A scoping review on smart mobile devices and physical strain
Abstract
BACKGROUND:
Smart mobile devices gain increasing importance at work. Integrating these smart mobile devices into the workplace creates new opportunities and challenges for occupational health and safety.
OBJECTIVES:
Therefore the aim of the following scoping review was to identify ergonomic challenges with the use of smart mobile devices at work with respect to physical problems.
METHOD:
A review of 36 papers based on literature including January 2016 was conducted.
RESULTS:
Biomechanical measures in the reviewed studies demonstrated i.e., head flexion angles exceeding 20° in 20 out of 26 different conditions described. Furthermore, laterally deviated wrists were frequently noted and thumb and finger flexor muscle activities generally greater than 5% MVC were reported.
CONCLUSION:
The reviewed literature indicated an elevated biomechanical risk, especially for the neck, the wrists and thumb. This was due to poor posture, ongoing and intermitted muscle tension, and/or repetitive movements. Papers addressing specific risks for smartphone and tablet use in different work environments are scarce. As the technology, as well as the use of smart mobile devices is rapidly changing, further research, especially for prolonged periods in the workplace is needed.
1Introduction
Mobile smart technologies like tablet-pcs, smartphones, smartwatches and different variants of smart glasses, are on the rise in everybody’s life [1]. Data for Germany, presented by Bitkom [2], indicate a rise of smartphone usage from 36 percent in 2012 to 65 percent in 2015. Over the same period the use of tablets tripled from 13 percent to 40 percent.
Together with an increased availability of wireless internet, as well as new technological trends, information can be retrieved in real-time from nearly everywhere allowing for new ways of working [3, 4]. Thus smartphones, tablets and other smart devices are not only used for private purposes, but in the office and field, either in addition to, or as replacements for laptop or desktop computers [3]. In the context of industry, they are also gaining increasing importance in logistics and production [5, 6].
Integrating these smart mobile devices into the workplace creates new opportunities and challenges for occupational health and safety. Already existing recommendations for workstations can be only partly transferred, because of the mobility provided by the new portable computing devices. For example, touchscreens and especially virtual keyboards are without haptic feedback with respect to the finger positions. Thus continuous visual feedback is needed for operation which in turn affects the whole body posture. Against typical ergonomic recommendations, depending on the positioning of the device, postures with heavily bended necks arise, sometimes for prolonged timespans [7, 8]. Also new facets of man-machine interaction evolve. With the small touchscreens, thumbs can play a more prominent role in operating the device through multi-touch gestures. There is a growing concern about detrimental impact on health associated with (extensive) use with respect to musculoskeletal disorders [1, 9].
In parallel to the growing use in the population, scientific research concerning effects of smart devices on the user has expanded explosively in the last few years. Therefore the aim of the following review (based on literature up to January 2016) was to identify ergonomic challenges associated with the use of smart mobile devices at work with respect to physical problems.
2Literature search/method
The search string for the conducted scoping review contained three string components: the smart devices, the physical effect, and a set to narrow the search with respect to context variables and population.
Smart mobile devices included, according to Kamp et al. [3], smartphones, mobile phones, tablets, e-readers as well as smartwatches of various types. Although laptops or smart glasses also are mobile computing technology, these were excluded because of the specifically different handling. Extensive research on physical strain while using head-mounted displays and smart glasses can be found, for example, in [10, 11], or [12].
With regards to the physical effects, the research ran for biomechanical parameters (posture, muscle activity), health complaints (pain, fatigue, discomfort, musculoskeletal disorders) plus aspects of motor performance.
The search was temporally limited to publications following the year 2007 up to January 2016, as the first iPhone was released in 2007. Not included were papers focusing on effects of electromagnetic radiation. Similarly, despite the use of smart devices, research with the focus on therapeutic outcome or effectivity of a specific health app was excluded. The search was restricted to papers in English and German with a strictly adult population, not including children.
The search using the final string was conducted on PubMed, EBSCOHOST, and Web of Science complemented through a manual search based on cross-references.
3Result
After eliminating duplicates, 465 records were screened based on title, abstract and keywords (see Fig. 1). Validation studies, theses, presentations of new or modified methods, as well as references not reporting concrete empiric results were excluded from further examination.
Fig.1
The review process.
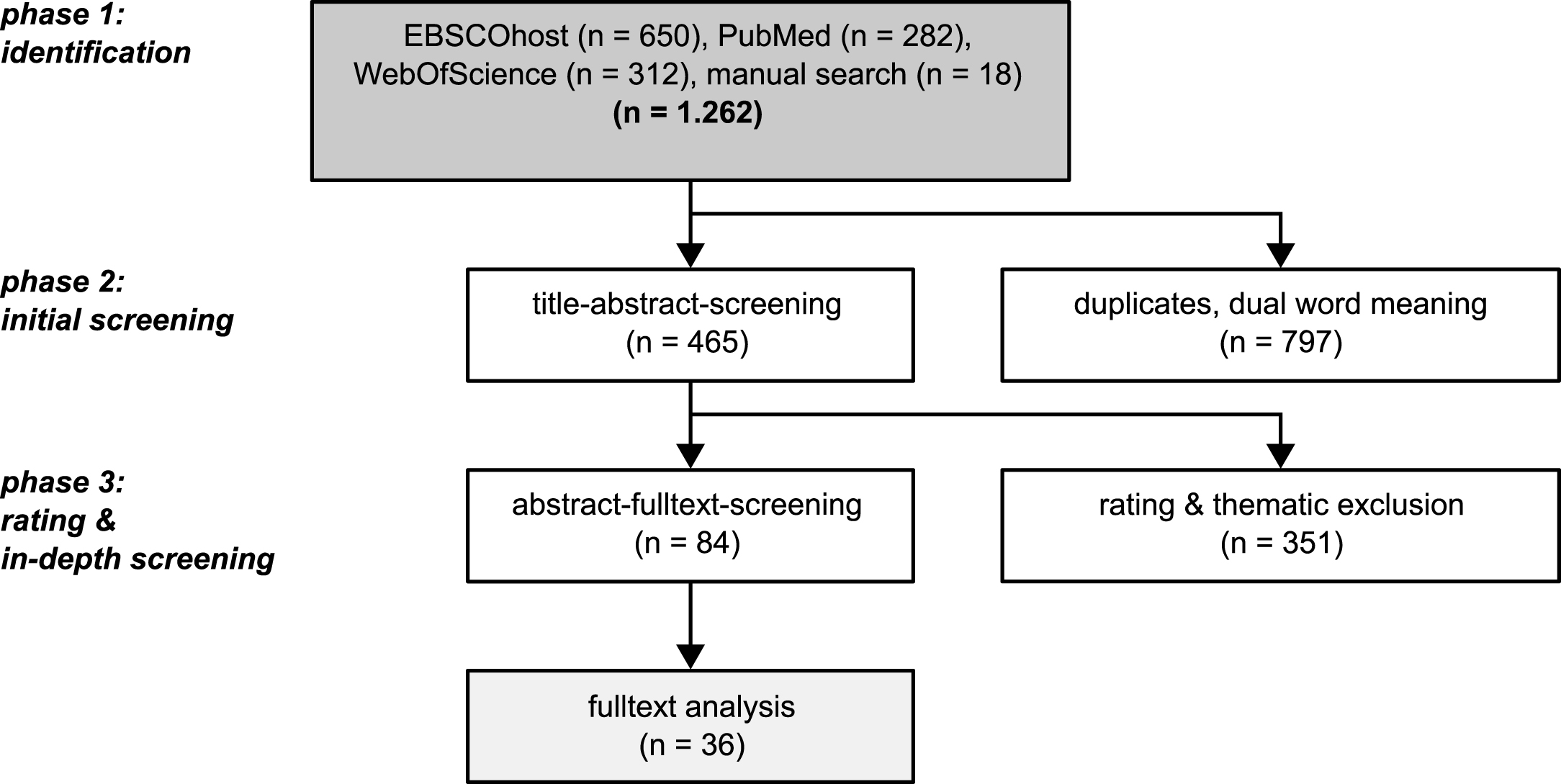
Subsequently 84 studies were assessed for eligibility on a full-text basis. Reasons for excluding full-text articles were a language other than English or German, data collection before 2004 because of the technological changes, insufficient study design or a theoretical article. Since most articles dated from 2015, reviews were equally not integrated in the further analysis.
