Differences in posture kinematics between using a tablet, a laptop, and a desktop computer in sitting and in standing
Abstract
BACKGROUND:
Alternative methods of accessing the internet and performing computing-related work tasks are becoming common, e.g., using tablets or standing workstations. Few studies examine postural differences while using these alternative methods.
OBJECTIVE:
To assess neck and upper limb kinematics while using a tablet, laptop and desktop computer (sitting and standing).
METHODS:
Differences in neck flexion/extension, lateral flexion, rotation; elbow flexion/extension; wrist flexion/extension, radial/ulnar deviation; and shoulder elevation in 30 participants were assessed in four conditions, three in sitting (tablet, laptop and desktop computer) and one in standing (desktop computer). Three-dimensional motion capture recorded posture variables during an editing task. Differences between variables were determined using one-way ANOVA with Bonferroni post-hoc tests.
RESULTS:
Compared to the desktop (sitting), tablet and laptop use resulted in increased neck flexion (mean difference tablet 16.92°, 95% CI 12.79–21.04; laptop 10.92, 7.86–13.97, P < 0.001) and shoulder elevation (right; tablet 10.29, 5.27–15.11; laptop 7.36, 3.72–11.01, P < 0.001). There were no meaningful posture differences between the sitting and standing desktop.
CONCLUSIONS:
These findings suggest that using a tablet or laptop may increase neck flexion, potentially increasing posture strain. Regular users of tablets/laptops should consider adjustments in their posture, however, further research is required to determine whether posture adjustments prevent or reduce musculoskeletal symptoms.
1Introduction
The majority of households (84–86%) in westernized countries have access to internet [1, 2]. Individuals use a variety of devices: desktop or laptop for 94% of users, mobile or smart phone for 86% and tablets for 62% [1]. The use of computers has been associated with neck and wrist pain [3], though there has been less research investigating the use of tablet computers [4], which are being used with increasing frequency [5]. Neck pain is a worldwide health problem, as it is the 4th greatest contributor to global disability [6] and results in reduced work productivity [7].
Poor posture during computer use is a likely contributor to altered muscle activation patterns contributing to neck and shoulder pain [8]. Of particular concern is that poor posture could be further exaggerated by using a tablet computer which is smaller and difficult to position efficiently for good posture [4]. Tablets are being used with increasing frequency, with one report indicating a 25% increase in tablet usage between 2014 and 2015, with 59% of 2000 respondents aged 18–75 having access to a tablet in 2015 [5]. Thus research on posture during tablet use is timely and relevant.
Many studies have investigated posture during the use of either a desktop or laptop computer [9–16]. Most of these studies investigated postural changes during the use of one electronic device with different workstation configurations, e.g., using or not using arm rest [16]; few studies examined postural differences during the use of different electronic devices [13] or the same electronic device at different workstations [9]. Increased neck flexion while using a laptop compared to a desktop computer has been previously reported [13, 17]. However, fewer studies have examined tablet computer use and posture [4, 15, 18]. Tablet use likely results in different postural changes compared to laptop computer use. Indeed, increased neck flexion has been demonstrated with tablet use [4, 18], as well as increased wrist flexion and ulnar deviation [4]. With increasing use of varied workstations such as tablets and laptops within society, the postures assumed during use of these alternative workstations warrant investigation.
No previous research was identified comparing neck and upper limb posture during the use of all three devices: desktop, laptop and tablet computer. Furthermore, only one identified study investigated kinematic changes of posture while working at a standing computer workstation as compared to a standard desk [19]. The current study aimed to determine whether there were differences in neck and upper limb postures when using different computing conditions including 1) a tablet in sitting; 2) a laptop computer in sitting; 3) a desktop computer in sitting and 4) a desktop computer in standing. We hypothesised that there would be differences in neck and upper limb postures with the use of tablets and laptops resulting in poorer posture. These data will improve the understanding of postures during computerised device use and ultimately lead to recommendations for workstation or device choices.
2Methods
2.1Study design
This within subject observational study compared the posture of the same individual in four different conditions (sitting with a desktop computer, standing with a desktop computer, sitting with a laptop and sitting with a tablet) using three dimensional (3D) motion analysis.
2.2Participant recruitment
Sample size calculation was based on previous data indicating the standard deviation of neck flexion/extension ranges of motion between 8.1° when sitting at a desk and 9.7° when using a laptop [4]. Using an average estimate of the variation in neck flexion/extension of 9°, a difference in neck flexion/extension angle of 5° between workstations could be detected with 80% power at an alpha of 0.05 with a sample size of 27 per comparison. As this was a within-subjects design, we set the sample size for recruitment at 30.
Volunteers for this study were recruited by advertising the study on noticeboards around the University campus where the study took place and on the University Facebook pages. Participants were required to have had at least some previous experience of using a tablet, a laptop and a desktop computer. Potential participants were excluded due to chronic disease or deformity in the neck, back or arms; or pain, injury or trauma in these areas for which they sought treatment in the past 2 years. This research complied with the American Psychological Association Code of Ethics and was approved by the Institutional Review Board at the University of Newcastle. Australia (H-2015-0039). Informed consent was obtained from each participant.
2.3Task
The most common online activities, email (performed by 97% of internet users), browsing (96%) and searching for information (95%), are related to typing and editing [20]. Therefore, a 5-minute editing task requiring typing and editing was performed on each device. Participants first performed a 2-minute typing task on each device as a warm-up to familiarize themselves with the workstation, the electronic device and the software used. This 2-minute typing task required participants to type out Edgar Allen Poe’s The Raven, and data were not collected during this task. The 5-minute editing task performed in each condition (laptop, tablet, and desktop sitting, desktop standing) involved selecting, deleting and typing new phrases using prompts in a standardised article in Microsoft Word (Microsoft Corp., Redmond, WA, USA), previously used by Gold et al. [12]. Data were collected during the fourth minute of the editing task for each condition. In order to minimise the effects of altered posture due to anticipation or familiarized content in the comparison of workstations, all participants completed each workstation in a randomised order determined by a computerised random number generator.
