The effect of mirror therapy can be improved by simultaneous robotic assistance
Abstract
Background:
Standard mirror therapy (MT) is a well-established therapy regime for severe arm paresis after acquired brain injury. Bilateral robot-assisted mirror therapy (RMT) could be a solution to provide visual and somatosensory feedback simultaneously.
Objective:
The study compares the treatment effects of MT with a version of robot-assisted MT where the affected arm movement was delivered through a robotic glove (RMT).
Methods:
This is a parallel, randomized trial, including patients with severe arm paresis after stroke or traumatic brain injury with a Fugl-Meyer subscore hand/finger < 4. Participants received either RMT or MT in individual 30 minute sessions (15 sessions within 5 weeks). Main outcome parameter was the improvement in the Fugl-Meyer Assessment upper extremity (FMA-UE) motor score. Additionally, the Motricity Index (MI) and the FMA-UE sensation test as well as a pain scale were recorded. Furthermore, patients’ and therapists’ experiences with RMT were captured through qualitative tools.
Results:
24 patients completed the study. Comparison of the FMA-UE motor score difference values between the two groups revealed a significantly greater therapy effect in the RMT group than the MT group (p = 0.006). There were no significant differences for the MI (p = 0.108), the FMA-UE surface sensibility subscore (p = 0.403) as well as the FMA-UE position sense subscore (p = 0.192). In both groups the levels of pain remained stable throughout the intervention. No other adverse effects were observed. The RMT training was well accepted by patients and therapists.
Conclusions:
The study provides evidence that bilateral RMT achieves greater treatment benefit on motor function than conventional MT. The use of robotics seems to be a good method to implement passive co-movement in clinical practice. Our study further demonstrates that this form of training can feasibly and effectively be delivered in an inpatient setting.
1Introduction
Hemiparesis is a frequent consequence of stroke or traumatic brain injury, and one of the most relevant predictors of long-term disability (Hendricks et al., 2002; Platz & Roschka, 2009). According to Wade et al. (1983) about 50% of stroke patients presenting with an initially flaccid arm in the subacute phase maintain the motor deficits six months post stroke. Kwakkel et al. (2003) further showed that only 12% of stroke patients manage to regain pre-stroke levels of arm functionality in the long term. Improving upper limb rehabilitation outcomes therefore remains a key challenge. This is especially so for those patients with severe arm paresis, not in the least because activities of daily living, such as washing or dressing are more difficult, which presents a barrier to independent living (Mayo et al., 2002).
Several therapeutic strategies for the rehabilitation of arm function have been proposed (Deutsche Gesellschaft für Neurorehabilitation, 2020). One of them, mirror therapy (MT), is a successful approach that is particularly useful for patients with severe arm paresis (Dohle et al., 2009; Pérez-Cruzado et al., 2017; Thieme et al., 2018). During MT patients move their unaffected limb next to a mirror so that the mirror image appears as if it were the affected limb. A range of studies has shown that the visual illusion of the affected hand moving drives the activation of the cortical, mainly contralateral, regions controlling the affected arm (Dohle et al., 2004; Dohle et al., 2011; Matthys et al., 2009; Michielsen et al., 2011). This neural activation supports the functional reorganization of the sensorimotor circuity damaged by the stroke, which in turn promotes improvements in affected arm motor control.
Mirror therapy can be performed in two different ways, the unilateral and the bilateral version respectively which differ in their clinical effect (Morkisch et al., 2019; Selles et al., 2014). In the unilateral version movements are only performed with the unaffected arm while the affected arm lies on the other side of the mirror passively. In the bilateral version the affected arm is actively involved in the movement exercises either through patients trying to move the affected arm themselves or the affected arm is moved passively with the aid of a therapist (Bae et al., 2012; Dohle et al., 2009; Michielsen et al., 2011; Miltner et al., 1999). We propose that the simultaneous activation of the affected arm representation, induced by the visual illusion and the actual attempt to move passively the affected arm, maximises the neuroplasticity mechanisms proposed to drive the treatment benefits. Through an additional sensory input it could be shown that especially the sensorimotor area and the superior temporal gyrus of the right hemisphere were activated (Gu et al., 2021). More effective ways of delivering bilateral MT therefore harbour the potential of greater treatment efficacy.
Theoretically a bilateral approach in MT has a good potential for better outcome. However, in practice it is not easily applied. Most patients with severe hemiparesis lack the degree of affected arm motor control necessary to engage in bilateral MT (Selles et al., 2014). The alternative, passive movement through a therapist, showed positive effects on affected arm motor skills (Miltner et al., 1999), but faces other challenges. Thus, the passive movement by the therapist must be performed as synchronously as possible (Dohle, 2012), because sizable deviations between the visual and the proprioceptive feedback can lead to irritations or pain (Fink et al., 1999; McCabe et al., 2005). Moreover, the desynchronization of visual and sensorimotor feedback results in desynchronised neural inputs which is likely to reduce adaptive neuroplastic changes, and hence treatment efficacy (Hummel & Cohen, 2005).
Robot-assisted mirror therapy (RMT) could be a solution to the challenges listed above because it provides visual and somatosensory feedback simultaneously and reliably. Robot-assisted therapies are increasingly finding their way into the treatment of neurological patients. They have the potential to improve the quality and intensity of rehabilitation treatments (Morone et al., 2020). Only few studies have investigated RMT so far (Beom et al., 2016; Kim et al., 2022). However, they have included mainly healthy people or patients with moderate upper limb impairment. Critically, the application had not been studied in a clinical setting. The present study was therefore designed to compare the treatment effects of unilateral MT with a version of bilateral MT where the unaffected arm movement was delivered through a robotic glove (RMT). Furthermore, patient and therapist experiences with RMT were captured through qualitative tools.
2Methods
2.1Trial design and Setting
The present study is a randomised controlled trial conducted at two inpatient neurorehabilitation facilities in Germany (Zentrum für Post-Akute Neurorehabilitation in Berlin; Aatalklinik, Bad Wünnenberg). The study protocol was approved by the local ethics committee (Ärztekammer Berlin) and registered with the German Register for Clinical Studies (DRKS00015893). Written informed consent of all participants was obtained prior to participation.
