery up to the 8th day [43], which might be a manifestation of delayed vestibular compensation and possibly attributed to neuroinflammation in central nervous structures.
Fig.1
The algorithm for the present study also defining the vestibular PREHAB protocol. n = the participants in each arm for the present study.
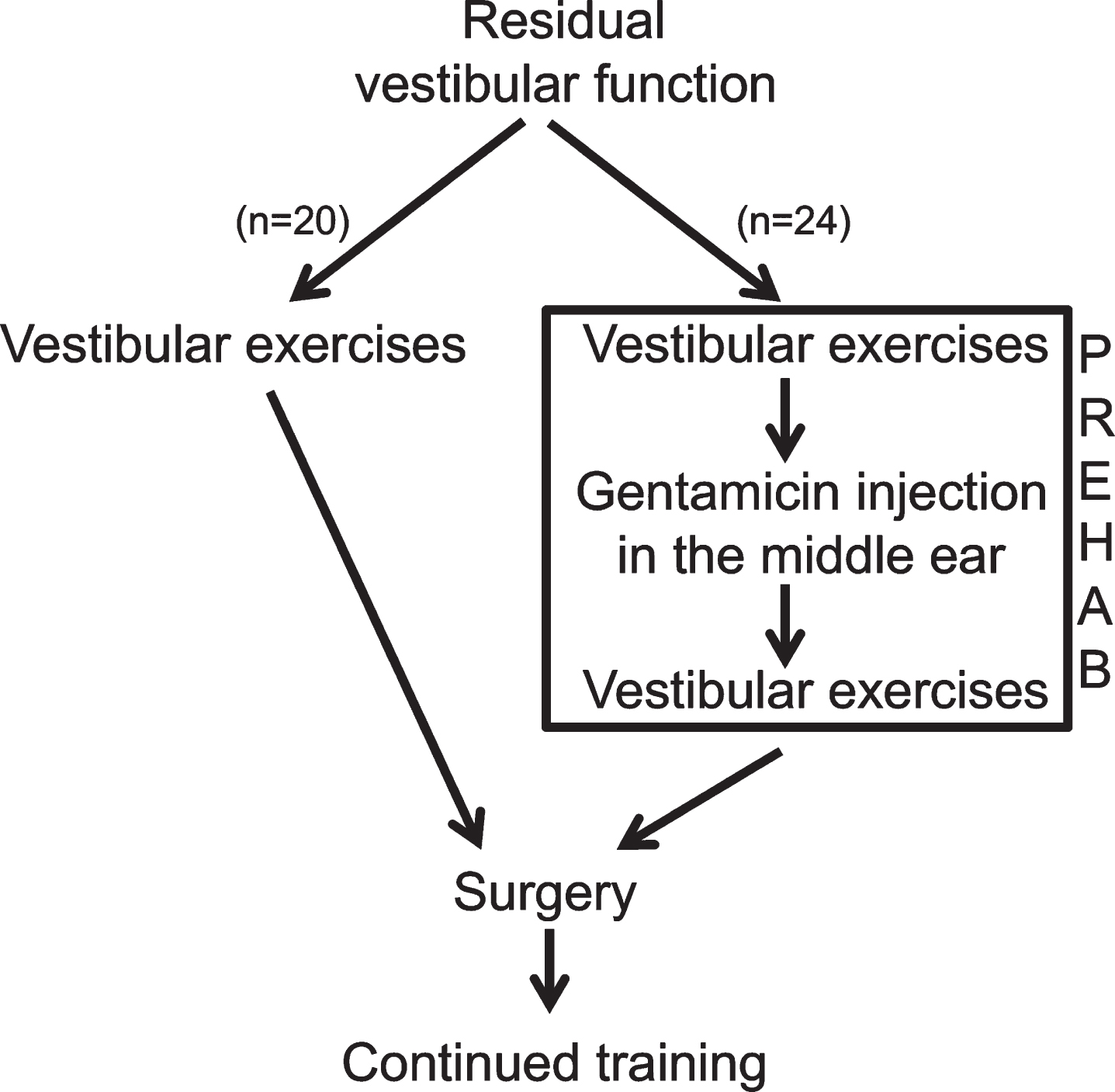
Fig.2
Theory behind the vestibular PREHAB protocol. In A, an abrupt deafferentation cause intense vestibular symptoms initially that gradually subsides as the cerebellar inhibition develops. Compensation becomes delayed as a consequence due to the inhibition as well as from the intensity of symptoms. In B. the vestibular function gradually attenuates with little or no symptoms allowing compensation to ensue unimpeded.
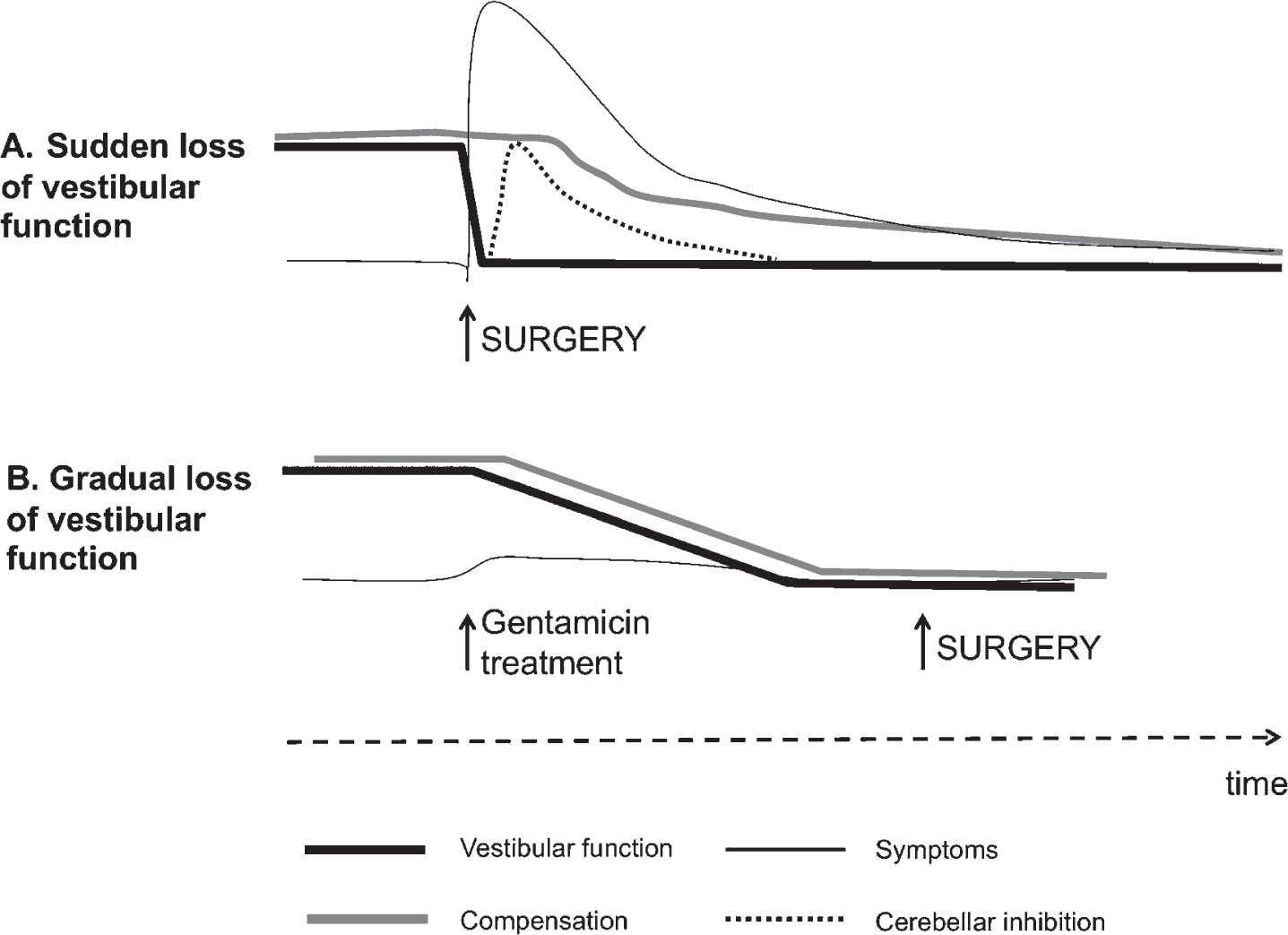
One way to investigate postural function is by the use of dynamic posturography. Apart from measuring actual postural performance, the test also assess the ability of the control system to resolve sensory conflicts over time [14]. This capacity is essential for coping with the postural demands in everyday life. Adaptation and habituation are important features for a dynamic postural control system for managing changing internal (sensorimotor) or external (postural challenges) constraints. Postural control is maintained by both feedback and feed-forward mechanisms [27]. Feedback control depends on sensory input (vision, vestibular and somatosensory) that are processed, integrated and weighted to their relative importance and context in the CNS [45]. Feed-forward mechanisms depend upon previous postural and sensory experience and involve the concept of “internal models”, whose output consists of preformed neuromuscular strategies activated in given situations automatically or voluntarily (anticipated movement). Repeated exposure to a postural perturbation, such as vibratory proprioceptive stimulation, generate a long-term memory through a consolidation process, similar to the general paradigm of memory formation [54]. The generated postural response corresponds to an internal model, designed to control posture when exposed to the vibratory stimulation, and most likely involve areas within the CNS involved in adaptation and learning, i.e. cerebellum and hippocampus [2, 40, 48], i.e. structures affected by both uVD and neuroinflammation.
