The effect of backpack load placement on physiological and self-reported measures of exertion
Abstract
BACKGROUND:
Backpacks are the most popular way of carrying additional weight; however, it puts the body under physical stress and may cause discomfort. It may also increase oxygen demand and energy cost. Manipulation of load placement may relieve the effects carrying a backpack has on the body.
OBJECTIVE:
This study investigated physiological and self-reported measures of exertion, movement economy and efficiency, carrying a loaded backpack in high and low load placement compared to a control condition.
METHOD:
Fifteen healthy adults were examined under three load conditions: no load, carrying a 20% of body weight in high and low load placement. Dependent variables were measured using a metabolic measurement system and participants rated their perceived exertion on a Borg scale.
RESULT:
Carrying load produced a significant increase in VO2, minute ventilation, heart rate, movement economy and overall perceived exertion in both load placements compared with the no backpack condition. However, no difference was observed between the high and low load placement conditions.
CONCLUSION:
While altering load placement did not influence physiological variables or overall exertion, participants reported lower perceived exertion on the shoulders in low load placement and low load placement might be preferable in this respect.
1Introduction
Carrying heavy loads is a necessity for many individuals such as students, military personnel and hikers. Backpacks are often considered the most convenient and appropriate way of achieving this [1–3]. Nevertheless, carrying heavy loads in a backpack can induce increased physical stress and cause discomfort and pain [4]. Additionally, increased oxygen consumption and energy cost have been observed when carrying heavy loads in backpacks compared with no backpack conditions [5, 6]. Quesada et al. [6] reported increased energy cost during walking while carrying 15% and 30% body weight in comparison to a no backpack condition. Patton et al. [5] also compared the energy cost of carrying a 31.5 and 49.4-kg load with a no backpack condition and reported higher energy costs when carrying extra loads.
In addition to the weight of the load, factors such as load placement may influence physical stress and energy cost [7, 8]. It has been suggested loads should be located centrally on the trunk and not carried asymmetrically, as in a briefcase, handbag, shoulder bag or backpack carried unilaterally [8, 9]. Furthermore, in relation to the sagittal plane, the load needs to be carried close to the centre of mass of the body [10], thus minimising the movement created by the load [11].
Despite the recommendations in relation to frontal and sagittal load carrying, controversy exists in relation to the optimal load placement in the axial plane and the importance of load placement within a backpack [12]. Stuempfle et al. [7] reported large differences between high and low load placement in a backpack in terms of oxygen uptake (VO2) and minute ventilation when a 25-kg load was carried in a backpack; however, they only examined female participants. Load placement is even more important during walking as dynamic movements can increase load movement up to 40% due to rotational inertia [13]. On the other hand, Liu [14] found no differences in physiological measures between high and low load placement while carrying 15% body weight at different speeds and grades. Zhao et al. recommended the cross and evenly distributed load mode (i.e. the load within a backpack is distributed evenly between the top-bottom and left-right side of the backpack) and stated that different load distribution modes did not affect the body posture and physiological parameters [15].
With regard to load placement in a backpack, the literature provides conflicting results on physiological variables and is limited regarding movement economy, efficiency and subjective measures such as perceived exertion. Most studies have concentrated on the effect of load placement on physiological variables, while it has also been stated that subjective measures are more sensitive and appropriate for comparing load carriage systems [2]. In addition, when it comes down to daily use, it is the subjective measures that present our perception of exertion. Therefore, subjective measures should be a powerful source of information for comparing different load placements in a backpack. Moreover, load placement conditions have only been compared against each other in the literature and not against a no backpack control condition [7, 14, 15]. It would be helpful to investigate what type of load placement is the closest to a no backpack condition.
The purpose of this study was to examine differences in physiological and self-reported measures of exertion, movement economy and efficiency when carrying a loaded backpack in both a high and low load placement compared to a no backpack control condition. The body can be thought of as an inverted pendulum [16] and we hypothesized that elevation of the centre of mass in high load placement will maximise postural displacement and attenuate imbalance, meaning the body requires more energy to maintain equilibrium [17]. In addition, it has been reported high load placement led to a greater trunk inclination angle [18, 19], resulting in increased displacement of the centre of mass [20]. Therefore, we also hypothesize that increased displacement of centre of mass in high load placement will result in lower movement economy.