2.2Inclusion criteria and randomisation
Patients with acquired brain injury (stroke or traumatic brain injury) were screened for eligibility by the study team using the following inclusion criteria: (a) severe arm paresis with a Fugl-Meyer subscore hand/finger < 4, (b) Ashworth Spasticity Index < 3 in hand and finger joints, and (c) ability to follow therapist instructions. Exclusion criteria comprised (d) contractures in shoulder, elbow, hand or finger joints, (e) acute rheumatism, (f) advanced osteoarthritis in shoulder, elbow, hand or finger joints, (g) botulinum toxin injection in the affected arm less than three months prior to study enrolment.
The study procedure is shown in Fig. 1. Participants were randomly allocated to one of two groups, the robot-assisted mirror therapy group (RMT) or the mirror therapy group (MT). Randomisation took place in blocks of 4; group allocation was blinded via a predefined list available only to a person not involved in the study (secretary’s office).
Fig. 1
Study flow chart.
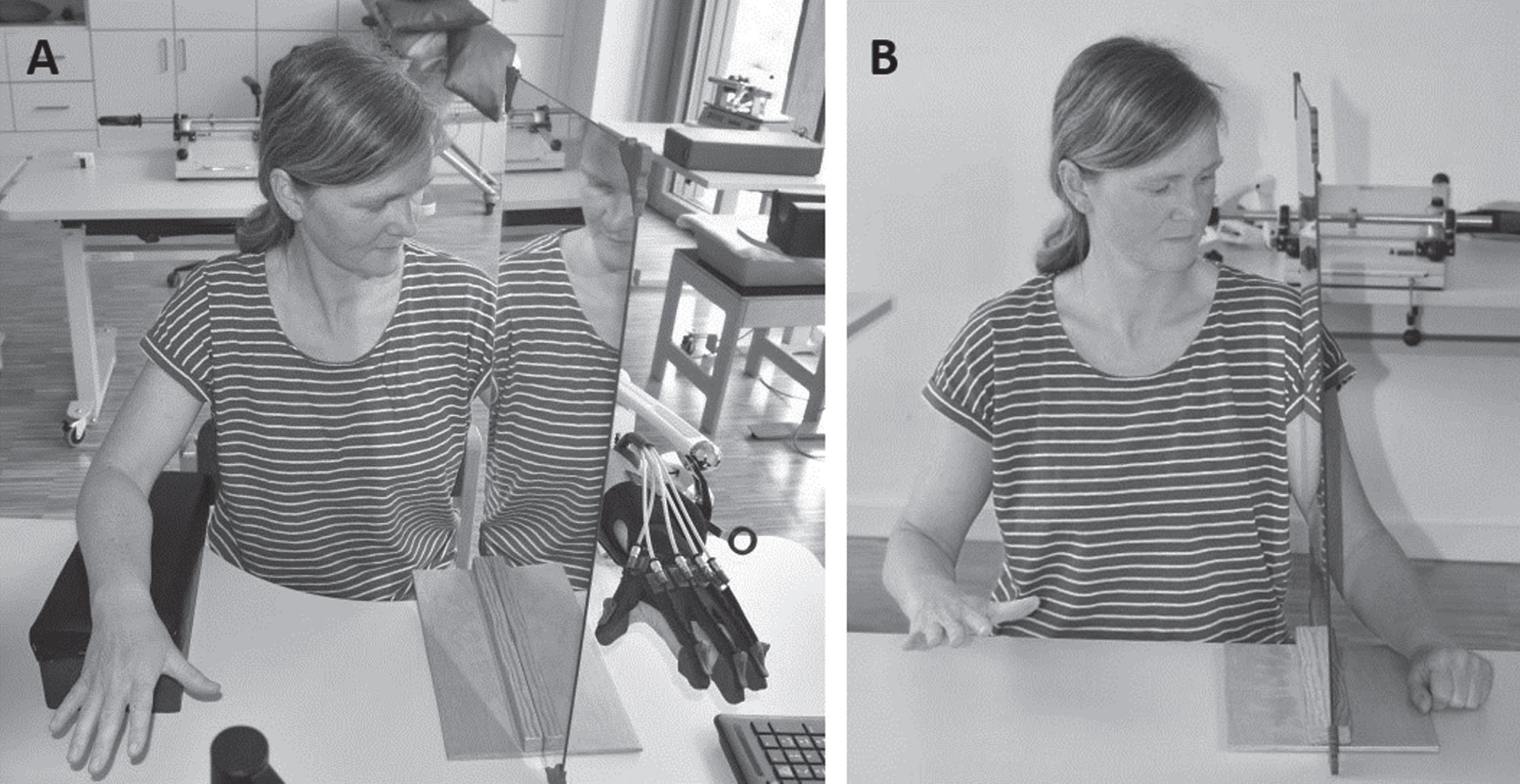
2.3Intervention
Patients received either RMT or MT in individual 30 minute sessions. 3–5 sessions were provided per week with the aim of completing 15 training sessions within 5 weeks. The therapists who carried out the intervention were properly trained and followed the standard operating procedures specified in the therapy manual.
In both therapy groups a mirror (40×60 cm) was placed in the patients’ midsagittal plane (Fig. 2). Patients were asked to move the unaffected arm so that its reflection in the mirror created the illusion of the affected arm moving. In both intervention groups participants performed three hand exercises for five minutes respectively: (a) open and close fist (b) single finger sequence where each finger is stretched and flexed individually and (c) finger-count from 1–5 then close to fist. The order of these exercises was varied across participants and sessions. Before every exercise, the task was practiced without a mirror for about one minute (approx. eight repetitions).
Fig. 2
(A) Setting of robotic assisted mirror therapy (RMT); (B) Setting of mirror therapy (MT).
