Curcumin is a potential therapeutic agent that ameliorates diabetes among non-alcoholic fatty liver disease coexist with type 2 diabetes
Abstract
Type 2 diabetes mellitus (T2DM) and non-alcoholic fatty liver disease (NAFLD) harmonize and act synergistically in clinical practices. About 70–80% of diabetic patients develop NAFLD. At the same time, NAFLD existence increases T2DM development. Meanwhile, the presence of T2DM increases the progression to liver disease such as NAFLD, and to non-alcoholic steatohepatitis (NASH). The most prevalent chronic liver disease worldwide is a NAFLD. NAFLD and (T2DM) have a two-way pathophysiologic relationship, with the latter driving the development of the former into NASH. Nonetheless, NASH enhances the threat of cirrhosis as well as hepatocellular carcinoma (HCC), both cases in turn need transplantation of the liver. The only treatment for NAFLD is still lifestyle management because there are no FDA-approved drugs for the condition. In the current study, we review how curcumin (a naturally occurring phytopolyphenol pigment) treats NAFLD. Also we showed broad insights on curcumin-based therapy, by severe reduction of hepatic inflammation. Thus, our review showed that curcumin ingestion considerably decreased glycemic parameters (fasting blood glucose, glycosylated hemoglobin, insulin resistance index (HOMA-IR), and free fatty acids) and adipocyte-fatty acid binding protein (A-FABP), and adipokine released from adipocytes. Clinical trials are needed to evaluate the effects of curcumin and its specific dosage on liver enzymes, glycemic consequences, among NAFLD coexist with T2DM patients.
1Introduction
Over 400 million people are affected by diabetes globally [1], and type T2DM is the most common form of diabetes among diverse populations worldwide [2]. The Global Burden of Diseases [3] found that 22% upsurge in debility over previous 10 years was due to T2DM and its effects. Although glycemic control and diabetes treatment have made tremendous strides recently, the frequency of cardiovascular problems among T2DM patients continues to be a significant problem [4]. Despite the availability of numerous anti-diabetic medications managing hyperglycemia, therapeutic strategies addressing additional DM-related disorders as dyslipidemia and oxidative stress have also received significant attention. Oxidative stress may be the main reason for the emergence of T2DM. T2DM, upsurge oxidative species generation and decreased anti-oxidant capability have frequently been observed [5]. By increasing the flow of the polyol pathway, activating protein kinase C, changing the metabolism of eicosanoids, and inducing glucose autoxidation, hyperglycemia may contribute to oxidative stress and increase the production of reactive oxygen species (ROS). Diabetes can be brought on or made worse by ROS, which has many harmful effects, including reduced insulin secretion, decreased insulin synthesis, protein oxidation and fragmentation, DNA damage, production of free fatty acids, and augmented vascular permeability. Additionally, oxidative stress triggers the production of advanced glycation end products that promote the development of T2DM microvascular and macrovascular problems as well as endothelial dysfunction [6–8] (Fig. 1).
Fig. 1
Schematic illustration of the pathophysiological mechanism through which T2DM and NAFLD cause an increase in oxidative stress and inflammation. One of the most common cardiac complications in diabetic patients, diabetic cardiomyopathy (DCM), is associated with oxidative stress, which results from a serious imbalance between the production of reactive oxygen species and/or reactive nitrogen species.
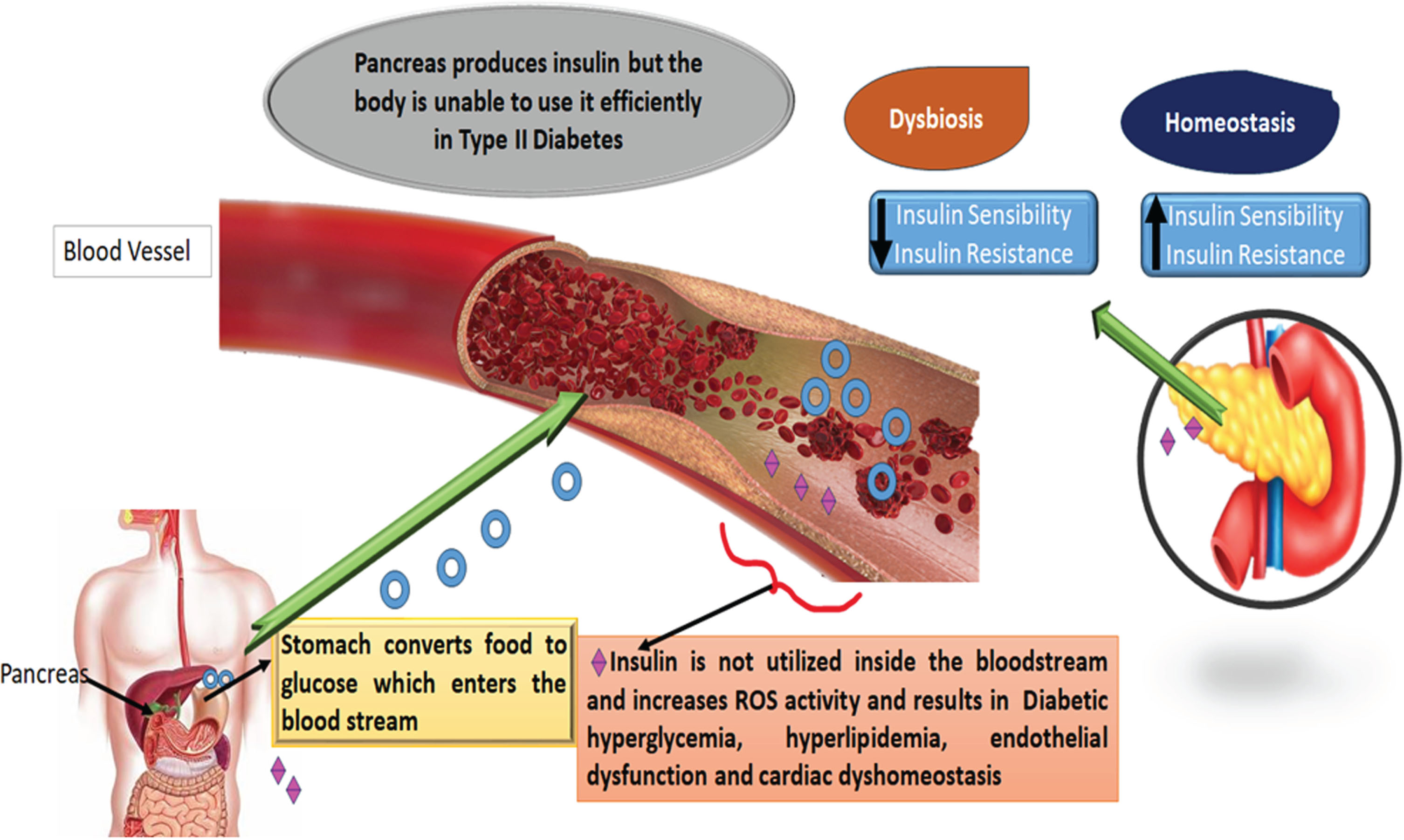
Anti-diabetic drugs, lifestyle modifications, frequent exercise, monitoring lipid profiles and arterial blood pressure are currently the most widely used treatments for T2DM. Effectual deterrence and medication are for diabetic patients. T2DM is a malady that can be avoided [9]. High risk T2DM subjects, pre-diabetes, and low glucose tolerance subjects must get a tight preventative strategy. Part of such prevention is changing the lifestyle, having balanced diet and consistent exercise.
Currently, pharmaceutical therapy, and lifestyle change are the most recent suggestions for diabetes control [10, 11]. The biological benefits of extracts or isolated polyphenolic monomers from diets, may improve metabolism, decrease oxidative stress and inflammation, and increase insulin resistance, have received a lot of attention recently [12, 13]. According to a growing number of randomized controlled studies [14–16], dietary polyphenols can improve a range of clinical indicators in NAFLD patients through gut, brain, liver, and their interconnected pathways.
Evidence supporting the effectiveness of using medical plant supplements for T2DM prevention and control is currently expanding [17–19]. The scientific world is becoming increasingly interested in curcumin (a medicinal herb). The rhizome of the turmeric plant, Curcuma longa, contains the active chemical curcumin. Turmeric contains a naturally occurring polyphenol molecule, which both in vitro and in vivo displays two crucial bioactivities [20]. First curcumin works as an antioxidant by scavenging ROS [21]. Curcumin also possesses hepato-protective ingredients which treat liver damage. According to numerous studies, curcumin may delay the onset of NASH by lowering inflammation and restoring the balance of the liver’s antioxidant systems. The second is curcumin, has an anti-inflammatory, antibacterial, and anti-carcinogenic compounds. As oxidative stress and inflammation are two major causes of insulin resistance and NAFLD, curcumin enhances natural peroxisome proliferator-activated receptor (PPAR) expression, which is essential for lowering them [22, 23]. The purpose of the present article is to provide evidence that curcumin reduces the severity of NAFLD by diminishing lipid accumulation, oxidative stress and inflammation.
Furthermore, our review explores the relationship between NAFLD and diabetes development in people with specific genetic variants and showed the pathophysiological relationships between T2DM and NAFLD.
2Pervasiveness of NAFLD and its threat factors
NASH is a set of progressive liver disorders that vary from basic hepatic steatosis that is characterized by hepatocyte inflammation follow stop, Liver fibrosis conceivably leads to hepatocellular carcinoma (HCC) or end-stage liver disease progress in roughly represent 35% of NASH cases [24, 25]. NAFLD epidemic is widespread through western countries disarrays approximately 20–30% of the general populace and 45–75% of persons identified with T2DM [26, 27].