2.4Electronic devices
The keyboard of each electronic device was positioned 6–7 cm from the edge of the desk, and for the desktop conditions using the external keyboard, it was laid flat without using the two posteriorly placed supporting legs [21, 22]. The 9.7-inch iPad4 with no case or other add-ons was placed on the desk flat and in landscape orientation. For the tablet condition, Microsoft 365 Word was used on iPad 4 with iOS 8 (Apple Inc., Cupertino, CA, USA) operating system to ensure the computerized editing activity was the same on each device. A 15.6-inch laptop (Model No. E6540, Dell Inc., Round Rock, TX, USA) with no mouse or other add-ons was used for the laptop component in this study. The screen tilt angle was adjusted to the participant’s preference, as ideal tilt angles for working on laptops have not been established [23]. For the laptop, Microsoft Office Word Home and Business 2013 (Microsoft Corp., Redmond, WA, USA) was used with the Microsoft Windows 7 operating system. For the simulated desktop conditions (sitting and standing), the same laptop was equipped with a standard 104-key Windows keyboard (Model No. KB212B), a chorded optic mouse (Model No. MS116) and a 23-inch monitor (Model No. P2314 H, Dell, Inc, Round Rock TX, USA) mounted on an adjustable sitting/standing workstation. The display was projected on the monitor using a standard Video Graphics Array (VGA) cable with the laptop lid in the closed position. The devices and their positioning for each condition are illustrated in Fig. 1.
Fig. 1.
Device placement for each experimental condition: (A) tablet, (B) laptop, (C) desktop, sitting, and (D) desktop, standing, with retro-reflective marker configuration.
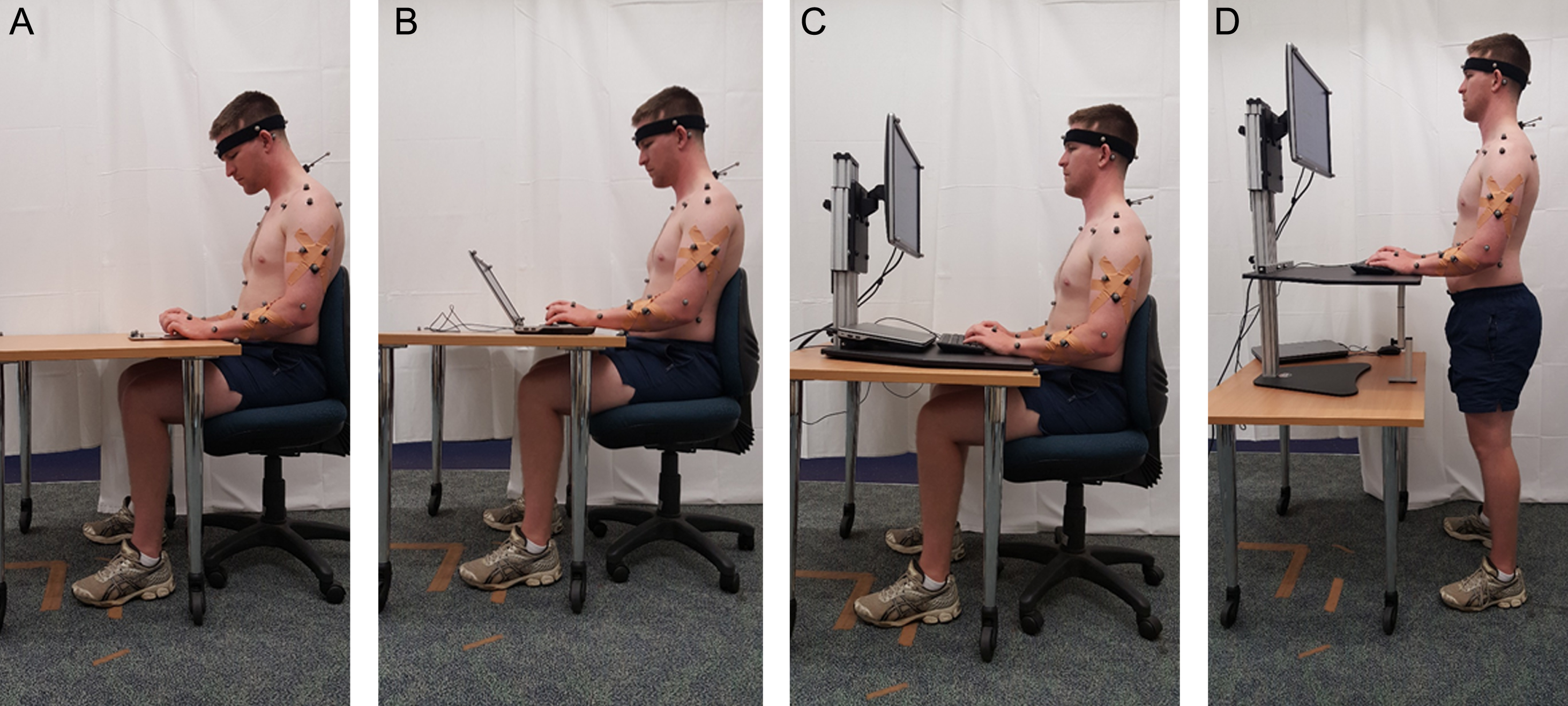
2.5Condition descriptions
The sitting workstation for the tablet, laptop and desktop computer included a standard 760 mm height desk and a 5-pronged caster base, height adjustable office chair (Model No. QU26, Sturdy Framac, Padstow, NSW, Australia). Chair height was measured in standing with the highest point of the seat right below kneecap. Participants were seated so the back support of the office chair was used at all times when they were in sitting [22].
