Design of a horizontally dynamic armrest for joystick controlled mobile equipment
Abstract
BACKGROUND:
Joystick operators often experience constant low level muscle loading in the upper limb when using joysticks, which can lead to repetitive strain injuries. It has been hypothesized that the strain on the muscles may be reduced by supporting the arm during joystick manipulation.
OBJECTIVE:
To design a horizontally dynamic armrest which appropriately supports the motion of the forearm during side-to-side joystick movements.
METHODS:
The paper describes the process used to design a dynamic armrest which appropriately supports an operator’s arm throughout the range of side-to-side joystick movements.
RESULTS:
The trajectory of the elbow and wrist during these joystick movements was determined, and a new horizontally dynamic armrest designed and built.
CONCLUSIONS:
Most heavy mobile equipment seat armrests fail to provide appropriate support throughout the range of joystick motion resulting in constant upper body muscle loading. Our paper describes the development of a dynamic armrest which is designed to provide appropriate support throughout side-to-side joystick movements.
1.Introduction
Operators of joystick controlled mobile machines often experience repetitive loading on the arm and shoulder musculature. Several studies have reported constant low level loading at or above 2% maximum voluntary contraction (MVC) in the upper trapezius during light repetitive tasks [1, 2, 3]. It has been shown that constant low level muscle loading for long periods of time may lead to repetitive strain injuries [4].
The operation of joystick controlled mobile machines involves making small amplitude, repetitive arm movements [5]. It has been reported that joystick operators in the forestry industry can make as many as 20,000 joystick movements over a 10 hour work day [6], and operators can be working hand operated controls for up to 95% of their working hours in logging machines [7]. A study by Axelsson and Ponten [8] on logging forwarder, processor and harvester operators showed that neck and upper arm pain could be linked to one-sided, short repetitive movements. Unfortunately, no further detail was given as to the nature of the controls, other than that they required one-sided, repetitive, short cycle movements of the arms and hands. A literature review conducted by van der Windt et al. [9] concluded that potential risk factors for arm and shoulder pain include work load, awkward postures, repetitive movements, and duration. The short, repetitive movements made by joystick operators could therefore lead to shoulder, arm, and neck pain.
There are several muscles involved in making the repetitive movements necessary for joystick control. Oliver et al. [10] investigated the effects of joystick stiffness and speed on eight upper limb and shoulder muscles. The primary muscles involved in each direction of joystick movement can be seen in Table 1. It was also determined that each joystick motion required constant low level loading between 2–5% task based MVC for all muscles investigated.
Table 1
Muscles involved in four joystick movement directions [10]
Joystick movement stage | ||
---|---|---|
Neutral to hard endpoint | Hard endpoint to neutral | |
Forward | Anterior deltoid, extensor carpi radialis, triceps | Upper trapezius, posterior deltoid |
Back | Upper trapezius, posterior deltoid, pectoralis major | Variety, at low levels |
Inwards | Flexor carpi radialis, pectoralis major | Extensor carpi radialis |
Outwards | Extensor carpi radialis, pectoralis major | Flexor carpi radialis, pectoralis major |
It has been hypothesized that the constant low level loading on muscles during joystick manipulation could lead to RSIs [4], and other musculoskeletal problems [7, 11]. To reduce the loading on the upper limb and possibly the prevalence of musculoskeletal injury in operators of large mobile machines, researchers have investigated a variety of joystick designs. Lindbeck (1985), as reported by [7] investigated the effects of using control levers with three resistance ranges on muscle activation in four shoulder and neck muscles, the upper trapezius (UT), anterior deltoid (AD), medial deltoid (MD), and posterior deltoid (PD). It was determined that changes in joystick resistance affected the PD and MD muscles the most, and had a smaller effect on the UT and AD. However, a similar study showed that joystick stiffness had no effect on the PD and UT, but did have an effect on prime mover muscles including the AD [10]. Although the results of these studies are conflicting, both studies show the UT was not affected by joystick stiffness. This is likely because the UT is a stabilizing muscle and not a prime mover during joystick control. Altering joystick stiffness is one way to reduce muscle activation, but alternative joystick designs have also been investigated.
Both pronated joysticks and mini levers have been investigated as alternatives to conventional joysticks. The use of pronated joysticks has been linked to increased shoulder pain, neck pain, and sick leave [11, 12]. Using a forestry machine simulator, Asikainen and Harstela [13] compared muscle activity when using mini levers with conventional control levers. Trapezius muscle activity was reduced while using the smaller lever, which was likely a result of the smaller range of motion required by the operator. It was also found that after forestry machine operators adopted the mini lever there were less instances of work related shoulder and neck pain. However, in another study the UT activation increased when operators performed a precision task in comparison to a simple task [1]. Although using a mini lever can reduce the stabilization requirements on the UT, it can also increase activation due to increased precision requirements. As an alternative to control re-designs, some researchers have chosen to investigate the arm supports. It has been hypothesized that the use of an armrest would help to stabilize the arm, and thus reduce the need for UT activation.