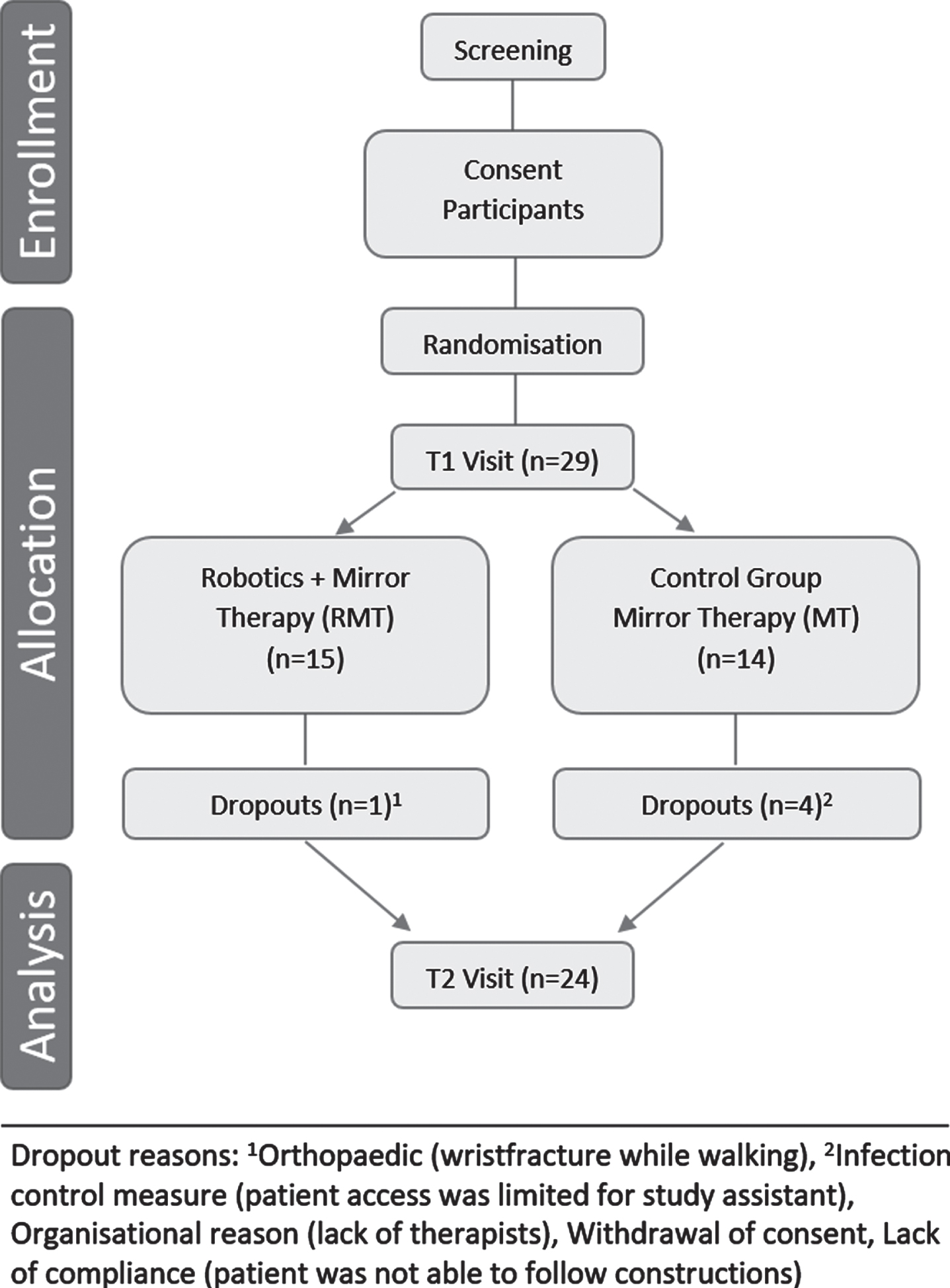
In the MT Group patients were asked to rest the affected arm comfortably behind the mirror (Fig. 2B). In the RMT group, the affected hand was also placed behind the mirror but moved passively by the robotic glove via a Bowden cable from the back of the hand to the finger caps (Gloreha, IDROGENET SRL, Lumezzane Italy; Fig. 2A). Patients were instructed by the therapist to synchronise the active unaffected hand movement with the passive movement of the affected arm delivered through the robotic glove. The robotic glove exerted a mechanical noise during its movement, this auditory feedback helped patients to keep movements of both arms synchronized.
During the intervention phase, patients continued to receive the standard neurorehabilitation care provided by the respective clinic. This included individual and group sessions in physiotherapy, sports therapy, occupational therapy, speech therapy and neuropsychology for about 12.5 hours per week. In the P.A.N. Zentrum, activities of daily living were instructed by neuropedagogic staff in the residential groups throughout the day. In the Aatal Clinic, activating care was provided by specially trained nursing staff.
2.4Outcome parameters
Outcome variables were measured before (T1) and after the 5-week intervention (T2). The Fugl-Meyer Assessment of the upper extremity (FMA-UE) motor score was used as the primary outcome parameter (Fugl-Meyer et al., 1975). Due to the assessments characteristics, reflex activity was not included in the survey (Woytowicz et al., 2017). The FMA-UE motor score without reflex items consists of 30 items, rated from 0–2 (0 = cannot be performed, 1 = can be partially performed, 2 = can be fully performed). Thus, the maximum FMA-UE motor score was 60 points (compared to 66 points in the original version).
Secondary variables included the FMA-UE sensation subtest (section H). Based on previous observations by Dohle et al. (2009) the values for FMA-UE surface sensibility and FMA-UE position sense were analysed separately. The FMA-UE surface sensibility included 2 items with a score of 0–2 (0 = anaesthesia 1 = hypaesthesia/dysaesthesia, 2 = normaesthesia) and FMA-UE position sense 4 items (0 = absence of sensation, 1 = considerable difference but at least 3/4 correct, 2 = all correct or little difference). We further acquired the Motricity Index (MI) (Demeurisse et al., 1980). The MI assesses the extent of paralysis (precision grip, elbow flexion, shoulder abduction) for the affected and the unaffected arm respectively. The maximum score is 100, which corresponds to full gross strength in the arm. Patients further rated their perceived pain on a 10-point rating scale (NRS; 0 = no pain, 10 = the worst pain imaginable) at T1 and T2, and after each session.
In the RMT group only, a feasibility questionnaire for patients and therapists was administered at T2. The questionnaire contained questions on the topics of set-up time, patients’ ability to synchronise, motivational aspects and intervention acceptance in the therapy setting. The questions were rated using a Likert scale.
2.5Statistical analysis
The outcome parameters were evaluated using SPSS Statistics 28.0 in a “per protocol” analysis for the time point after the 5-week intervention period. In order to examine statistical group differences of demographical variables and outcome parameters at T1, a T-Test, a Chi2-Test or Mann-Whitney-U (MWU) Test was performed depending on the presence of normal distribution (as assessed by the Kolmogorov-Smirnov test). Due to the small sample size, outcome parameters were analysed by non-parametric tests only. Post-pre differences were calculated for all FMA-UE subscores and the MI. Within-group changes were compared by the Wilcoxon Rank-Sum Test (WRST; paired samples) and between-groups by the MWU Test (unpaired samples). Furthermore, in order to determine if the time since incidence correlated with the change score, a Spearman correlation was calculated. For all statistical analysis, a significance level of 5% was set. The results of the pain scale and the questionnaire were analysed descriptively.