2Methods
2.1Participants
We performed a one-way repeated measures study examining the effects of three loading conditions on physiological and self-reported measures of exertion, movement economy and efficiency.
Fifteen healthy participants (eight females and seven males) with mean age of 30.4±5.5 years (18–43 years) from the students and staff of Murdoch University volunteered to participate in this study. All participants were screened through the American Heart Association/American College of Sports Medicine health/fitness facility participation screening questionnaire [21] prior to inclusion.
Participants were included if they were 18 to 45 years of age. Individuals were excluded from participation if they had heart disease, asthma, lung disease, diabetes and/or musculoskeletal problems that limited their physical activity or if they were pregnant. They were also excluded if they were deemed moderate or high risk according to the screening questionnaire.
Prior to data collection, all participants signed a consent form approved by the Human Research Ethics Committee of Murdoch University.
2.2Baseline evaluation and maximal graded exercise test
All participants completed a baseline maximal graded exercise test and three experimental exercise sessions. To prevent carryover effects, we allocated a duration of at least 48 hours between the maximal graded exercise test and the first experimental session. All experimental sessions were separated by no less than one and no more than seven days. Participants wore comfortable light clothing and abstained from intense physical activity for 24 hours prior to testing and fasted for three hours prior to each testing session. Participants then completed a maximal graded exercise test on a Trackmaster treadmill (JAS fitness systems, model TMX 55, KS, USA) during which the volume of expired oxygen (VO2) and carbon dioxide (VCO2) was measured using a Pravo TrueOne 2400 metabolic cart (ParvoMedics, Sandy, UT, USA). The TrueOne metabolic measurement system has been shown to be a reliable and accurate system to measure gas exchange variables [22]. We calibrated the metabolic cart before using gasses of known concentration and through a range of flow rates. Prior to testing, participants were fitted with a Polar heart rate monitor telemetry chest strap (model number T31 Coded-Polar Electro, Kempele, Finland) and heart rate was recorded continuously throughout the duration of the test. The maximal graded exercise test started at a speed of 3 km.h-1 with a 1% grade for the first minute and an increase of 1 kmċh-1 each minute thereafter until a respiratory exchange ratio (RER) equal to 1.0 was achieved and maintained for one minute. After identifying the RER, the treadmill grade was increased by 2% each minute until VO2max was achieved. Participants were deemed to have achieved VO2max if their RER exceeded 1.1, heart rate was greater than 85% of age-predicted max and rating of perceived exertion (RPE) exceeded 17 [23].
2.3Experimental sessions
During the remaining three sessions, the participants completed three 10-minute walking trials, in a randomized and counterbalanced order, using three backpack loading conditions: no backpack, high load placement and low load placement. It is unlikely a backpack user would wear an entirely empty backpack. Thus, we operationalized our unloaded condition as not wearing a backpack at all. Participants walked for ten minutes on a treadmill at a grade of 1.0% at the individualized speed while continuously measuring heart rate and expired ventilation. Walking speed was identified by a RER of 1.0 during the maximal graded exercise test and was constant during all experimental conditions. We chose a constant grade of 1.0% as it accurately simulates outdoor running and compensates for the lack of air resistance in treadmill running [24]. A duration of ten minutes was selected to ensure steady-state physiological measures were achieved during exercise [1, 6]. Only the final two minutes of data collection were used in the data analysis to ensure all variables represented steady-state values.
We used the following formulas to calculate our variables of interest:
Efficiency is a measure of effective work and is an important factor in relation to exercise performance. Efficiency (%) = (work rate/energy expended)×100% [25].
Work rate (kcal.min-1) = weight (kg) [body weight + backpack weight]×speed of treadmill (m/min)×sin Θ (the angle of treadmill inclination).
Energy Expenditure (kcal.min-1) = 3.9 VO2 + 1.1 VCO2 [26].