Stationary armrests have been investigated to determine if they could reduce muscle activation in operators completing repetitive tasks; however, studies investigating this have been equivocal in their results. One study found that using an armrest in addition to other ergonomic workplace improvements such as changing from a standing to sitting posture, and workspace height adjustments reduced muscle activation in the UT [14, 15]. On the other hand, Lindbeck (1982), as reported by Hansson [7] found no significant difference between shoulder and neck muscle activity when comparing a stationary armrest with no armrest. Although not significantly different, there was a reduction in UT muscle activation while Participants used the armrest for all joystick movement directions except backwards. This is likely because stationary armrests are designed to support a stationary arm, not a moving arm. During joystick manipulation, the arm lifts off a stationary armrest during forward, left, and right movements, and the arm support impedes the downward movement of the elbow during backward joystick movements [16].
Though it has been unclear whether stationary armrests are beneficial, it has been hypothesized that dynamic armrests could reduce muscle activity during joystick use. A dynamic armrest could support the arm throughout the entire range of motion of the arm while performing work related tasks. A study by Attebrant et al. [1] investigated a fore-aft dynamic armrest in comparison with a stationary armrest. EMG was recorded for the UT, infraspinatus, extensor carpi ulnaris (ECU) and flexor carpi radialis (FCR). Participants performed a targeting task simulating crane operation, and a tracking task simulating truck driving movement patterns. The UT muscle activation decreased while the FCR and ECU activation increased when using the translating armrest in comparison to a stationary armrest. When