36 studies were finally included in the subsequent quantitative syntheses. Two studies only addressed the use of smart devices in a specific work context [13, 14]. Most studies focused on biomechanical parameters.
Below results concerning the head/neck, the upper back as well as the shoulders and upper arms are reported first. Results are given starting with postural metrics, followed by muscle activities and health complaints. This is continued with results for wrists and hands. The results are concluded with clinical studies specifically addressing effects of smart device use on the thumb.
3.1Postural metrics for neck and shoulders
The postural metrics are reported in relation to a neutral zero posture with a straight back. Also the head and neck are not bent or twisted, with eyes looking straight ahead. The upper and lower arms are located sideways of the body. In order to match results between studies, other specifications e.g. the Frankfurt plane were converted accordingly.
These study results were assessed with respect to the potential for musculoskeletal disorders in reference to the Rapid Upper Limb Assessment (RULA) by McAtamney and Corlett [15] which is widely used in different ergonomics settings. In RULA, a higher score signifies greater levels of risk. So a head/neck flexion between 0° to 10° results in the lowest risk assessment. A neck flexed forward between 11°–20° gets the next highest single score and a bend above 20° scores highest. In addition to RULA, where shown separately, head tilt was compared to the comfort zone (0°–20° of neutral) specified in DIN 9241-303.
Gold et al. [16] observed people using mobile phones in different public places. They stated that 91% operated their phones with a neck flexion above 10° (thus scoring in the first non-neutral RULA category). Using a goniometer in experimental settings, three research groups [17–19] reported necks flexed between 20° to 40°. In view of RULA (second risk level) as well as the comfort zone described DIN 9241-303 these are to be regarded as critical. Ko et al. [20] measured the head tilt, depending on four different smartphone positions, while seated. If the phone was kept at eye level or in the lap, with the arms supported on the thighs and the trunk inclined, the neck was held without much flexion. Despite a neck flexion angle around zero the postures were not described as unproblematic because auf the trunk or the elbow flexion involved. For two other variations in smartphone position, Ko et al. [20] reported neck flexion angles around 20°. Using mobile phones with keypads, Gustafsson et al. [21] detected a flexion below 20° only for every seventh participant. Also participants reporting trouble in particular bent their neck over 40° whilst operating the phones.
Similarly all tablet users observed by Stawarz und Benedyk [14] showed a combination of flexed necks and trunks while working in the office, leading to raised RULA scores, as assessed by the researchers. The authors emphasized additional detrimental effects on postures due to tilted tablets in order to avoid glare and reflection on the glossy touchscreen surface. In experimental studies using tablets [17, 19, 22–24] intense flexions above 20°, partly beyond 40°, were uniformly reported. Three research groups displayed head and neck flexion angles separately. Next to a head flexed forward for more than 20° [23, 24], up to above 40° [22], neck flexions between 10° and 20° were found. In these positions the weight of the head leads to up to five times higher gravity loads on the neck and shoulder muscles than in the neutral erected posture [22]. Vasavada et al. [22] checked the external derived angles against radiographs recorded in parallel. The head flexion angle measured via radiogram lay at 30° and correlated highly with the external one (r = 0.98). The neck (r = 0.79) and a combined head-neck angle (r = 0.50) correlated a bit less. The combined head-neck angle seemed especially vulnerable to distortion, because of opposite angle directions of the involved joints. All in all, explicit neck angles were reported for 26 different conditions that could be assessed according to RULA, most of which were categorized in the highest single score (see Fig. 2).
Fig.2
Frequency distribution of explicit neck flexions reported in 9 different papers assessed according to the Rapid Upper Limb Assessment (RULA).