The aim for the present study was to evaluate the dynamics of the postural control system and if it, and hence the human capacity of upright stance, benefits from a gradual sensory attenuation prior to surgery (vestibular PREHAB) in patients subjected to vestibular schwannoma surgery.
2Material and methods
Between the years 2001 and 2011, 153 patients received vestibular schwannoma surgery at Lund University Hospital. During the initial assessment of the patients the vestibular function was investigated with video-recorded head impulse test of all 3 canals of each ear, bi-thermal calorics, vestibular evoked myogenic potential measured on the sternocleidomastoid muscle (cVEMP), subjective horizontal and vertical, rod and frame tests, posturography and eye movement analyses. The inclusion criteria to the retrospective study was remaining vestibular function on the affected ear and exclusion criteria; signs of CNS affection (Bruhns nystagmus, ataxia etc.). In 64 patients the criteria were met. Twenty-nine patients were subjected to gentamicin therapy while 35 did not receive gentamicin installations prior to surgery (19 were diagnosed before 2004 (i.e. before the presurgical gentamicin treatment was introduced), 14 were candidates for hearing preserving surgery, 1 when surgery was deemed not be delayed by the PREHAB program due to tumor size (29 mm) and 1 because of concurrent malignancy). From these 64 patients posturography data both pre and post-surgery could be retrieved in 44 patients in total, 24 patients who were not treated with gentamicin and 20 patients who were. Nine patients treated with gentamicin had incomplete data; 4 lived too far away for follow-up (reported well), 2 suffered from other diseases and did not want to come (malignancies) and 3 did not perform posturography at the follow up due to laboratory issues (computer failure/move of laboratory). Eleven patients not treated with gentamicin had incomplete data; 2 lived too far away (reported well), 1 could not perform posturography at all before or after surgery, 2 could not perform posturography with their eyes closed after surgery, 5 patients did not perform posturography before surgery due to lab issues (computer failure/move of laboratory/wrong vibrators) and 1 patient was tested prior to a known diagnose and posturography was not performed. The subjects were not prospectively randomized into an active intervention, but they do represent all patients treated with microsurgery with complete data during the study years. The only active criteria for not participating in the PREHAB program was the (surgeons) ambition to preserve hearing and no criteria for inclusion/exclusion based on vestibular function or postural performance prior to surgery, nor age nor tumor size.
Group 1 consisted of patients not treated with gentamicin, serving as controls for the intervention, 14 males and 10 females, aged 50.0±12.4 years. Half of the patients were submitted to translabyrinthine extirpation, the other half to retrosigmoidal approach. Tumor extrameatal size was 17.3±9.0 mm.
Group 2 consisted of patients treated with gentamicin according to the vestibular PREHAB protocol, 9 males and 11 females, aged 51.4±13.6 years. Nine of the patients were submitted to translabyrinthine extirpation, the remaining 11 to retrosigmoidal approach. Tumor extrameatal size was 17.5±6.3 mm.
At the time for follow-up 6 months after surgery the same tests as above were performed except for calorics and cVEMP. Only the posturography data are presented in this study, whereas the vestibular tests served the purpose of screening tools to ensure that all subjects included in the study fulfilled the inclusion and exclusion criteria. All patients performed vestibular exercises 2 weeks before and at least 6 weeks after surgery, and in the case of gentamicin treatment, 2 weeks before and 6 weeks after the intratympanic injections [55]. Adherence to exercise instructions was not checked in any of the patients, but every patient was reminded at each contact (evaluation, gentamicin installation, before and after surgery). The exercises were designed to the train the vestibulo-ocular reflex (VOR) in different postural settings, including dynamic gait and VOR training [55].
2.1Ethics statement
Ethical approval was obtained through the local ethical board (EPN, Lund, Sweden). Specific ethical approval for gentamicin treatment was not necessary since that followed a set clinical practice. All patients provided informed oral consent (approved by the board and documented in the medical records), and the study was performed according to the Helsinki declaration of 2004.
2.2Test procedure
Postural control was evaluated by perturbing stance while standing on a force platform (400×400×75 mm) equipped with six strain-gauge sensors. Forces and torques actuated by the feet were recorded with six degrees of freedom by a force platform. Data were sampled at 50 Hz by a computer equipped with a 16-bit AD converter. The vibrations were applied simultaneously to both the lateral and medial gastrocnemius muscles and to both legs by two cylindrical vibrators (0.06 m long and 0.01 m in diameters), held in place with an elastic strap around each leg. The vibration amplitude was 1.0 mm at a constant frequency of 85 Hz. Of note, the vibration is produced by a small electrical motor that rotates a weight placed asymmetrically on an axis. This device is completely surrounded by but not touching a circular unyielding plastic casing. Thus, a light or strong pressure on this plastic casing from the attachment on the muscles will not affect the vibration produced. The vibratory stimulation was executed according to a computer controlled pseudorandom binary sequence (PRBS) schedule [25] for 205 seconds by turning on/off the vibratory stimulation. The PRBS schedule was composed of stimulation shift periods with random duration between 0.8–6.4 seconds (yielding an effective bandwidth of 0.1–2.5 Hz). Thus, the designated PRBS stimuli covered a broad power spectrum and the randomized stimulation reduced the opportunity to make anticipative and pre-emptive adjustments. However, of note, although the balance perturbations have a specific spectral bandwidth, the human postural control responses to these perturbations may not be linear in spectral space or appear at the same frequency. For example, a common strategy to handle different kinds of balance perturbations is to make slow modulations below 0.1 Hz of the body’s multi-segmental alignment [15]. Another example of indirect stimulus-response relationships in spectral space is that closing the eyes, i.e., vision is known to be mainly receptive to body movements slower than 0.1 Hz, produce a large increase in postural control activity above 0.1 Hz [14].
After information about the test procedure the subjects were instructed to stand erect but not at attention, with arms crossed over the chest and feet at an angle of about 30 degrees open to the front and the heals approximately 3 cm apart. Two tests were conducted at each trial occasion, eyes open, fixating on mark on the wall at a distance of 1.5 m, and eyes closed. The test order followed our set clinical procedure, always starting with eyes open followed by eyes closed. In order to minimize the external disturbances for the test subjects, the recordings were performed while the test subjects listened to classical music relayed through headphones.
2.3Data analysis
The variance of torque was calculated for five periods for each test: the quiet stance period (0–30 seconds) before stimulation was applied, and from four periods (1–4) during the stimulation (see Fig. 3). In the data analysis, the variance of recorded torque was divided into three spectral categories, viz. total, low frequency (<0.10 Hz), and high frequency (>0.10 Hz. A fifth-order digital Finite duration Impulse Response (FIR) filter [47], with filter components selected to avoid aliasing was used for spectral separation. The frequency cut-off level of 0.1 Hz was based on Fast Fourier Transformation (FFT) analysis of the sway composition under eyes closed and eyes open conditions [46]. The frequency limit at 0.1 Hz was also based upon empirical tests on recorded body sway, which have shown that this frequency limit is efficient when separating between fast corrective movements to maintain balance, and the smooth corrective changes in the overall stance. The data were normalized by squared mass and squared height since regression analysis showed dependence on those factors.