surveyed, six out of eight Participants preferred the translating armrest. The increase in the forearm muscle activation (i.e., the FCR and ECU) could have been because the armrest translated in only the fore-aft direction, and the tasks performed involved side-to-side joystick movements as well as fore-aft. The forearm muscles flex and extend the wrist and are prime movers in side-to-side joystick movement [10].
Building on work by Attebrant et al. [1], another fore-aft dynamic armrest was designed and validated [16, 17]. The motion of the elbow and wrist was tracked during fore-aft joystick manipulation without an armrest, and a dynamic armrest was designed to mimic this movement. The final design accounted for fore-aft translation as well as the vertical movement of the elbow (the elbow rises during forward joystick movement and lowers during backward joystick movement). The design was tested in a lab setting while participants performed forward and backward joystick movements. Surface EMG data were collected from the UT, PD and AD muscles [18]. The muscle activity in all three muscles was reduced when using the dynamic armrest in comparison to a stationary armrest and no armrest. Assuming that muscle activation would be reduced when the forearm was appropriately supported during side-to-side joystick movements, the purpose of the current work was to develop a horizontally dynamic armrest addition to support the arm during side-to-side joystick movements that could be added to the fore-aft dynamic armrest developed by [16].
2.Methods
To design the horizontally dynamic armrest addition, forearm trajectories during side-to-side joystick movements were determined for three participants (one female and two males; 24
Figure 1.
Participant seated in a laboratory mock-up of an excavator cab.
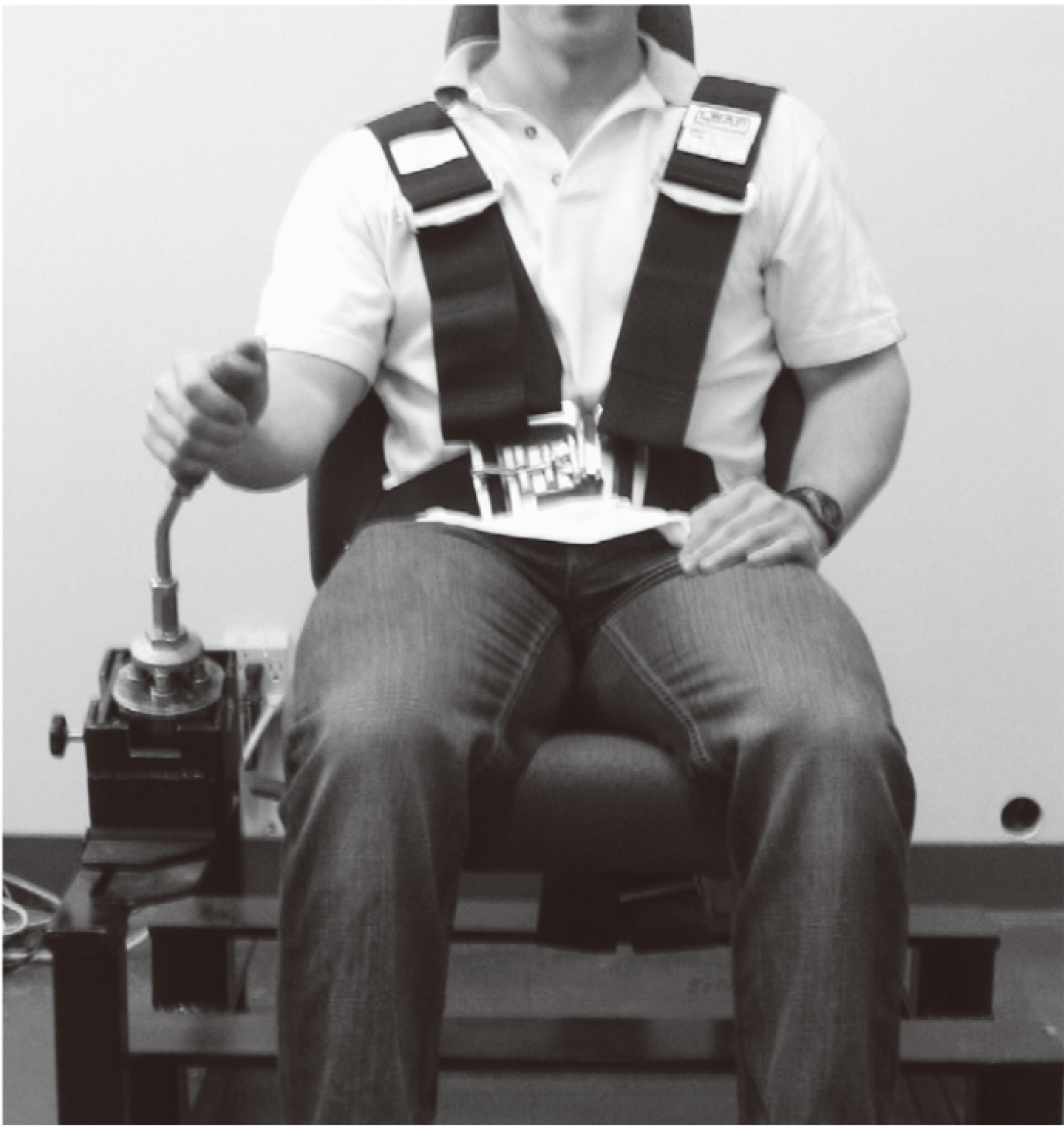
2.1Kinematic data collection