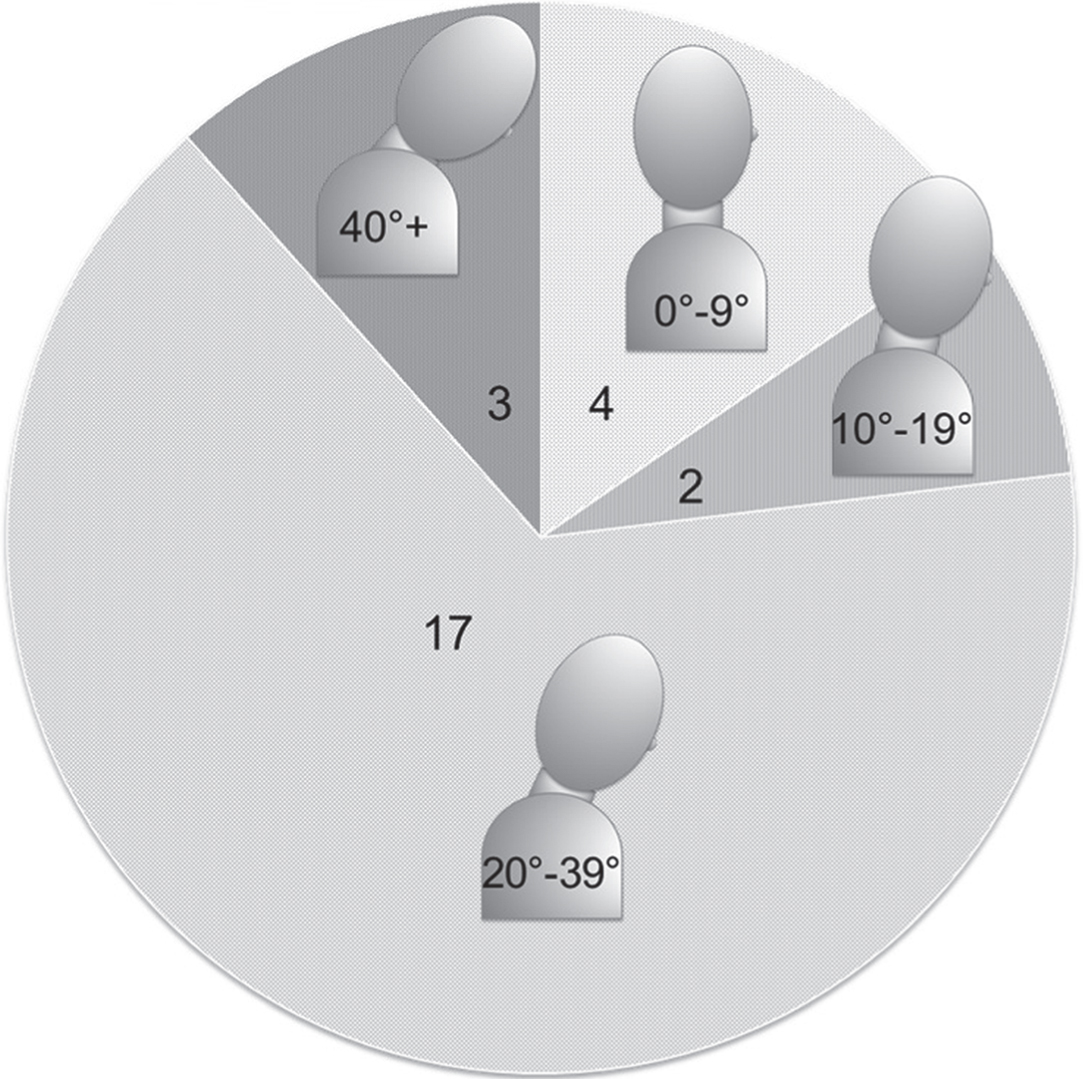
In addition to bent necks, Gold et al. [16] noticed protracted shoulders in more than a quarter of the smartphone users observed (more prominent in men). Young et al. [25] discovered shoulder elevation of about 6°, especially if a tablet was deposited on a table while texting. Participants in the Stawarz and Benedyk field study [14] also used their tablets with elevated shoulders, at the same time keeping the elbows in the air, and ignoring available armrests. The latter was equally noted in the study by Bachynskyi et al. [26].
3.2Muscle activity in the neck, shoulders, and upper back
Apart from specific joint angles, most studies analyzed the muscle activity shown while using smart devices, as an objective parameter for physical strain. Ongoing and intermitted muscle tension leads to physiological fatigue. Because of muscle activities holding only a small amount of risk for musculoskeletal disorders, some different thresholds can be found in the literature. Jørgensen et al. [27] found no muscle fatigue after working for an hour with a muscle activity of 10% as the maximum voluntary muscle contractions (MVC). Whereas Jonsson [28] advised to fall short of 5% MVC, in order to avoid musculoskeletal disorders, and subjects in studies of Sjøgaard et al. [29] reported even muscle activities below 5% MVC as tiring after an hour. Regarding muscle activities in the neck and shoulders in particular, Aarås [30, 31] indicated a reduced risk for musculoskeletal disorders for every portion of 1% MVC reduction for a given time. The following muscle activities concerning the findings for the 50th percentile reported are matched to the 5% MVC corresponding to Jonsson. Unfortunately according to Fountain [32] there are no correlations between recorded muscle activities and joint angles as used by RULA. Thus activities in specific muscles cannot be transferred to exact joint angles.
While standing participants texted on a smartphone or tablet, Ning et al. [19] measured muscle activities on the left of the neck of more than 5% MVC and more than 10% MVC on the right. Equally Xie et al. [33] reported activities in the neck muscles of more than 10% MVC in participants’ texting on a smartphone while seated.
Depending on the handling, Lee et al. [34] reported muscle activity in the upper back exceeding 5% MVC while using both hands for texting and more than 10% while texting one-handed. As an additional indicator of muscle fatigue, they found a drop of the pressure induced pain threshold after smartphone usage, especially for one-handed texting. In contrast, Gustafsson et al. [35] reported muscle activity above 5% MVC in the upper back for texting on a mobile phone only while standing. In all seated conditions, like in the studies of Kietrys et al. [17] (smartphone, mini-tablet, and tablet) and Young et al. [25] (tablet) the activities found lay below 5% MVC. Equally for three out of four smartphone positions, Ko et al. [20] reported muscle activities lower than 5% MVC. However, holding the phone at about eye level while texting resulted in activities above 6% MVC. And whereas Xie et al. [33] detected activities between 2 to 3% MVC only in a control group, subjects reporting pain in the neck and/or shoulders showed muscle activities above the 5% MVC threshold. For tablet-pcs muscle activities in the upper back above 5% MVC were only detected, if the devices were to be held unsupported [24, 36].
As for the shoulders, in a study by Knight and Barber [37], holding an arm-mounted smart device at breast height in a position mimicking reading a watch, resulted in muscle activities in the frontal shoulder exceeding 20% MVC and above 10% MVC in the lateral. Magnitudes greater than 5% MVC in the frontal shoulder while holding a tablet in a frontal central position in the non-dominant hand were reported by Lozano et al. [38]. They further discovered an influence of multi-touch gestures in the interacting side. Depending on the gesture, they found activities in the (dominant) frontal shoulder muscle ranging between a bit over 5 up to over 9% MVC. Young et al. [25] also indicated more than 5% MVC in the frontal shoulder (interacting arm) for texting, while the tablet was rested on a table.
Muscle activities above 5% MVC in the upper arm, caused by holding a device are reported by [36–38]. Ko et al. [20] found a significant effect of position equivalent to the one for the upper back: The higher a smartphone was placed towards the face, the more activity above 5% MVC they recorded in the upper arm.
3.3Discomfort in the neck and shoulders
In the study of Lin und Peper [39], ten out of twelve participants indicated pain in the neck and/or the hand after texting on a smartphone. Likewise in a survey by Berolo et al. [40] 68% of the respondents reported feeling pain in the neck and 62% in the upper back in connection with the use of diverse (smart) mobile devices. In an investigation by Bretschneider-Hagemes [13] a group of technical service staff using smart mobile devices on a regular basis while working, reported pain in the upper back (89% vs. 64%) and/or the neck (89% vs. 55%) significantly more often in comparison to technical service staff not using mobile IT. Furthermore 52% of the interviewees indicated having trouble in the right shoulder and 46% in the left.