For the desktop conditions, the flat panel monitor was mounted on an adjustable sitting/standing workstation (Kangaroo Pro, Ergonomic Essentials, North Ryde, Australia) placed on top of the standard office desk. The sitting/standing workstation had an independently adjustable screen height and work surface height. For sitting and standing, the screen height was set to have the top one-third of the screen at or below eye level. For standing work surface height was set to have the top of the desk at elbow height [22]. This adjustable workstation was removed from the desk for the laptop and tablet conditions.
2.6Procedure
Participants attended a university laboratory where they completed a demographic questionnaire including: age, gender, dominant hand, occupation, physical activity using the Godin-Shepherd Leisure-time Physical Activity Questionnaire (GLPAQ) [24], types of electronic devices used every day (desktop computer, laptop computer, tablet, phone), and average time spent on each. Six Qualisys motion capture cameras (100 Hz; 300+, Qualisys AB, Gothenburg, Sweden) were used to record the three-dimensional (3D) movements of the head, trunk and upper limbs. Retro-reflective markers were taped on bony land marks bilaterally on the mastoid, acromioclavicular (AC) joint, greater tubercle of the humerus, anterior and posterior glenohumeral joint, medial and lateral epicondyle, radial and ulnar styloid process, head of the third metacarpal, and laterally on the iliac crest and lowest point on the ribs. Single markers were also taped on the C2 spinous process, sternal notch (SN), and xiphoid process. In addition, five tracking clusters of three markers each were positioned with medical adhesive tape on bilateral humeri, forearms and C7. A tracking headband of four markers was positioned around the head, so that markers aligned vertically with the nose anteriorly, tragus of the ear laterally, and midpoint of the external occipital protuberance posteriorly.
2.7Kinematic analysis and reduction
Three-dimensional kinematic data were analysed using Visual 3D software (Version 5; C-Motion, Germantown, MD, USA). All raw kinematic coordinates were filtered using a fourth-order zero phase-shift Butterworth digital low-pass filter (fc = 8 Hz) prior to calculation of individual joint kinematics. Geometric primitives were used to model the inertial properties of each segment [25], classifying the neck and thorax as cylindrical [26]; the upper arm and forearm as a frusta of a cone [27]; the hand as spherical; and the head and head-neck as an elliptical cylinder.
Traditionally, the head and neck segments are treated as one segment (head-neck); however, to enable more complex kinematic modelling, separate head and neck segments were created. The distal joint centre of the head was defined as the mid-head landmark (the midpoint of the four headband markers), with a radius of 50% of the distance between the left and right lateral headband markers. The proximal joint centre of the head was defined as the prox-head (the axial and medial lateral position of the mid-head landmark and the axial position of C7-SN landmark (50% distance between these C7 and SN markers) with a radius of 50% of the distance between the left and right C7 markers. The neck joint centres were defined as for the proximal joint as the C7-SN landmark, and distal joint as the C7-SN landmark with a 0.1 m axial offset inferiorly with a radius of 50% of the distance between the left and right C7 markers. The traditional head-neck segment joint centres were defined as the C7-SN and mid-head landmarks, with a radius of 50% of the distance between the left and right lateral headband markers.
Cartesian local coordinate system sign convention was conserved by defining all segments as follows: the x-axis as mediolateral axis, the y-axis as anterior-posterior axis and the z-axis as superior-inferior axis. Intersegmental joint angles were conveyed using an x,y,z Cardan sequence of rotation. Data collected at 100 Hz included joint angles (°) for head-neck (HN) and headneck-trunk (HNT) flexion/extension, lateral flexion (left or right) and rotation (left or right), bilateral elbow flexion, bilateral wrist flexion-extension and radial-ulnar deviation. As a measure of shoulder elevation usually considered as a marker of excessive upper trapezius muscle activity and tension in the upper shoulders and neck, AC-C7 vertical distance (mm) was measured by calculating the mean vertical distance from the acromioclavicular joint marker perpendicular to a horizontal line bisecting C7. To calculate mean bilateral shoulder elevation (mm) during a task, the AC-C7 vertical distance measured in a static standing position was subtracted from the mean AC-C7 vertical distance during the one-minute typing tasks. Although the psychometric properties of posture measurement using 3D motion analysis have not been fully established [28], 3D motion analysis has demonstrated high test-retest reliability for joint angles [29] (the focus of the current study), and greater reliability when using marker ‘clusters’ (as used in the current study) rather than single markers for measuring spine movements [30].
2.8Statistical analysis
Descriptive statistics were used to report participant characteristics and posture variables. Differences in the posture variables between the four workstation conditions were compared using repeated measures analyses of variance (RM ANOVA) with Bonferroni post-hoc tests. The significance threshold was set at (P < 0.05). Each posture variable was examined separately using RM ANOVA, with the repeated factor being workstation (tablet, laptop, desktop [sitting] and desktop [standing]). Partial Eta2 are reported for each RM ANOVA to indicate the size of the effect of workstation on posture. Greenhouse-Geisser adjustment was used when Mauchley’s test of sphericity was not met. SPSS v21.0 (IBM Inc., Armonk, NY, USA) was used for all statistical analyses.
3Results
3.1Participants
Thirty participants completed the study (17 females, 13 males, mean age 25.9±7.42 years, range 20–50, mean height 171.62±9.22 cm, mean weight 69.90±14.14 kg). Three participants (10%) reported left hand dominance, however all of them reported that they typically used a computer mouse in their right hand; therefore, all participants used a right-handed mouse in this study. Twenty-three (76.7%) were university students and seven (23.3%) were employed full-time in various occupations, e.g. clerical, wait staff. The majority of participants were considered active, scoring≥24 on the GLPAQ (80.0%, n = 24) with 10% (n = 3) moderately active and 10.0% (n = 3) insufficiently active.
Eleven participants (36.7%) self-reported daily desktop computer use, 25 (83.3%) laptop, 16 (53.3%) tablet and all 30 (100%) daily smart phone usage. The daily desktop usage had a mean of 1.45±2.45 hours; laptop 3.18±2.54 hours, tablet 0.70±1.09 hours; and smart phone 3.07±3.61 hours.