Data were collected using a six camera Vicon 460 motion capture system (Vicon Peak, Oxford, UK) while participants performed side-to-side joystick movements. Five retro-reflective markers were placed on the participant’s right shoulder, elbow, wrist, and middle knuckle, as well as on the joystick to monitor wrist and elbow displacement. Kinematic data were sampled at a rate of 250 Hz.
2.2Test protocol
Participants performed side-to-side joystick movements while the forearm was unsupported. Two repetitions were performed of inward and outward joystick movements. Each trial started with the joystick in the neutral position, then moved to the inward endpoint, then immediately to the outward endpoint, and back to neutral (Fig. 2). Participants were instructed to perform the joystick movements at a moderate, comfortable speed.
Figure 2.
Joystick movement directions.
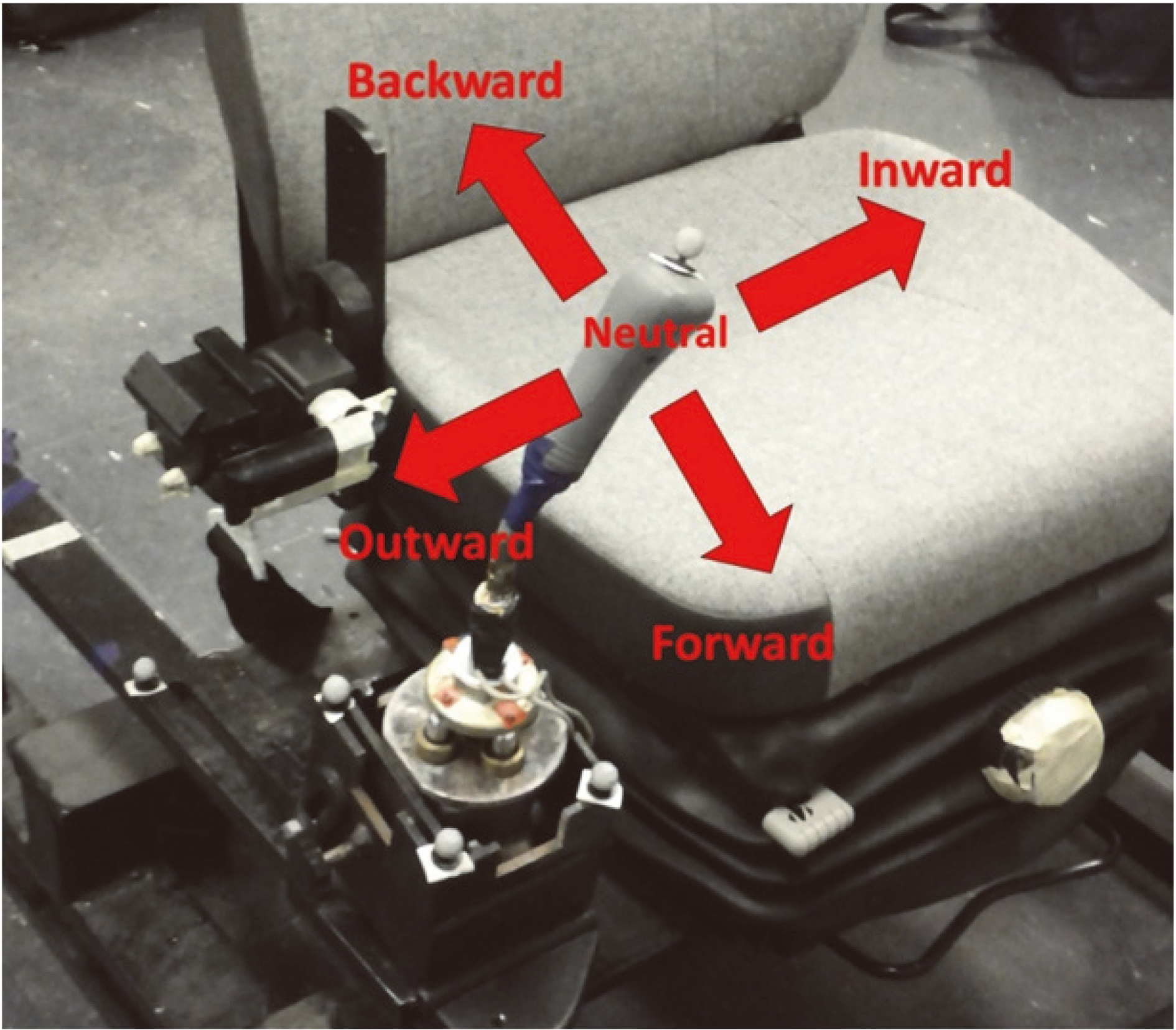
Kinematic data were analyzed using custom Matlab programs (Matlab version 7.8, The MathWorks Inc., Natick, MA). Data were cleaved using the x-displacement of the joystick marker (i.e., side-to-side movement in the frontal plane) so that the data represented the joystick movement starting and ending in a neutral position. Each trial represented movement from the neutral joystick position to the inwards endpoint, then to the outwards endpoint, and back to neutral. Elbow and wrist marker movement ranges in each direction (x, y, and z) were determined for each participant by finding the maximum and minimum x, y, and z displacement values for each marker. The trials were then normalized to the same length and averaged to show the average movement trajectory.
Figure 3.
Wrist marker displacement in the x-y plane during side-to-side joystick movement, mean and standard deviation for three participants (the solid black line represents the quadratic curve fit). The blue solid and dashed lines are the
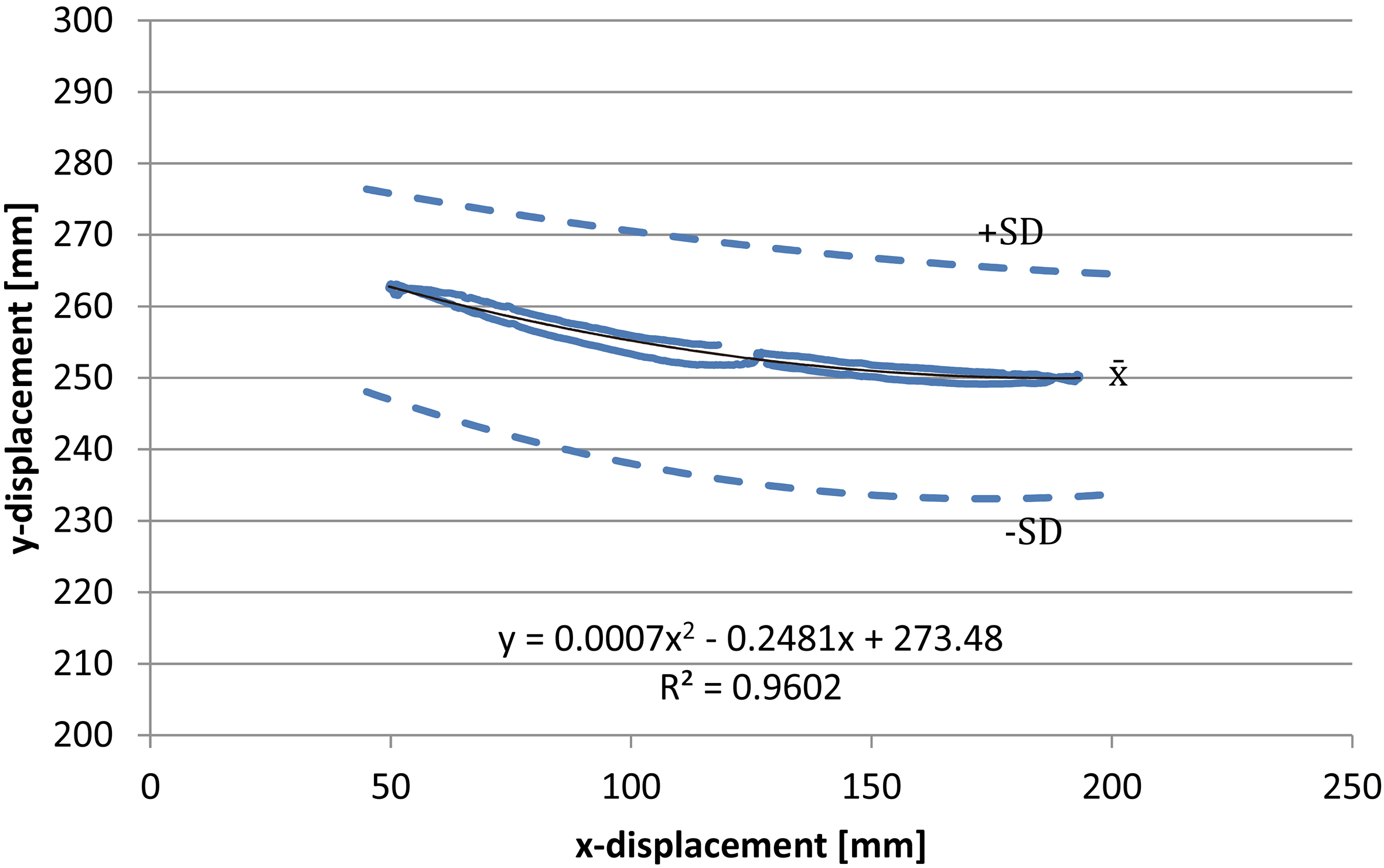
Figure 4.