3.4Postural metrics for the wrists
As before, all joint angles are referred to in reference to a neutral zero position of the hand. For this, the wrist is unbent and in line with the lower arm while the thumb rests alongside, close to the palm.
With respect to the wrist, every deviation from this neutral position between 15° flexion and extension is assessed as a risk factor for musculoskeletal disorders (MSD) according to RULA [15]. If the wrist is flexed or extended above 15°, it scores as an even greater risk for MSD. If the wrist is visibly bent away from the middle (ulnar or radial deviation), or the wrist is twisted (pronation or supination), the posture score is increased by an extra point respectively. In the modified version (mRula) for evaluating computer work developed by Lueder [41], the wrists score an additional risk assessment point, if they are held above the keyboard flexed less than 15°, or two points if flexed 15° or more. In contrast, Hoehne-Hückstädt et al. [42] following Drury [43] does not classify a wrists extension as a risk until it exceeds 25° and a wrist flexion of 20°. Ulnar or radial deviations have to exceed 10° before being measured as not neutral. Twisting the hand is seen as a strain for a pronation of 20° and a supination of 30° and more. The following assessment of the reported joint angles is based on RULA with more extreme joint angles according to Hoehne-Hückstädt et al. [42] referred to separately.
Gold et al. [16] observed 90% typing with a non-neutral wrist in which they saw a severe risk for disorders of the arm and hand, especially for carpal-tunnel-syndrome. Unfortunately the amount of deviation of the wrist (flexion/extension, radial/ulnar deviation) was not reported separately. Postural analysis for tablet use by Stawarz and Benedyk [14] revealed wrists being extended and (due to the narrow virtual keyboard) deviated sideways. Also, participants kept their fingers floating above the touch-screen, even when not typing, keeping the wrists permanently extended.
Both Kietrys et al. [17] using a 3.5″ smartphone, and Ko et al. [20] for a 4″ smartphone, found extensions below 15° and lateral deviation not reaching 10°. Equally, Pereira et al. [24] reported wrist extensions below 15° for a small 5.3″ tablet. But they also showed ulnar deviations over 25° from neutral when using the 5.3″ device. Using a 3.5″ device in a single-handed tapping task between 2 keys out of 12 emulated keys, Trudeau et al. [44] reported wrist angles between an extension missing the threshold of 25° by just 1°, if a key in the upper left corner was tapped, and a flexion of 5° if subjects had to tap on the bottom right key.
Using a 7″ tablet Trudeau et al. [45] measured wrist extensions of less than 15°, if a split keyboard layout was used. By contrast, typing with a standard keyboard layout resulted in a wrist extension above 15° (but below the threshold of 25° according to [42]. Pereira et al. [24], Kietrys et al. [17] and Young et al. [25] reported wrists being extended from a 15° minimum up to more than 25° for tablets 7″ and larger. The greatest extensions from neutral were observed while the subjects were texting.
Details given on lateral deviations while using the tablet were somewhat mixed. Young et al. [25] only observed deviations smaller than 10°. As with the 5.3″ tablet, in the 7″ condition Pereira et al. [24] measured ulnar deviations more than 25° in the hand holding the device. With respect to a 10″ tablet they, as well as Trudeau et al. [45] described wrist angles above 10° but below 25° lateral deviation. Kietrys et al. [17] picture an opposite effect, with deviations increasing from just less than 25° for a mini-tablet, to above this threshold for the biggest tablet-pc, if participants were free to choose tablet position and way of texting.
3.5Thumb postures
Compared to using an external physical keyboard or mouse, the operating of smart devices is changed, due to the need to hold the device while using a touch-screen. Especially the thumbs, which cannot reach the whole touch-screen equally well, are used a lot in tapping and texting.
Accordingly, all participants in a study by Otten et al. [46] concerning the functional range of the thumb avoided the bottom right and top left corner of a 4″ touch device. Adolescents displayed a slightly larger range of reach, by holding the device more loosely. Using 3.5″ touch devices, Park and Han [47, 48] observed that smaller touch keys, and those positioned at the bottom of the device were operated with the tip of very steeply held thumbs. This is confirmed by results from Xiong und Muraki [49] investigating the relationship between age, thumb length and screen size (3.5″ vs. 5″). Subjects sporting longer thumbs needed to flex the base as well as the upper joint of the thumb very hard, in order to reach the bottom right corner. Younger subjects in particular, operated the phone with the tip of a vertically flexed thumb. Similar to the adolescent in the research by Park and Han [47], participants wrapped their fingers around the phone and shifted the screen towards and away from the thumb in order to cover more space. Especially for the 5″ Device this controlled shift was easier with larger hands. Participants with smaller hands displayed a larger extension of the thumb near the maximal range instead. Comparable effects for larger devices are found by Bergstrom-Lehtovirta and Oulasvirta [50]. Depending on display size, the upper left corner could only be reached by extreme stretching of the thumb, and/or shifting the device in the hand which required a deviation of the wrists.
Gustafsson et al. [35] reported that subjects held their own phone rather loosely with the thumb lateral (radial) abducted/extended for more than 20°. By comparison the phones provided by the experimenters were held more closely. Especially, if subjects texted while standing, the radial abduction was less than 10°. This was somewhat compensated for with a greater opposition of the thumb over the palm of nearly 17°. When texting while seated, the opposition did not reach 13°. Irrespective of body posture, the thumb was in opposition and reduction about eight times a second as well as eleven times per second radially abducted and adducted while texting. For one-handed use of a 3.5″ smartphone Trudeau et al. [44] reported various thumb angles as a function of key position on the phone. The upper thumb (interphalangeal) joint was least flexed with about 17°, combined with a palmar abduction in the basal (carpometacarpal) thumb joint of 30° and radial extension of 10° for a key position in the top left corner. If the keys on the bottom row of the device had to be tapped, the flexion for the interphalangeal joint ranged from 30° for the left key, up to 58° for the right corner while the carpometacarpal radial flexed between 12° to 8°. Comparing different virtual keyboards for tablets, Trudeau et al. [45] observed only small angles below 10° in the carpometacarpal and middle (metacarpophalangeal) thumb joints. For the interphalangeal joint however, they reported flexions between 28° to 44°, depending on layout conditions. To date, there are no assessment criteria available comparable to RULA with respect to the three thumb joints. Based on a categorization by Drury [43] concerning other joints for risk exposure, and data provided by Barakat et al. [51] for thumb range of motion, some risk assessment could be derived. A flexion of the upper thumb as reported by Trudeau et al. [44, 45] equals moderate exposure ranging between 20–39° up to severe exposure for the angles above 40°.