Fig.3
Posturography test. Division of each recorded test into quiet stance and 4 perturbation periods. Each test lasted for 230 seconds, with an initial 30 s recording of quiet stance before any perturbing stimulus was applied, and subsequent 200 s of perturbing stimuli. The perturbation periods were split into four periods during the stimulation (30–80, 80–130, 130–180, and 180–230 seconds, respectively). Each of the perturbation periods contained the same amplitude and duration of perturbing stimulus.
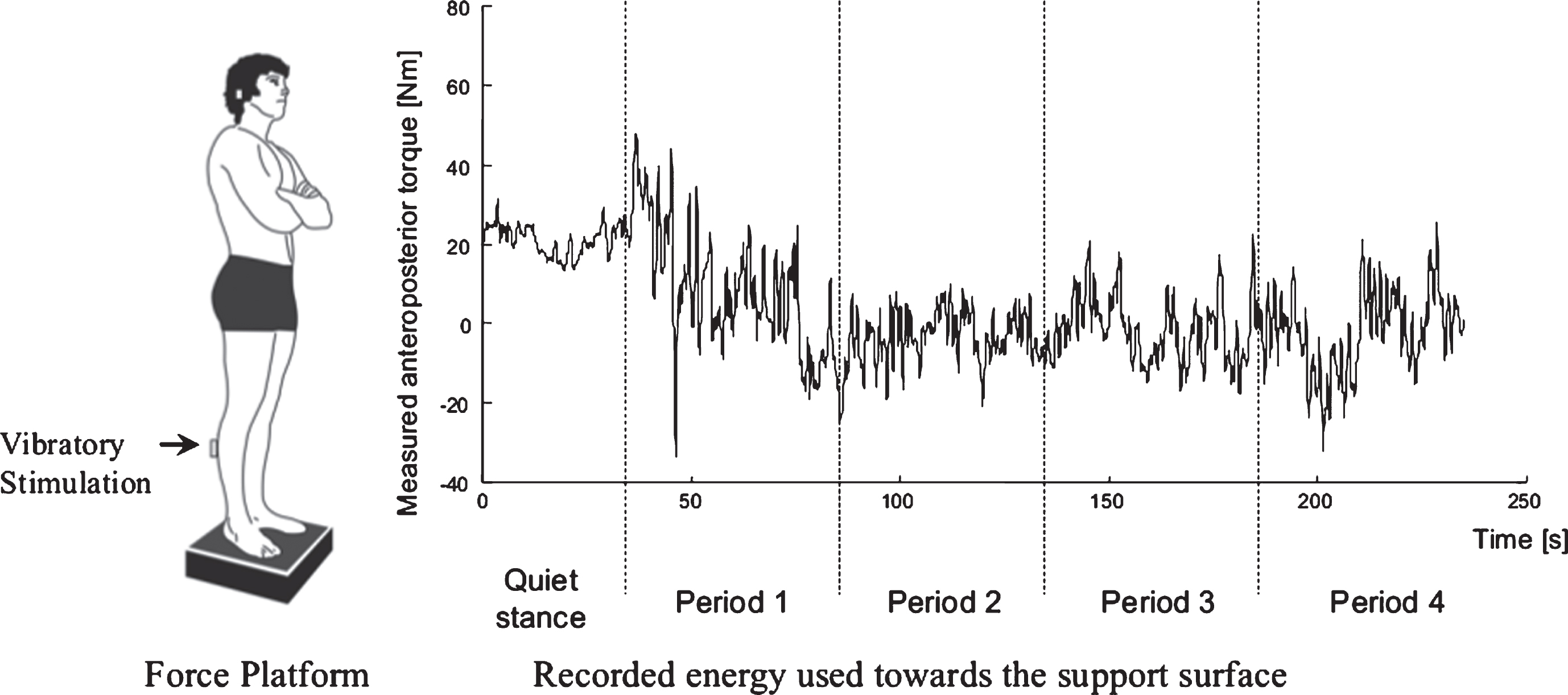
Although the vibratory perturbations predominately cause sway in the anteroposterior plane, postural control in humans is bipedal with weight transfer between the feet as well as sway in the lateral plane (although it being quantitatively less) [16]. For these reasons both anteroposterior and lateral sway were analyzed.
Postural stability while standing is commonly analyzed using force platforms and the movements of the center of pressure (CoP), i.e., the point of application of the ground reaction force. In this study, we present torque variance values from the force platform recording because these values corresponds directly with the energy used towards the support surface to maintain stability, normalized for anthropometrical differences between subjects [26, 36], and changes in recorded torque from the force platform correspond well to the actual body movements and posture changes induced by vibratory stimulus [15]. However, though mathematically processed differently (i.e., including anthropometrical normalization), the information gathered from recording torque is identical to recording CoP [44].
2.4Statistical analysis
The anteroposterior and lateral torque variance values within three spectral ranges (Total, <0.1 Hz and > 0.1 Hz) during quiet (unperturbed) stance and during balance perturbations were analyzed using repeated measures GLM ANOVA (General Linear Model univariate Analysis of Variance) on log-transformed values. The main factors and subsequent factor interactions analyzed were: ‘Gentamicin” (Received gentamicin, No gentamicin; d.f. 1) groups; “Pre/Post” (Before, After; d.f. 1) receiving surgery and in one group gentamicin treatment, “Vision” (Eyes closed, Eyes open; d.f. 1) posturography, and when applicable ‘Period’ (Vibration periods 1, 2, 3, 4; d.f. 3) during posturography.
The Mann-Whitney U (Exact sig. 2-tailed) test was used for between-groups post hoc comparisons. The Wilcoxon matched-pairs signed-rank test (Exact sig. 2-tailed) was used for with-subjects post hoc comparisons, i.e., analyzing the changes between before and after treatments. Spearman correlation test was used for examining whether there were any significant relationships on individual level between recorded postural stability and whether the patient had received gentamicin installations. Correlation analyses were also performed to exclude that the two patient populations were asymmetrically affected by tumor size, age, and surgical technique or had significantly different caloric responses from the ipsilateral and contralateral sides relative to the position of the tumor.
In all analyses, p-values <0.05 were considered statistically significant, whereas trends were defined as (p < 0.1). No individual dataset was used more the once in the Mann-Whitney and Wilcoxon post hoc comparisons, thus, significant Bonferroni corrected level was p < 0.05. Non-parametric statistical tests were used in all statistical evaluations since the Shapiro-Wilk test revealed that some of the obtained datasets were not normally distributed and normal distribution could not be obtained after log-transformation.
3Results
3.1Differences between patient groups
There were no differences in age, tumor size, surgical technique or vestibular function between the groups prior to surgery.
3.2Multivariate repeated measures GLM ANOVA evaluation
3.2.1Quiet stance stability
The GLM ANOVA results from quiet stance are demonstrated in Table 1. The parameter gentamicin yielded significant better stability, i.e., the patients had lower torque variance values, and thus, used less energy in lateral direction both in total and high frequency range (p≤0.029). Moreover, the positive effect of vision was clearly apparent on used energy in all frequency ranges and in both anteroposterior (p < 0.001) and lateral directions (p≤0.005).
Table 1
Effects of Gentamicin, Pre/post treatments and vision on the stability during quiet stance
Quiet Stance | Gentamicin | Pre/Post | Vision |
Antero Posterior | |||
Total | 0.163 | 0.103 | < 0.001 |
[2.0] | [2.8] | [72.7] | |
>0.1 Hz | 0.290 | 0.078 | < 0.001 |
[1,1] | [3.3] | [116.1] | |
<0.1 Hz | 0.392 | 0.219 | < 0.001 |
[0.7] | [1.6] | [22.9] | |
Lateral | |||
Total | 0.022 | 0.821 | < 0.001 |
[5.7] | [0.1] | [16.4] | |
>0.1 Hz | 0.029 | 0.521 | < 0.001 |
[5.1] | [0.4] | [16.5] | |
<0.1 Hz | 0.197 | 0.381 | 0.005 |
[1.7] | [0.8] | [8.8] |
Repeated measures GLM ANOVA analysis of how the quiet stance stability were affected by main factors “Gentamicin” group, “Pre/post” treatments, and “Vision” alone and by the main factor interactions. Nene of the interactions were significant and are therefore not shown in the table. The notation “<0.001” means that the p-value is smaller than 0.001. The F-values are presented within the squared parenthesis below the p-values.