NAFLD is characterized by hepatic steatosis that is determined by histology/imaging with macrovesicular steatosis in more than 5% of hepatocytes in accordance with histological analysis. Hepatic steatosis also determined by proton density fat fraction, by proton magnetic resonance spectroscopy (MRS) or quantitative fat/water selective magnetic resonance imaging (MRI) with no secondary reason for steatosis [28].
Numerous medications may result in steatosis or steatohepatitis, which share clinical characteristics with NAFLD or alcoholic fatty liver disease. Primary steatotic trait can be utilized to categorize the steatosis by medicines (Table 1).
Table 1
Drugs that cause obesity/insulin resistance, phospholipidosis, steatohepatitis, macrovesicular steatosis, and microvesicular steatosis and further drug-induced fatty liver disease and their theorized toxicity mechanisms
Microvesicular steatosis | Macrovesicular steatosis | |
1 | Drugs like tetracycline, zidovudine, valproic acid, aspirin, vitamin A, ibuprofen, have been linked to microvesicular steatosis [34]. | Acetaminophen, NSAIDs such as ibuprofen, indomethacin, and sulindac, metoprolol, chlorinated hydrocarbons as chloroform, carbon tetrachloride and chemotherapeutic medications such as tamoxifen, 5-fluorouracil and cisplatin, can all result in macrovesicular steatosis [38–40]. |
2 | Microvesicular steatosis is often defined histologically by a number of tiny lipid vesicles that exit the nucleus of the hepatocyte at the center of the cell in an acute liver damage. Clinically, it is linked to lactic acidosis, acute liver failure, and a rise in blood aminotransferase levels [35]. | Both alcoholic and non-alcoholic liver illness frequently exhibit macrovesicular steatosis (macrosteatosis), also referred to as fatty liver [41]. |
3 | Cytolytic hepatitis is associated with drug-induced mitochondrial DNA (mtDNA) depletion and impairment of oxidative phosphorylation.Drug-induced cytolytic hepatitis, mitochondrial dysfunction, and microsteatosis [36]. | Diabetes and obesity are two cardiometabolic risk factors that are associated with non-alcoholic fatty liver [42]. |
4 | Drugs may obstruct one or more mitochondrial fatty acid oxidation (FAO) enzymes directly. Drugs can also negatively affect the production of coenzyme A and L-carnitine esters, two of mitochondrial FAO’s main cofactors, as is the case with valproic acid (VPA). Drugs can also inhibit the mitochondrial respiratory chain (MRC), which affects how well FAD and NAD+are renewed. Deep mtDNA depletion causes MRC dysfunction, which then causes FAO inhibition and results in microsteatosis [37]. | The microsomal triglyceride transfer protein (MTP), causes macrovesicular steatosis, may be inhibited by medications like amiodarone and perhexiline. Efavirenz that enriches the actions of AMP-activated protein kinase (AMPK), which leads to mitochondrial dysfunction. The activation of lipogenic transcription factors, like PPAR, PXR, and glucocorticoid receptor...Direct drug intervention can increase liver lipid synthesis [43–45]. |
The presence of tiny to large lipid droplets in the hepatocyte cytoplasm together with peripheral nucleus displacement is referred to as macrovesicular steatosis [29–31]. Although this type of liver damage is frequently curable, it may eventually progress to cirrhosis and possibly steatohepatitis. Excessive alcohol consumption and the use of glucocorticoids, total parenteral nutrition, methotrexate, and amiodarone as treatments are also linked to macrovesicular steatosis. The use of 5-fluorouracil, tamoxifen, irinotecan (IRI), cisplatin, and asparaginase during chemotherapy may also result in macrovesicular steatosis [32].
Hepatocytes undergo microvesicular steatosis when their nuclei are surrounded by a large number of tiny lipid vesicles. Microvesicular steatosis is associated with substantial impairment of fatty acid beta-oxidation in the mitochondria. Since mitochondria are unable to efficiently oxidize non-esterified fatty acids, they undergo enhanced esterification into triglycerides, which is the major lipid type that builds up under these circumstances. Since acute microvesicular steatosis develops quickly to either death or resolution, significant necrosis, cholestasis, and fibrosis are typically absent in this condition [33]. Drugs such as valproic acid, tetracycline, aspirin, ibuprofen, zidovudine, and vitamin A have been related to microvesicular steatosis.
The most common cause of chronic liver disease is thought to be NAFLD, which is also a growing public health problem with pandemic proportions [46]. Histology and proton magnetic resonance spectroscopy (MRS) [47] both detected 31% and 12.2% of NAFL patients, respectively, of which 5% had NASH. Although 20% of asymptomatic T2DM patients have biopsy-proven NASH and normal liver function tests, NAFLD is widespread in T2DM (59.67% ) [48]. Serum liver enzymes are less illuminating due fat deposition and the normal reference values for blood liver enzymes need to be altered. Armstrong et al. [49] found that 5%–7% of T2DM individuals without symptoms had advanced fibrosis (Table 2).
Table 2
Prevalence of NAFLD and NASH Globally
NAFLD Prevalence | NASH Prevalence | Population studied | Population size | Method of diagnosis | Remark | Reference |
Mild, moderate, and severe NAFL: 49.3% NAFL was found in 38.9%, 9.0%, and 1.4% of the donor candidates (mild steatosis was defined as fatty alterations in 5% to 30% of hepatocytes, moderate steatosis in 30% to 60% of hepatocytes, and severe steatosis in > 60% of hepatocytes), respectively.Liver histology shows steatosis in > 60% of hepatocytes but no discernible inflammation | The population of Asians-2.2% | Applicants from Korea for LIVE liver donation | 589 | 589 liver biopsies with US guidance | [55] | |
NAFLD was characterized as having > or=5% of hepatocytes harboring macrovesicular fat in the pediatric US population (9.6% ) | Not Reported | Children in the United States (2–19 years old) | From 1993 to 2003, 742 kids (2–19 years old) underwent autopsies | During an autopsy, histopathology | prevalence varies (Asians: 10.2%; Blacks: 1.5%; Hispanics: 11.8%; Whites: 8.6% ). Children who were obese had the highest risk of NAFLD (38% ) | [56] |
Adult Urban US NAFLD 31% population | Not Reported | A sizable, ethnic diverse, probability-based adult demographic an example of Texas’s Dallas, the United States-contributors to the Houston Heart Study | 2349 | The liver’s H-MRS to calculate HTGC | Serum alanine aminotransferase levels were within normal range in 79% of patients with hepatic steatosis. varying rates of hepatic steatosis in various subpopulations: whites 33%, blacks 24%, and Hispanics 45% | [57] |
46 (40% ) of the US cohort overall had NAFLD | 12.2% of the US cohort as a wholeNAFLD patients’ prevalence was 29.9% | US citizens between the ages of 18 and 70 cohort | 328 | Liver biopsy (in 134 patients who had undergone ultrasonography screening) | Hispanics and people with diabetes are at the highest risk for both NAFLD and NASH. | [58] |
NAFLD’s public health significance arises from its numerous effects on mortality, morbidity, and the use of medical services internationally [50, 51]. In general population, NAFLD and NASH fibrosis are linked to to liver-related mortality. Hepatocellular carcinoma, chronic liver disease, and cirrhosis of the liver are all frequently brought on by NASH and frequently develop in non-cirrhotic livers as well [52–54]. In the United States, the United Kingdom, and even in developing nations, NASH is the fastest-growing reason for liver transplantation.
3Pathophysiological connections between T2DM and NAFLD
NAFLD and T2DM are significantly predisposed as a result of obesity along with insulin resistance [59–61]. Clarifying whether the presence of liver disease in NAFLD upsurges the threat of developing T2DM is crucial from a clinical standpoint because it’s possible that treating NAFLD (particularly remedy intended to treat insulin resistance and obesity) could also reduce the threat of augmenting T2DM in addition to treating the metabolic risk factors linked to NAFLD. Contrarily, lifestyle modifications (such as a low-calorie diet and modest exercise) are acknowledged to significantly improve liver damage, fibrosis, and hepatic steatosis in people with NAFLD [62]. They are particularly successful at postponing T2DM in those who are at high threat for the illness [63, 64].
Genetics has been extensively studied and is the chief threat factor for the succession of NAFLD [65]. Romeo et al. [66] in a genome-wide association study (GWAS) highlight the critical part of (PNPLA3 I148M) patatin-like phospholipase domain containing 3 in NAFLD patients. They showed how PNPLA3 I148M contributed to the increased hepatic fat content and severe hepatic inflammation [67]. By using GWAS, Kozlitina et al. [68] identified the transmembrane 6 superfamily member 2 (TM6SF2 E167K) as a significant polymorphism site for the risk ofNAFLD.
The liver converts cholesterol into the 2 primary bile acids (Cholic acid and Chenodeoxycholic acid) that delivered into the intestine as conjugates of glycine and taurine. Subsequently, the intestinal microbiome transmutes primary bile acids into secondary bile acids like deoxycholic acid, litho-cholic acid, and urso-deoxycholic acid. These secondary bile acids then interrelate with a number of nuclear receptors in the intestine, involving Takeda G protein-coupled membrane receptor 5 (TGR5) and farnesoid X receptor (FXR). Bile acids interconnect with TGR5 and FXR receptors and regulate hepatic lipid and glucose metabolism.