3.6Muscle activities in the wrists, thumbs, and fingers
As in 3.2 the EMG described for different hand muscles are viewed in contrast to the 5% MVC for the 50th percentile corresponding to Jonsson [28].
In concert with the wrist angles reported by Kietrys et al. [17] EMG for wrists extension (extensor carpi radialis, ECR) laid below 5% MVC for phone use, and exceeded 5% MVC while using a mini-tablet or tablet. Young et al. [25] described task-oriented muscle activities in ECR of about 9% MVC and more, if the subjects used the virtual keyboard with the tablet positioned on a table or the lap. If the tablet was used for browsing or playing games instead the ECR activities lay well under 5% MVC. Lozano et al. [38] stated activities in the wrist extensor muscle between 8 up to 16% MVC for varied multi-touch gestures. They noted that the found average activity was higher than maximum activities reported for computer mouse use.
For holding a tablet, Plegge and Alexander [36], measured both the wrist extensor (ECR) and the flexor muscles (flexor carpi radialis and ulnaris) at below 5% MVC. Pereira et al. [24] however reported more than 5% activity in the FCR and the ECR for holding a tablet.
For the hand, activity can be acquired for eight muscles in the thumb and additional finger muscles. Due to their near proximity, it is not yet possible to register them all in parallel. Different researchers investigated varying thumb and finger muscles, therefore the results cannot all be matched. According to Jonsson et al. [52] EMG and goniometry data of the hand represent different aspects of operational stress which cannot be substituted for each other. Based on the low association between different EMG and joint angles of the thumb and fingers as reported by [52], it must be noted, that awkward joint angles cannot be eliminated due to low muscle activities obtained and vice versa.
Starting with the finger muscles involved, Plegge und Alexander [36] found muscle activity lower than 5% MVC in the flexed fingers (flexor digitorum superficialis, FDS) for holding a tablet, as also did Xie et al. [33] for FDS and the muscles extending the fingers (extensor digitorum, ED) for smartphones.
In contrast, holding a mobile phone or smartphone in the cupped hand in the study by Kietrys et al. [17] led to muscle activities above 5% MVC in the FDS. Equally Ko et al. [20] discovered EMG higher than 5% MVC in the FDS as well as in ED while holding a phone.
Using different sizes of tablets, [17] and [24] measured muscle activity clearly higher than 5% MVC in the FDS; more so with the larger tablets. The larger tablets were grasped more tightly because of the greater weight and center of gravity being further from the holding hand. Consistently, subjects in the study by Pereira et al. [24] estimated being able to hold the 10″ tablet for only about 15 additional minutes in contrast to 26 and 36 minutes for the 5.3″ and 7″ tablets. Kietrys et al. [17] regarded the different way of use of 10″ tablets as an additional factor for the higher EMG found in the finger flexor as these were more often placed on the lap with all ten fingers being used to type.
Gustafsson et al. [21] confirmed a considerable effect of texting speed. Though EMG for flexing and extending the fingers lay slightly below 5% MVC in most conditions, it rose above the threshold when texting with high speed. Holding the phone in one hand and texting with the same also led to muscle activity of more than 5% MVC. Both findings, Gustafsson et al. [21] noted, were due to the need to stabilize the phone. Xiong and Muraki [53] described correlations between thumb posture and EMG in the index finger. The more the tapping induced a vertical posture of the thumb, the more activity was registered in the index finger muscles. Analog to [21] they attributed this to the significance of the index finger for stabilizing the smartphone.
Gustafsson et al. [21] stated activities in the muscles abducting the thumb vertical to the palm (musculi abductor pollicis longus, APL & brevis, APB) over 5% MVC. High texting frequencies resulted in even higher activity in both thumb muscles and one-handed texting in particular, caused more than twice the movement repetitions. Similarly, Kietrys et al. [17] collected APB activities greater than 5% MVC using smartphones as well as mobile phones with a keypad. Concerning 7″ as well as 10″ tablets [17] recorded muscle activities in the APB beyond 20% MVC. The researchers attributed this to the larger thumb radius needed in the tablet conditions. In contrast, Xie et al. [33] obtained APB activity lower than 5% MVC, while subjects texted on a smartphone.
Ko et al. [20] found EMG in the muscle flexing the thumb at the carpometacarpal and the metacarpophalangeal joint (flexor pollicis brevis, FPB) of on average more than 5% MVC. Especially with one-handed texting, they reported activities in the FPB exceeding 9% MVC, which they regarded as a serious risk for musculoskeletal disorders and repetitive strain injuries.
Significant differences between one-handed and two-handed texting on a smartphone were affirmed by Lee et al. [54]. They reported EMG in the muscle extending the tip of the thumb at the interphalangeal joint (extensor pollicis longus, EPL) as well as the APL above 10% MVC. Concerning one-handed texting they measured muscle activities of 20% MVC.
3.7Discomfort and disorders in the hand
Sambrooks and Wilkinson [55] explored, amongst other parameters, fatigue ratings after using touch-screen devices of three sizes (4.7″, 7″, 10″) for seven interactive tasks each. For most of the tasks, the devices had to be held unsuspended. Independent of task, the lightest device received the lowest fatigue score and the 10″ device the highest.
Moderate to severe pain in the base of the right thumb associated with a daily use of mobile devices for more than 140 minutes were reported by Berolo et al. [40].
Adverse effects on the hand, due to extensive use of mobile phones for texting, are stated by Sharan et al. [56, 57]. A post hoc analysis of 70 medical diagnosis charts revealed tendinosis of the EPL and myofascial pain syndrome effecting the ED and 1st interosseous (abducting the index finger and assisting in thumb adduction) for all included subjects. Furthermore, associated co-morbidities, such as myofascial pain syndrome of the neck and upper back were depicted.
In a pilot study Gold et al. [58] searched for increased biomarkers in the serum of high volume texters. Their results suggested that high volume texting may be a risk for thumb tendinopathy. Equally, Ali et al. [59] and Eapen et al. [60] associated intense texting with a heightened risk for musculoskeletal disorders of the thumb and wrist. Inal et al. [61] demonstrated somewhat enlarged median nerves in high frequent smartphone users, due to the repetitive wrist flexion and extension during smartphone use. As an enlarged median nerve is associated with carpal tunnel syndrome [61] it could be posited, that excessive smartphone use might lead to carpal tunnel syndrome.
4Discussion
The physical risks identified for the use of smart mobile devices, are basically similar to those of traditional computer technologies. Musculoskeletal complaints arise as a result of non-neutral, partly static posture, frequent repetitive motion and high muscle activity. Which specific physical issues actually occur, strongly depends on the task at hand, with self-imposed postures and positioning not leading to significantly less strain than predetermined ones [22].