Wrist marker displacement in the x-z plane during side-to-side joystick movement, mean and standard deviation for three participants (the solid black line represents the quadratic curve fit). The blue solid and dashed lines are the
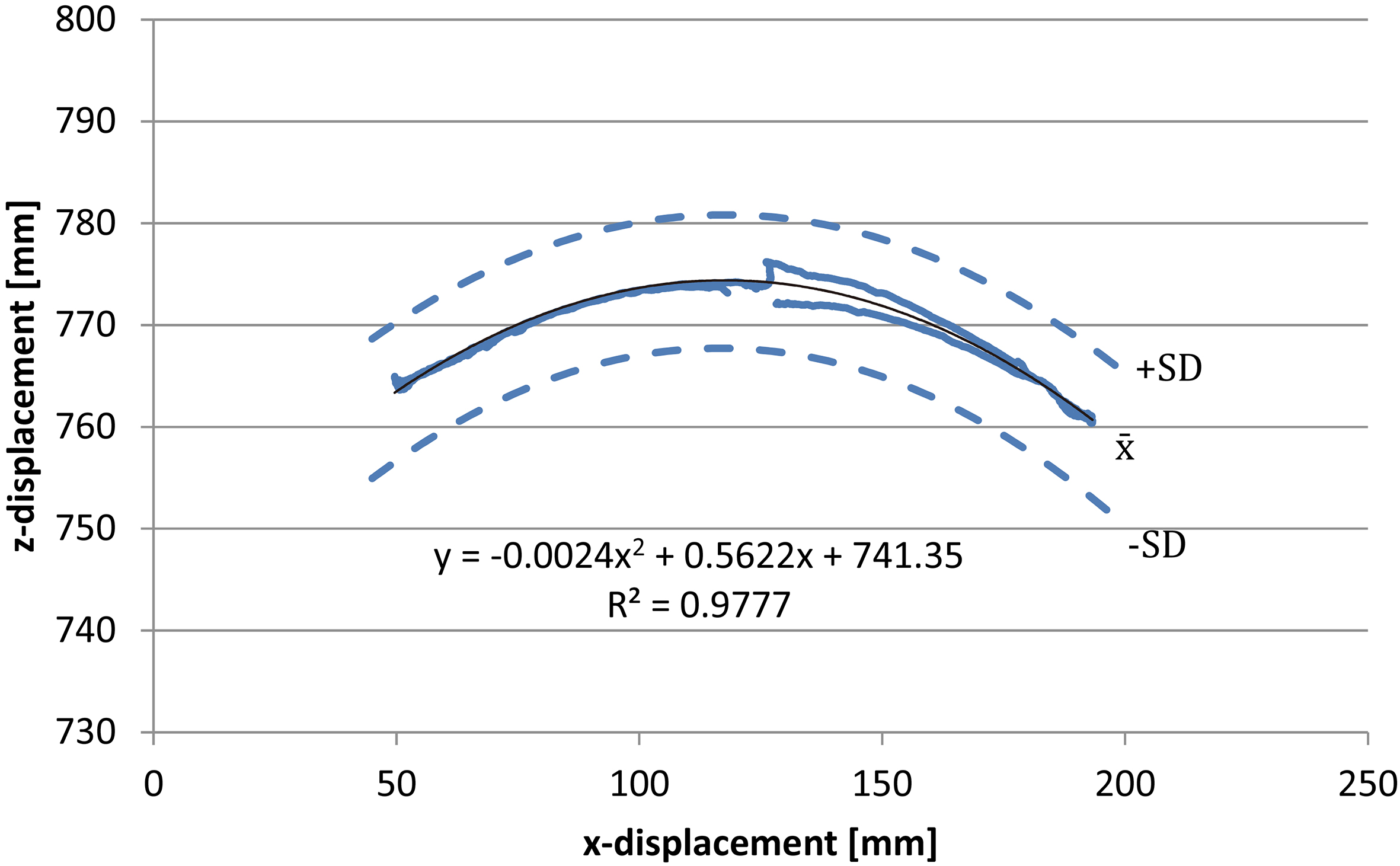
3.Results
The final wrist trajectories in the x-y and x-z planes were plotted (Figs 3 and 4). One trial for participant three was eliminated because off-axis joystick movement was observed. The results from the forearm trajectory pilot work showed that the wrist movement during side-to-side joystick manipulation occurred mostly in the x-axis (i.e., horizontally, with side-to-side joystick movement). Wrist displacement range in the x-axis was 145.6
The elbow displacement ranges were found to be small for the x, y, and z axes (30.7
Figure 5.
Horizontally dynamic armrest assembly drawing.