3Results
3.1Characteristics of patients
Between November 2018 and March 2020, 29 stroke patients were enrolled in the study. During the study, five drop-outs occurred (Fig. 1). As a result, 24 participants completed the study in accordance with the protocol and were included in the final “per protocol” analyses. Apart from gender distribution, the groups did not differ in demographic or health-related data at T1 (Table 1). Two extreme outliers were identified in the data (>3 times of the interquartile range of the differential value motor scores). However, these patients were not excluded from the analyses because time since injury was not significantly correlated to the primary outcome (FMA-UE motor function; Spearman’s rs = –0.361, p = 0.083). Furthermore a rank-based nonparametric procedure robust against outliers was used.
Table 1
Characteristics of patients to T1
RMT Group (n = 14) | MT Group (n = 10) | p-value | |||
Age | (M±SD) | 53,50±13,40 | 56,30±15,76 | 0.644 | (T-Test) |
Gender | (%) | ♀2 (14,3%); ♂12 (85,7%) | ♀7 (70,0%); ♂3 (30,0%) | 0.005** | (Chi2) |
Latency to brain damage month | (Md/Q1;Q3) | 13,13 [0,84; 17,00] | 13,64 [5,65;22,01] | 0.285 | (MWU) |
Diagnosis | 0.105 | (Chi2) | |||
Ichemic infarkt | (%) | 10 (71,4%) | 9 (90,0%) | ||
Intracerebral haemorrhage | (%) | 4 (28,6%) | – | ||
Subarachnoid haemorrhage | (%) | – | 1 (10,0%) | ||
FMA-UE motor function | (Md/Q1;Q3) | 4,00 [0,75;8,25] | 3,00 [0,00;4,50] | 0.358 | (MWU) |
FMA-UE surface sensibility | (Md/Q1;Q3) | 2,00 [0,75; 3,25] | 2,00 [2,00;2,50] | 0.546 | (MWU) |
FMA-UE position sense | (Md/Q1;Q3) | 4,00 [0,75;7,00] | 5,00 [2,5;8,00] | 0.472 | (MWU) |
Motricity Index | (Md/Q1;Q3) | 19,50 [1,00;30,25] | 5,50 [1,00;23,00] | 0.403 | (MWU) |
Pain Scale NRS-value | (Md/Q1;Q3) | 0,00 [0,00;0,00] | 0,00 [0,00;4,25] | 0.109 | (MWU) |
Abbreviations: RMT: Robotic-assisted mirror therapy; MT: mirror therapy; M: mean; SD: standard deviation; Md: median; Q: quartile; FMA-UE: Fugl-Meyer Assessment upper extremity; MWU: Mann-Whitney-U Test; **P < 0.01.
3.2Effects on motor function
Results are summarised in Tab. 2 and illustrated in Fig. 3. The pairwise comparison values showed a significant improvement of FMA-UE motor score for the RMT group (WRST: Z = –2,818, p = 0.005) but not for the MT group (WRST: Z = –0.647, p = 0.518). Analysis of the FMA-UE motor score difference values between the two groups revealed a significantly greater therapy effect in the RMT group than the MT group (MWU: U = 24.500, Z = –2.738, p = 0.006, r = –0.559). Spearman correlation between time since incidence and score chance showed no significant correlation (Spearman’s rs = –0.361, p = 0.083).
Table 2
Median change scores and interquartile range within groups for FMA-UE subscores and MI
RMT Group (n = 14) | MT Group (n = 10) | |||||
Initial (Tl) | Final (T2) | p-value (WRST) | Initial (Tl) | Final (T2) | p-value (WRST) | |
FMA-UE motor function (Md/Q1;Q3) | 4,00 [0,75;8,25] | 7,00 [3,00;1I,25] | 0.005** | 3,00 [0,00,4,50] | 1,50 [0,00;4,25] | 0.518 |
FMA-UE surface sensibility (Md/Q1;Q3) | 2,00 [0,75;3,25] | 2,00 [1,75;4,00] | 0.129 | 2,00 [2,00; 2,50] | 2,00 [2,00;2,25] | 0.655 |
FMA-UE position sense (Md/Q1;Q3) | 4,00 [0,75;7,00] | 5,50 [1,00;8,00] | 0.035* | 5,00 [2,50;8,00] | 4,00 [3,00;8,00] | 1.000 |
Motricity Index (Md/Q1;Q3) | 19,50 [1,00;30,25] | 32,00 [I3,75;40,25] | 0.007** | 5,50 [1,00; 23,00] | 12,5 [1,00;23,00] | 0.892 |
Abbreviations: RMT: Robotic-assisted mirror therapy; MT: mirror therapy; FMA-UE: Fugl-Meyer Assessment upper extremity; Md: median; Q: quartile; WRST: Wilcoxon Rank-Sum Test; *p < 0.05; **p < 0.01.
Fig. 3
Median change scores and interquartile range between groups for FMA-UE Motor Function (p = 0.006**) and Motricity Index (p = 0.108), p < 0.05.