TGR5 activation in enterocytes causes an increase in the amount of glucagon-like peptide 1 (GLP1) released from L cells. GLP1 release has an effect on plasma glucose level, which then invigorates the pancreatic islet to generate more insulin. The body expels insulin through the liver. Hepatic insulin extraction, which makes up 50–80% of insulin clearance, is decreased in NAFLD. By turning on hepatic FXR receptors, fatty acid and triglyceride production is reduced, potentially as a result of less hepatic lipid buildup [69, 70]. The formation of hepatic glycogen rises in addition to the production of hepatic gluconeogenesis falling as a result of FXR activation, that is shown in Fig. 2 are potential risk factors for T2DM.
Fig. 2
Illustrates the association between the liver, bile, and gut that may lead to receptor alteration and change insulin metabolism and bile acid production. In non-alcoholic fatty liver disease (NAFLD), hepatic insulin extraction decreases, which accounts for 50–80% of insulin clearance. Fatty acid and triglyceride production is decreased by activating hepatic FXR receptors, possibly due to less hepatic lipid accumulation.
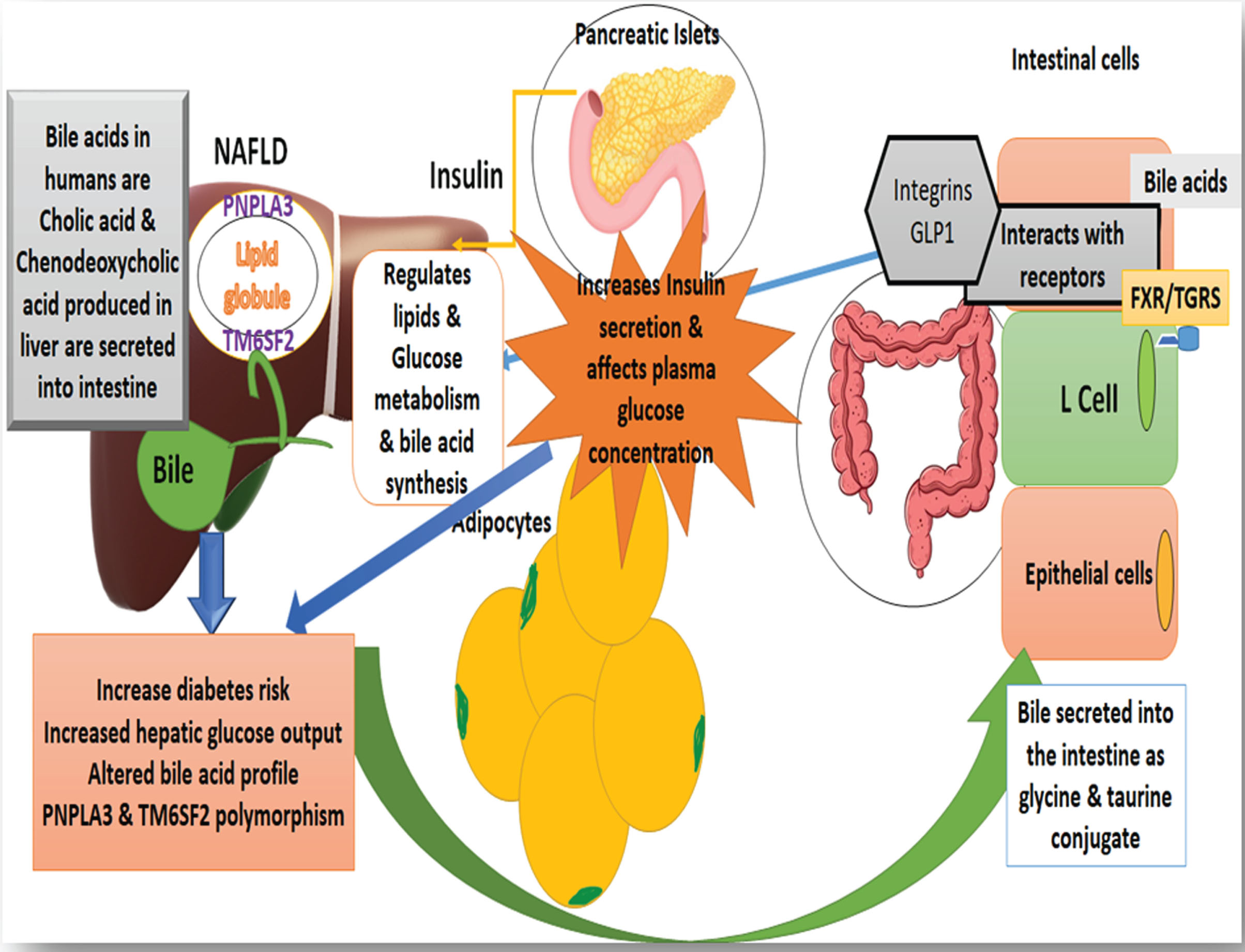
The precise NAFLD risk factors that increase T2DM risk are not known. However, it is well recognized that lipid accumulation is associated with hepatic insulin resistance and hepatic inflammation, both of which are significant NAFLD features. Therefore, by reducing hepatic fat accumulation in NAFLD, therapies can improve insulin sensitivity and chronic inflammation may help reduce the incidence of T2DM.
4Curcumin acts as a potential therapeutic agent
Turmeric is common name for the herbaceous plant Curcuma longa, which is consumed as a spice in many dishes. This plant species, was identified by its orange tuberculate rhizomes, and is extensively grown in South East Asia, where it has long been utilized as a natural medication for a broad range of pathological maladies. Curcumin has gained interest as a nutraceutical from scientists due to its anti-inflammatory and antioxidant activities as well as the safety of its pharmacological profile [71]. Curcumin aid in the deterrence and therapy of a wide range of maladies because of its broad spectrum of beneficial effects, including its antibacterial, anti-diabetic, antiviral, and anti-cancer qualities [72, 73].
Curcuma longa, is a member of the Zingiberaceae family that is grown in tropical and subtropical climates where it has been used as a curative agent in Indian and Chinese traditional medicine [74]. The main curcuminoids in commercial curcumin are 77% curcumin (curcumin I), 17% demethoxycurcumin (curcumin II), 3% bis-demethoxycurcumin (curcumin III), and 3% cyclocurcumin (curcumin IV) [75, 76]. Turmeric also contains 69.4% carbohydrates, 6.3% protein, 5.1% fat, 5.8% essential oils, and 3–6% curcuminoids.
The biological and pleiotropic effects of curcumin are numerous (Fig. 3) [77–79] including: antibacterial [80, 81], antineoplastic [82, 83], ant proliferative [84], and anti-inflammatory agent [85–88]. Curcumin also offers therapeutic promise for treating diabetes mellitus [89–91], hepatic damage [92, 93], renal illnesses [94, 95], many disorders [96, 97], cardiovascular diseases [98, 99], and hepatic damage [100].
Fig. 3
Depicts numerous pharmacological and pleiotropic benefits of curcumin including anti-inflammatory, anti-bacterial, antineoplastic, and antioxidant properties.
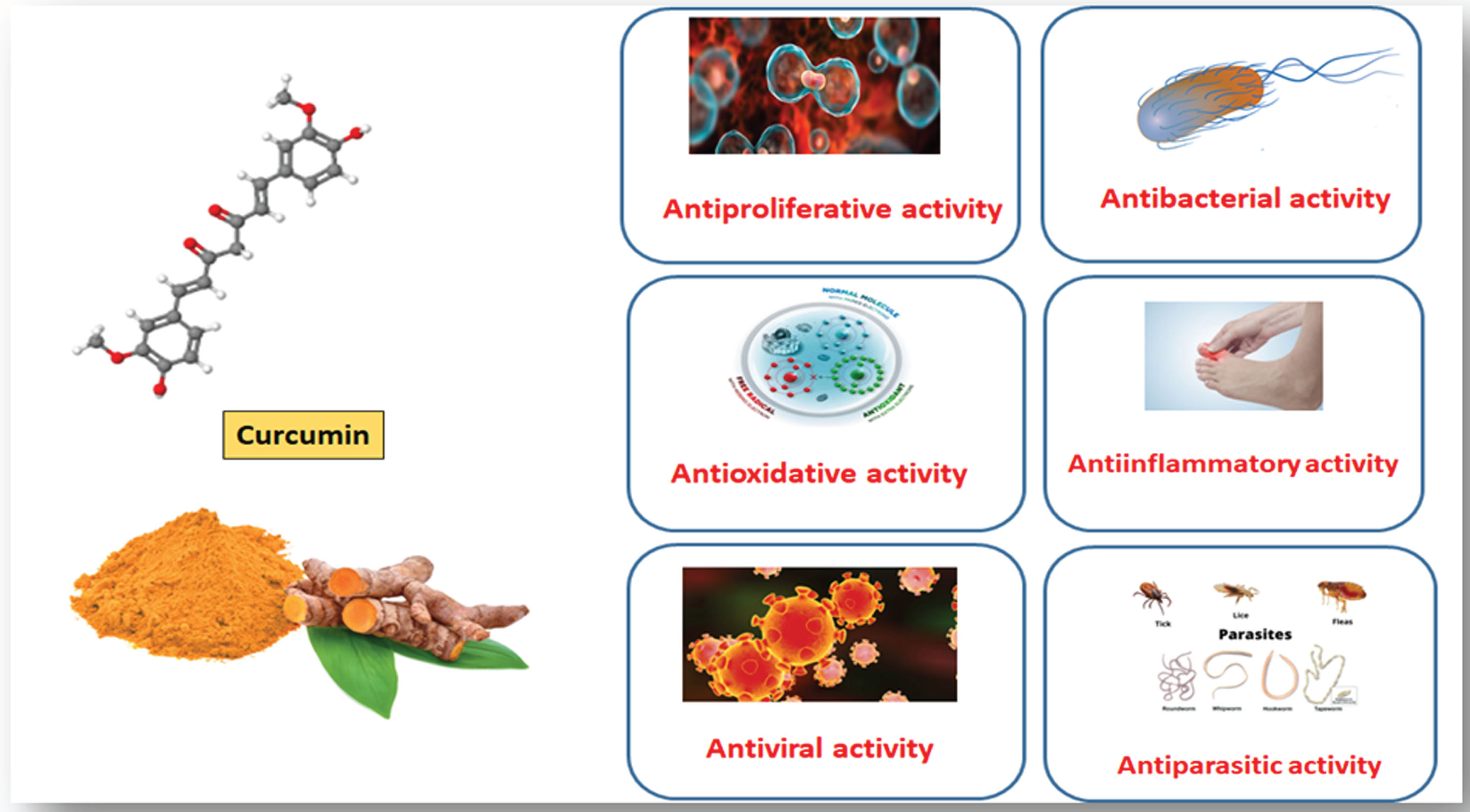
5Curcumin ameliorates insulin’s function in glucose homeostasis
The primary roles of the protein hormone insulin are balancing between nutrients and glucose [101]. In accordance with high blood sugar levels, the islets of Langerhans liberates insulin, which is then traveled into its target tissues, like liver, adipose tissue, and skeletal muscle [102]. Blood sugar levels return to normal as a result of insulin’s stimulation of adipose tissue and skeletal muscle resulting in the absorption of glucose as well as suppression of the liver’s endogenous production of glucose [103].