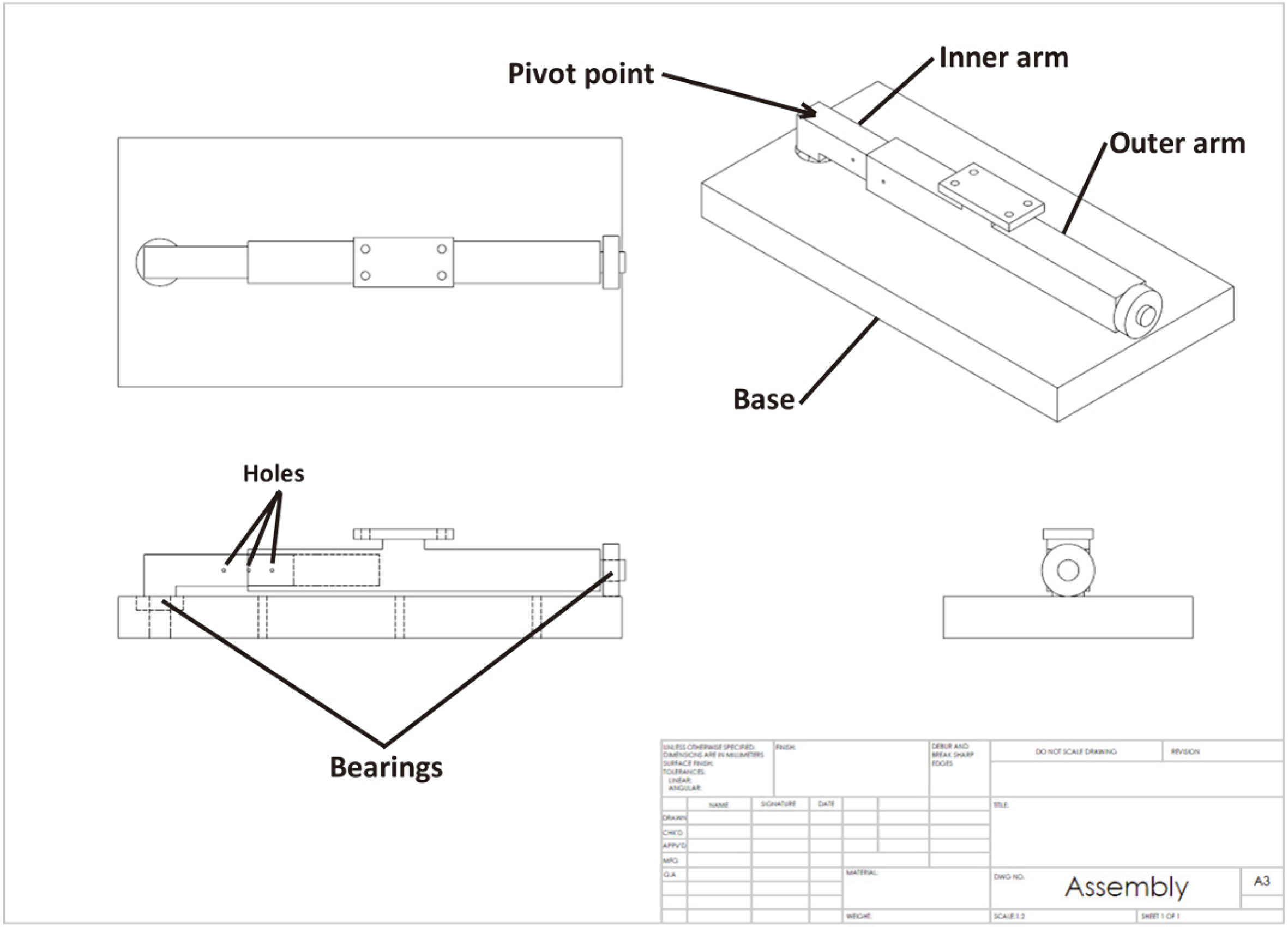
3.1Design outcome
Following forearm trajectory determination, an addition was designed for the original dynamically movable armrest. The addition did not affect the functionality of the original dynamic armrest in the fore-aft direction. It allowed the armrest to rotate in the transverse plane about a z-axis (vertical) pivot point located below the operator’s elbow, thereby supporting side-to-side joystick movements. The dynamic armrest design consisted of the following three parts: the base, inner arm, and outer arm. An assembly drawing of all three parts can be seen in (Fig. 5). Parts were machined from 6061 aluminum and two bearings were used. Three holes were machined into the inner arm to allow the arm to be shortened or lengthened based on the stature of the operator. The hole locations were determined based on 5th, 50th, and 95th %ile male forearm lengths [19]. A cotter pin could be inserted to hold the arm length in any of the three locations. Aluminum stops were also added to the final design to stop the front bearing from sliding off the base. The final product was fixed to the base of the original dynamic armrest as shown in Fig. 6.
Figure 6.
Final product, horizontally dynamic armrest with fore-aft dynamic armrest mounted above. Top figure – Side view; Bottom figure – Front view – from left to right – outward, neutral, inward locations.