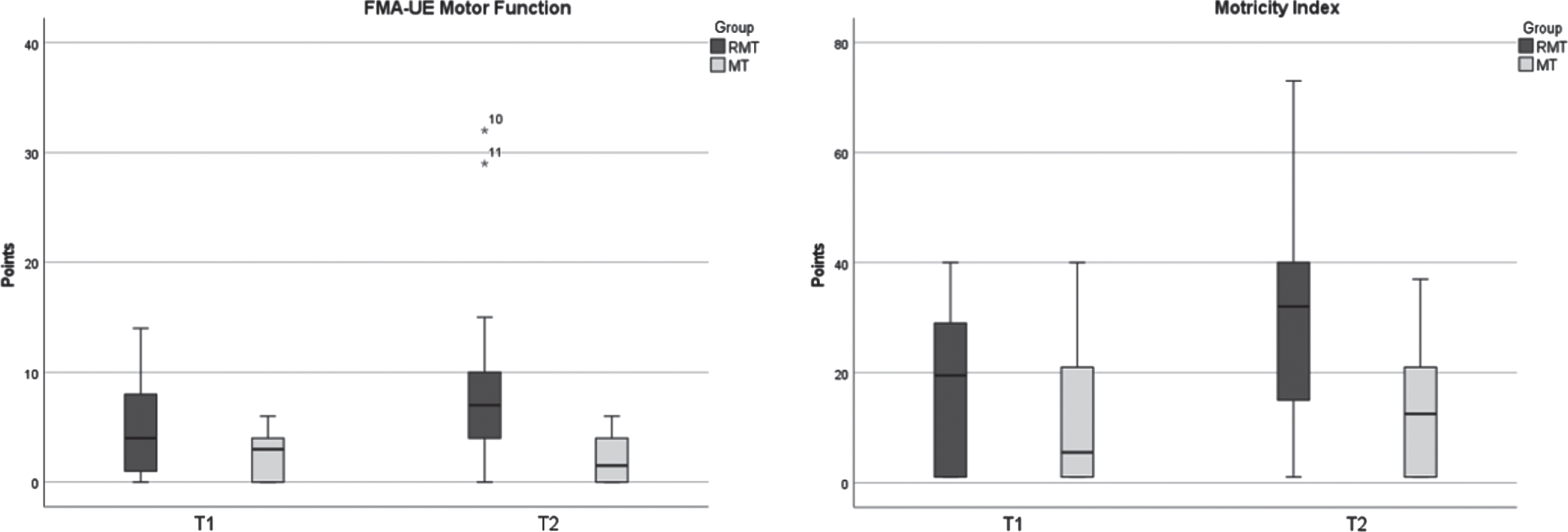
Pairwise comparison within groups of the MI revealed a statistically significant difference for the RMT group (WRST: Z = –2.675, p = 0.007), but not for the MT group (WRST: Z = 0.135, p = 0.892). Comparison of difference values for the MI between both groups revealed no significance (MWU: U = 42.500, Z = –1.673, p = 0.108, r = –0.341).
3.3Effects on sensory function
Regarding FMA-UE surface sensibility subscores, there were no significance differences within both groups (RMT WRST: Z = –1.518, p = 0.129; MT WRST: Z = –0.447, p = 0.655). There was also no significant difference between the two groups (MWU: U = 55.000, Z = –1.095, p = 0.403, r = –0.224).
For the FMA-UE position sense, the pairwise comparison showed a significant improvement for the RMT group (WRST: Z = –2.111, p = 0.035) but not for the MT group (WRST: Z = 0.000, p = 1.00.). Comparison between the two groups showed no statistical significance (MWU: U = 47.000, Z = –1.430, p = 0.192, r = –0,292).
3.4Effect on pain
With respect to pain, there were no major changes in either group observed. There were no statistical differences within the groups (RMT WRST: Z = –1.095, p = 0.273; MT WRST: Z = –1.633, p = 0.102). The difference values were also not significant between the two groups (MWU: U = 37.000, Z = –2.011, p = 0.109).
3.5RMT feasibility
10 participants of the RMT group and their associated therapists completed the questionnaires. The therapists rated the time needed to set up the therapy setting as “low (<7 min)” to “moderate (7–10 min)”. According to the therapists, more than half of the patients had no difficulties with synchronisation and were able to concentrate “well” to “very well” on the task. Only in two cases the therapists indicated that RMT was less suitable for the patient.
80% of all patients would like to integrate RMT into their therapy. Six patients from the RMT group were also familiar with standard MT from earlier stages in their recovery process. They reported that the additional use of robotics increased their motivation compared to the conventional MT. Five out of nine patients stated that their hand function had subjectively improved as a result of the therapy.
4Discussion
The present study was able to provide preliminary evidence that robot-assisted mirror therapy (RMT) can improve the effect of MT on hand motor function in severely affected patients. The combination of visual feedback through MT and the simultaneous somatosensory input on the affected side through robotics probably lead to a positive feedback loop. RMT uses two well-established hand rehabilitation procedures, the mirror illusion and the sensory input. The mirror illusion has already been proven to activate the contralateral hemisphere (Dohle et al., 2004; Dohle, Stephan et al., 2011; Matthys et al., 2009; Michielsen et al., 2011). It is generally assumed that this activation has an effect on functional motor recovery as many reviews have shown in stroke patients (Morkisch et al., 2019; Thieme et al., 2018; Yang et al., 2018; Zeng et al., 2018). Apart from the mirror illusion, RMT uses additional sensory input provided by robotics. This sensory input included proprioception triggered by the movement of the affected hand. Furthermore, surface sensitivity could be addressed through the wearing of the glove. Both stimuli could have a positive effect on motor function. Various somatosensory interventions are already in use to improve proprioception and motor function in stroke patients. These include passive and repeated cutaneous stimulation (Kattenstroth et al., 2018; Timm & Kuehn, 2020). Dechaumont-Palacin et al. (2008) were able to show that purely passive proprioceptive training for 4 weeks triggered fMRI changes in the ventral premotor and parietal cortex of the contralesional hemisphere in stroke patients. They hypothesise that through simple passive proprioception, increased contralesional activity in secondary sensorimotor areas positively influences motor function recovery (Dechaumont-Palacin et al., 2008). But even shorter robotic proprioceptive training can modulate plasticity in stroke patients (Vahdat et al., 2019). Kim et al. (2022) also demonstrated greater neural stimulation on the contralateral side of the motor cortex through bilateral robot-assisted training. Overall, both methods, mirror illusion and sensory input, show positive characteristics that are successfully combined in RMT.
Focusing of attention might be another mechanism contributing to this effect. Due to the design of the study, patients in the RMT group had to focus on the movements of the affected limb in order to synchronize their movements of the non-affected limb. This might have led to greater engagement with the task and hence enhanced processing of the sensorimotor stimulation. This idea is supported by animal studies on stimulation-induced neuroplasticity. Thus changes in S1 are strong when monkeys are rewarded for a tactile discrimination task associated with the tactile stimulation. However no S1 changes are observed when exactly the same tactile stimulation is given but the monkeys obtain their rewards in an auditory discrimination task, i.e. attention is diverted away from the sensory domain and the sensory stimulation is not behaviourally relevant (Recanzone et al., 1992). In our study, a few patients were overwhelmed temporarily with the complexity of the task and needed corrective instructions from the therapist. However, the results of these patients were not worse in comparison to those of the patients who had no difficulties with synchronisation. Patients with acquired brain damage often show deficits in attentional performance (Hyndman & Ashburn, 2003), so patients with a certain level of attention are more likely to benefit from this training. Therefore, only patients who were able to follow the instructions were included in the study. Whether the intervention is also suitable for patients with greater deficits in attentional performance remains open for now. Since MT is an active practice method, the patient’s motivation is another decisive factor. Without motivation and a willingness to concentrate on the mirror image, no MT can be performed meaningfully (Dohle et al., 2011).