3.2Postural variables
Descriptive data for posture variables for each workstation are reported in Table 1. The variables of wrist flexion/extension and radial/ulnar deviation at the tablet station only include 29 participants, as one participant was an outlier; they rested their left arm on table without using it for the editing task.
Table 1
Kinematic postural variables (mean, SD) at each workstation over one minute of editing with results of repeated measures ANOVAs for overall differences between workstations
Posture variable | Tablet | Laptop | Desktop (sitting) | Desktop (standing) | df** | F | P-value | Partial Eta2 |
HN† extension (+) /Flexion (–)°* | –13.68 (6.31) | –7.67 (6.57) | 3.24 (9.85) | 1.22 (8.47) | 3 | 92.086 | <0.001 | 0.761 |
HN lateral flexion, right (+)/Left (–)° | –0.12 (3.31) | 0.44 (3.72) | 0.51 (3.96) | 0.17 (3.09) | 3 | 0.816 | 0.489 | 0.027 |
HN rotation, left (+) /Right (–)° | –1.27 (5.65) | –0.88 (6.01) | –0.23 (7.17) | –1.88 (6.01) | 2.00 | 2.951 | 0.060 | 0.092 |
HNT‡ extension (+) /Flexion (–)°* | –36.20 (8.56) | –30.88 (7.85) | –24.85 (8.35) | –24.93 (6.84) | 3 | 98.89 | <0.001 | 0.773 |
HNT lateral flexion, right (+)/Left (–)° | –0.91 (2.71) | 0.77 (3.21) | 0.77 (3.24) | 0.35 (2.87) | 3 | 7.81 | <0.001 | 0.212 |
HNT rotation, left (+) /Right (–)° | –1.83 (4.22) | –1.22 (3.97) | –0.29 (3.90) | –1.57 (4.04) | 1.98 | 3.20 | 0.049 | 0.100 |
Left elbow flexion (+)/Extension (–) | 96.72 (12.04) | 87.17 (10.55) | 90.49 (11.12) | 85.81 (9.94) | 3 | 12.75 | <0.001 | 0.305 |
Left wrist flexion (+)/Extension (–)° | –17.23 (10.49) | –12.92 (8.56) | –17.36 (9.96) | –19.09 (9.98) | 2.49 | 7.47 | <0.001 | 0.211 |
Left wrist ulnar (+) /Radial deviation (–)° | –15.65 (10.89) | –10.79 (11.46) | –17.76 (9.61) | –20.38 (10.16) | 2.12 | 7.81 | 0.001 | 0.218 |
Right elbow flexion (+)/Extension°* | 100.97 (10.12) | 89.85 (11.16) | 84.26 (13.80) | 81.53 (10.50) | 3 | 37.39 | <0.001 | 0.563 |
Right wrist flexion (+)/Extension (–)° | –14.83 (8.10) | –13.36 (9.17) | –19.41 (10.12) | –22.10 (9.84) | 3 | 23.82 | <0.001 | 0.451 |
Right wrist ulnar (+) /Radial deviation (–)° | –16.33 (7.74) | –15.58 (7.28) | –18.89 (9.50) | –22.24 (8.67) | 3 | 8.39 | <0.001 | 0.224 |
Left AC-C7 distance (mm) | 78.23 (16.25) | 72.88 (15.39) | 66.62 (16.18) | 68.69 (15.01) | 2.29 | 21.58 | <0.001 | 0.427 |
Left shoulder elevation (mm) | 21.57 (14.51) | 16.22 (12.65) | 9.96 (13.58) | 12.03 (11.23) | 2.29 | 21.58 | <0.001 | 0.427 |
Right AC-C7 distance (mm) | 80.08 (18.16) | 77.15 (16.02) | 69.78 (15.24) | 73.70 (14.74) | 3 | 11.95 | <0.001 | 0.292 |
Right shoulder elevation (mm) | 17.02 (14.70) | 14.09 (13.30) | 6.73 (13.86) | 10.65 (10.41) | 3 | 11.95 | <0.001 | 0.292 |
[i] *(+) = positive; a positive number for that value indicates that movement direction; (–) = negative; a negative number for that value indicates that movement direction.
[ii] **df = degrees of freedom. Values for the Greenhouse-Geisser adjustment are reported when Mauchley’s test of sphericity was significant.
[iii] †HN = Head segment on neck segment.
[iv] ‡HNT = Headneck segment on trunk segment.
3.3Effect of workstation
There was a statistically significant effect of workstation on all postural variables, except for HN lateral flexion and HN rotation (Table 1). Therefore, pairwise differences in posture variables between workstations are reported for all variables excluding these two in Tables 2 and 3. Observations of differences between workstations for the HN and the HNT postural variables were similar (Table 1), but overall differences did not reach significance for HN lateral flexion and HN rotation whereas these were significant for the HNT angles. The difference between workstations for AC-C7 vertical distance and shoulder elevation was the same, as the calculation of shoulder elevation represented a simple transformation of the AC-C7 vertical distance data. Therefore workstation differences are only included for shoulder elevation in Tables 2 and 3.