Economy is a measure of oxygen consumption per unit of power output. Economy (kj.L-1) = speed (m.min-1) / VO2 [25].
In addition, participants rated their overall and regional (neck, shoulder and back) perceived exertion using a Borg scale [27] immediately upon completion of each testing session. The values in Borg scale increase linearly with work load and heart rate, making the scale a good indicator of the degree of physical strain. To fill out the Borg scale, participants were instructed to rate overall and local perceived exertion and not discomfort or pain. This scale consists of 15 points with potential scores ranging from 6 which is very, very light to 20 which is maximum effort.
2.4Backpack configuration
A backpack (Promopak Pty Ltd, Australia) measuring 47×21×15 cm was used for all experimental sessions. The backpack included two main compartments, adjustable padded shoulder straps, adjustable non-elastic hip and elastic sternum belts, two side stabiliser straps and plastic quick-release buckles. Consistent placement of the backpack on the participant across the loading conditions was achieved by measuring the length of the shoulder straps and checking their equality. We divided the interior of the backpack into upper and lower compartments (see Fig. 1). In the high and low load placement, we placed loads in the upper or lower compartments of the backpack respectively and loads were held in place with high-density foam inserts. The no backpack condition was defined as wearing no backpack. We utilized a backpack load equal to 20% of the participant’s bodyweight and not exceeding 20 kg as 20% body weight has been previously used in the relevant literature [1, 28] and reflects common recommendations that the backpack weight should be in the range of 15–30% body weight [6, 29]. The shoulder straps and hip belt of the backpack were adjusted to ensure the best fit for the participants.
Fig.1
The loading conditions in the backpack. a) high load placement b) low load placement.
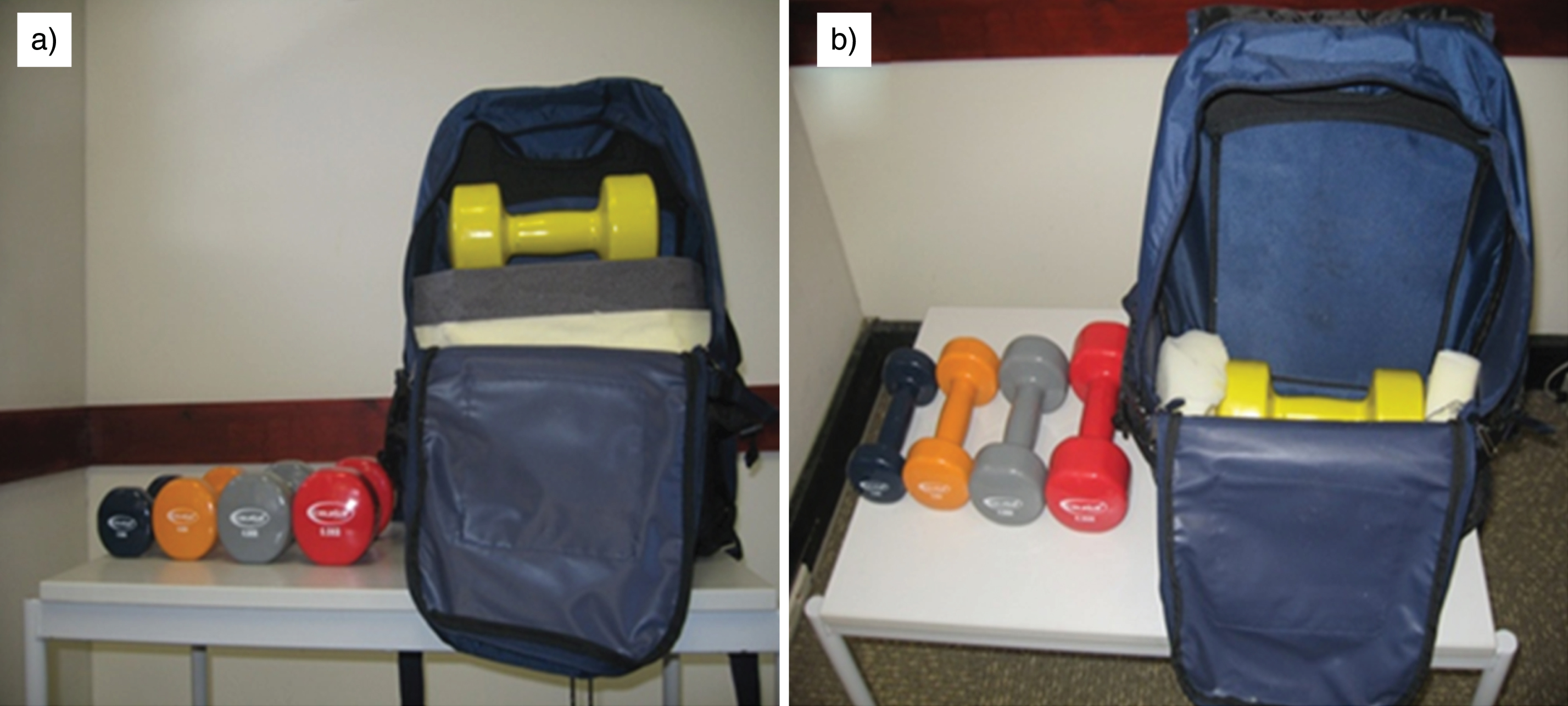
2.5Statistical analysis