Unless input method and display are separated via external (and less mobile) accessories, a change of one physical parameter affects all others as well. With the devices held at head height, there is little to no strain on the neck. In this case however, the upper back, arm and wrist bear the burden. Alternatively, smartphones and tablets are placed low on the lap. While resulting in a low activity in the back and arms, this leads to a strongly bent neck.
Arm and back rests can help to prevent additional muscle activity in the entire upper part of the body triggered by holding and balancing the devices. The suitable height for these rests results from the intended interaction. If smartphones and tablets are to be held unsupported for a longer time, smaller and lighter variants should be favored. Beside the reduced required muscle activity, it is easier to use the entire touchscreen of small devices, particularly for persons with small hands and/or short thumbs. A device of 3–3.5″ can be simultaneously held and tilted in the hand better than a larger one, therefore enabling the thumb to reach across the touchscreen. Participants in a study by [55] consistently indicated less fatigue and higher preferences for a small smartphone, if the device had to be held unsupported for a longer time. If the visible screen area was crucial to the task execution, they favored tablets.
Sales figures, however, indicate a trend towards ever larger units: The proportion of 3–3.5″ devices sold in Germany has dropped from 18 percent in 2013 to 4 percent in 2015. Likewise sales of 4–4.5″ touchscreens have shrunk from 44 percent to 25 percent, whereas 5–5.5″ displays for smartphones gained accordingly [2]. The majority of the studies referred to had used smaller smartphones ranging from 3.5 to 4.8″. The smallest tablet of 5.3″ used in the study of [24] equates the smartphone size currently popular. Thus boundaries between the different mobile devices become blurred.
While trying to prevent glare and reflections, users sometimes adopted a range of unbeneficial positions [14]. Thus, special displays with high luminance contrast or as modification to existing installed displays, reflection repressive foils may not only prevent glare and reflections but avoid self-imposed bad postures.
The high physical strain on the thumbs, induced through texting and multitouch gestures was unknown so far. For in classic typing, thumbs were used for the space bar only. The results presented in various studies lead to the conclusion, that the fast and repetitive one-handed movements during texting increase the risk for tenosynovitis especially of the thumbs. In order to reduce the demands on the thumb, medium tempo and two-handed use of smartphones and tablets is recommended especially for texting longer passages.
Then again, using both hands on the touchscreens of smartphones and tablets, involves not only the thumbs, but also wrists and shoulders. The smaller the touchscreen, the more closely both hands have to be placed together. Especially while using a virtual keyboard, the shoulders are hunched forward and the wrists are deviated outwards. Using mobile touch devices brings physical strain for both shoulders and wrists. Overall the results reported, contingent on device position can be assessed according to RULA at least at action level 3, indicating that investigation and changes are required soon.
Specifically texting for longer periods should be avoided, along with frequent breaks to rest the hands. Divided virtual keyboards, using both sides of the display instead of the bottom row, can help to avoid extensive stretching of wrists and thumbs. Admittedly thus divided keyboards need some time adjusting for the unfamiliar key positions. External keyboards and accessories may help to prevent strain, if the devices are used for longer time periods. Although with respect to the study of Stawarz und Benedyk [14], it must be pointed out, that most users are likely to reject external accessories because these limit the mobility of the devices.
Whether smartphones or tablet-pcs are more suitable, or perhaps a laptop is the better alternative, depends on the specific tasks intended. For large quantities of Data, a bigger display, and therefore a tablet, seems more adequate. Likewise, due to the larger virtual keyboard, a tablet is easier on the thumbs while texting for longer periods. But if the device has to be carried and held unsupported for longer time spans, smaller smartphones might be the device of choice. So device weight and display size need to be balanced, according to the predominant use within the specific working conditions. Wherever possible, the positioning of the used devices should be varied to spread the physical strain evenly. Individually adjustable display settings and/or stands may encourage alternative postures (e. g. eye to display distance) as well as a wider range of movements while working with the devices.
5Conclusion and future research
Overall the results reported in the reviewed literature indicated an elevated biomechanical risk, especially for the neck, wrists and thumbs, due to poor posture, ongoing and intermitted muscle tension, and/or repetitive movements. Especially repetitive movements involving the thumbs may induce repetitive strain injury.
As duration and intensity of use are crucial factors, particularly with regard to biomechanical workload, and in light of the postures and muscle activities induced by the unity of display and input, smart mobile devices are recommended for short-termed use intermitted with frequent breaks and/or work activities.
With only two exceptions, the studies referred to focused on short time usage unrelated to particular work conditions. So up to now, insights concerning specific risks, when using smartphone and tablet in different work environments, are scarce. Therefore only some basic advice for using smart mobile devices while working can be derived.
For more distinctive recommendations with respect to different working conditions and/or occupational categories further research seems necessary. Thus with regard to activities, identified tasks can be clustered and formal principles for workplace, environment, and equipment derived.
Conflict of interest
None to report.
Acknowledgments
The author extends special thanks to Dominik Bonin for discussions and insights concerning various joint angles possible and Tomas Allis for his support in preparing this manuscript.