3.2.2Perturbed stance stability
During perturbed stance, the gentamicin patients had significantly better stability than the non-treated subjects as reflected by significantly lower total energy (p = 0.032) in anteroposterior direction and lower energy in all spectral ranges (p≤0.044) in lateral direction (Table 2). The combination of gentamicin with pre/post-surgery yielded a significant better stability in the gentamicin group during vibratory perturbation as reflected by lower low frequency energy in anteroposterior direction (p = 0.027), and lower total energy in lateral direction (p = 0.039) post-surgery.
Table 2
Effects of Gentamicin, Pre/post treatments, vision and vibration period on the stability during balance perturbations
Vibratory Perturbation | Gentamicin | Pre/post | Vision | Period | Pre/post x Gentamicin | Vision x Gentamicin | Pre/post x Vision | Pre/post x Period | Pre/post x Period x Gentamicin | Vision x Period | Vision x Period x Gentamicin |
Antero Posterior | |||||||||||
Total | 0.032 | 0.037 | < 0.001 | < 0.001 | 0.061 | 0.044 | 0.015 | 0.424 | 0.240 | 0.026 | 0.282 |
[4.9] | [4.6] | [135.0] | [109.6] | [3.7] | [4.3] | [6.4] | [0.7] | [1.4] | [5.3] | [1.2] | |
>0.1 Hz | 0.059 | 0.018 | < 0.001 | < 0.001 | 0.118 | 0.099 | 0.045 | 0.049 | 0.330 | 0.253 | 0.115 |
[3.8] | [6.0] | [194.2] | [100.6] | [2.6] | [2.8] | [4.3] | [4.1] | [1.0] | [1.3] | [2.6] | |
<0.1 Hz | 0.136 | 0.248 | 0.001 | < 0.001 | 0.027 | 0.492 | 0.684 | 0.527 | 0.101 | 0.145 | 0.047 |
[2.3] | [1.4] | [12.1] | [39.2] | [5.2] | [0.5] | [0.2] | [0.4] | [2.8] | [2.2] | [4.2] | |
Lateral | |||||||||||
Total | 0.017 | 0.029 | < 0.001 | < 0.001 | 0.039 | 0.004 | 0.374 | 0.669 | 0.004 | 0.783 | 0.918 |
[6.2] | [5.1] | [83.8] | [50.1] | [4.6] | [9.0] | [0.8] | [0.2] | [9.3] | [0.1] | [0.0] | |
>0.1 Hz | 0.041 | 0.037 | < 0.001 | < 0.001 | 0.071 | 0.002 | 0.232 | 0.379 | 0.013 | 0.601 | 0.222 |
[4.5] | [4.6] | [123.5] | [28.3] | [3.4] | [11.4] | [1.5] | [0.8] | [6.8] | [0.3] | [1.5] | |
<0.1 Hz | 0.044 | 0.047 | < 0.001 | < 0.001 | 0.057 | 0.049 | 0.826 | 0.305 | 0.064 | 0.688 | 0.192 |
[4.3] | [4.2] | [15.8] | [33.9] | [3.8] | [4.1] | [0.0] | [1.1] | [3.6] | [0.2] | [1.8] |
Repeated measures GLM ANOVA analysis of how the perturbed stance stability were affected by main factors “Gentamicin” group, “Pre/post” treatments, “Vision” and “Period” alone and by their main factor interactions. Only main factor combinations that yielded significant results are presented in the table.
The results also indicate that stability was overall better after surgery (p≤0.047) and that vision, when available, contributed greatly for stability in all spectral ranges (p≤0.001). The combination gentamicin and vision yielded significant improvement in stability most pronounced in lateral direction (p≤0.049) but also in the anteroposterior plane of direction (p = 0.044), reflecting that beyond the general beneficial impact of vision has on stability, gentamicin treated subjects profited more.
The triple combination of gentamicin, pre/post-surgery and period yielded significant improvement in the lateral total (p = 0.004) and high frequency stability (p = 0.013), reflecting that the gentamicin treated subjects after surgery were more able to improve their stability and use less energy over time, and thus, better adapted to cope with the vibratory induced perturbations. Additionally, the combination gentamicin, vision and period, shows that in the low frequency anteroposterior stability (p = 0.047), the gentamicin treated subjects were better able to handle the 1st period of vibratory perturbation tests with eyes open, i.e., when the effects of the balance perturbations still were novel to the subjects.
3.3Post hoc evaluations
3.3.1Between-groups comparisons
Figure 4 A-D show the posturography data before and after surgery in both groups. In Table 3 the difference in amount of torque variance, reflecting the stability difference between the groups, is shown before and after surgery. For all significant differences found, the group that did not receive gentamicin performed worse than the group that did receive gentamicin. Although there were some significant differences in stability before surgery (Table 3), the differences became systematically larger at the follow-up assessments performed 6 months after surgery. The improvement in stability was most pronounced during closed eyes condition after surgery in the total spectra and in the frequency range >0.1 Hz in both anteroposterior (p≤0.032) and lateral directions (p≤0.009).
Table 3
Stability differences pre- and post-surgery between the group that received gentamicin treatment and the group that received no gentamicin pre-surgery
Quiet stance | Period 1 | Period 2 | Period 3 | Period 4 | ||
Antero Posterior | Closed Eyes | |||||
Before Surgery | ||||||
Total | 0.420 | 0.071 | 0.067 | 0.092 | 0.112 | |
>0.1 Hz | 0.649 | 0.246 | 0.136 | 0.060 | 0.075 | |
<0.1 Hz | 0.434 | 0.368 | 0.583 | 1.000 | 0.599 | |
After Surgery | ||||||
Total | 0.088 | 0.002 | 0.016 | 0.006 | 0.011 | |
>0.1 Hz | 0.276 | 0.008 | 0.032 | 0.011 | 0.023 | |
<0.1 Hz | 0.030 | 0.088 | 0.046 | 0.136 | 0.028 | |
Open Eyes | ||||||
Before Surgery | ||||||
Total | 0.287 | 0.935 | 0.861 | 0.683 | 0.462 | |
>0.1 Hz | 0.297 | 0.916 | 0.718 | 0.771 | 0.462 | |
<0.1 Hz | 0.935 | 0.700 | 0.935 | 0.843 | 0.567 | |
After Surgery | ||||||
Total | 0.287 | 0.025 | 0.201 | 0.004 | 0.041 | |
>0.1 Hz | 0.148 | 0.046 | 0.079 | 0.071 | 0.038 | |
<0.1 Hz | 0.991 | 0.567 | 0.297 | 0.003 | 0.079 | |
Lateral | Closed Eyes | |||||
Before Surgery | ||||||
Total | 0.054 | 0.054 | 0.256 | 0.092 | 0.043 | |
>0.1 Hz | 0.162 | 0.118 | 0.380 | 0.266 | 0.088 | |
<0.1 Hz | 0.079 | 0.536 | 0.806 | 0.129 | 0.064 | |
After Surgery | ||||||
Total | 0.170 | 0.008 | < 0.001 | 0.003 | 0.003 | |
>0.1 Hz | 0.148 | 0.009 | 0.001 | 0.006 | 0.007 | |
<0.1 Hz | 0.567 | 0.320 | < 0.001 | 0.007 | 0.028 | |
Open Eyes | ||||||
Before Surgery | ||||||
Total | 0.218 | 0.700 | 1.000 | 0.825 | 0.583 | |
>0.1 Hz | 0.434 | 0.898 | 0.683 | 0.771 | 0.552 | |
<0.1 Hz | 0.380 | 0.898 | 0.771 | 0.567 | 0.972 | |
After Surgery | ||||||
Total | 0.032 | 0.129 | 0.064 | 0.010 | 0.016 | |
>0.1 Hz | 0.006 | 0.210 | 0.201 | 0.057 | 0.026 | |
<0.1 Hz | 0.476 | 0.380 | 0.118 | 0.022 | 0.102 |
*For all significant differences found, the group that did not receive gentamicin performed worse than the group that did receive gentamicin. The differences in size between groups are displayed in Fig. 4.