Using VO2 data from a previous study [30], we performed an a priori power analysis using G-Power 3.1.3 software [31] for a priori sample size estimation. Assuming an alpha level of 0.05 and within–group standard deviation of 0.58 ml.kg-1.min-1, recruiting 13 participants provides 80% power to detect a change in VO2. To account for an expected dropout rate of 15%, we recruited 15 participants to ensure sufficient power. We entered data into SPSS version 17.0 and calculated descriptive statistics including mean and standard deviation for all dependent variables.
We assessed the normality of the data with the Shapiro–Wilk test and sphericity using Mauchly’s test. The data from the high and low load placement and no backpack condition were compared using separate one–way repeated measures analysis of variance (ANOVA) to examine for differences between loading conditions. Least significant difference was adopted for post hoc pair wise comparisons. The independent variable was the loading condition with three levels (no backpack, high load placement, low load placement), and the dependent variables were VO2 (L.min-1), minute ventilation (L.min-1), heart rate (beats.min-1), movement economy, efficiency and RPE (overall, neck, shoulders and back). An alpha level of 0.05 was used to indicate statistical significance.
3Results
All data measured in this study were normally distributed. None of the participants dropped out of the study. Participant demographic and baseline data are presented in Table 1. The means, standard deviations and mean differences (95% CI) of the dependent variables between loading conditions are reported in Table 2.
Table 1
Demographic and Baseline Characteristics of Participants (N = 15)
Variables | Value |
Age (years) | 30.4 (5.5) |
Sex | 8 female |
BMI (kg.m–2) | 23.3 (5.2) |
Weight (kg) | 71.1 (22.7) |
Height (cm) | 173.3 (11.3) |
VO2 max (L.min–1) | 2.5 (1.0) |
VO2 max (ml.kg–1.min–1) | 36.1 (10.7) |
Heart rate max (beats.min–1) | 167 (16) |
Backpack weight (kg) | 13.1 (3.5) |
Treadmill speed (km.h–1) | 5.3 (1.0) |
Values are mean (standard deviation) unless otherwise indicated.