The process of triggering insulin’s mode of action is by the attachment of insulin to its receptor, which is present on the target cell membrane [104]. The receptor tyrosine kinase gets activated and instigates the insulin receptor substrate (IRS) to be phosphorylated [105]. Further downstream processing, the lipid kinase-like phosphatidylinositol-3 kinase (PI3-K) along with the serine/threonine kinase as well as Akt/PKB are stimulated [106]. In adipose as well as muscle cells, the GLUT4 is glucose transporter which is translocated from cells internal side to the exterior cell membrane, resulting in enhanced glucose uptake. By inhibiting glycogenolysis and gluconeogenesis, insulin signaling and action reduce endogenous glucose production (Fig. 4) in liver cells [107].
Fig. 4
The pharmacological actions of curcumin on insulin receptor activation and glucose absorption. Protein kinase-B, Phosphatidylinositol-3,4,5-triphosphate and 4,5-bisphosphate (PIP3 & PIP2), respectively; Insulin receptor substrate 1 (IRS1), tumor necrosis factor (TNF), AMP-activated protein kinase (AMPK), Nuclear factor kappa-light-chain- activates B-cells (NF-kB), Peroxisome proliferator-activated receptor gamma co-activator 1 (PGC-1), Acetyl-CoA carboxylase (ACC) and for free fatty acids (FFAs).
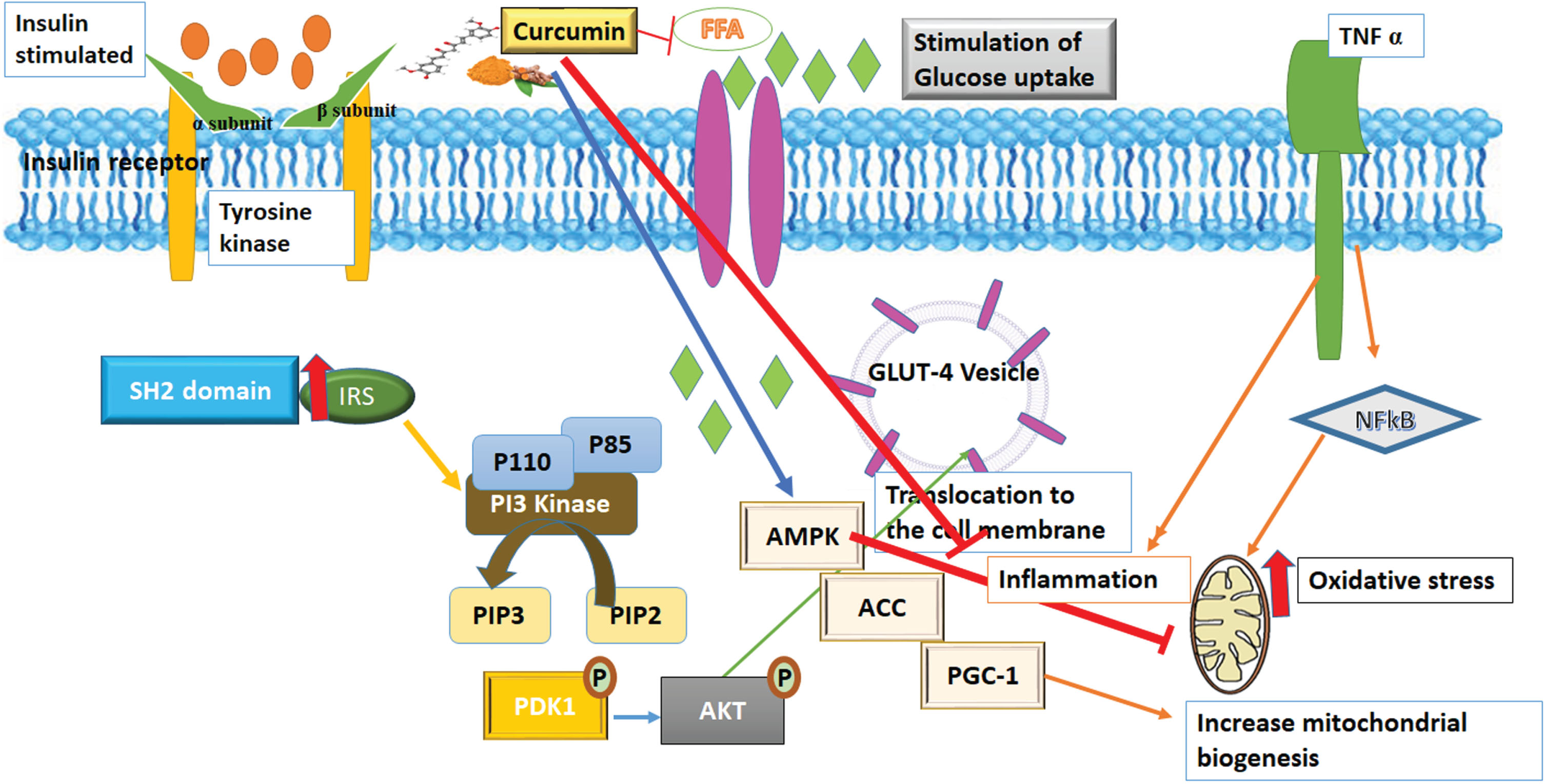
Defects in the insulin signaling system are responsible for both insulin resistance, which is outlined by lower responsiveness of circulating levels of insulin in target tissues [108], and (T2DM) [109]. T2DM along with insulin resistance have been linked to ageing, obesity, inflammation, and a deskbound lifestyle [110]. Hyperglycemia brought on by insulin resistance over time damages macro- and microvascular tissue and results in conditions such, retinopathy, cardiovascular disease, nephropathy and neuropathy [111]. Increased plasma-free fatty acids (FFAs) diminish insulin’s capacity to lower hepatic glucose synthesis along with promotion of glucose absorption by fat and muscle cells, which is associated with insulin resistance and obesity [112, 113].
Asia has a long history of using Curcuma long medicinally, especially in South East Asia and China [114]. The chief naturally occurring polyphenol found in Curcuma longa and other species of curcuma were curcumin. It is identified as diferuloylmethane [115]. 1, 7-bis(4-hydroxy-3-methoxyphenyl)-1,6 heptadiene-3,5-dione makes up this substance. Two methoxylated phenols, an enol form of diketone, and two methoxylated phenols make up the structure of curcumin, with the latter serving as the active site for the former’s antioxidant activities [116]. The amount of methoxy groups on their aromatic rings is the only structural distinction between curcumin and other curcuminoids, like de-methoxy-curcumin and bis-demethoxy-curcumin. The potential anti-atherosclerotic, anti-cancer, anti-inflammatory, hepatoprotective, immunomodulatory, nephroprotective, and anti-diabetic properties of turmeric and curcumin have been proven by scientists.
According to literature reports, curcumin (20μM) was subjected to 3T3-L1 cell line and primary adipocytes for 6 days. It resulted in the browning of white adipocytes and mitochondrial growth [117]. After curcumin administration, PGC-1, PPAR, (PRDM16)-PR domain containing 16, Ucp1 protein and levels of mRNA upsurge. Mitochondrial proteins CPT1 and cytochrome-c were also augmented by curcumin therapy [118]. Curcumin therapy increased both the total and phosphorylated levels of AMPK. PGC-1, PRDM16, and Ucp1 protein levels reduced when curcumin and the AMPK inhibitor dorsomorphin were supplied concurrently, demonstrating that curcumin’s actions are brought on by AMPK activation [119]. This study unequivocally shows that curcumin therapy has controlled obesity, insulin resistance, and T2DM.