References
[1] | Dennerlein JT . The state of ergonomics for mobile computing technology. Work: A Journal of Prevention, Assessment and Rehabilitation (2015) ;52: (2):269–77. |
[2] | Lutter T , Pentsi A , Poguntke M , Böhm K , Esser R . Zukunft der Consumer Electronics – 2015. Marktentwicklung, Schlüsseltrends, Mediennutzung, Konsumentenverhalten, Neue Technologien. Berlin: Bitkom e.V., (2015) . |
[3] | Kamp I , Van Veen SAT , Vink P . Comfortable mobile offices: Aliterature review of the ergonomic aspects of mobile device use intransportation settings. Work: A Journal of Prevention, Assessmentand Rehabilitation (2015) ;52: (2):279–87. |
[4] | Welskop-Deffaa EM . Die Gestaltung des Arbeitsschutzes in der Arbeitswelt 4.0. In: Schröder L, Urban H-J, editors. Gute Arbeit Digitale Arbeitswelt: Trends und Anforderungen. Ausg. 2016 ed Frankfurt am Main: Bund-Verl; (2016) . pp. 410. |
[5] | Mauritz N . Die vierte industrielle Revolution, ihre Auswirkungen un dwie Unternehmen diesen begegnen können - eine Literaturanalyse. In: Franken S, editor. Industrie 40 und ihre Auswirkungen auf die Arbeitswelt. Aachen: Shaker; (2015) . pp. 177. |
[6] | Adolph L . Menschengerechte Arbeit in der digitalen Arbeitswelt. Herausforderungen auf dem Weg zur guten Gestaltung. In: Schröder L, Urban H-J, editors. Gute Arbeit Digitale Arbeitswelt: Trends und Anforderungen. Ausg. 2016 ed. Frankfurt am Main: Bund-Verl; (2016) . pp. 410. |
[7] | DIN. Ergonomie der Mensch-Maschine-Interaktion - Teil 303: Anforderungen an elektronische optische Anzeigen. Deutsche Fassung EN ISO 9241-303:2011 ed. |
[8] | Masseida J , Philipp J-J , Wicher C , Jaschinski W . Verschiedene Kenngrößen für die physiologisch günstige Kopfneigung. Zeitschrift für Arbeitswissenschaft (2013) ;67: (4):207–19. |
[9] | Honan M . Mobile work: Ergonomics in a rapidly changing work environment. Work: A Journal of Prevention, Assessment and Rehabilitation (2015) ;52: (2):289–301. |
[10] | Theis S , Pfendler C , Alexander T , Mertens A , Brandl C , Schlick CM . Head-Mounted Displays - Bedingungen des sicheren und beanspruchungsoptimalen Einsatzes: Physische Beanspruchung beim Einsatz von HMDs. Dortmund/Berlin/Dresden: Bundesanstalt für Arbeitsschutz und Arbeitsmedizin; (2016) . |
[11] | Wille M . Head-Mounted Displays - Bedingungen des sicheren und beanspruchungsoptimalen Einsatzes: Psychische Beanspruchung beim Einsatz von HMDs. Dortmund/Berlin/Dresden: Bundesanstalt für Arbeitsschutz und Arbeitsmedizin; (2016) . |
[12] | Grauel BM , Terhoeven JN , Wischniewski S , Kluge A . Erfassung akzeptanzrelevanter Merkmale von Datenbrillen mittels Repertory Grid Technik. Zeitschrift für Arbeitswissenschaft (2014) ;68: (4):250–6. |
[13] | Bretschneider-Hagemes M . Belastungen und Beanspruchungen beimobiler IT-gestützter Arbeit - Eind empirische Studie im Bereich mobiler, technischer Dienstleistungen. Zeitschrift für Arbeitswissenschaften (2011) ;65: (2011/3):223–33. |
[14] | Stawarz K , Benedyk R , editors. Bent necks and twisted wrists: Exploring the impact of touch-screen tablets on the posture of office workers. Proceedings of the 27th International BCS Human Computer Interaction Conference; (2013) : British Computer Society. |
[15] | McAtamney L , Corlett EN . RULA: A survey method for the investigation of work-related upper limb disorders. Applied Ergonomics (1993) ;24: (2):91–9. |
[16] | Gold JE , Driban JB , Thomas N , Chakravarty T , Channell V , Komaroff E . Postures, typing strategies, and gender differences in mobile device usage: An observational study. Applied Ergonomics (2012) ;43: (2):408–12. |
[17] | Kietrys DM , Gerg MJ , Dropkin J , Gold JE . Mobile input device type, texting style and screen size influence upper extremity and trapezius muscle activity, and cervical posture while texting. Applied Ergonomics (2015) ;50: :98–104. |
[18] | Lee S , Lee D , Park J . Effect of the cervical flexion angle during smart phone use on muscle fatigue of the cervical erector spinae and upper trapezius. Journal of Physical Therapy Science (2015) ;27: (6):1847–9. |
[19] | Ning X , Huang Y , Hu B , Nimbarte AD . Neck kinematics and muscle activity during mobile device operations. International Journal of Industrial Ergonomics (2015) ;48: :10–5. |
[20] | Ko PH , Hwang YH , Liang HW . Influence of smartphone use styles on typing performance and biomechanical exposure. Ergonomics (2015) :1–8. |
[21] | Gustafsson E , Johnson PW , Lindegård A , Hagberg M . Technique, muscle activity and kinematic differences in young adults texting on mobile phones. Ergonomics (2011) ;54: (5):477–87. |
[22] | Vasavada AN , Nevins DD , Monda SM , Hughes E , Lin DC . Gravitational demand on the neck musculature during tablet computer use. Ergonomics (2015) ;58: (6):990–1004. |
[23] | Young JG , Trudeau MB , Odell D , Marinelli K , Dennerlein JT . Touch-screen tablet user configurations and case-supported tilt affect head and neck flexion angles. Work (Reading, Mass) (2012) ;41: (1):81–91. |
[24] | Pereira A , Miller T , Huang Y-M , Odell D , Rempel D . Holding a tablet computer with one hand: Effect of tablet design features on biomechanics and subjective usability among users with small hands. Ergonomics (2013) ;56: (9):1363–75. |
[25] | Young JG , Trudeau MB , Odell D , Marinelli K , Dennerlein JT . Wrist and shoulder posture and muscle activity during touch-screen tablet use: Effects of usage configuration, tablet type, and interacting hand. Work (Reading, Mass) (2013) ;45: (1):59–71. |
[26] | Bachynskyi M , Palmas G , Oulasvirta A , Steimle J , Weinkauf T . Performance and Ergonomics of Touch Surfaces: A Comparative Study using Biomechanical Simulation. Proceedings of the 33rd Annual ACM Conference on Human Factors in Computing Systems; Seoul, Republic of Korea. 2702607: ACM; (2015) ; pp. 1817–26. |
[27] | Jørgensen K , Fallentin N , Krogh-Lund C , Jensen B . Electromyography and fatigue during prolonged, low-level static contractions. European Journal of Applied Physiology (1988) ;57: (3):316–21. |
[28] | Jonsson B . Measurement and evaluation of local muscular strain in the shoulder during constrained work. Journal of Human Ergology (1982) ;11: :73–88. |
[29] | Sjøgaard G , Kiens B , Jorgensen K , Saltin B . Intramuscular pressure, EMG and blood flow during low-level prolonged static contraction in man. Acta Physiologica Scandinavica (1986) ;128: (3):475–84. |
[30] | Aarås A . Relationship between trapezius load and the incidence of musculaskeletal illness in the neck and shoulder. International Journal of Industrial Ergonomics (1994) ;14: :341–8. |