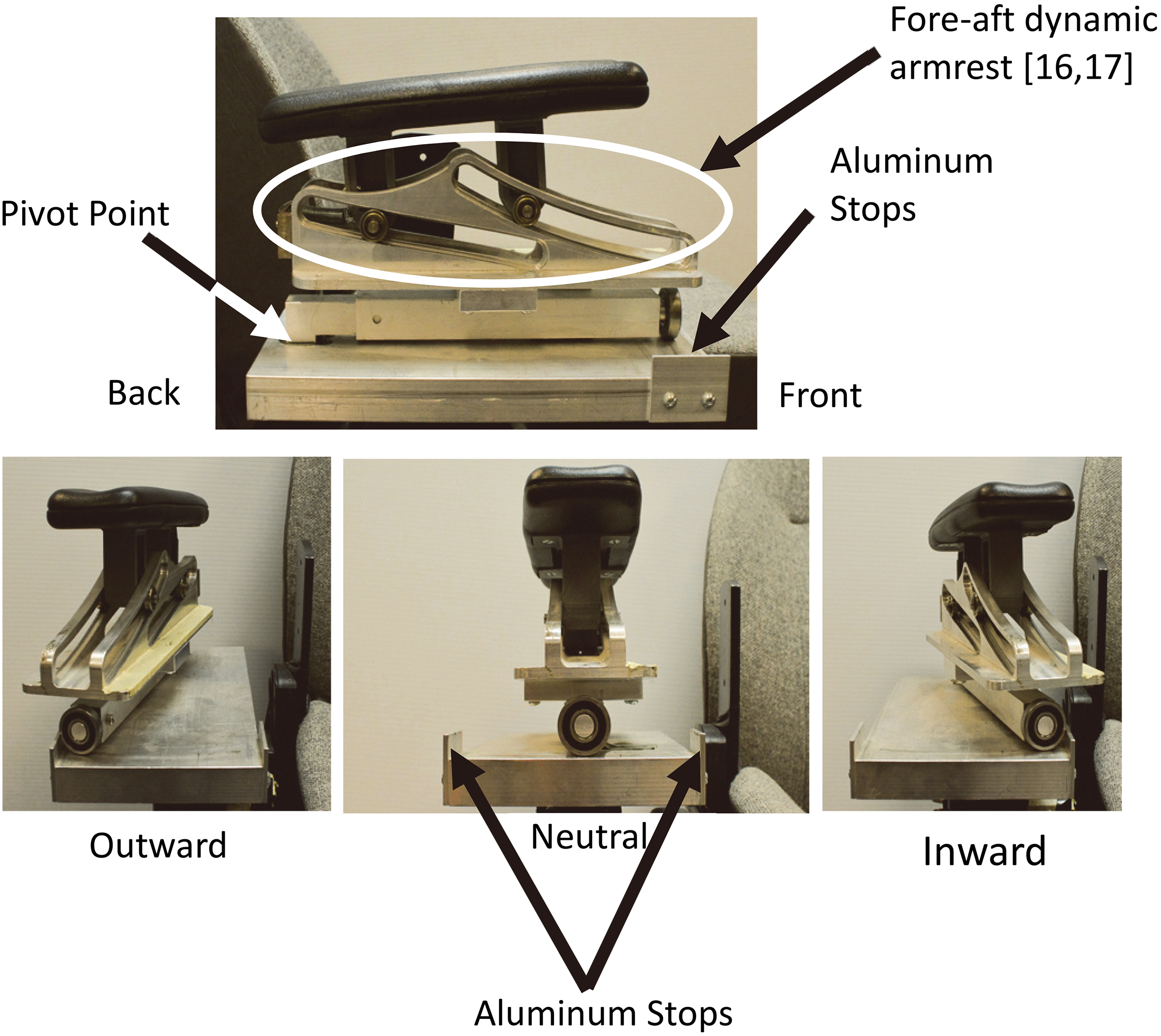
4.Discussion
The objective of this paper was to describe the process involved in designing a horizontally dynamic armrest according to the natural motion of the forearm during side-to-side joystick movements. The horizontally dynamic armrest design was intended to provide an addition to a fore-aft dynamic armrest designed by Murphy and Oliver [16]. This fore-aft dynamic armrest reduced loading in three shoulder and neck muscles when compared to a stationary armrest or no armrest during joystick use [16]. The device was designed to be used in a laboratory setting, so modifications would probably be required if it were to be used in the field.
Some studies have shown stationary armrests to be effective at reducing muscle loading during joystick use in the arm and shoulder [20], and some have found no significant difference between using a stationary armrest and no armrest (Lindbeck 1982, as reported by [7]). This could be because stationary armrests are not capable of supporting the arm through the full range of motion during joystick use. Fore-aft dynamic armrests have been investigated, and proven to reduce some muscle loading, but a horizontally dynamic armrest has not been designed or tested.
The wrist and elbow movements of operators during side-to-side joystick movement were recorded and analysed. It was determined that the elbow was relatively stationary (x, y, and z movement ranges all below 32 mm) and the wrist had a large horizontal (x-axis) range of motion (approximately 145 mm). The wrist also moved in the fore-aft and vertical directions (ranges less than 20 mm), however, these movements were so small relative to the side-to-side wrist movement that they were ignored in the final design.