6Clinical trials provided evidences that curcumin is an effective anti hyperglycemic drug
The likelihood of developing T2DM, IR, NAFLD, obesity, and other metabolic disorders is amplified by genetics, and gender [120]. Lifestyle, nutritional, and circadian rhythm adjustments have a long-term positive impact on the clinical and Para clinical outcomes of NAFLD and T2DM [67]. Curcumin was given to overweight/obese T2DM [121] at a dose of 300 mg/day, and Na et al. demonstrated that curcumin decreased body mass index (BMI), fasting blood glucose, glycosylated hemoglobin, insulin resistance index (HOMA-IR), and free fatty acids. Glucose drop was only 18% and the glycosylated hemoglobin drop was only 11% from baseline. Later, the same group found that curcumin reduces adipocyte-fatty acid binding protein (A-FABP), an adipokine released from adipocytes [121, 122] that coordinate lipid-mediated processes [123] that decrease free fatty acid levels.
The possibility of reaching its target has been one of the key issues with curcumin/curcuminoids’ potential as medicinal agents. Because of their poor stability and pharmacokinetic characteristics, curcuminoids’ therapeutic benefits have recently come under scrutiny [124]. Curcumin has limited bioavailability since it is poorly absorbed and readily destroyed [125]. Docking studies are needed to understand its pharmacokinetic and pharmacodynamics activity of curcumin.
7Conclusion
Globally, T2DM affects thousands of people and has a complex pathophysiology. Changes in lifestyle, food quality, and increasing physical activity and curcumin supplements can improve diabetes control. Although research is needed to understand the optimal amount of curcumin that can be used. Curcumin is a natural anti-inflammatory and anti-diabetic drug that offers a secure and affordable alternative for treating diabetes. To establish the role of curcumin in the treatment of T2DM, we advise conducting randomized controlled clinical trials. In conclusion, it is challenging to discern between the cause and effect of NAFLD and T2DM, since the interaction between them is so complicated. While NAFLD may increase the risk of T2DM, T2DM offers the best metabolic environment for NAFLD advancement. Numerous elements are similar in both clinical conditions—such as an accumulation of fatty acids, pro-inflammatory cytokines, oxidative stress, that are implicated in the disease’s progression. Treatment options for NAFLD and T2DM may become stronger with a complete understanding of their underlying mechanisms. The restricted solubility and low bioavailability of curcumin require numerous strategies to overcome. These include creating innovative curcumin formulations, such as sustained-release tablets, liposomal encapsulation, nanoparticles, emulsions, and synthesizing curcuminoids. The development of a “super curcumin” in the near future is awaited for result from curcumin’s improved bioavailability and clinical trial findings, boosting this promising natural product to the vanguard as a therapeutic remedy for diabetes. This article provides insights for researchers to further carry out docking studies to have clear-cut comprehension of curcumin’s potential to cure diabetes.
Acknowledgments
Manar Atoum is thankful for The Hashemite University for Support, KRP is thankful to the Department of Biotechnology, Sri Padmavati Mahila Visva Vidyalayam (Women’s) University, Tiruapti-India and KRD is thankful for Sree Balaji Dental College and Hospital, Bharath Institute of Higher Education and Research (BIHER) Bharath University, Chennai, Tamil Nadu.
Fund
No fund because it is a review article.
Author contributions
Manar Atoum draft manuscript preparation, revision and editing of the article.KR Padma contributed in draft manuscript preparation, writing, drawing figures.
KR Don contribute drafting this review article and drawing figures.
Conflict of interest
No conflict of interest between the authors.
References
[1] | International Diabetes Federation (IDF). IDF Diabetes Altas, 8th ed.; International Diabetes Federation: Brussels, Belgium, 2017; ISBN 9782930229874. |
[2] | World Health Organization (WHO), Global Report on Diabetes; World Health Organization: Geneva, Switzerland, 2016. |
[3] | Vos T , Allen C , Arora M , Barber RM , Brown A , Carter A , Casey DC , Charlson, FJ, Chen AZ, Coggeshall M, et al. Global, regional, and national incidence, prevalence, and years lived with disability for 310 diseases and injuries, 1990–2015: A systematic analysis for the Global Burden of Disease Study 2015. Lancet. (2016) ;388: :1545–1602. |
[4] | Pirola L , Balcerczyk A , Okabe J , El-Osta A . Epigenetic phenomena linked to diabetic complications. Nat Rev Endocrinol. (2010) ;6: :665. |
[5] | Sousa RAL , Improta-Caria AC , Souza BSF . Exercise-linked irisin: consequences on mental and cardiovascular health in type 2 diabetes. Int J Mol Sci. (2021) ;22: (4):2199. doi: 10.3390/ijms22042199 |
[6] | Adams JA , Uryash A , Lopez JR , Sackner MA . The Endothelium as a therapeutic target in Diabetes: A Narrative Review and Perspective. Front Physiol. (2021) ;12: :638491. doi: 10.3389/fphys.2021.638491. |
[7] | Shen CY , Lu CH , Wu CH , Li KJ , Kuo YM , Hsieh SC , et al. The Development of Maillard Reaction, and Advanced Glycation End Product (Age)-Receptor for AGE (Rage) Signalling Inhibitors as Novel Therapeutic Strategies for Patients with age-related diseases. Molecules (Basel Switzerland). (2020) ;25: (23):5591. doi: 10.3390/molecules25235591. |
[8] | Salvatore T , Pafundi PC , Galiero R , Rinaldi L , Caturano A , Vetrano E , et al. Can metformin exert as an Aactive Ddrug on endothelial dysfunction in diabetic subjects? Biomedicines. (2021) ;9: (1):3. doi: 10.3390/biomedicines9010003. |
[9] | Johnson EL , Feldman H , Butts A , Billy CDR , Dugan J , Leal S , Rhinehart AS , Shubrook JH , Trujillo J , Neumiller JJ , et al. Standards of medical care in diabetes—2019 abridged for primary care providers. Clin Diabetes. (2019) ;37: :11–34. |
[10] | Tacke F , Weiskirchen R . Non-alcoholic fatty liver disease (NAFLD)/nonalcoholic steatohepatitis (NASH)-related liver fibrosis: Mechanisms, treatment and prevention. Ann Transl Med. (2021) ;9: (8):729. doi: 10.21037/atm-20-4354. |
[11] | Głuszyńska P , Lemancewicz D, Dzięcioł JB, Razak Hady H. Non-alcoholic fatty liver disease (nafld) and bariatric/metabolic surgery as its treatment option: A review. J Clin Med. (2021) ;10: (24):5721. doi: 10.3390/jcm10245721. |
[12] | Abenavoli L , Larussa T , Corea A , Procopio AC , Boccuto L , Dallio M , et al. Dietary polyphenols and non-alcoholic fatty liver disease. Nutrients. (2021) ;13: (2):494. doi: 10.3390/nu13020494. |
[13] | Simental-Mendía LE , Gamboa-Goómez CI , Guerrero-Romero F , Simental-Mendía M , Saónchez-García A , Rodríguez-Ramírez M . Beneficial effects of plant derived natural products on non-alcoholic fatty liver disease. Adv Exp Med Biol. (2021) ;1308: :257–72. doi: 10.1007/978-3-030-64872-5_18. |
[14] | Salomone F , Godos J , Zelber-Sagi S . Natural antioxidants for non-alcoholic fatty liver disease: molecular targets and clinical perspectives. Liver Int. (2016) ;36: (1):5–20. doi: 10.1111/liv.12975. |
[15] | Bagherniya M , Nobili V , Blesso CN , Sahebkar A . Medicinal plants and bioactive natural compounds in the treatment of non-alcoholic fatty liver disease: A clinical review. Pharmacol Res. (2018) ;130: :213–40. doi: 10.1016/j.phrs.2017.12.020. |
[16] | Ji Y , Yin Y , Sun L , Zhang W . The molecular and mechanistic insights based on gut-liver axis: Nutritional target for non-alcoholic fatty liver disease (nafld) improvement. Int J Mol Sci. (2020) ;21: (9):3066. doi: 10.3390/ijms21093066. |
[17] | Demmers A , Korthout H , van Etten-Jamaludin FS , Kortekaas F , Maaskant JM . Effects of medicinal food plants on impaired glucose tolerance: A systematic review of randomized controlled trials. Diabetes Res Clin Pract. (2017) ;131: :91–106. |