Table 2
Mean differences in kinematic postural variables between workstations focussing on tablet use [Tablet – Laptop, Tablet – Desktop (sitting), Tablet – Desktop (standing)]
Tablet – Laptop** | Tablet – Desktop (sitting) | Tablet – Desktop (standing) | |||||||
Mean Difference# | 95% CI | P | Mean Difference | 95% CI | P | Mean Difference | 95% CI | P | |
HN† extension (+) /Flexion (–)°* | –6.00 | –8.74, –3.26 | <0.001 | –16.92 | –21.04, –12.79 | <0.001 | –14.89 | –18.22, –11.57 | <0.001 |
HNT‡ Extension (+) /Flexion (–)°* | –5.32 | –7.05, –3.58 | <0.001 | –11.35 | –13.99, –8.71 | <0.001 | –11.26 | –13.49, –9.04 | <0.001 |
HNT Lateral Flexion, Right (+)/Left (–)° | –1.69 | –2.77, – 0.61 | 0.001 | –1.68 | –3.07, –0.30 | 0.011 | –1.26 | –2.29, –0.23 | 0.010 |
HNT Rotation, Left (+)/Right (–)° | –0.61 | –1.83, 0.61 | 1.000 | –1.54 | –3.62, 0.54 | 0.267 | –0.26 | –1.66, 1.15 | 1.000 |
Left elbow flexion (+)/Extension (–) | 9.55 | 4.73, 14.38 | <0.001 | 6.23 | 0.90, 11.57 | 0.015 | 10.91 | 4.55, 17.27 | <0.001 |
Left Wrist Flexion (+)/Extension (–)° | –4.08 | –8.31, 0.16 | 0.064 | 0.33 | –3.00, 3.67 | 1.000 | 2.10 | –2.38, 6.58 | 1.000 |
Left Wrist Ulnar (+)/Radial Deviation (–)° | –4.80 | –11.96, 2.37 | 0.41 | 2.15 | –2.92, 7.21 | 1.000 | 4.45 | –1.30, 10.20 | 0.218 |
Right Elbow Flexion (+)/Extension (–)° | 11.13 | 6.79, 15.47 | <0.001 | 16.71 | 10.97, 22.46 | <0.001 | 19.44 | 14.02, 24.86 | <0.001 |
Right Wrist Flexion (+)/Extension (–)° | –1.47 | –4.95, 2.01 | 1.000 | 4.57 | 1.27, 7.88 | 0.003 | 7.27 | 3.73, 10.80 | <0.001 |
Right Wrist Ulnar (+)/Radial Deviation (–)° | –0.75 | –4.67, 3.17 | 1.000 | 2.56 | –1.33, 6.45 | 0.434 | 5.91 | 1.29, 10.53 | 0.007 |
Left Shoulder Elevation (mm) | 5.35 | 0.99, 9.72 | 0.010 | 11.61 | 5.91, 17.31 | <0.001 | 9.55 | 4.73, 14.37 | <0.001 |
Right Shoulder Elevation (mm) | 2.93 | –2.44, 8.230 | 0.798 | 10.29 | 5.47, 15.11 | <0.001 | 6.37 | 0.15, 12.60 | 0.042 |
[i] *(+) = positive; a positive number for that value indicates that movement direction; (–) = negative; a negative number for that value indicates that movement direction.
[ii] **To indicate the direction of comparisons, ’ – ‘ denotes the difference was calculated as the first workstation ‘minus’ the second workstation for each comparison.
[iii] #Mean differences for Bonferroni post-hoc tests following one-way analysis of variance based on the estimated marginal means, with Bonferroni-adjusted p values.
[iv] †HN = Head segment on neck segment.
[v] ‡HNT = Headneck segment on trunk segment.
Significantly greater HN and HNT flexion, bilateral elbow flexion, and shoulder elevation occurred when using the tablet compared to all three other workstations, though the difference in right shoulder elevation between the tablet and the laptop did not reach significance (Table 2). There was greater wrist flexion using the tablet compared to the desktop in sitting or standing, but not the laptop (Table 2). Though there was statistically less HNT lateral flexion using the tablet compared to the three other workstations, the magnitudes of the absolute differences were functionally irrelevant (<2°, with 95% CIs for each of the three comparisons spanning approximately 3° or less), and also suggested by a small effect size (0.212).
When comparing the laptop to the desktop computer (in both sitting or standing), laptop use resulted in significantly greater HN, HNT, elbow and wrist flexion and shoulder elevation (Table 3), except for right shoulder elevation between the laptop and the desktop in standing. Participants displayed significantly greater right wrist ulnar deviation using the laptop compared to the desktop in standing, but this difference did not reach significance when comparing the laptop to the desktop in sitting. Most postural variables were similar between a sitting and a standing desktop (Table 3). Although participants displayed significantly greater HNT left rotation when using a desktop in sitting compared to standing, the magnitude of the absolute difference was functionally irrelevant (1.28°, 95% CI 0.23, 2.29, effect size 0.100).