[31] | Aarås A . Acceptable muscle load on the neck and shoulder regions assessed in relation to the incidence of musculoskeletal sick leave: Implications for human-computer interaction. International Journal of Human-Computer Interaction (1990) ;2: (1):29–39. |
[32] | Fountain LJ . Examining RULA’s postural scoring system with selected physiological and psychophysiological measures. International Journal of occupational safety and ergonomics: JOSE (2003) ;9: (4):383–92. |
[33] | Xie Y , Szeto GP , Dai J , Madeleine P . A comparison of muscle activity in using touchscreen smartphone among young people with and without chronic neck-shoulder pain. Ergonomics (2015) :1–12. |
[34] | Lee M , Hong Y , Lee S , Won J , Yang J , Park S , et al. The effects of smartphone use on upper extremity muscle activity and pain threshold. Journal of Physical Therapy Science (2015) ;27: (6):1743. |
[35] | Gustafsson E , Johnson PW , Hagberg M . Thumb postures and physical loads during mobile phone use - a comparison of young adults with and without musculoskeletal symptoms. Journal of Electromyography and Kinesiology (2010) ;20: (1):127–35. |
[36] | Plegge C , Alexander T . Untersuchung der muskulären Beanspruchung bei unterschiedlichen Schwerpunktlagen von Tablet-PCs im Gehen. Zeitschrift für Arbeitswissenschaft (2015) ;69: (3):129–36. |
[37] | Knight JF , Baber C . Assessing the physical loading of wearable computers. Applied Ergonomics (2007) ;38: (2):237–47. |
[38] | Lozano C , Jindrich D , Kahol K , editors. The impact on musculoskeletal system during multitouch tablet interactions. Proceedings of the SIGCHI Conference on Human Factors in Computing Systems, May 7-12, 2011; (2011) ; Vancouver, Canada: ACM. |
[39] | Lin I-M , Peper E . Psychophysiological patterns during cell phone text messaging: A preliminary study. Applied Psychophysiology and Biofeedback (2009) ;34: (1):53–7. |
[40] | Berolo S , Wells RP , Amick BC III . Musculoskeletal symptoms among mobile hand-held device users and their relationship to device use: A preliminary study in a Canadian university population. Applied Ergonomics (2011) ;42: (2):371–8. |
[41] | Lueder R , editor. A proposed RULA for Computer Users. Proceedings of the Ergonomics Summer Workshop; (1996) ; UC Berkeley Center for Occupational & Enviromental Health Continuing Education Program, San Francisco, August 8-9, 1996. |
[42] | Hoehne-Hückstädt UH, C., Ellegast RP, Hermanns I, Hamburger R, Ditchen D. Muskel-Skelett-Erkrankungen der oberen Extremität - Entwicklung eines Systems zur Erfassung und arbeitswissenschaftlichen Bewertung von komplexen Bewegungen der oberen Extremität bei beruflichen Tätigkeiten. BGIA-Report. Sankt Augustin: Hauptverband der gewerblichen Berufsgenossenschaften; (2007) . pp. 73–5. |
[43] | Drury CG . A biomechanical evaluation of the repetitive motion injury potential of industrial jobs. Seminars in Occupational Medicine (1987) ;2: (1):41–9. |
[44] | Trudeau MB , Young JG , Jindrich DL , Dennerlein JT . Thumb motor performance varies with thumb and wrist posture during single-handed mobile phone use. Journal of Biomechanics (2012) ;45: (14):2349–54. |
[45] | Trudeau MB , Catalano PJ , Jindrich DL , Dennerlein JT . Tablet Keyboard Configuration Affects Performance, Discomfort and Task Difficulty for Thumb Typing in a Two-Handed Grip. PloS one (2013) ;8: (6):e67525. |
[46] | Otten EW , Karn KS , Parsons KS . Defining thumb reach envelopes for handheld devices. Hum Factors (2013) ;55: (1):48–60. |
[47] | Park YS , Han SH . One-handed thumb interaction of mobile devices from the input accuracy perspective. International Journal of Industrial Ergonomics (2010) ;40: (6):746–56. |
[48] | Park YS , Han SH . Touch key design for one-handed thumb interaction with a mobile phone: Effects of touch key size and touch key location. International Journal of Industrial Ergonomics (2010) ;40: (1):68–76. |
[49] | Xiong J , Muraki S . Effects of age, thumb length and screen size on thumb movement coverage on smartphone touchscreens. International Journal of Industrial Ergonomics (2016) ;53: :140–8. |
[50] | Bergstrom-Lehtovirta J , Oulasvirta A . Modeling the functional area of the thumb on mobile touchscreen surfaces. Proceedings of the 32nd annual ACM conference on Human factors in computing systems; Toronto, Ontario, Canada. 2557354: ACM; (2014) . pp. 1991–2000. |
[51] | Barakat M , Field J , Taylor J . The range of movement of the thumb. Hand (2013) ;8: (2):179–82. |
[52] | Jonsson P , Johnson PW , Hagberg M , Forsman M . Thumb joint movement and muscular activity during mobile phone texting – A methodological study. Journal of Electromyography and Kinesiology (2011) ;21: (2):363–70. |
[53] | Xiong J , Muraki S . An ergonomics study of thumb movements on smartphone touch screen. Ergonomics (2014) ;57: (6):943–55. |
[54] | Lee S , Kang H , Shin G . Head flexion angle while using a smartphone. Ergonomics (2015) ;58: (2):220–6. |
[55] | Sambrooks L , Wilkinson B . Handheld augmented reality: Does size matter? In: Marks S, Blagojevic R, editors. Proceedings of the 16th Australasian User Interface Conference (AUIC 2015) Conferences in Research and Practice in Information Technology Series. 162. Sydney: Australian Computer Society Inc; (2015) . pp. 11–20. |
[56] | Sharan D , Ajeesh PS . Risk factors and clinical features of text message injuries. Work: A Journal of Prevention, Assessment and Rehabilitation (2012) ;41: (1, Number 1 Supplement 1):1145–8. |
[57] | Sharan D , Mohandoss M , Ranganathan R , Jose J . Musculoskeletal Disorders of the Upper Extremities Due to Extensive Usage of Hand Held Devices. Annals of Occupational and Environmental Medicine (2014) ;26: (1):1–4. |
[58] | Gold JE , Mohamed FB , Ali S , Barbe MF . Serum and MRI Biomarkers in Mobile Device Texting. Human Factors: The Journal of the Human Factors and Ergonomics Society (2014) ;56: (5):864–72. |
[59] | Ali M , Asim M , Danish SH , Ahmad F , Iqbal A , Hasan SD . Frequency of De Quervain’s tenosynovitis and its association with SMS texting. Muscles, Ligaments and Tendons Journal (2014) ;4: (1):74–8. |
[60] | Eapen C , Kumar B , Bhat AK , Venugopal A . Extensor Pollicis Longus Injury in Addition to De Quervain’s with Text Messaging on Mobile Phones. J Clin Diagn Res (2014) ;8: (11):Lc01–4. |
[61] | Inal EE , Demirci K , Çetintürk A , Akgönül M , Savaş S . Effects of smartphone overuse on hand function, pinch strength, and the median nerve. Muscle and Nerve (2015) ;52: (2):183–8. |