[18] | Poolsup N , Suksomboon N , Kurnianta PDM , Deawjaroen K . Effects of curcumin on glycaemic control and lipid profile in prediabetes and type 2 diabetes mellitus: A systematic review and meta-analysis. PLoS ONE. (2019) ;14: :e0215840. |
[19] | Suksomboon N , Poolsup N , Boonkae S , Suthisisang CC . Meta-analysis of the effect of herbal supplement on glycaemic control in type 2 diabetes. J Ethnopharmacol. (2011) ;137: :1328–1333. |
[20] | Li HL , Liu C , de Couto G , Ouzounian M , Sun M , Wang AB , Huang Y , He CW , Shi Y , Chen X , et al. Curcumin prevents and reverses murine cardiac hypertrophy. J Clin Investig. (2008) ;118: :879–893. |
[21] | Yarru LP , Settivari RS , Gowda NK , Antoniou E , Ledoux DR , Rottinghaus GE . Effects of turmeric (Curcuma longa) on the expression of hepatic genes associated with biotransformation, antioxidant, and immune systems in broiler chicks fed aflatoxin. Poult. Sci. (2009) ;88: :2620–2627. |
[22] | Jazayeri-Tehrani SA , Rezayat SM , Mansouri S , Qorbani M , Alavian SM , Daneshi-Maskooni M , Hosseinzadeh-Attar MJ . Efficacy of nanocurcumin supplementation on insulin resistance, lipids, inflammatory factors and nesfatin among obese patients with non-alcoholic fatty liver disease (NAFLD): A trial protocol. BMJ Open. (2017) ;1;7: (7):e016914. |
[23] | Jazayeri-Tehrani SA , Rezayat SM , Mansouri S , Qorbani M , Alavian SM , Daneshi-Maskooni M , Hosseinzadeh-Attar MJ . The nanocurcumin reduces appetite in obese patients with non-alcoholic fatty liver disease (nafld): A double-blind randomized placebo-controlled clinical trial. Nanomed J. (2018) ;5: (2):67–76. |
[24] | Singh S , Allen AM , Wang Z , Prokop LJ , Murad MH , Loomba R . Fibrosis Progression in Nonalcoholic Fatty Liver vs Nonalcoholic Steatohepatitis: A Systematic Review and Meta-analysis of Paired-Biopsy Studies. Clin Gastroenterol Hepatol. (2015) ;13: (4):643–54.e9. |
[25] | Chalasani N , Younossi Z , Lavine JE , Charlton M , Cusi K , Rinella M , et al. The diagnosis and management of nonalcoholic fatty liver disease: practice guidance from the American Association for the Study of Liver Diseases. Hepatology. (2018) ;67: (1):328–57. |
[26] | Lonardo A , Bellentani S , Argo CK , Ballestri S , Byrne CD , Caldwell SH , et al. Epidemiological modifiers of non-alcoholic fatty liver disease: focus on high risk groups. Dig Liver Dis. (2015) ;47: (12):997–1006. |
[27] | Younossi ZM , Golabi P , de Avila L , Paik JM , Srishord M , Fukui N , et al. The global epidemiology of NAFLD and NASH in patients with type 2 diabetes: A systematic review and meta-analysis. J Hepatol. (2019) ;71: (4):793–801. |
[28] | European Association for the Study of the Liver (EASL), European Association for the Study of Diabetes (EASD), European Association for the Study of Obesity (EASO). EASL-EASD-EASO clinical practice guidelines for the management of non-alcoholic fatty liver disease. J Hepatol. (2016) ;64: :1388–402. |
[29] | Kleiner DE . The pathology of drug-induced liver injury, Seminars in Liver Disease. (2009) ;29: (4):364–372. |
[30] | Zhang X , Ouyang J , Thung S N . Histopathologic manifestations of drug-induced hepatotoxicity. Clin Liver Dis. (2013) ;17: (4):547–564. |
[31] | Ramachandran R and Kakar, S. Histological patterns in drug induced liver disease. J Clin Pathol. (2009) ;62: (6):481–492. |
[32] | Sahoo S and Hart, J. Histopathological features of Lasparaginase-induced liver disease. Seminars in Liver Disease. (2003) ;23: (3):295–299. |
[33] | Stravitz RT , Sanyal AJ . Drug-induced steatohepatitis. Clin Liver Dis. (2003) ;7: :435–51. |
[34] | Ramachandran R and Kakar S. Histological patterns in drug-induced liver disease. J Clin Pathol. (2009) ;62: :481–492. |
[35] | Bissuel F , Bruneel F , Habersetzer F , Chassard D , Cotte L , Chevallier M , Bernuau J , Lucet JC , Trepo C . Fulminant hepatitis with severe lactate acidosis in HIV-infected patients on didanosine therapy. J Intern Med. (1994) ;235: :367–371. |
[36] | Freneaux E , Fromently B , Berson A , et al. Stereoselective and nonstereoselective effects of ibuprofen enantiomers on mitochondrial beta-oxidation of fatty acids. J Pharmacol Exp Ther. (1990) ;255: (2):529–535. |
[37] | Aires C CP , IJlst L , Stet F , et al, Inhibition of hepatic carnitine palmitoyl-transferase I (CPT IA) by valproyl-CoA as a possible mechanism of valproate-induced steatosis. Biochem. Pharmacol. (2010) ;79: (5):792–799. |
[38] | Pandit MK , Burke J , Gustafson AB , Minocha A , Peiris AN . Drug-induced disorders of glucose tolerance. Ann Intern Med. (1993) ;118: :529–539. |
[39] | Farrell GC . Drugs and steatohepatitis. Semin Liver Dis. (2002) ;22: :185–194. |
[40] | Amacher DE , Chalasani N . Drug-induced hepatic steatosis. Semin Liver Dis. (2014) ;34: :205–214. |
[41] | Ramachandran R and Kakar S. Histological patterns in drug-induced liver disease. J Clin Pathol. (2009) ;62: :481–92. |
[42] | Zhao YC , Zhao GJ , Chen Z , She ZG , Cai J , Li H . Nonalcoholic fatty liver disease: An emerging driver of hypertension. Hypertens. (2020) ;75: :275–284. doi: 10.1161/HYPERTENSIONAHA.119.13419. |
[43] | Reddy JK . Non-alcoholic steatosis and steatohepatitis. III. Peroxisomal beta-oxidation, PPAR alpha, and steatohepatitis. Am J Physiol Gastrointest Liver Physiol. (2001) ;281: :G1333–G1339. |
[44] | Rao MS , Reddy JK . Peroxisomal beta-oxidation and steatohepatitis. Semin Liver Dis. (2001) ;21: :43–55. |
[45] | Guglielmi FW , Boggio-Bertinet D , Federico A , et al. Total parenteral nutrition-related gastroenterological complications. Dig Liver Dis. (2006) ;38: (9):623–642. |
[46] | Targher G , Byrne CD . Clinical review: Non-alcoholic fatty liver disease: A novel cardiometabolic risk factor for type 2 diabetes and its complications. J Clin Endocrinol Metab. (2013) ;98: :483–95. |
[47] | Browning JD , Szczepaniak LS , Dobbins R , Nuremberg P , Horton JD , Cohen JC , et al. Prevalence of hepatic steatosis in an urban population in the United States: Impact of ethnicity. Hepatol. (2004) ;40: :1387–95. |
[48] | Kotronen A , Juurinen L , Hakkarainen A , Westerbacka J , Cornér A , Bergholm R , et al. Liver fat is increased in type 2 diabetic patients and underestimated by serum alanine aminotransferase compared with equally obese nondiabetic subjects. Diabetes Care. (2008) ;31: :165–9. |
[49] | Armstrong MJ , Hazlehurst JM , Parker R , Koushiappi E , Mann J , Khan S , et al. Severe asymptomatic non-alcoholic fatty liver disease in routine diabetes care; a multi-disciplinary team approach to diagnosis and management. QJM. (2014) ;107: :33–41. |
[50] | Loomba R , Sanyal AJ . The global NAFLD epidemic. Nat Rev Gastroenterol Hepatol. (2013) ;10: :686–90. |
[51] | Rinella M , Charlton M . The globalization of nonalcoholic fatty liver disease: prevalence and impact on world health. Hepatol. (2016) ;64: :19–22. |
[52] | Stepanova M , Rafiq N , Makhlouf H , et al. Predictors of all-cause mortality and liver-related mortality in patients with nonalcoholic fatty liver disease (NAFLD). Dig Dis Sci. (2013) ;58: :3017–23. |
[53] | Matteoni CA , Younossi ZM , Gramlich T , et al. Nonalcoholic fatty liver disease: A spectrum of clinical and pathological severity. Gastroenterol. (1999) ;116: :1413–19. |
[54] | Wong RJ , Aguilar M , Cheung R , et al. Nonalcoholic steatohepatitis is the second leading etiology of liver disease among adults awaiting liver transplantation in the United States. Gastroenterol. (2015) ;148: :547–55. |
[55] | Lee JY , Kim KM , Lee SG , Yu E , Lim YS , Lee HC , Chung YH , Lee YS , Suh DJ . Prevalence and risk factors of non-alcoholic fatty liver disease in potential living liver donors in Korea: A review of 589 consecutive liver biopsies in a single center. J Hepatol. (2007) ;47: :239–244. |
[56] | Schwimmer JB , Deutsch R , Kahen T , Lavine JE , Stanley C , Behling C . Prevalence of fatty liver in children and adolescents. Pediatr. (2006) ;118: :1388–1393. |