Table 3
Mean differences in kinematic postural variables between workstations focussing on laptop use [(Laptop – Desktop (sitting), Laptop – Desktop (standing), Desktop (sitting) – Desktop (standing)]
Laptop – Desktop Sitting** | Laptop – Desktop (standing) | Desktop (sitting) – Desktop (standing) | |||||||
Mean Difference# | 95% CI | P | Mean Difference | 95% CI | P | Mean Difference | 95% CI | P | |
HN† extension (+) /flexion (–)°* | –10.92 | –13.97, –7.86 | <0.001 | –8.89 | –11.94, –5.84 | <0.001 | 2.02 | –1.26, 5.31 | 0.549 |
HNT‡ Extension (+) /Flexion (–)°* | –6.04 | –8.07, –4.01 | <0.001 | –5.95 | –7.99, –3.90 | <0.001 | 0.09 | –2.28, 2.46 | 1.000 |
HNT Lateral Flexion, Right (+)/Left (–)° | 0.01 | –1.24, 1.25 | 1.000 | 0.43 | –0.57, 1.42 | 1.000 | 0.42 | –0.65, 1.49 | 1.000 |
HNT Rotation, Left (+)/Right (–)° | –0.93 | –2.59, 0.71 | 0.740 | 0.35 | –0.87, 1.57 | 1.000 | 1.28 | 0.02, 2.55 | 0.046 |
Left elbow flexion (+)/Extension (–) | –3.32 | –8.08, 1.43 | 0.344 | 1.35 | –4.61, 7.32 | 1.000 | 4.68 | –0.63, 9.98 | 0.111 |
Left Wrist Flexion (+)/Extension (–)° | 4.41 | 0.65, 8.17 | 0.015 | 6.18 | 1.99, 10.36 | 0.002 | 1.77 | –0.97, 4.50 | 0.465 |
Left Wrist Ulnar (+)/Radial Deviation (–)° | 6.94 | 1.53, 12.35 | 0.007 | 9.25 | 2.48, 16.02 | 0.003 | 2.30 | –0.36, 4.97 | 0.124 |
Right Elbow Flexion (+)/Extension° | 5.59 | 0.54, 10.64 | 0.024 | 8.31 | 1.82, 14.80 | 0.007 | 2.72 | –3.74, 9.18 | 1.000 |
Right Wrist Flexion (+)/Extension (–)° | 6.05 | 2.94, 9.15 | <0.001 | 8.74 | 5.23, 12.25 | <0.001 | 2.69 | –0.22, 5.60 | 0.083 |
Right Wrist Ulnar (+)/Radial Deviation (–)° | 3.31 | –0.82, 7.45 | 0.184 | 6.66 | 2.02, 11.30 | 0.002 | 3.35 | –0.31, 7.01 | 0.089 |
Left Shoulder Elevation (mm) | 6.26 | 2.62, 9.90 | <0.001 | 4.19 | 0.12, 8.27 | 0.041 | –2.06 | –5.61, 1.49 | 0.663 |
Right Shoulder Elevation (mm) | 7.36 | 3.72, 11.01 | <0.001 | 3.44 | –2.02, 8.91 | 0.509 | –3.92 | –8.90, 1.07 | 0.204 |
[i] *(+) = positive; a positive number for that value indicates that movement direction; (–) = negative; a negative number for that value indicates that movement direction.
[ii] **To indicate the direction of comparisons, ’ – ‘ denotes the difference was calculated as the first workstation ‘minus’ the second workstation for each comparison.
[iii] #Mean differences from Bonferroni post-hoc tests following one-way analysis of variance, with Bonferroni-adjusted p values.
[iv] †HN = Head segment on neck segment.
[v] ‡HNT = Headneck segment on trunk segment.
4Discussion
Prior to this study, there was little evidence for posture differences between the use of a tablet, a laptop, a desktop computer, and a standing workstation. Significant differences were found in postures between all four types of workstations. The key finding was that tablet and laptop use were associated with increased neck flexion, elbow flexion and shoulder elevation compared to the desktop (sitting or standing), suggesting these devices position the neck and shoulder joints in potentially damaging positions. These three variables (neck and elbow flexion, shoulder elevation) were higher for the tablet compared to the laptop, suggesting that of the four devices tested in this study the postures during tablet use are potentially the most problematic for joints. Another notable finding was that right wrist ulnar deviation was greater using a tablet or laptop compared to using a desktop in standing. This finding suggests that using a tablet or laptop may be problematic for the wrist, as non-neutral wrist postures are associated with chronic musculoskeletal symptoms such as carpal tunnel syndrome [31]. The posture differences observed while using a tablet or laptop as compared to a desktop computer have the potential to lead to joint pain or muscle dysfunction, particularly if a person spends long periods using these devices.
Consistent with previous studies [4, 13, 17], this study found significantly greater neck flexion with tablet and laptop use for both the HN and HNT angles, compared to a desktop computer (Tables 2 and 3). Greater neck flexion while using the tablet and laptop computer is likely related to the position of the screen. Comparing screen positions between the four workstations, the tablet had the lowest screen position, being flat on the typing surface. The laptop screen was also low, being erected directly on top of the desk as it would normally be positioned, whereas the desktop screen was adjusted for each participant in relation to their eye level as per ergonomic guidelines [21]. Previous studies using a desktop computer have demonstrated greater neck flexion is associated with lower vertical height of the screen [32, 33], consistent with our finding of greater neck flexion using a tablet and laptop. However, tablets are often used in a variety of tablet positions and tilt angles [18], with some authors recommending a tilt angle of between 20 and 50 degrees [34]. It has also been previously shown that reading a book with it flat on a desk results in greater cervical erector spinae muscle activity [35]. This activity is somewhat analogous to using a tablet flat on a desk, and although the current study did not measure muscle activity, the significant increase in neck flexion during tablet use might be expected to increase muscle activity in the posterior muscles of the cervical spine. Together these findings suggest probable increased loading on cervical spine structures due to increased neck flexion during tablet and laptop use, supporting the development of strategies to limit or manage the use of these devices, for example, timed breaks or ergonomic guidelines for tablet and laptop use.
The current study reported the wrist was in extension and radial deviation when typing on any of the four devices (Table 1). Donoghue et al. [36] previously reported that the most frequently used wrist position while typing on a standard keyboard is 20° wrist extension and 20° wrist ulnar deviation. Our findings for wrist extension are consistent with this, however, we found the mean wrist position for our participants was in radial rather than ulnar deviation. This between-study difference is likely due to the kinematic modelling employed to calculate the 3D wrist joint angle and the methods used in reporting the wrist joint variables. Whereas Donoghue et al. [36] used only a four marker set to create a planar angle for reporting radial and ulnar deviation, the current study employed an eight marker set and the Cartesian coordinate system using an x,y,z Cardan sequence of rotation to calculate the radial/ulnar deviation angle. Furthermore, the current study calculated the mean joint angle over the entire one minute typing task, whereas the study by Donoghue et al. [36] reported the percentage of time that joints were in each position (radial or ulnar deviation), and excluded values from small time increments where the wrist was in extreme ranges (e.g.>50° any direction).