Table 2
Mean (standard deviation), mean difference (95% CI) values during no backpack, high and low load placement conditions
Mean (SD) | Mean difference (95% CI) | |||||
Variables | No backpack | High load | Low load | No backpack-high load | No backpack-low load | High load-low load |
VO2 (L.min-1) | 1.1 (0.4) | 1.2 (0.5) | 1.2 (0.5) | –0.1 (–0.2, 0.0) | –0.2 (–0.3, 0.0) | 0.0 (–0.1, 0.0) |
MV (L.min-1) | 27.5 (9.3) | 31.7 (11.0) | 32.7(11.9) | –4.2 (–5.8, –2.5) | –4.7 (–7.4, –1.9) | –0.5 (–2.4, 1.3) |
HR (beats. min-1) | 117 (20) | 127 (21) | 124 (16) | –11 (–19, –3) | –8 (–19, 2) | 2 (–5, 10) |
Economy (kj.L-1) | 92.8 (25.0) | 82.9 (22.4) | 81.6(22.2) | 9.9 (4.7, 15.1) | 11.2 (5.3, 16.9) | 1.3 (–1.1, 3.6) |
Efficiency (%) | 18.9 (2.3) | 19.6 (1.9) | 19.4 (2.3) | –0.7 (–1.7, 0.3) | –0.5 (–1.6, 0.7) | 0.3 (–0.3, 0.8) |
Overall RPE | 8.6 (2.0) | 13.1 (2.3) | 12.3 (3.3) | –4.5 (–5.6, –3.3) | –3.7 (–5.3, –2.1) | 0.80 (–0.5, 2.1) |
Shoulder RPE | 7.7 (0.4) | 14.7 (0.7) | 13.0 (0.9) | –7.0 (–8.4, –5.6) | –5.3 (–6.9, –3.8) | 1.7 (0.2, 3.2) |
Neck RPE | 6.5 (0.5) | 12.8 (2.7) | 11.7 (3.8) | –6.3 (–7.8, –4.8) | –5.2 (–7.3, –3.2) | 1.1 (–0.3,2.4) |
Back RPE | 7.5 (0.6) | 11.1 (2.0) | 10.8 (3.1) | –3.5 (–4.8, –2.3) | –3.3 (–5.1, –1.4) | 0.3 (–0.7,1.2) |
HR, heart rate; MV, minute ventilation, RPE, rating of perceived exertion; VO2, oxygen uptake; Significant differences are in bold (p < 0.05).
The omnibus tests revealed significant differences in VO2 (F(2,13) = 4.12, effect size = 0.4, p < 0.05), however the magnitude of the difference was very small and unlikely to represent an important difference. The omnibus tests also revealed significant differences in minute ventilation (F(2,13) = 14.0, effect size = 0.7, p < 0.001), movement economy (F(2,13) = 8.1, effect size = 0.6, p < 0.001,), heart rate (F = (2,13) = 2.3, effect size = 0.3, p < 0.05), overall RPE (F (2,13) = 32.6, effect size = 0.8, p < 0.001), shoulder RPE, neck RPE (F(2,13) = 42.2, effect size = 0.8 p < 0.001), and back RPE (F = (2,13) = 23.2, effect size = 0.8, p < 0.001). Post hoc analysis identified increased VO2 (p < 0.05), minute ventilation (p < 0.05) and decreased economy (p < 0.001) in loaded conditions as compared to the no backpack condition, while no difference (p > 0.05) was observed between the high and low load placement conditions. Increased heart rate was seen in high load placement as compared to the no backpack condition (p < 0.05), while no significant difference (p > 0.05) was observed between no backpack–low load placement and high–low load placements. Overall and local RPEs increased in loaded conditions as compared to the no backpack condition (p < 0.001). Increased shoulder RPE was identified in high load placement as compared to low load placements (p < 0.05), while no difference (p > 0.05) was observed in overall, neck and back RPEs between high and low load placements. There were no differences in efficiency between the no backpack-loaded conditions and high-low load placement (p > 0.05).
4Discussion
We examined differences in physiological and self-reported measures of exertion, movement economy and efficiency of carrying a loaded backpack in high and low load placements compared to a no backpack condition. VO2, minute ventilation and overall RPE increased and movement economy decreased in loaded conditions compared to the no backpack condition; however, there were no differences between high and low load placements. Additionally, shoulder region RPE was higher in high load placement as compared to low load placement and yet load weight and load placement did not influence efficiency.