However, not all motor outcomes showed clear trends. While the FMA-UE motor score showed significant results, these could not be confirmed in the MI. The MI for the upper extremity is a simple test consisting of 3 items. The authors assumed that the change sensitivity of this test is probably too low for patients with very severe arm paresis. Sunderland et al. (1989) already pointed out that particularly for patients in the chronic stage the sensitivity of MI to small improvements is very limited.
Furthermore, the effect of the combination of MT and robotic glove on FMA-UE sensation was investigated. It is hypothesised that patients with sensory deficits benefit from MT alone (Sathian et al., 2000). Thus, MT with the additional sensory input delivered by robotics was expected to map improvements in the two sensory domains. However, this assumption could not be confirmed since no significant improvements for FMA-UE surface sensibility were found for RMT. It is generally assumed that the perception of touch occurs at the point where it is performed. However, there are first indications in healthy people that a touch is perceived on the contralateral side when seen as a mirror image (Doyle et al., 2010), possibly based on immediate activation of the contralateral hemisphere (Fritzsch et al., 2014). This means that the use of the mirror alone could have triggered a change. An earlier study confirms that MT can increase surface sensibility in stroke patients (Dohle et al., 2009). In our study this could not be confirmed although there was an additional stimulus of the skin surface. For a more differentiated statement on the change in surface sensibility, a more sensitive method than the 2 items from the FMA-UE surface sensibility should be used in the future. Regarding the FMA-UE position sense there was also no significant difference between the two groups observed. There are differentiated statements on the trainability of position sense in stroke patients. Yeh et al. (2021) point to a good trainability of proprioception through robotics. In contrast, Doyle et al. (2010) could not identify any intervention in their review that had a positive influence on proprioception, not even the MT. RMT would have to be studied on a larger sample in order to be able to make more precise statements about the modification of proprioception.
In addition to the functional outcomes, the occurrence of pain was examined in both groups before and after the intervention block, and after every training session. These data suggest that pain levels remained unchanged throughout the MT and RMT training respectively. As a positive side effect according to therapists informal observations spasticity seems to be reduced immediately after the sessions. This is also noted by patients themselves.
In the present study, six patients in the RMT group had previous experience with MT. This made it possible to shed light on the motivational aspect of both therapy methods. Five of these patients stated that robotics had additionally motivated them. The therapists also rated the patients’ motivation as increased compared to conventional MT. Lack of motivation is often a barrier to physical activity after stroke (Nicholson et al., 2013). To what extend RMT helps patients to train their hand function in a motivated and committed manner is therefore an important future question.
A limitation of the study is the heterogeneity of the study participants. Due to the small number of subjects, no differentiated statements can be made about the effects in acute and chronic patients. In addition, some patients were not able to participate in the study due to cognitive limitations. Thus, a certain level of attentiveness is needed to meaningfully engage in the intervention and perform the unaffected arm movement in synchronisation with passive movement delivered by the robotic glove. An additional system in the form of a second glove, which is worn on the unaffected hand and transmits the movements directly to the affected hand via sensors, could provide a solution for patients with more limited cognitive abilities. Technical solutions are also being developed in other directions but they do not appear to be very practical at the moment (Ruggiu & Rea, 2022). Another limitation of the study is the measurement of purely motor functions within the treatment setting. The observation of functional changes in everyday life should be considered in further studies, as well as potential long-term effects.
Overall it has been shown that passive movement in combination with MT leads to additional clinical improvement, this is reflected on clinical scales. In this context, the use of robotics seems to be a good method to implement passive co-movement in clinical practice.
5Conclusion
The results of our study provide initial evidence that RMT with a bilateral approach achieves higher treatment effects on motor function than conventional MT. The combination of MT and the simultaneous somatosensory input, which can be enabled by a technical solution, seems to be the decisive factor for the enhanced treatment benefit seen in the RMT group. Furthermore, this form of training is well accepted by patients and therapists and is feasible to implement in an inpatient setting. Larger studies are needed to further confirm these conclusions.
Acknowledgments
This study was not supported by any grant. We would like to thank all therapists, especially Daniela Krohne and Evelyn Müller, for their help with data collection. We further express our gratitude to Tobias Strank for editorial assistance.