Fig.4
A-D. Torque variance before, and 6 months after surgery. Torque variance (mean and standard error of mean) in total sway, >0.1 Hz and <0.1 Hz in both groups before and after surgery during the various visual conditions and direction of postural sway. Above each graph is the mean difference during the vibratory perturbation and between pre and postsurgical postural assessments presented. (+) indicates less stability at the follow up (6months), (–) indicates better stability at the follow up (6months).
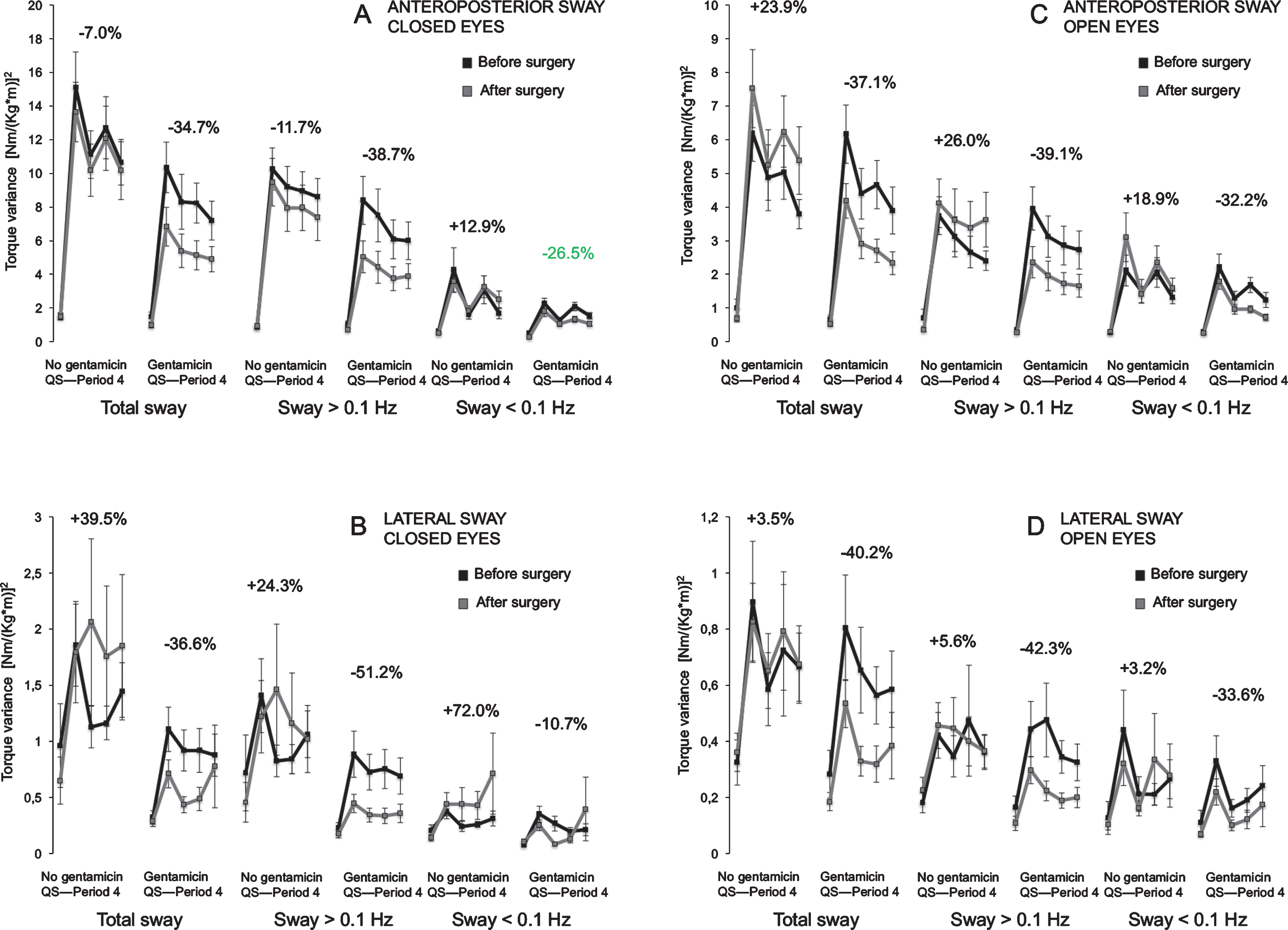
3.3.2Within-subjects comparisons
In Table 4 the difference in amount of torque variance, reflecting the stability change between before and after surgery in each patient group, is shown. The stability was improved only in the population submitted to gentamicin treatment pre-surgery while no improvements were observed in the non-treated group. The gentamicin group predominantly enhanced the stability during closed eyes condition after surgery in the total spectra and in the frequency range >0.1 Hz in both anteroposterior (p≤0.017) and lateral directions (p≤0.044).
Table 4
Stability change between pre- and post-surgery for the group that received gentamicin treatment and the group that received no gentamicin pre-surgery
Quiet stance | Period 1 | Period 2 | Period 3 | Period 4 | ||
Antero Posterior | Closed Eyes | |||||
No Gentamicin | ||||||
Total | 0.877 | 0.229 | 0.491 | 0.603 | 0.390 | |
>0.1 Hz | 0.509 | 0.303 | 0.264 | 0.406 | 0.095 | |
<0.1 Hz | 0.390 | 0.584 | 0.303 | 0.623 | 0.390 | |
Gentamicin | ||||||
Total | 0.114 | 0.006 | 0.017 | 0.003 | 0.006 | |
>0.1 Hz | 0.245 | 0.011 | 0.015 | 0.006 | 0.009 | |
<0.1 Hz | 0.027 | 0.231 | 0.368 | 0.030 | 0.009 | |
Open Eyes | ||||||
No Gentamicin | ||||||
Total | 0.527 | 0.663 | 0.663 | 0.152 | 0.812 | |
>0.1 Hz | 0.208 | 0.944 | 0.812 | 0.643 | 0.833 | |
<0.1 Hz | 0.726 | 0.473 | 0.812 | 0.623 | 0.900 | |
Gentamicin | ||||||
Total | 0.245 | 0.064 | 0.064 | 0.002 | 0.053 | |
>0.1 Hz | 0.048 | 0.009 | 0.040 | 0.015 | 0.027 | |
<0.1 Hz | 0.475 | 0.388 | 0.261 | 0.001 | 0.058 | |
Lateral | Closed Eyes | |||||
No Gentamicin | ||||||
Total | 0.790 | 0.422 | 0.136 | 0.833 | 0.643 | |
>0.1 Hz | 0.941 | 0.565 | 0.877 | 0.663 | 0.178 | |
<0.1 Hz | 0.527 | 0.705 | 0.188 | 0.922 | 0.643 | |
Gentamicin | ||||||
Total | 0.729 | 0.044 | 0.019 | 0.024 | 0.388 | |
>0.1 Hz | 0.430 | 0.048 | 0.017 | 0.005 | 0.090 | |
<0.1 Hz | 0.312 | 0.189 | 0.011 | 0.083 | 0.452 | |
Open Eyes | ||||||
No Gentamicin | ||||||
Total | 0.331 | 0.944 | 0.833 | 0.623 | 0.790 | |
>0.1 Hz | 0.331 | 0.989 | 0.747 | 0.603 | 0.726 | |
<0.1 Hz | 0.390 | 0.643 | 0.684 | 0.944 | 0.747 | |
Gentamicin | ||||||
Total | 0.648 | 0.261 | 0.008 | 0.003 | 0.058 | |
>0.1 Hz | 0.261 | 0.114 | 0.011 | 0.003 | 0.048 | |
<0.1 Hz | 0.812 | 0.294 | 0.133 | 0.114 | 0.114 |
*All significances found represent an improvement in performance from pre-surgery to post-surgery. Only the group that received gentamicin improved performance. The differences in size between groups are displayed in Fig. 4.