Greater wrist flexion and ulnar deviation occurred when using a laptop compared to a desktop in the current study, though the difference for right wrist ulnar deviation only reached significance for the standing desktop. While the keyboard was not propped up for this study, its design had approximately 5° of inclination when it was flat on the table, similar to the 5° to 7.5° built-in inclination in most commercial keyboards [37, 38]. Participants displayed less wrist extension when using a laptop and typing onto its flat surface compared to using a desktop where the keyboard had an inclination, supporting previous research that reported a 1° decrease in wrist extension for every 2° decrease in keyboard inclination [37]. As working with a downwardly tilted keyboard reduces wrist extension angles by up to 15° [37] and slightly reduces forearm muscle activity [39], it is postulated that typing on a laptop where the wrists are in a less extended position might place less pressure on the carpal tunnel than using a standard keyboard. However, this change in keyboard tilt to reduce wrist extension does not take into account the greater ulnar deviation observed during laptop use that may also affect carpal tunnel pressures.
Increased shoulder elevation during tablet and laptop use compared to a desktop computer in sitting or standing was observed in the current study, potentially suggesting increased muscle activity in the upper trapezius muscles in order to raise the shoulders. This is supported by findings from previous research demonstrating 3–5% greater upper trapezius muscle activity when reading a book placed flat on a desktop [35] – a task that may require a similar neck and head position to the tablet task in the current study – as compared to working with computer screen. Interestingly, when comparing the tablet to the laptop in the current study, only the left shoulder and not the right demonstrated increased elevation while using the tablet. This side-to-side variation may be related to the slight, though statistically significant, increased left neck lateral flexion observed during tablet use. Increased lateral flexion during tablet use is a new finding not reported in other studies, and may possibly relate to handedness, as 90% of participants were right handed. As participants assumed neck flexion and hovered over the tablet, their dominant right hand might have blocked their view of the screen if it was used more for typing, therefore, they may have had to tilt their head slightly to the left to allow better visualization of the screen, accompanied by an increase in left shoulder elevation. The findings for left shoulder elevation may have also been influenced by participants’ arm movement while using a mouse with the desktop workstations and moving between the mouse and keyboard.
4.1Limitations
The five-minute tasks participants completed in this study may not reflect a real working environment where individuals remain in postures for much longer time durations. The short length of the task may be one possible reason that no differences in postures were found between the sitting and standing desk conditions. A longer duration of study tasks would provide a more realistic work environment and should be considered in future research. Furthermore, different participants have various levels of English proficiency and computer literacy; therefore, the editing task may be very easy for some participants but difficult for others. This may possibly affect posture as the participant struggling with the task becomes more skilled as each subsequent workstation is evaluated. To minimise any learning effects of the task, we randomised the order of workstations for each participant and the within-subject design reduces confounding factors in the posture comparisons between workstations.
In the current study participants used the tablet with it placed flat on a desk rather than a participants preferred position, in order to minimise additional confounding factors between participants from variations in positions. This use of a tablet may not be as common as holding it on one’s lap, or propping it up, and future studies could investigate postural differences between these tablet positions. Postures during the use of smart phone were not included in the current study as the standardised typing task could not be performed on a smart phone. However, postures during smart phone use would be an important addition to future studies investigating the role of posture and neck pain.
5Conclusion
Using a tablet or laptop computer significantly alters posture, resulting in greater neck, elbow and wrist flexion, and shoulder elevation compared to a standard desktop computer. This suggests that tablet and laptop users should consider propping up their device, connecting to an external monitor, and/or reducing the time these devices are used to decrease exposure to poor neck postures. When comparing desktop computer use in sitting and standing, few differences were identified, suggesting the possible benefits of a standing desk may not be related to neck and upper limb posture as measured in the current study. These findings contribute to the evidence for postural differences when using different types of electronic devices.
Conflict of interest
None to report.
References
[1] | Household use of information technology, Australia, 2014-15. Catalogue No. 8146.0 [Internet]. 2016 [cited Februrary 2, (2017) ]. Available from http://www.abs.gov.au/ausstats/[email protected]/mf/8146.0. |
[2] | American’s internet access: 2000-2015: As internet use nears saturation for some groups, a look at patterns of adoption [Internet]. Pew Research Center; (2015) [cited March 20, 2017]. Available from: http://www.pewinternet.org//06/26/americans-internet-access-2000-2015/. |
[3] | Waersted M , Hanvold TN , Veiersted KB . Computer work and musculoskeletal disorders of the neck and upper extremity: A systematic review. BMC Musculoskelet Disord. (2010) ;11: :79. |
[4] | Werth A , Babski-Reeves K . Effects of portable computing devices on posture, muscle activation levels and efficiency. Appl Ergon. (2014) ;45: :1603–9. |
[5] | Mobile Consumer Survey 2015 – The Australian Cut [Internet]. Deloitte Touche Tohmatsu; (2015) [cited February 2, 2017]. Available from: http://landing.deloitte.com.au/rs/761-IBL-328/images/deloitte-au-tmt-mobile-consumer-survey-2015-291015.pdf. |