References
1 | Deutsche Gesellschaft für Neurorehabilitation.. ((2020) ). S3-Leitlinie “Rehabilitative Therapie bei Armparese nach Schlaganfall” der DGNRLangversion vom 01.06.2020. https://www.awmf.org/uploads/tx_szleitlinien/080-001l_S3_Rehabilitative_Therapie_bei_Armparese_nach_Schlaganfall_2020-07.pdf |
2 | Bae, S. H. , Jeong, W. S. & Kim, K. Y. ((2012) ). Effects of Mirror Therapy on Subacute Stroke Patients’ BrainWaves and Upper Extremity Functions. Journal of Physical Therapy Science, 24: (11), 1119–1122. https://doi.org/10.1589/jpts.24.1119 |
3 | Beom, J. , Koh, S. , Nam, H. S. , Kim, W. , Kim, Y. , Seo, H. G. , Oh, B.-M. , Chung, S. G. & Kim, S. ((2016) ). Robotic Mirror Therapy System for Functional Recovery of Hemiplegic Arms. Journal of Visualized Experiments : JoVE. https://doi.org/10.3791/54521 |
4 | Dechaumont-Palacin, S. , Marque, P. , Boissezon de, X. , Castel-Lacanal, E. , Carel, C. , Berry, I. , Pastor, J. , Albucher, J. F. , Chollet, F. & Loubinoux, I. ((2008) ). Neural correlates of proprioceptive integration in the contralesional hemisphere of very impaired patients shortly after a subcortical stroke: An FMRI study. Neurorehabilitation and Neural Repair, 22: (2), 154–165. https://doi.org/10.1177/1545968307307118 |
5 | Dohle, C. ((2012) ). Spiegeltherapie nach Schlaganfall. NeuroGeriatrie, (9), 71–78. |
6 | Dohle, C. , Kleiser, R. , Seitz, R. J. & Freund, H.-J. ((2004) ). Body scheme gates visual processing. Journal of Neurophysiology, 91: (5), 2376–2379. https://doi.org/10.1152/jn.00929.2003 |
7 | Dohle, C. , Morkisch, N. , Lommack, R. & Kadow, L. ((2011) ). Spiegeltherapie. Neuroreha, 3: (04), 184–190. https://doi.org/10.1055/s-0031-1295557 |
8 | Dohle, C. , Püllen, J. , Nakaten, A. , Küst, J. , Rietz, C. & Karbe, H. ((2009) ). Mirror therapy promotes recovery from severe hemiparesis: A randomized controlled trial. Neurorehabilitation and Neural Repair, 23: (3), 209–217. https://doi.org/10.1177/1545968308324786 |
9 | Dohle, C. , Stephan, K. M. , Valvoda, J. T. , Hosseiny, O. , Tellmann, L. , Kuhlen, T. , Seitz, R. J. & Freund, H.-J. ((2011) ). Representation of virtual arm movements in precuneus. Experimental Brain Research, 208: (4), 543–555. https://doi.org/10.1007/s00221-010-2503-0 |
10 | Doyle, S. , Bennett, S. , Fasoli, S. E. & McKenna, K. T. ((2010) ). Interventions for sensory impairment in the upper limb after stroke. The Cochrane Database of Systematic Reviews, 2010: (6). https://doi.org/10.1002/14651858.CD006331.pub2 |
11 | Fink, G. R. , Marshall, J. C. , Halligan, P. W. , Frith, C. D. , Driver, J. , Frackowiak, R. S. & Dolan, R. J. ((1999) ). The neural consequences of conflict between intention and the senses. Brain: A Journal of Neurology, 122: (Pt 3), 497–512. |
12 | Fritzsch, C. , Wang, J. , Dos Santos, L. F. , Mauritz, K.-H. , Brunetti, M. & Dohle, C. ((2014) ). Different effects of the mirror illusion on motor and somatosensory processing. Restorative Neurology and Neuroscience, 32: (2), 269–280. https://doi.org/10.3233/RNN-130343 |
13 | Fugl-Meyer, A. R. , Jääskö, L. , Leyman, I. , Olsson, S. & Steglind, S. ((1975) ). The post-stroke hemiplegic patient. 1. A method for evaluation of physical performance. Scandinavian Journal of Rehabilitation Medicine, 7: (1), 13–31. |
14 | Gu, P. , Tu, W. , Deng, F. , Ye, L. , Li, S. , Bai, G. , Jin, X. , Li, S. & Jiang, S. ((2021) ). Sequential neural activity in sensorimotor area and mirror neural system for graded mirror therapy with imagined hand movements. NeuroRehabilitation, 49: (4), 641–654. https://doi.org/10.3233/NRE-210185 |
15 | Hendricks, H. T. , van Limbeek, J. , Geurts, A. C. & Zwarts, M. J. ((2002) ). Motor recovery after stroke: A systematic review of the literature. Archives of Physical Medicine and Rehabilitation, 83: (11), 1629–1637. https://doi.org/10.1053/apmr.2002.35473 |
16 | Hummel, F. C. & Cohen, L. G. ((2005) ). Drivers of brain plasticity. Current Opinion in Neurology, 18: (6), 667–674. https://doi.org/10.1097/01.wco.0000189876.37475.42 |
17 | Hyndman, D & Ashburn, A. ((2003) ). People with stroke living in the community: Attention deficits, balance, ADL ability and falls. Disability and Rehabilitation, 25: (15), 817–822. https://doi.org/10.1080/0963828031000122221 |
18 | Kattenstroth, J. C. , Kalisch, T. , Sczesny-Kaiser, M. , Greulich, W. , Tegenthoff, M. & Dinse, H. R. ((2018) ). Daily repetitive sensory stimulation of the paretic hand for the treatment of sensorimotor deficits in patients with subacute stroke: Reset, a randomized, sham-controlled trial. BMC Neurology, 18: https://doi.org/10.1186/s12883-017-1006-z |
19 | Kim, D. H. , Lee, K.-D. , Bulea, T. C. & Park, H.-S. ((2022) ). Increasing motor cortex activation during grasping via novel robotic mirror hand therapy: A pilot fNIRS study. Journal of Neuro-Engineering and Rehabilitation, 19: . https://doi.org/10.1186/s12984-022-00988-7 |
20 | Kwakkel, G. , Kollen, B. J. , van der Grond, J. & Prevo, A. J. H. ((2003) ). Probability of regaining dexterity in the flaccid upper limb: Impact of severity of paresis and time since onset in acute stroke. Stroke, 34: (9), 2181–2186. https://doi.org/10.1161/01.STR.0000087172.16305.CD |
21 | Matthys, K. , Smits, M. , van der Geest, J. N. , van der Lugt, A. , Seurinck, R. , Stam, H. J. & Selles, R. W. ((2009) ). Mirror-induced visual illusion of hand movements: A functional magnetic resonance imaging study. Archives of Physical Medicine and Rehabilitation, 90: (4), 675–681. https://doi.org/10.1016/j.apmr.2008.09.571 |
22 | Mayo, N. E. , Wood-Dauphinee, S. , Côté, R. , Durcan, L. & Carlton, J. ((2002) ). Activity, participation, and quality of life 6 months poststroke. Archives of Physical Medicine and Rehabilitation, 83: (8), 1035–1042. https://doi.org/10.1053/apmr.2002.33984 |