In our study we observed an increase in VO2 and minute ventilation and a decrease in movement economy from the no backpack to loaded conditions that were consistent with previous research [1, 32], although the sample population of the studies were different. Carrying a loaded backpack causes some modifications in the body, such as increased trunk forward inclination [29], as well as increased activity of respiratory and postural muscle activity [19, 33]. Carrying a backpack loaded with 20% of body weight has shown to result in significantly higher muscle fatigue, blood pressure and forward trunk and head angle [34]. It has been reported that increased muscular activity and changes in posture can lead to higher oxygen consumption, minute ventilation and lower movement economy [33, 35]. The results of the current study showed increased VO2, decreased movement economy and unchanged efficiency. The lack of change in efficiency across the conditions evidenced that, in contrast to the abovementioned theory, increased VO2 and decreased economy is mostly as a result of the additional loading and a small component is due to the normal resultant changes in posture or muscle activity associated with carrying load.
Load placement did not elicit a difference in VO2, minute ventilation or movement economy. Carrying an additional 20% of body weight led to greater VO2 and minute ventilation and lower movement economy regardless of load placement. Our findings are consistent with some studies [14, 15, 36] but not with the study conducted by Stuempfle et al. [7]. Stuempfle et al. studied females and used a constant weight of 25 kg which was about 35% of participants’ body weight and was considerably heavier than the load used in the current study.
Consistent with previous research, we did not identify differences in overall RPE between the high and low load placement conditions [18, 19, 37, 38], which contradicts the finding of Stuempfle et al. [7], who reported high load placement as being the most desirable backpack placement on the spine. Unlike the overall RPE, the shoulder region RPE was greater during the high load placement condition as compared to low load placement. This finding is comparable to the results of Frank et al. [39], who reported higher forces on the shoulders of children while carrying backpacks with high load placement. Previous research has reported RPE incorporates sensations of exertion originating from two sources, the active muscles and the cardiopulmonary system [40]. RPE values of the shoulder area were higher during high load placement; we observed no corresponding increase in VO2, heart rate and overall RPE. One explanation for this could be higher levels of activity of the shoulder girdle muscular system. While we measured physiological variables, we did not investigate muscular activity in this study. However, Bobet and Norman [41] reported higher activity in the upper trapezius muscle during high load placement (the load’s centre of mass was located at the level of the ear lobe) compared to low load placement (the load’s centre of mass was located at the level of the xiphoid process). They suggested this difference may arise from the angular and linear accelerations of the load and trunk [41]; however, their definitions of high and low load placement differed from those of the current study. The second explanation could be localized muscular fatigue due to strap pressure during high load placement. Fatigue of local muscles has been reported as a limiting factor in load carriage [42]. Local muscular fatigue is more likely due to local ischemia and not limitations in aerobic muscular processes [2]. Third, load carriage leads to mental detection of fatigue even when physiological variables are not influenced. Higher perception of effort in the shoulder area during high load placement may override constant physiological variables and overall RPE values [43].
This study had several strengths and weaknesses. We obtained both objective physiological measures and self-reported measures which may measure different aspects of exertion. We used a standardized method; participants walked at a constant forced pace and were therefore unable to modify their walking velocity. They might have adapted differently to load and load placement if they had been able to walk at a self-selected pace. Moreover, walking on a treadmill may not replicate a real-world scenario (speed, gradient, duration). Therefore, while this approach supports the internal validity of our study, the generalisability of our results is limited in this sense.
This study revealed that during carrying a 20% body weight loaded backpack physiological and perceptual variables of exertion are more vulnerable to backpack load than load placement. Although it has previously been recommended to pack heavier items at the top and lighter items at the bottom of the backpack [7], this study showed no difference between the high and low load placements in most of the variables studied. While altering the load placement may not change physiological variables or overall RPE, participants reported lower perceived exertion on the shoulders in low load placement, thus low load placement might be preferable in this respect.
Future research might examine heavier weight as 20% of body weight might not have been heavy enough to present a difference between a high and low load placement. Moreover, future research should seek to replicate our study on children.
Conflict of interest
None to report.