3.3.3Correlation evaluation of Gentamicin pre-surgery treatment effect
In Table 5 correlations between the recorded stability (i.e. amount of torque variance) and the factor gentamicin are demonstrated. As expected, the correlations were in all but one case non-significant pre-surgery. However, post- surgery a large number significant correlations appeared, both anteroposterior and lateral directions as well as in both visual conditions, and all with negative correlation coefficients. A negative R-correlation reflects a better postural stability in gentamicin treated subjects after surgery.
Table 5
Correlation between postural stability and whether the patients were treated pre-surgery with gentamicin
Before Treatment | After Treatment | |||||||||
A. Closed Eyes | Quiet stance | Period 1 | Period 2 | Period 3 | Period 4 | Quiet stance | Period 1 | Period 2 | Period 3 | Period 4 |
Antero posterior | ||||||||||
Total | 0.416 | 0.069 | 0.065 | 0.090 | 0.110 | 0.085 | 0.002 | 0.014 | 0.005 | 0.010 |
[–0.126] | [–0.277] | [–0.280] | [–0.259] | [–0.244] | [–0.262] | [–0.456] | [–0.367] | [–0.413] | [–0.385] | |
>0.1 Hz | 0.643 | 0.243 | 0.133 | 0.058 | 0.073 | 0.273 | 0.007 | 0.030 | 0.010 | 0.022 |
[–0.072] | [–0.180] | [–0.230] | [–0.288] | [–0.273] | [–0.169] | [–0.403] | [–0.327] | [–0.385] | [–0.345] | |
<0.1 Hz | 0.429 | 0.364 | 0.578 | 1.000 | 0.594 | 0.028 | 0.085 | 0.044 | 0.133 | 0.027 |
[–0.122] | [–0.140] | [–0.086] | [0.000] | [0.083] | [–0.331] | [–0.262] | [–0.306] | [–0.230] | [–0.334] | |
Lateral | ||||||||||
Total | 0.052 | 0.052 | 0.253 | 0.090 | 0.041 | 0.167 | 0.007 | < 0.001 | 0.003 | 0.002 |
[–0.295] | [–0.295] | [–0.176] | [–0.259] | [–0.309] | [–0.212] | [–0.399] | [–0.525] | [–0.439] | [–0.449] | |
>0.1 Hz | 0.160 | 0.115 | 0.377 | 0.263 | 0.085 | 0.146 | 0.008 | 0.001 | 0.005 | 0.006 |
[–0.216] | [–0.241] | [–0.137] | [–0.173] | [–0.262] | [–0.223] | [–0.395] | [–0.485] | [–0.417] | [–0.406] | |
<0.1 Hz | 0.077 | 0.531 | 0.799 | 0.127 | 0.062 | 0.562 | 0.316 | < 0.001 | 0.006 | 0.027 |
[–0.270] | [–0.097] | [–0.040] | [–0.234] | [–0.284] | [–0.090] | [–0.155] | [–0.550] | [–0.406] | [–0.334] | |
Antero posterior | ||||||||||
Total | 0.283 | 0.926 | 0.853 | 0.676 | 0.457 | 0.283 | 0.023 | 0.198 | 0.004 | 0.039 |
[–0.165] | [–0.014] | [–0.029] | [–0.065] | [–0.115] | [–0.165] | [–0.341] | [–0.198] | [–0.428] | [–0.313] | |
>0.1 Hz | 0.294 | 0.908 | 0.711 | 0.763 | 0.457 | 0.146 | 0.044 | 0.077 | 0.069 | 0.036 |
[–0.162] | [0.018] | [–0.058] | [–0.047] | [–0.115] | [–0.223] | [–0.306] | [–0.270] | [–0.277] | [–0.316] | |
<0.1 Hz | 0.926 | 0.694 | 0.926 | 0.835 | 0.562 | 0.982 | 0.562 | 0.294 | 0.002 | 0.077 |
[0.014] | [0.061] | [0.014] | [0.032] | [–0.090] | [0.004] | [–0.090] | [–0.162] | [–0.449] | [–0.270] | |
Lateral | ||||||||||
Total | 0.215 | 0.694 | 1.000 | 0.817 | 0.578 | 0.030 | 0.127 | 0.062 | 0.009 | 0.014 |
[–0.191] | [–0.061] | [0.000] | [–0.036] | [–0.086] | [–0.327] | [–0.234] | [–0.284] | [–0.392] | [–0.367] | |
>0.1 Hz | 0.429 | 0.889 | 0.676 | 0.763 | 0.546 | 0.005 | 0.207 | 0.198 | 0.055 | 0.025 |
[–0.122] | [0.022] | [0.065] | [0.047] | [–0.093] | [–0.413] | [–0.194] | [–0.198] | [–0.291] | [–0.338] | |
<0.1 Hz | 0.377 | 0.889 | 0.763 | 0.562 | 0.963 | 0.471 | 0.377 | 0.115 | 0.020 | 0.099 |
[–0.137] | [–0.022] | [0.047] | [–0.090] | [–0.007] | [–0.111] | [–0.137] | [–0.241] | [–0.349] | [–0.252] |
Spearman correlation test examining relationships between recorded postural stability and whether the patients were treated with gentamicin installations or not during posturography with A) Closed eyes and B) Open eyes. The correlation R-values are presented within the squared parenthesis below the p-values. A negative R-value reflects that subjects treated pre-surgery with gentamicin used less energy, and thus, had better stability.
4Discussion
Patients benefitted from the vestibular PREHAB protocol in two important aspects of postural control. One was a better ability to cope with vibratory postural perturbation at the time for follow-up. This is line with results previous published on a small sample of patients [55], however the present data also support the benefit in patients when operated with retrosigmoidal approach. The other benefit was a greater adaptability to the vibratory stimulation, manifested as better coping of a postural disturbance and use of less energy over time during the tests [54]. Both the above effects could be described as processes of adaptation, the former being a more long-term habituation to a better preparedness to sensory conflicts as such, and the latter a more efficient short-term adaptive behavior to the current sensory context (vibration). The parameter recorded in this study during posturography, torque variance, is equivalent to energy spent maintaining stance [36], so physical evidence support that postural control was maintained with more ease, and thus, that the postural control system was more efficient in resolving the sensory conflict vibratory calf perturbation entails [54]. Furthermore, less energy expenditure in maintaining postural control, also implicate an increased physical reserve capacity to cope with a slip or any other sudden threat to upright posture.
The explanation to these effects on the postural control system cannot be found within the peripheral vestibular system, so it must be an effect from processes within the central nervous system. It has been suggested that the postural disturbances, that patients with vestibular deficits experience, are related to the loss of vestibular function in the role as an orientational and internal reference to other conflicting sensory input [41]. The vestibular nuclei in the brainstem receives afferent sensory information also from vision and somatosensation, making the nuclei an important integrative and a first relay station for multisensory processing [50]. Following an uVD, extra-vestibular input and neck proprioception in particular has been shown to be important for the sensory reweighting, which seems to occur within the nuclei complex [50]. Possibly due to its intimate connections with the nodulus and flocculus (cerebellum) [3]. The vestibular training before surgery, that all patients executed, aim at initiating and establishing motor learning also at a cellular level before the actual lesion, in line with multiple plasticity mechanisms active in cerebellar and hippocampal adaptation [1, 2, 28]. The ability to perform active head movements and not being hindered by acute oscillopsia probably enhance the cellular mechanisms involved both in the brainstem as well as cerebellum [11].
Performing the exercises simultaneously as the remaining vestibular sensory information on the schwannoma side gradually subsides induced by the gentamicin treatment [38], enabled patients in the vestibular PREHAB group to continuously compensate and adapt to the changing sensory information [5], both preparing the patients for the resultant sensory context (unilateral vestibular deaferentation) as well as in priming the postural control system to function in a new sensory context. Furthermore, the nausea and vertigo experienced if vestibular deafferentation occurs abruptly restrict patients to strict supine positioning because any head movements cause severe increases in nausea, oscillopsia and vertigo symptoms. This physical inactivity, and thus also limited activation of the remaining sensory systems that can provide accurate postural control information, might be counterproductive, since early active movements promote compensation and inactivity delay rehabilitation [33]. Here it must be stressed that vertigo and nausea from gentamicin deafferentation were slight and did not restrict the patients in their daily activities, an experience mirrored by those from treating debilitating Menieres disease with intratympanic gentamicin.