23 | McCabe, C. S. , Haigh, R. C. , Halligan, P. W. & Blake, D. R. ((2005) ). Simulating sensory-motor incongruence in healthy volunteers: Implications for a cortical model of pain. Rheumatology (Oxford, England), 44: (4), 509–516. https://doi.org/10.1093/rheumatology/keh529 |
24 | Michielsen, M. E. , Selles, R. W. , van der Geest, J. N. , Eckhardt, M. , Yavuzer, G. , Stam, H. J. , Smits, M. , Ribbers, G. M. & Bussmann, J. B. J. ((2011) ). Motor recovery and cortical reorganization after mirror therapy in chronic stroke patients: A phase II randomized controlled trial. Neurorehabilitation and Neural Repair, 25: (3), 223–233. https://doi.org/10.1177/1545968310385127 |
25 | Miltner, R. , Simon, U. , Netz, J. & Hömberg, V. ((1999) ). Bewegungsvorstellung in der Therapie von Patienten mit Hirninfarkt. Neurologie & Rehabilitation (5), 66–72. |
26 | Morkisch, N. , Thieme, H. & Dohle, C. ((2019) ). How to perform mirror therapy after stroke? Evidence from a meta-analysis. Restorative Neurology and Neuroscience, 37: (5), 421–435. https://doi.org/10.3233/RNN-190935 |
27 | Morone, G. , Cocchi, I. , Paolucci, S. & Iosa, M. ((2020) ). Robotassisted therapy for arm recovery for stroke patients: State of the art and clinical implication. Expert Review of Medical Devices, 17: (3), 223–233. https://doi.org/10.1080/17434440.2020.1733408 |
28 | Nicholson, S. , Sniehotta, F. F. , van Wijck, F. , Greig, C. A. , Johnston, M. , McMurdo, M. E. T. , Dennis, M. & Mead, G. E. ((2013) ). A systematic review of perceived barriers and motivators to physical activity after stroke. International Journal of Stroke : Official Journal of the International Stroke Society, 8: (5), 357–364. https://doi.org/10.1111/j.1747-4949.2012.00880.x |
29 | Pérez-Cruzado, D. , Merchán-Baeza, J. A. , González-Sánchez, M. & Cuesta-Vargas, A. I. ((2017) ). Systematic review of mirror therapy compared with conventional rehabilitation in upper extremity function in stroke survivors. Australian Occupational Therapy Journal, 64: (2), 91–112. https://doi.org/10.1111/1440-1630.12342 |
30 | Platz, T. & Roschka, S. ((2009) ). Rehabilitative Therapie bei Armparese nach Schlaganfall. Neurologie & Rehabilitation, 15: (2), 81–106. |
31 | Recanzone, G. H. , Merzenich, M. M. , Jenkins, W. M. , Grajski, K. A. & Dinse, H. R. ((1992) ). Topographic reorganization of the hand representation in cortical area 3b owl monkeys trained in a frequency-discrimination task. Journal of Neurophysiology, , 67: (5). https://doi.org/10.1152/jn.1992.67.5.1031 |
32 | Ruggiu, M. & Rea, P. ((2022) ). Development of a Mechatronic System for the Mirror Therapy. Actuators, 11: (1), 14. https://doi.org/10.3390/act11010014 |
33 | Sathian, K. , Greenspan, A. I. & Wolf, S. L. ((2000) ). Doing it with mirrors: A case study of a novel approach to neurorehabilitation. Neurorehabilitation and Neural Repair, 14: (1), 73–76. https://doi.org/10.1177/154596830001400109 |
34 | Selles, R. W. , Michielsen, M. E. , Bussmann, J. B. J. , Stam, H. J. , Hurkmans, H. L. , Heijnen, I. , Groot de, D. & Ribbers, G. M. ((2014) ). Effects of a mirror-induced visual illusion on a reaching task in stroke patients: Implications for mirror therapy training. Neurorehabilitation and Neural Repair, 28: (7), 652–659. https://doi.org/10.1177/1545968314521005 |
35 | Sunderland, A. , Tinson, D. , Bradley, L. & Hewer, R. L. ((1989) ). Arm function after stroke. An evaluation of grip strength as a measure of recovery and a prognostic indicator. Journal of Neurology, Neurosurgery, and Psychiatry, 52: (11), 1267–1272. https://doi.org/10.1136/jnnp.52.11.1267 |
36 | Thieme, H. , Morkisch, N. , Mehrholz, J. , Pohl, M. , Behrens, J. , Borgetto, B. & Dohle, C. ((2018) ). Mirror therapy for improving motor function after stroke. The Cochrane Database of Systematic Reviews, 7: , CD008449. https://doi.org/10.1002/14651858.CD008449.pub3 |
37 | Timm, F. & Kuehn, E. ((2020) ). A Mechanical Stimulation Glove to Induce Hebbian Plasticity at the Fingertip. Frontiers in Human Neuroscience, 14: , 177. https://doi.org/10.3389/fnhum.2020.00177 |
38 | Vahdat, S. , Darainy, M. , Thiel, A. & Ostry, D. J. ((2019) ). A Single Session of Robot-Controlled Proprioceptive Training Modulates Functional Connectivity of Sensory Motor Networks and Improves Reaching Accuracy in Chronic Stroke. Neurorehabilitation and Neural Repair, 33: (1), 70–81. https://doi.org/10.1177/1545968318818902 |
39 | Wade,D. T., Langton-Hewer,R., Wood,V. A., Skilbeck,C. E., & Ismail,H. M. ((1983) ). The hemiplegic arm after stroke: measurement and recovery. Journal of Neurology, Neurosurgery & Psychiatry, 46: (6), 521–524. https://doi.org/10.1136/jnnp.46.6.521 |
40 | Woytowicz, E. J. , Rietschel, J. C. , Goodman, R. N. , Conroy, S. S. , Sorkin, J. D. , Whitall, J & McCombe Waller, S. ((2017) ). Determining Levels of Upper Extremity Movement Impairment by Applying a Cluster Analysis to the Fugl-Meyer Assessment of the Upper Extremity in Chronic Stroke. Archives of Physical Medicine and Rehabilitation, 98: (3), 456–462. https://doi.org/10.1016/j.apmr.2016.06.023 |
41 | Yang, Y. , Zhao, Q. , Zhang, Y. , Wu, Q. , Jiang, X. & Cheng, G. ((2018) ). Effect of Mirror Therapy on Recovery of Stroke Survivors: A Systematic Review and Network Meta-analysis. Neuroscience, 390: , 318–336. https://doi.org/10.1016/j.neuroscience.2018.06.044 |
42 | Yeh, I.-L. , Holst-Wolf, J. , Elangovan, N. , Cuppone, A. V. , Lakshminarayan, K. , Capello, L. , Masia, L. & Konczak, J. ((2021) ). Effects of a robot-aided somatosensory training on proprioception and motor function in stroke survivors. Journal of NeuroEngineering and Rehabilitation, 18: . https://doi.org/10.1186/s12984-021-00871-x |
43 | Zeng, W. , Guo, Y. , Wu, G. , Liu, X. & Fang, Q. ((2018) ). Mirror therapy for motor function of the upper extremity in patients with stroke: A meta-analysis. Journal of Rehabilitation Medicine, 50: (1), 8–15. https://doi.org/10.2340/16501977-2287 |