References
[1] | Hong Y , Li JX , Wong ASK , Robinson PD . Effects of load carriage on heart rate, blood pressure and energy expenditure in children. Ergonomics. (2000) ;43: (6):717–27. |
[2] | Legg SJ , Perko L , Campbell P . Subjective perceptual methods for comparing backpacks. Ergonomics. (1997) ;40: (8):809–17. |
[3] | Pal MS , Majumdar D , Bhattacharyya M , Kumar R . Optimum load for carriage by soldiers at two walking speeds on level ground. Int J Ind Ergonomics. (2009) ;39: (1):68–72. |
[4] | Golriz S , Walker BF . Can load carriage system weight, design and placement affect pain and discomfort? A systematic review. J Back Musculoskelet Rehabil. (2011) ;24: (1):1–16. |
[5] | Patton JF , Kaszuba J , Mello RP , Reynolds KL . Physiological responses to prolonged treadmill walking with external loads. Eur J Appl Physiol. (1991) ;63: (2):89–93. |
[6] | Quesada PM , Mengelkoch LJ , Hale RC , Simon SR . Biomechanical and metabolic effects of varying backpack loading on simulated marching. Ergonomics. (2000) ;43: (3):293–309. |
[7] | Stuempfle KJ , Drury DG , Wilson AL . Effect of load position on physiological and perceptual responses during load carriage with an internal frame backpack. Ergonomics. (2004) ;47: (7):784–9. |
[8] | Zultowski I , Aruin A . Carrying loads and postural sway in standing: The effect of load placement and magnitude. Work. (2008) ;30: (4):359–68. |
[9] | Macias BR , Murthy G , Chambers H , Hargens AR . Asymmetric loads and pain associated with backpack carrying by children. J Pediatr Ortho. (2008) ;28: (5):512–7. |
[10] | Knapik JJ , Ang P , Meiselman H , Johnson W , Kirk J , Bensel C , et al. Soldier performance and strenuous road marching: Influence of load mass and load distribution. Mil Med. (1997) ;162: (1):62–7. |
[11] | Knapik J , Harman E , Reynolds K . Load carriage using packs: A review of physiological, biomechanical and medical aspects. Appl Ergon. (1996) ;27: (3):207–16. |
[12] | Golriz S , Walker BF . Backpacks. Several factors likely to influence design and usage: A systematic literature review. Work. (2012) ;42: (4):519–31. |
[13] | Bloom D , Woodhull-McNeal AP . Postural adjustments while standing with two types of loaded backpack. Ergonomics. (1987) ;30: (10):1425–30. |
[14] | Liu BS . Backpack load positioning and walking surface slope effects on physiological responses in infantry soldiers. Int J Ind Ergonomics. (2007) ;37: (9-10):754–60. |
[15] | Zhao MY , Ni YK , Tian S , Tang QH , Wang LZ , Fan YB . Effects of different load distribution modes on human physiological parameters during walking. Journal of Medical Biomechanics. (2015) ;30: (1):8–13. |
[16] | Winter DA , Mackinnon CD , Ruder GK , Wieman C . An integrated EMG/biomechanical model of upper body balance and posture during human gait. Prog Brain Res. (1993) ;97: , 359–67. |
[17] | Pedersen AV , Stokke R , Mamen A . Effects of extra load position on energy expenditure in treadmill running. Eur J Appl Physiol. (2007) ;102: (1):27–31. |
[18] | Brackley HM , Stevenson JM , Selinger JC . Effect of backpack load placement on posture and spinal curvature in prepubescent children. Work. (2009) ;32: (3):351–60. |
[19] | Devroey C , Jonkers I , de Becker A , Lenaerts G , Spaepen A . Evaluation of the effect of backpack load and position during standing and walking using biomechanical, physiological and subjective measures. Ergonomics. (2007) ;50: (5):728–42. |
[20] | Lloyd R , Cooke C . Biomechanical differences associated with two different load carriage systems and their relationship to economy. Human Movement. (2011) ;12: (1):65–74. |
[21] | ACSM. ACSM’s guidelines for exercise testing and prescription. 8th ed. Philadelphia: Wolters Kluwer Health Ltd; (2009) . |