The horizontally dynamic armrest design included an arm that rotated about a pivot point located below the operator’s elbow. This allowed the armrest to follow the rotational movement of the forearm about the elbow that was shown by operators during side-to-side joystick movement.
Future modifications to the armrest that may increase its success include adjusting the motion path of the armrest to incorporate the minimal vertical movement that occurs in the wrist during side-to-side joystick movements. If the design were to be used in the field it would also need to be fitted to the specific vehicles it is used in to ensure that it is compatible with the machine controls while allowing the required range of arm motion. If this design is successful, it could be modified accordingly and implemented in mobile machines that use joysticks in industries such as: forestry, construction, mining and metal smelting operations. The design could also be modified for other applications, and used for any manual task to reduce muscle strain.
5.Conclusion
The horizontally dynamic armrest design follows the natural pivoting motion of the forearm during side-to-side joystick manipulation. The wrist and elbow trajectories were determined for three participants (50
6.Future research
Future work will involve validating the armrest design to determine if it can reduce muscle activation levels during the performance of inward and outward joystick movements for a wide range of operator statures.
Conflict of interest
None to report.
References
[1] | Attebrant M, Winkel J, Mathiassen SE, Kjellberg A. Shoulder-arm muscle load and performance during control operation in forestry machines? Effects of changing to a new arm rest, lever and boom control system. Appl Ergon. (1997) ; 28: (2): 85-97. |
[2] | Nakata M, Hagner IM, Jonsson B. Trapezius muscle pressure pain threshold and strain in the neck and shoulder regions during repetitive light work. Scand J Rehabil Med. (1993) ; 25: (3): 131-7. |
[3] | Gellerstedt S. Mechanised Cleaning of Young Forest – the Strain on the Operator. Int J Ind Ergon. (1997) ; 20: (2): 137-43. |
[4] | Jonsson B. Kinesiology: with special reference to electromyographic kinesiology. Electroencephalogr Clin Neurophysiol Suppl. (1978) ; 34: (Supplement): 417-28. |
[5] | Oliver M, Tingley M, Rogers R, Rickards J, Biden E. Effect of joystick stiffness, movement speed and movement direction on joystick and upper limb kinematics when using hydraulic-actuation joystick controls in heavy vehicles. Ergonomics. (2007) ; 50: (6): 837-858. |
[6] | Golsse JM. Ergonomic Evaluation of Feller-buncher Cabs and Controls – Technical Note. Pointe Claire, Quebec, Canada For Eng Res Inst Canada – Wood Harvesting, (1989) . |
[7] | Hansson JE. Ergonomic design of large forestry machines. Int J Ind Ergon. (1990) ; 5: (3): 255-66. |
[8] | Axelsson S-Å, Pontén B. New ergonomic problems in mechanized logging operations. Int J Ind Ergon. (1990) ; 5: (3): 267-73. |
[9] | van der Windt D, Pope D, de Winter AF, Macfarlane G, Bouter L, et al. Occupational risk factors for shoulder pain: a systematic review. Occup Environ Med. (2000) ; 57: (7): 433-42. |
[10] | Oliver ML, Northey GW, Murphy TA, MacLean A, Sexsmith JR. Joystick stiffness, movement speed and direction effects on upper limb muscular loading. Occup Ergon. (2011) ; 10: (4): 175-187. |
[11] | Grevsten S, Sögren B. Symptoms and sickleave among forestry machine operators working with pronated hands. Appl Ergon. (1996) ; 27: (4): 277-80. |
[12] | Hagberg J, Lidén E. Mini-controls decrease musculo-skeletal symptoms [Minispakar minskar belastningsbesvär]. Forskningsstiftelsen Skogsarbetaren. (1991) ; (24): 4. |
[13] | Asikainen A, Harstela P. Influence of small control levers of grapple loader on muscle strain, productivity and control errors. J For Eng. (1993) ; 5: (1): 23-8. |
[14] | Westgaard RH, Aarås A. The effect of improved workplace design on the development of work-related musculo-skeletal illnesses. Appl Ergon. (1985) ; 16: (2): 91-7. |
[15] | Westgaard RH, Aarås A. Postural muscle strain as a causal factor in the development of musculo-skeletal illnesses. Appl Ergon. (1984) ; 15: (3): 162-74. |
[16] | Murphy T, Oliver ML. Development and design of a dynamic armrest for hydraulic-actuation joystick controlled mobile machines. Appl Ergon. (2008) ; 39: (3): 316-324. |
[17] | Murphy T, Oliver ML. Evaluation of a dynamic armrest for hydraulic-actuation controller use. Appl Ergon. (2011) ; 42: (5): 692-698. |
[18] | Murphy TA, Oliver ML. A comparison of task and muscle specific isometric submaximal electromyography data normalization techniques. Occup Ergon. (2011) ; 10: (1–2): 39-52. |
[19] | NASA. Anthropometry and Biomechanics. Available at: http//msis.jsc.nasa.gov/sections/section03.htm [Accessed May 15, 2017]. |
[20] | Westgaard RH. Measurement and evaluation of postural load in occupational work situations. Eur J Appl Physiol Occup Physiol. (1988) ; 57: (3): 291-304. |