It might be beneficial if compensation processes essential for coping with altered sensory information and in resolving arising sensory conflicts are initiated at a time when there are as little influences on central processes as possible. Neurosurgery as such may interfere with hippocampal function [29, 49], and post-operative cognitive and memory function in particular, can both become impaired [4, 24]. Also simply the gradual decrease of vestibular function over a couple of days might be important and beneficial to adaptation and compensation, since consolidation processes involved in adaptation and learning are, if not dependent upon, then greatly beneficially affected by sleep [48, 52]. Deafferentation through gentamicin would enable the consolidation processes to evolve gradually and continue unabated. Furthermore the combination of stress loads (perioperative stress + sensory trauma) might exceed the well-documented beneficial aspects of stress and could thus be detrimental for compensatory plastic changes throughout the nervous system [51].
The ability to use visual cues for stabilizing posture, important in the feedback control seemed to be better in the vestibular PREHAB patients. This could partly be attributed to the above reasoning, but possibly also to the fact that the vestibular training before surgery (in patients that did not receive intratympanic gentamicin) was performed in a sensory context that was going to change abruptly following surgery. When compensating for an unilateral vestibular loss the individual weighting of remaining senses are recalibrated [32], and specific training after surgery is beneficial [6, 21]. Specific vestibular rehabilitation is used to enhance central vestibular compensation mechanisms when they are insufficient or to accelerate recovery [20], and are efficient when the deafferentation is manifest. However, it could be argued that sensory training might cement existing sensory weighting, which could impede or disarray central vestibular compensation processes in another sensory setting [56]. Taken together, training in the ‘wrong’ sensory context (that will abruptly change at the time for surgery) [54] together with a possible lesser central nervous ability to adapt, may account for the worse postural outcomes for the group that did not receive the PREHAB protocol. That being stated, a familiarity with the exercises before surgery is also important, since beneficial aspects of training after a manifest unilateral loss is beyond question, and although the sensory weighting might be affected, the muscle memory for the exercises are important in post-surgical rehabilitation [19].
No study has been published from other centers with the specific intervention described. We have previously, in a case study, reported findings suggesting enhanced postural control functions after surgery in 6 patients enrolled in the vestibular PREHAB protocol [55]. The strength of the present study is that it was performed on a substantially larger number of patients and the findings could be compared with a matching control group, making statistical comparisons feasible as well as providing substantiated evidence for that under identical conditions the enhancements found after using the PREHAB procedure were systematic in nature and of practical and clinical relevant size.
The weakness of the study is that no other assessment of balance other than posturography with vibratory perturbation was performed. However, the test itself is robust and has been shown to be able to analyze postural adaptation, and has the great benefit to measure equivalence to energy expenditure, making the results easier to interpret.
The implications from this study are at present limited to those patients with vestibular function prior to deafferentation surgery (approximately 42% of the patients at our clinic). However considering that symptoms of vertigo is common and spontaneous nystagmus occur after surgery, even if the caloric responsiveness is void or very low [43], it follows that it is possible that most patients have residual vestibular function and would in theory benefit from vestibular PREHAB. Further research in this aspect would be required.
5Conclusion
The findings suggest that by separating the sensory loss from the surgical trauma in patients with remaining vestibular function prior to surgery, both long-term postural control as well as short-term adaptive mechanisms is improved when facing postural challenges.
Disclosure
The study was supported by a grant from ALF (Medical Training and Research Agreement) and is a national agreement between the Swedish government and seven county councils.
References
[1] | Boele H.J. , Koekkoek S.K. , De Zeeuw C.I. and Ruigrok T.J., Axonal sprouting and formation of terminals in the adult cerebellum during associative motor learning, J Neurosci 33: ((2013) ), 17897–17907. |
[2] | Boyden E.S. , Katoh A. and Raymond J.L. , Cerebellum-dependent learning: The role of multiple plasticity mechanisms, Annu Rev Neurosci 27: ((2004) ), 581–609. |
[3] | Broussard D.M. , Titley H.K. , Antflick J. and Hampson D.R. , Motor learning in the VOR: The cerebellar component, Exp Brain Res 210: ((2011) ), 451–463. |
[4] | Caza N. , Taha R. , Qi Y. and Blaise G. , The effects of surgery and anesthesia on memory and cognition, Prog Brain Res 169: ((2008) ), 409–422. |
[5] | Cohen H.S. , Disability and rehabilitation in the dizzy patient, Curr Opin Neurol 19: ((2006) ), 49–54. |
[6] | Cohen H.S. , Gottshall K.R. , Graziano M. , Malmstrom E.M. , Sharpe M.H. and Whitney S.L. , International guidelines for education in vestibular rehabilitation therapy, J Vestib Res 21: ((2011) ), 243–250. |
[7] | Courjon J.H. , Flandrin J.M. , Jeannerod M. and Schmid R. , The role of the flocculus in vestibular compensation after hemilabyrinthectomy, Brain Res 239: ((1982) ), 251–257. |
[8] | Cullen K.E. , Minor L.B. , Beraneck M. and Sadeghi S.G. , Neural substrates underlying vestibular compensation: Contribution of peripheral versus central processing, J Vestib Res 19: ((2009) ), 171–182. |
[9] | Curthoys I.S. , Vestibular compensation and substitution, Curr Opin Neurol 13: ((2000) ), 27–30. |
[10] | Curthoys I.S. and Halmagyi G.M. , Vestibular compensation: A review of the oculomotor, neural, and clinical consequences of unilateral vestibular loss, J Vestib Res 5: ((1995) ), 67–107. |
[11] | Dutheil S. , Brezun J.M. , Leonard J. , Lacour M. and Tighilet B. , Neurogenesis and astrogenesis contribution to recovery of vestibular functions in the adult cat following unilateral vestibular neurectomy: Cellular and behavioral evidence, Neuroscience 164: ((2009) ), 1444–1456. |
[12] | Ekvall Hansson E. and Magnusson M., Vestibular asymmetry predicts falls among elderly patients with multi- sensory dizziness, BMC Geriatr 13: ((2013) ), 77. |
[13] | Enticott J.C. , J. O’Leary S and Briggs R.J., Effects of vestibulo-ocular reflex exercises on vestibular compensation after vestibular schwannoma surgery, Otol Neurotol 26: ((2005) ), 265–269. |
[14] | Fransson P.A. , Hafstrom A. , Karlberg M. , Magnusson M. , Tjader A. and Johansson R. , Postural control adaptation during galvanic vestibular and vibratory proprioceptive stimulation, IEEE Trans Biomed Eng 50: ((2003) ), 1310–1319. |
[15] | Fransson P.A. , Hjerpe M. and Johansson R. , Adaptation of multi-segmented body movements during vibratory proprioceptive and galvanic vestibular stimulation, J Vestib Res 17: ((2007) ), 47–62. |
[16] | Fransson P.A. , Johansson R. , Tjernstrom F. and Magnusson M. , Adaptation to vibratory perturbations in postural control, IEEE Eng Med Biol Mag 22: ((2003) ), 53–57. |