[57] | Browning JD , Szczepaniak LS , Dobbins R , Nuremberg P , Horton JD , Cohen JC , Grundy SM , Hobbs HH . Prevalence of hepatic steatosis in an urban population in the United States: Impact of ethnicity. Hepatol. (2004) ;40: :1387–1395. |
[58] | Williams CD , Stengel J , Asike MI , Torres DM , Shaw J , Contreras M , Landt CL , Harrison SA . Prevalence of nonalcoholic fatty liver disease and nonalcoholic steatohepatitis among a largely middle-aged population utilizing ultrasound and liver biopsy: A prospective study. Gastroenterol. (2011) ;140: :124–131. |
[59] | Targher G , Lonardo A and Byrne C.D. Nonalcoholic fatty liver disease and chronic vascular complications of diabetes mellitus. Nat Rev Endocrinol. (2018) ;14: :99–114. |
[60] | Tilg H , Moschen AR , and Roden M . NAFLD and diabetes mellitus. Nat Rev Gastroenterol Hepatol. (2017) ;14: :32–42. |
[61] | Valenti L , Bugianesi, Pajvani U’ and Targher G. Nonalcoholic fatty liver disease: cause or consequence of type 2 diabetes? Liver Int. (2016) ;3: :1563–1579. |
[62] | Hallsworth K , and Adams LA . Lifestyle modification in NAFLD/NASH: facts and figures. . JHEP Rep. (2019) ;1: :468–479. |
[63] | Knowler WC et al. 10-year follow-up of diabetes incidence and weight loss in the diabetes prevention program outcomes study. Lancet. (2009) ;374: :1677–1686. |
[64] | Lindström J , et al. Sustained reduction in the incidence of type 2 diabetes by lifestyle intervention: follow-up of the Finnish Diabetes Prevention Study. Lancet. (2006) ;368: :1673–1679. |
[65] | Arab JP , Arrese M , Trauner M . Recent insights into the pathogenesis of nonalcoholic fatty liver disease. Annu Rev Pathol. (2018) ;13: :321–50. |
[66] | Romeo S , Kozlitina J , Xing C , Pertsemlidis A , Cox D , Pennacchio LA , Boerwinkle E , Cohen JC , Hobbs HH . Genetic variation in PNPLA3 confers susceptibility to nonalcoholic fatty liver disease. Nat Genet. (2008) ;40: :1461–5. |
[67] | Romero-Gomez M , Zelber-Sagi S , Trenell M . Treatment of NAFLD with diet, physical activity and exercise. J Hepatol. (2017) ;67: :829–46. |
[68] | Kozlitina J , Smagris E , Stender S , Nordestgaard BG , Zhou HH , TybjaergHansen A , Vogt TF , Hobbs HH , Cohen JC . Exome-wide association study identifies a TM6SF2 variant that confers susceptibility to nonalcoholic fatty liver disease. Nat Genet. (2014) ;46: :352–6. |
[69] | Byrne CD . Dorothy Hodgkin Lecture 2012: Nonalcoholic fatty liver disease, insulin resistance and ectopic fat: A new problem in diabetes management. Diabet Med. (2012) ;29: :1098–1107. |
[70] | Scorletti E and Byrne CD. Extrahepatic Diseases and NAFLD: the triangular relationship between NAFLD, type 2-diabetes and dysbiosis. Dig. Dis. (2016) ;34: (Suppl. 1):11–18. |
[71] | Kunnumakkara AB , Bordoloi D , Padmavathi G , Monisha J , Roy NK , Prasad S , Aggarwal BB . Curcumin, the golden nutraceutical: multitargeting for multiple chronic diseases. Br J Pharmacol. (2017) ;174: :1325–1348. |
[72] | Nabavi SF , Daglia M , Moghaddam AH , Habtemariam S , Nabavi SM . Curcumin and liver disease: From chemistry to medicine. Compr Rev Food Sci Food Saf. (2014) ;13: :62–77. |
[73] | Perrone D , Ardito F , Giannatempo G , Dioguardi M , Troiano G , Lo Russo L , De Lillo A , Laino L , Lo Muzio L . Biological and therapeutic activities, and anticancer properties of curcumin (Review). Exp Ther Med. (2015) ;10: :1615–1623. |
[74] | Prasad S , Aggarwal BB . Turmeric, the golden spice: from traditional medicine to modern medicine. In: Benzie IFF, Wachtel-Galor S, editors. Herb. Med Biomol Clin Asp. second ed. Boca Raton (FL): CRC Press/Taylor & Francis; 2011. http://www.ncbi.nlm.nih.gov/books/NBK92752/. [Accessed 18 May 2022]. |
[75] | Amalraj A , Pius A , Gopi S , Gopi S . Biological activities of curcuminoids, other biomolecules from turmeric and their derivatives e a review. J Tradit Complement Med. (2017) ;7: :205e33. https://doi.org/10.1016/j.jtcme.2016.05.005. |
[76] | Goel A , Kunnumakkara AB , Aggarwal BB . Curcumin as Curecumin: From kitchen to clinic. Biochem Pharmacol. (2008) ;75: :787e809. https://doi.org/10.1016/j.bcp.2007.08.016. |
[77] | Castro CN , Barcala Tabarrozzi AE , Winnewisser J , Gimeno ML , Antunica Noguerol M , Liberman AC , Paz DA , Dewey RA , Perone MJ . Curcumin ameliorates autoimmune diabetes. Evidence in accelerated murine models of type 1 diabetes. Clin Exp Immunol. (2014) ;177: :149–60. https://doi.org/10.1111/cei.12322. |
[78] | Poole KM , Nelson CE , Joshi RV , Martin JR , Gupta MK , Haws SC , Kavanaugh TE , Skala MC , Duvall CL . ROS-responsive microspheres for on demand antioxidant therapy in a model of diabetic peripheral arterial disease. Biomater. (2015) ;41: :166–75. https://doi.org/10.1016/j.biomaterials.2014.11.016. |
[79] | Trujillo J , Molina-Jijon E , Medina-Campos ON , Rodríguez-Murioz R , Reyes JL , Loredo ML , Barrera-Oviedo D , Pinzon E , Rodríguez-Rangel DS , Pedraza-Chaverri J . Curcumin prevents cisplatin-induced decrease in the tight and adherens junctions: relation to oxidative stress. Food Funct. (2016) ;279–93. https://doi.org/10.1039/C5FO00624D. |
[80] | Trujillo J , Chirino YI , Molina-Jijon E , Anderica-Romero AC , Tapia E , Pedraza-Chaverrí J . Renoprotective effect of the antioxidant curcumin: recent findings. Redox Biol. (2013) ;1: :448–56. https://doi.org/10.1016/j.redox.2013.09.003. |
[81] | K.S, Lee HJ, Jeong SH, Chung KH, Kim BI. Antibacterial photodynamic therapy with curcumin and Curcuma xanthorrhiza extract against Streptococcus mutans. Photodiagnosis Photodyn Ther. (2017) ;S1572-1000:30300-9. |
[82] | Sylvester WS , Son R , Lew KF , Rukayadi Y . Antibacterial activity of java turmeric (Curcuma xanthorrhiza Roxb.) extract against Klebsiella pneumoniae isolated from several vegetables. Int Food Res J. (2015) ;22: :1770–6. |
[83] | Bin He , Liu J , Xu Y , Zhao G . Synergistic anticancer effect of curcumin and chemotherapy regimen FP in human gastric cancer MGC-803 cells. Oncol Lett. (2017) ;14: :3387–94. https://doi.org/10.3892/ol.2017.6627. |
[84] | Furong L , Song G , Yang Y , Zhao X , Fan Y , Ma W , Yang D , Yang A , Yu Y . Curcumin induced autophagy anticancer effects on human lung adenocarcinoma cell line A549. Oncol Lett. (2017) ;14: :2775–82. https://doi.org/10.3892/ol.2017.6565. |
[85] | Montazeri P-SY , Mohaghegh M , Panahi A , Khodi Zarghami N , Sadeghizadeh M . Antiproliferative and apoptotic effect of dendrosomal curcumin nanoformulation in P53 mutant and wide-type cancer cell lines. AntiCancer Agents Med Chem. (2017) ;17: :662–73. |
[86] | Fan Z , Yao J , Li Y , Hu X , Shao H , Tian X . Anti-inflammatory and antioxidant effects of curcumin on acute lung injury in a rodent model of intestinal ischemia reperfusion by inhibiting the pathway of NF-KB. Int J Clin Exp Pathol. (2015) ;8: :3451–9. |
[87] | Gokce EC , Kahveci R , Gokce A , Sargon MF , Kisa U , Aksoy N , Cemil B , Erdogan B . Curcumin attenuates inflammation, oxidative stress, and ultrastructural damage induced by spinal cord ischemia-reperfusion injury in rats. J Stroke Cerebrovasc Dis. (2016) ;25: :1196–207. https://doi.org/10.1016/j.jstrokecerebrovasdis.2016.01.008. |
[88] | Griffiths K , Aggarwal BB , Singh RB , Buttar HS , Wilson D , De Meester F . Food antioxidants and their anti-inflammatory properties: A potential role in cardiovascular diseases and cancer prevention. Diseases. (2016) ;4: :28. https://doi.org/10.3390/diseases4030028. |
[89] | Li W , Suwanwela NC , Patumraj S . Curcumin prevents reperfusion injury following ischemic stroke in rats via inhibition of NF-kB, ICAM-1, MMP-9 and caspase-3 expression. Mol Med Rep. (2017) ;16: :4710e20. https://doi.org/10.3892/mmr.2017.7205. |
[90] | Noratiqah SB , Zulfarina MS , Qodriyah HM . Natural polyphenols in the treatment of Alzheimer’s disease. Curr Drug Targets. (2017) . https://doi.org/10.2174/1389450118666170328122527. |