[6] | Hoy D , March L , Woolf A , Blyth F , Brooks P , Smith E , et al. The global burden of neck pain: Estimates from the Global Burden of Disease 2010 study. Ann Rheum Dis. (2014) ;73: :1309–15. |
[7] | March L , Smith EU , Hoy DG , Cross MJ , Sanchez-Riera L , Blyth F , et al. Burden of disability due to musculoskeletal (MSK) disorders. Best Pract Res Clin Rheumatol. (2014) ;28: :353–66. |
[8] | Szeto GP , Straker LM , O’Sullivan PB . A comparison of symptomatic and asymptomatic office workers performing monotonous keyboard work–2: Neck and shoulder kinematics. Man Ther (2005) ;10: :281–91. |
[9] | Berkhout AL , Hendriksson-Larsen K , Bongers P . The effect of using a laptop station compared to using a standard laptop PC on the cervical spine torque, perceived strain and productivity. Appl Ergon. (2004) ;35: :147–52. |
[10] | Bubric K , Hedge A . Differential patterns of laptop use and associated musculoskeletal discomfort in male and female college students. Work. (2016) ;55: :663–71. |
[11] | Chiou WK , Chou WY , Chen BH . Notebook computer use with different monitor tilt angle: Effects on posture, muscle activity and discomfort of neck pain users. Work. (2012) ;41: (Suppl 1):2591–5. |
[12] | Gold JE , Driban JB , Yingling VR , Komaroff E . Characterization of posture and comfort in laptop users in non-desk settings. Appl Ergon. (2012) ;43: :392–9. |
[13] | Szeto GP , Lee R . An ergonomic evaluation comparing desktop, notebook, and subnotebook computers. Arch Phys Med Rehabil. (2002) ;83: :527–32. |
[14] | Vasavada AN , Nevins DD , Monda SM , Hughes E , Lin DC . Gravitational demand on the neck musculature during tablet computer use. Ergonomics. (2015) ;58: :990–1004. |
[15] | Young JG , Trudeau MB , Odell D , Marinelli K , Dennerlein JT . Wrist and shoulder posture and muscle activity during touch-screen tablet use: Effects of usage configuration, tablet type, and interacting hand. Work. (2013) ;45: :59–71. |
[16] | Cook C , Burgess-Limerick R , Papalia S . The effect of upper extremity support on upper extremity posture and muscle activity during keyboard use. Appl Ergon. (2004) ;35: :285–92. |
[17] | Straker L , Jones KJ , Miller J . A comparison of the postures assumed when using laptop computers and desktop computers. Appl Ergon. (1997) ;28: :263–8. |
[18] | Young JG , Trudeau M , Odell D , Marinelli K , Dennerlein JT . Touch-screen tablet user configurations and case-supported tilt affect head and neck flexion angles. Work. (2012) ;41: :81–91. |
[19] | Babski-Reeves K , Calhoun A . Muscle activity and posture differences in the sit and stand phases of sit-to-stand workstation use: A comparison of computer configurations. IISE Transactions on Occupational Ergonomics and Human Factors. (2016) ;4: :236–46. |
[20] | Communications Report 2015-16 [Internet]. Commonwealth of Australia; (2016) [cited August 12, 2017]. Available from: www.acma.gov.au/commsreport. |
[21] | Rempel D , Barr A , Brafman D , Young E . The effect of six keyboard designs on wrist and forearm postures. Appl Ergon. (2007) ;38: :293–8. |
[22] | Office ergonomics guideline [Internet]. Queensland Government, Australia; (2013) [cited August 12, 2017]. Available from: http://education.qld.gov.au/health/pdfs/healthsafety/ergonomics-guide-pdf. |
[23] | Jonai H , Villanueva MBG , Takata A , Sotoyama M , Saito S . Effects of the liquid crystal display tilt angle of a notebook computer on posture, muscle activities and somatic complaints. Int J Ind Ergon. (2002) ;28: :219–29. |
[24] | Godin G . The Godin-Shephard Leisure-Time Physical Activity Questionnaire. Health & Fitness Journal of Canada. (2011) ;4: :18–22. |
[25] | Hanavan EP . A mathematical model for the human body. Ohio: Aerospace Medical Research Laboratories, Aerospace Medical Division, Air Force Systems Command; (1964) . pp. 64–102. |
[26] | Seay J , Selbie WS , Hamill J . In vivo lumbo-sacral forces and moments during constant speed running at different stride lengths. J Sports Sci. (2008) ;26: :1519–29. |
[27] | Ford KR , Myer GD , Hewett TE . Reliability of landing 3D motion analysis: Implications for longitudinal analyses. Med Sci Sports Exerc. (2007) ;39: :2021–8. |
[28] | Brink Y , Louw Q , Grimmer-Somers K . The quality of evidence of psychometric properties of three-dimensional spinal posture-measuring instruments. BMC Musculoskelet Disord. (2011) ;12: :93. |
[29] | Fernandes R , Armada-da-Silva P , Pool-Goudzwaard AL , Moniz-Pereira V , Veloso AP . Test-retest reliability and minimal detectable change of three-dimensional gait analysis in chronic low back pain patients. Gait Posture. (2015) ;42: :491–7. |
[30] | Whittle MW , Levine D . Measurement of lumbar lordosis as a component of clinical gait analysis. Gait Posture. (1997) ;5: :101–7. |
[31] | You D , Smith AH , Rempel D . Meta-analysis: Association between wrist posture and carpal tunnel syndrome among workers. Saf Health Work. (2014) ;5: :27–31. |
[32] | Straker L , Pollock C , Burgess-Limerick R , Skoss R , Coleman J . The impact of computer display height and desk design on muscle activity during information technology work by young adults. J Electromyogr Kinesiol. (2008) ;18: :606–17. |
[33] | Villanueva MB , Jonai H , Sotoyama M , Hisanaga N , Takeuchi Y , Saito S . Sitting posture and neck and shoulder muscle activities at different screen height settings of the visual display terminal. Ind Health. (1997) ;35: :330–6. |
[34] | Albin TJ , McLoone HE . The effect of tablet tilt angle on users’ preferences, postures, and performance. Work. (2014) ;47: :207–11. |
[35] | Straker L , Burgess-Limerick R , Pollock C , Coleman J , Skoss R , Maslen B . Children’s posture and muscle activity at different computer display heights and during paper information technology use. Hum Factors. (2008) ;50: :49–61. |
[36] | Donoghue MF , O’Reilly DS , Walsh MT . Wrist postures in the general population of computer users during a computer task. Appl Ergon. (2013) ;44: :42–7. |
[37] | Simoneau GG , Marklin RW . Effect of computer keyboard slope and height on wrist extension angle. Hum Factors. (2001) ;43: :287–98. |
[38] | Simoneau GG , Marklin RW , Monroe JF . Wrist and forearm postures of users of conventional computer keyboards. Hum Factors. (1999) ;41: :413–24. |
[39] | Simoneau GG , Marklin RW , Berman JE . Effect of computer keyboard slope on wrist position and forearm electromyography of typists without musculoskeletal disorders. Phys Ther. (2003) ;83: :816–30. |