[22] | Crouter SE , Antczak A , Hudak JR , DellaValle DM , Haas JD . Accuracy and reliability of the ParvoMedics TrueOne and MedGraphics VOmetabolic systems. Eur J Appl Physiol. (2006) ;98: (2):139–51. |
[23] | Peiffer JJ , Abbiss CR , Chapman D , Laursen PB , Parker DL . Physiological characteristics of masters-level cyclists. J Strength Cond Res. (2008) ;22: (5):1434–40. |
[24] | Jones AM , Doust JH . A 1% treadmill grade most accurately reflects the energetic cost of outdoor running. J Sports Sci. (1996) ;14: (4):321–7. |
[25] | Moseley L , Jeukendrup AE . The reliability of cycling efficiency. Med Sci Sports Exerc. (2001) ;33: (4):621–7. |
[26] | Weir JB . New methods for calculating metabolic rate with special reference to protein metabolism. J Physiol. (1949) ;109: , 1–9. |
[27] | Borg GAV . Perceived exertion: A note on ‘history’ and methods. Med Sci Sports Exerc. (1973) ;5: (2):90–3. |
[28] | Singh T , Koh M . Effects of backpack load position on spatiotemporal parameters and trunk forward lean. Gait Posture. (2009) ;29: (1):49–53. |
[29] | Goh JH , Thambyah A , Bose K . Effects of varying backpack loads on peak forces in the lumbosacral spine during walking. Clin Biomech. (1998) ;13: (Suppl. 1):S26–S31. |
[30] | Wallman K , Goodman C , Morton A , Grove R , Dawson B . Test-retest reliability of the aerobic power index component of the tri-level fitness profile in a sedentary population. J Sci Med Sport. (2003) ;6: (4):443–54. |
[31] | Erdfelder E , Faul F , Buchner A . Gpower: A general power analysis program. Behavior Research Methods, Instruments and Computers. (1996) ;28: , 1–11. |
[32] | Hong Y , Li JX , Fong DTP . Effect of prolonged walking with backpack loads on trunk muscle activity and fatigue in children. J Electromyogr Kinesiol. (2008) ;18: (6):990–6. |
[33] | Li JX , Hong Y , Robinson PD . The effect of load carriage on movement kinematics and respiratory parameters in children during walking. Eur J Appl Physiol. (2003) ;90: (1-2):35–43. |
[34] | Zhao M , Tian S , Tang Q , Ni Y , Wang L , Fan Y . Effect of different backpack loads on physiological parame ters in walking. J Biomed Eng. (2014) ;31: (5):978–83. |
[35] | Yoshizaki H , Yoshida A , Hayashi F , Fukuda Y . Effect of posture change on control of ventilation. Jpn J Physiol. (1998) ;48: (4):267–73. |
[36] | Johnson RC , Pelot RP , Doan JB , Stevenson JM . The effect of load position on biomechanical and physiological measures during a short duration march. Defense Technical Information, (2000) . |
[37] | Golriz S , Hebert JJ , Foreman KB , Walker BF . The effect of hip belt use and load placement in a backpack on postural stability and perceived exertion: A within-subjects trial. Ergonomics. (2015) ;58: (1):140–7. |
[38] | Simpson KM , Munro BJ , Steele JR . Does load position affect gait and subjective responses of females during load carriage? Appl Ergon. (2012) ;43: (3):479–85. |
[39] | Frank E , Stevenson JM , Stothart P . The effect of load placement on static posture and reaction forces in youth. Med Sci Sports Exerc. (2003) ;35: (5):S21. |
[40] | Ekblom B , Goldbarg AN . The influence of physical training and other factors on the subjective rating of perceived exertion. Acta Physiol Scand. (1971) ;83: (3):399–406. |
[41] | Bobet J , Norman RW . Effects of load placement on back muscle activity in load carriage. Eur J Appl Physiol. (1984) ;53: (1):71–5. |
[42] | Legg SJ , Mahanty A . Comparison of five modes of carrying a load close to the trunk. Ergonomics. (1985) ;28: (12):1653–60. |
[43] | Kirk J , Schneider DA . Physiological and perceptual responses to load-carrying in female subjects using internal and external frame backpacks. Ergonomics. (1992) ;35: (4):445–55. |