[17] | Gauchard G.C. , Parietti-Winkler C. , Lion A. , Simon C. and Perrin P.P. , Impact of pre-operative regular physical activity on balance control compensation after vestibular schwannoma surgery, Gait Posture 37: ((2013) ), 82–87. |
[18] | Gauden A. , Weir P. , Hawthorne G. and Kaye A. , Systematic review of quality of life in the management of vestibular schwannoma, J Clin Neurosci 18: ((2011) ), 1573–1584. |
[19] | Hassanpoor H. , Fallah A. and Raza M. , New role for astroglia in learning: Formation of muscle memory, Med Hypotheses 79: ((2012) ), 770–773. |
[20] | Herdman S.J. , Role of vestibular adaptation in vestibular rehabilitation, Otolaryngol Head Neck Surg 119: ((1998) ), 49–54. |
[21] | Herdman S.J. , Clendaniel R.A. , Mattox D.E. , Holliday M.J. and Niparko J.K. , Vestibular adaptation exercises and recovery: Acute stage after acoustic neuroma resection, Otolaryngol Head Neck Surg 113: ((1995) ), 77–87. |
[22] | Herdman S.J. , Schubert M.C. and Tusa R.J. , Strategies for balance rehabilitation: Fall risk and treatment, Ann N Y Acad Sci 942: ((2001) ), 394–412. |
[23] | Hillier S.L. and Hollohan V. , Vestibular rehabilitation for unilateral peripheral vestibular dysfunction, Cochrane Database Syst Rev ((2007) ), CD005397. |
[24] | Howland J.G. and Wang Y.T. , Synaptic plasticity in learning and memory: Stress effects in the hippocampus, Prog Brain Res 169: ((2008) ), 145–158. |
[25] | Johansson R. , System Modeling and Identification, Prentice Hall Englewood Cliffs, NJ; (1993) . |
[26] | Johansson R. and Magnusson M. , Optimal Coordination and Control of Posture and Locomotion, Math Biosci 103: ((1991) ), 203–244. |
[27] | Johansson R. , Magnusson M. and Akesson M. , Identification of human postural dynamics, IEEE Trans Biomed Eng 35: ((1988) ), 858–869. |
[28] | Jorntell H. and Hansel C. , Synaptic memories upside down: Bidirectional plasticity at cerebellar parallel fiber-Purkinje cell synapses, Neuron 52: ((2006) ), 227–238. |
[29] | Kalb A. , von Haefen C. , Sifringer M., Tegethoff A., Paeschke N., Kostova M., Feldheiser A. and Spies C.D., Acetylcholinesterase inhibitors reduce neuroinflammation and -degeneration in the cortex and hippocampus of a surgery stress rat model, PLoS One 8: ((2013) ), e62679. |
[30] | Kitahara T. , Takeda N. , Kiyama H. and Kubo T. , Molecular mechanisms of vestibular compensation in the central vestibular system–review, Acta Otolaryngol Suppl 539: ((1998) ), 19–27. |
[31] | Kristinsdottir E. , Jarnlo G.-B. and Magnusson M. , Asymmetric vestibular function in the elderly might be a significant contributor to hip fractures, Scand J Rehab Med 32: ((2000) ), 56–60. |
[32] | Lacour M. , Barthelemy J. , Borel L. , Magnan J. , Xerri C. , Chays A. and Ouaknine M. , Sensory strategies in human postural control before and after unilateral vestibular neurotomy, Exp Brain Res 115: ((1997) ), 300–310. |
[33] | Lacour M. , Roll J.P. and Appaix M. , Modifications and development of spinal reflexes in the alert baboon (Papio papio) following an unilateral vestibular neurotomy, Brain Res 113: ((1976) ), 255–269. |
[34] | Lacour M. , Xerri C. and Hugon M. , Compensation of postural reactions to fall in the vestibular neurectomized monkey. Role of the reamining labyrinthine afferences, Exp Brain Res 37: ((1979) ), 563–580. |
[35] | Lynn S.G. , Driscoll C.L. , Harner S.G. , Beatty C.W. and Atkinson E.J. , Assessment of dysequilibrium after acoustic neuroma removal, Am J Otol 20: ((1999) ), 484–494. |
[36] | Magnusson M. , Johansson R. and Wiklund J. , Galvanically induced body sway in the anterior-posterior plane, Acta Otolaryngol 110: ((1990) ), 11–17. |
[37] | Magnusson M. , Kahlon B. , Karlberg M. , Lindberg S. , Siesjo P. and Tjernstrom F. , Vestibular “PREHAB”, Ann N Y Acad Sci 1164: ((2009) ), 257–262. |
[38] | Magnusson M. and Padoan S. , Delayed onset of ototoxic effects of gentamicin in treatment of Meniere’s disease. Rationale for extremely low-dose therapy, Acta Otolaryngol 111: ((1991) ), 671–676. |
[39] | McCabe B.F. and Ryu J.H. , Experiments on vestibular compensation, Laryngoscope 79: ((1969) ), 1728–1736. |
[40] | Nadel and Bohbot, Consolidation of memory, Hippocampus 11: ((2001) ), 56–60. |
[41] | Nashner L.M. , Black F.O. and Wall C. 3rd , Adaptation to altered support and visual conditions during stance: Patients with vestibular deficits, J Neurosci 2: ((1982) ), 536–544. |
[42] | Parietti-Winkler C. , Gauchard G.C. , Simon C. and Perrin P.P. , Sensorimotor postural rearrangement after unilateral vestibular deafferentation in patients with acoustic neuroma, Neurosci Res 55: ((2006) ), 171–181. |
[43] | Parietti-Winkler C. , Gauchard G.C. , Simon C. and Perrin P.P. , Visual sensorial preference delays balance control compensation after vestibular schwannoma surgery, J Neurol Neurosurg Psychiatry ((2008) ). |
[44] | Patel M. , Gomez S. , Berg S. , Almbladh P. , Lindblad J. , Petersen H. , Magnusson M. , Johansson R. and Fransson P.A. , Effects of 24-h and 36-h sleep deprivation on human postural control and adaptation, Exp Brain Res 185: ((2008) ), 165–173. |
[45] | Peterka R.J. , Sensorimotor integration in human postural control, J Neurophysiol 88: ((2002) ), 1097–1118. |
[46] | Petersen H. , Magnusson M. , Fransson P.A. and Johansson R. , Vestibular disturbance at frequencies above 1Hz affects human postural control, Acta Otolaryngol 114: ((1994) ), 225–230. |
[47] | Manolakis D.G., Proakis J.G., Introduction to Digital Signal Processing, Macmillan, New York; (1989) . |
[48] | Reiner M. , Rozenkurt R. and Barnea A. , Better than sleep: Theta neurofeedback training accelerates memory consolidation, Biol Psychol ((2013) ). |
[49] | Rosczyk H.A. , Sparkman N.L. and Johnson R.W. , Neuroinflammation and cognitive function in aged mice following minor surgery, Exp Gerontol 43: ((2008) ), 840–846. |
[50] | Sadeghi S.G. , Minor L.B. and Cullen K.E. , Neural correlates of motor learning in the vestibulo-ocular reflex: Dynamic regulation of multimodal integration in the macaque vestibular system, J Neurosci 30: ((2010) ), 10158–10168. |
[51] | Saman Y. , Bamiou D.E. , Gleeson M. and Dutia M.B. , Interactions between Stress and Vestibular Compensation - A Review, Front Neurol 3: ((2012) ), 116. |
[52] | Stickgold R. , Sleep-dependent memory consolidation, Nature 437: ((2005) ), 1272–1278. |
[53] | Strupp M. , Arbusow V. , Maag K.P. , Gall C. and Brandt T. , Vestibular exercises improve central vestibulospinal compensation after vestibular neuritis, Neurology 51: ((1998) ), 838–844. |
[54] | Tjernstrom F. , Fransson P.A. , Hafstrom A. and Magnusson M. , Adaptation of postural control to perturbations–a process that initiates long-term motor memory, Gait Posture 15: ((2002) ), 75–82. |
[55] | Tjernstrom F. , Fransson P.A. , Kahlon B. , Karlberg M. , Lindberg S. , Siesjo P. and Magnusson M. , Vestibular PREHAB and gentamicin before schwannoma surgery may improve long-term postural function, J Neurol Neurosurg Psychiatry 80: ((2009) ), 1254–1260. |
[56] | Tjernstrom F. , Fransson P.A. , Patel M. and Magnusson M. , Postural control and adaptation are influenced by preceding postural challenges, Exp Brain Res 202: ((2010) ), 613–621. |
[57] | Wilson C.J. , Finch C.E. and Cohen H.J. , Cytokines and cognition–the case for a head-to-toe inflammatory paradigm, J Am Geriatr Soc 50: ((2002) ), 2041–2056. |
[58] | Yamanaka T. , Him A. , Cameron S.A. and Dutia M.B. , Rapid compensatory changes in GABA receptor efficacy in rat vestibular neurones after unilateral labyrinthectomy, J Physiol 523: (Pt 2) ((2000) ), 413–424. |