[91] | van der Merwe C , van Dyk HC , Engelbrecht L , van der Westhuizen FH , Kinnear C , Loos B , Bardien S . Curcumin rescues a PINK1 knock down SH-SY5Y cellular model of Parkinson’s disease from mitochondrial dysfunction and cell death. Mol Neurobiol. (2017) ;54: :2752–62. https://doi.org/10.1007/s12035-016-9843-0. |
[92] | Guo S , Meng X , Yang X , Liu X , Ou-Yang C , Liu C . Curcumin administration suppresses collagen synthesis in the hearts of rats with experimental diabetes. Acta Pharmacol Sin. (2018) ;39: :195–204. https://doi.org/10.1038/aps.2017.92. |
[93] | Elmansi AM , Shishtawy MMEl , Eissa LA . Hepatoprotective effect of curcumin on hepatocellular carcinoma through autophagic and apoptic pathways. Ann Hepatol. (2017) ;16: :607–18. https://doi.org/10.5604/01.3001.0010.0307. |
[94] | Lee KS , Lee HY , Choi GH , Chung MK , Lee HW , Kim YC , Kwon HR , Chae HJ . Curcumin and Curcuma longa L. extract ameliorate lipid accumulation through the regulation of the endoplasmic reticulum redox and ER stress. . Sci Rep. (2017) ;7: :6513. https://doi.org/10.1038/s41598-017-06872-y. |
[95] | Aparicio-Trejo OE , Tapia E , Molina-Jijon E , Medina-Campos ON , Macías-Ruvalcaba NA , Leon-Contreras JC , Hernandez-Pando R , García-Arroyo FE , Cristobal M , Sanchez-Lozada LG , Pedraza-Chaverri J . Curcumin prevents mitochondrial dynamics disturbances in early 5/6 nephrectomy: relation to oxidative stress and mitochondrial bioenergetics. BioFactors. (2017) ;43: :293–310. https://doi.org/10.1002/biof.1338. |
[96] | Molina-Jijon E , Tapia E , Zazueta C , El Hafidi M , Zatarain-Barron ZL , Hernan-dez-Pando R , Medina-Campos ON , Zarco-Marquez G , Torres I , PedrazaChaverri J . Curcumin prevents Cr(VI)-induced renal oxidant damage by a mitochondrial pathway. Free Radic Biol Med. (2011) ;51: :1543–57. https://doi.org/10.1016/j.freeradbiomed.2011.07.018. |
[97] | Ortega-Domínguez B , Aparicio-Trejo OE , García-Arroyo FE , Leon-Contreras JC , Tapia E , Molina-Jijon E , Hernandez-Pando R , Sanchez-Lozada LG , Barrera-Oviedo D , Pedraza-Chaverri J . Curcumin prevents cisplatin-induced renal alterations in mitochondrial bioenergetics and dynamic. Food Chem Toxicol. (2017) ;107: :373–85. https://doi.org/10.1016/j.fct.2017.07.018. |
[98] | Panahi Y , Khalili N , Sahebi E , Namazi S , Reiner Z , Majeed M , Sahbekar A . Curcuminoids modify lipid profile in type 2 diabetes mellitus: A randomized controlled trial. Complement. Ther Med. (2017) ;33: :1–5. https://doi.org/10.1016/j.ctim.2017.05.006. |
[99] | Rashid K , Chowdhury S , Ghosh S , Sil PC . Curcumin attenuates oxidative stress induced NFkB mediated inflammation and endoplasmic reticulum dependent apoptosis of splenocytes in diabetes. Biochem Pharmacol. (2017) ;1: :140–55. https://doi.org/10.1016/j.bcp.2017.07.009. |
[100] | Petersen MC , Shulman GI . Mechanisms of Insulin Action and Insulin Resistance. Physiol Rev. (2018) ;98: :2133–2223. |
[101] | Sweet IR , Cook DL , DeJulio E , Wallen AR , Khalil G , Callis J , Reems J . Regulation of ATP/ADP in Pancreatic Islets. Diabetes. (2004) ;53: :401–409. |
[102] | Tripathy D , Chavez AO . Defects in insulin secretion and action in the pathogenesis of type 2 diabetes mellitus. Curr Diabetes Rep. (2010) ;10: :184–191. |
[103] | Saltiel AR . New perspectives into the molecular pathogenesis and treatment of type 2 diabetes. Cell. (2001) ;104: :517–529. |
[104] | Alam MA , Subhan N , Rahman MM , Uddin SJ , Reza HM , Sarker SD . Effect of citrus flavonoids, naringin and naringenin, on metabolic syndrome and their mechanisms of action. Adv Nutr. (2014) ;5: :404–417. |
[105] | Lee CH , Olson P , Hevener A , Mehl I , Chong LW , Olefsky JM , Gonzalez FJ , Ham J , Kang H , Peters JM , et al. PPAR regulates glucose metabolism and insulin sensitivity. Proc Natl Acad Sci. USA. (2006) ;103: :3444–3449. |
[106] | Defronzo RA . Banting Lecture. From the triumvirate to the ominous octet: A new paradigm for the treatment of type 2 diabetes mellitus. Diabetes. (2009) ;58: :773–795. |
[107] | Frigolet ME , Torres N , Tovar AR . The renin-angiotensin system in adipose tissue and its metabolic consequences during obesity. J Nutr Biochem. (2013) ;24: :2003–2015. |
[108] | Hotamisligil GS , Spiegelman BM . Tumor necrosis factor alpha: A key component of the obesity-diabetes link. Diabetes. (1994) ;43: :1271–1278. |
[109] | Cho NH , Shaw JE , Karuranga S , Huang Y , da Rocha Fernandes JD , Ohlrogge AW , Malanda B . IDF Diabetes Atlas: Global estimates of diabetes prevalence for 2017 and projections for 2045. Diabetes Res Clin Pract. (2018) ;138: :271–281. |
[110] | Baur JA , Sinclair DA . Therapeutic potential of resveratrol: The in vivo evidence. Nat Rev Drug Discov. (2006) ;5: :493–506. |
[111] | Park EJ , Pezzuto JM . The pharmacology of resveratrol in animals and humans. Biochim. Biophys. Acta. (2015) ;1852: :1071–1113. |
[112] | Anja B , Laura R . The cost of diabetes in Canada over 10 years: Applying attributable health care costs to a diabetes incidence prediction model. Health Promot Chronic Dis Prev Can. (2017) ;37: :49–53. |
[113] | Yahfoufi N , Alsadi N , Jambi M , Matar C . The Immunomodulatory and Anti-Inflammatory Role of Polyphenols. Nutrients. (2018) ;10: :1618. |
[114] | Kocaadam B , Sanlier N . Curcumin, an active component of turmeric (Curcuma longa), and its effects on health. Crit Rev Food Sci Nutr. (2017) ;57: :2889–2895. |
[115] | Chattopadhyay I , Biswas K , Bandyopadhyay U , Banerjee RK . Turmeric and curcumin: Biological actions and medicinal applications. Curr Sci. (2004) ;87: :10. |
[116] | Reddy AC , Lokesh BR . Studies on spice principles as antioxidants in the inhibition of lipid peroxidation of rat liver microsomes. Mol Cell. Biochem. (1992) ;111: :117–124. |
[117] | Lone J , Choi JH , Kim SW , Yun JW . Curcumin induces brown fat-like phenotype in 3T3-L1 and primary white adipocytes. J Nutr Biochem. (2016) ;27: :193–202. |
[118] | Pan Y , Zhao D , Yu N , An T , Miao J , Mo F , Gu Y , Zhang D , Gao S , Jiang G . Curcumin improves glycolipid metabolism through regulating peroxisome proliferator activated receptor γ signaling pathway in high-fat diet-induced obese mice and 3T3-L1 adipocytes. R Soc Open Sci. (2017) ;4: :170917. |
[119] | Priyanka A , Shyni GL , Anupama N , Raj PS , Anusree SS , Raghu KG . Development of insulin resistance through sprouting of inflammatory markers during hypoxia in 3T3-L1 adipocytes and amelioration with curcumin. Eur J Pharmacol. (2017) ;812: :73–81. |
[120] | Eslam M. and George J. Genetic contributions to NAFLD: leveraging shared genetics to uncover systems biology. Nat. Rev. Gastroenterol. Hepatol. (2020) ;17: (1):40–52. |
[121] | Na LX , Yan BL , Jiang S , Cui HL , Li Y , Sun CH . Curcuminoids target decreasing serum adipocyte-fatty acid binding protein levels in their glucose-lowering effect in patients with type 2 diabetes. Biomed Environ Sci. (2014) ;27: :902e6. https://doi.org/10.3967/bes2014.127. |
[122] | Kralisch S , Ebert T , Lossner U , Jessnitzer B , Stumvoll M , Fasshauer M . Adipocyte fatty acid-binding protein is released from adipocytes by a nonconventional mechanism. Int J Obes. (2014) ;38: :1251e4. https://doi.org/10.1038/ijo.2013.232. |
[123] | Graupera I , Coll M , Pose E , Elia C , Piano S , Sol a E , Blaya D , Huelin P , Sole C , Moreira R , de Prada G , Fabrellas N , Juanola A , Morales-Ruiz M , Sancho-Bru P , Villanueva C , Gines P . Adipocyte fatty-acid binding protein is overexpressed in cirrhosis and correlates with clinical outcomes. Sci Rep. (2017) . https://doi.org/10.1038/s41598-017-01709-0. |
[124] | Nelson KM , Dahlin JL , Bisson J , Graham J , Pauli GF , Walters MA . The essential medicinal chemistry of curcumin: miniperspective. J Med Chem. (2017) ;60: :1620e37. https://doi.org/10.1021/acs.jmedchem.6b00975. |
[125] | Zhu J , Sanidad KZ , Sukamtoh E , Zhang G . Potential roles of chemical degradation in the biological activities of curcumin. Food Funct. (2017) ;8: :907e14. https://doi.org/10.1039/C6FO01770C. |