Ankle rehabilitation robot training for stroke patients with foot drop: Optimizing intensity and frequency
Abstract
BACKGROUND:
Robotic solutions for ankle joint physical therapy have extensively been researched. The optimal frequency and intensity of training for patients when using the ankle robot is not known which can affect rehabilitation outcome.
OBJECTIVE:
To explore the optimal ankle robot training protocol on foot drop in stroke subjects.
METHODS:
Subjects were randomly divided into four groups, with 9 in each group. The subjects received different intensities (low or high intensity) with frequencies (1 session/day or 2 sessions/day) of robot combination training. Each session lasted 20 minutes and all subjects were trained 5 days a week for 3 weeks.
RESULTS:
After 3 weeks of treatment, all groups showed an improvement in passive and active ankle dorsiflexion range of motion (PROM and AROM) and Fugl-Meyer Assessment for lower extremity (FMA-LE) compared to pre-treatment. When training at the same level of intensity, patients who received 2 sessions/day of training had better improvement in ankle dorsiflexion PROM than those who received 1 session/day. In terms of the improvement in dorsiflexion AROM and FMA-LE, patients who received 2 sessions/day with high intensity training improved better than other protocols.
CONCLUSION:
High frequency and high intensity robot training can be more effective in improving ankle dysfunction.
1Introduction
Stroke is the third leading cause of morbidity and mortality worldwide, and approximately 80% of stroke survivors suffer from varying degrees of limb movement disorders after a stroke occur (Brewer et al., 2013). Foot drop is a common lower extremity sequela of stroke and at least 20% to 30% of post-stroke patients have foot drop (Peishun et al., 2021). Patients with stroke are unable to dorsiflex or have inadequate dorsiflexion due to triceps spasm and ankle dorsiflexion muscle weakness, resulting in a “circling gait” when walking (Fernandez-Gonzalez et al., 2016; Nikamp et al., 2018). The compensatory gait pattern affects balance and walking efficiency, and increases the risk of falls, thus restricting their participation in many activities of daily life and in the community (de Haart et al., 2004; Mao et al., 2022). Therefore, it is crucial to discover effective treatments for foot drop that facilitate the recovery of lower limb functions in individuals with hemiplegia, thereby enhancing their quality of life and facilitating reintegration into family and society.
In traditional ankle exercises, physiotherapists manually manipulate the affected ankle to perform internal/external rotation, dorsiflexion/plant-arflexion, and inversion/eversion movements during ankle rehabilitation (Park et al., 2019). The reliance on manual training methods heavily depends on the hands-on skills and experience of the therapists. This approach presents challenges in providing targeted, repeated, and intense rehabilitation training, as well as inhibiting real-time monitoring of the effects of rehabilitation treatment (Hussain et al., 2021). Ankle rehabilitation robots represent a novel intelligent intervention for enhancing post-stroke ankle dysfunction. This advanced approach offers personalized, high-intensity, multi-modal rehabilitation training, which is efficient and time-saving. Extensive clinical studies have been conducted to investigate its effectiveness in neurological rehabilitation (Yoo et al., 2018; Alvarez-Perez et al., 2020). Wearable robotic orthoses/exoskeletons and end-effector-based parallel robotic mechanisms are two major research directions in the field of ankle rehabilitation assistance (Jamwal et al., 2015; Hussain et al., 2016). Several clinical trials have consistently shown the potential of ankle rehabilitation robots in improving foot drop (Renfrew et al., 2019; Zhai et al., 2021). However, to date, there is a lack of research exploring the optimal training protocols for ankle robot-assisted training, making it challenging for patients to receive precise treatment during their recovery.
This study aims to compare four different training methods with varying frequencies and intensities, utilizing a parallel structured robotic system. The primary objective is to investigate the optimal approach for quantitative ankle motion training, thereby providing guidelines for robotic rehabilitation training in the clinical setting.
2Methods
2.1Participants
This was a single-centre, single-blind, prospective, randomized controlled trial with a two-by-two factorial design (Figs. 1 and 2). A total of 36 post-stroke foot drop subjects who were admitted to the Inpatient Rehabilitation Center of Shenzhen Second People’s Hospital between December 2020 and October 2021 were recruited. All subjects were diagnosed with stroke, as confirmed by computed tomography or magnetic resonance imaging. The main inclusion criteria for subjects were as follows: (1) first episode of unilateral stroke with hemiparesis, (2) the duration of disease ranged from 2 weeks to 12 months, (3) age between 18 to 75 years, (4) adequate cognitive function to follow simple verbal instructions, and (5) presence of voluntary motor ability on the healthy side. The exclusion criteria were (1) limb dysfunction due to other causes than stroke, (2) unable to cooperate with training or poor compliance with treatment, (3) fractures, infections or active bleeding in the affected ankle joint, and (4) a fixed contracture at the affected ankle. All subjects provided written informed consent.
Fig. 1
2×2 factorial trial design.
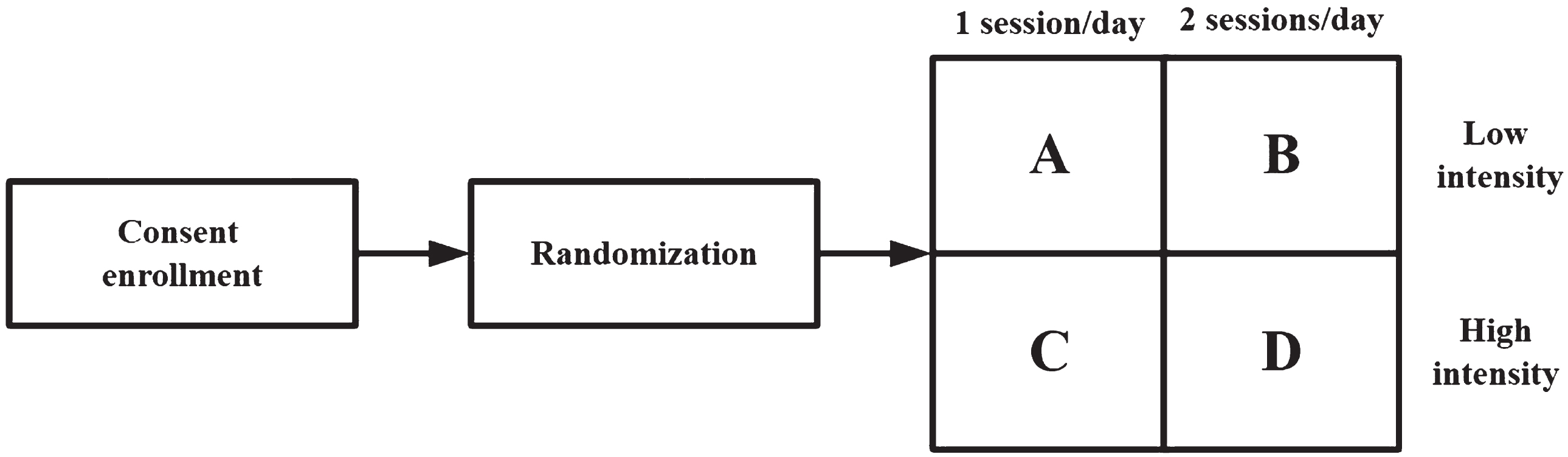
Fig. 2
Flow diagram of the study.
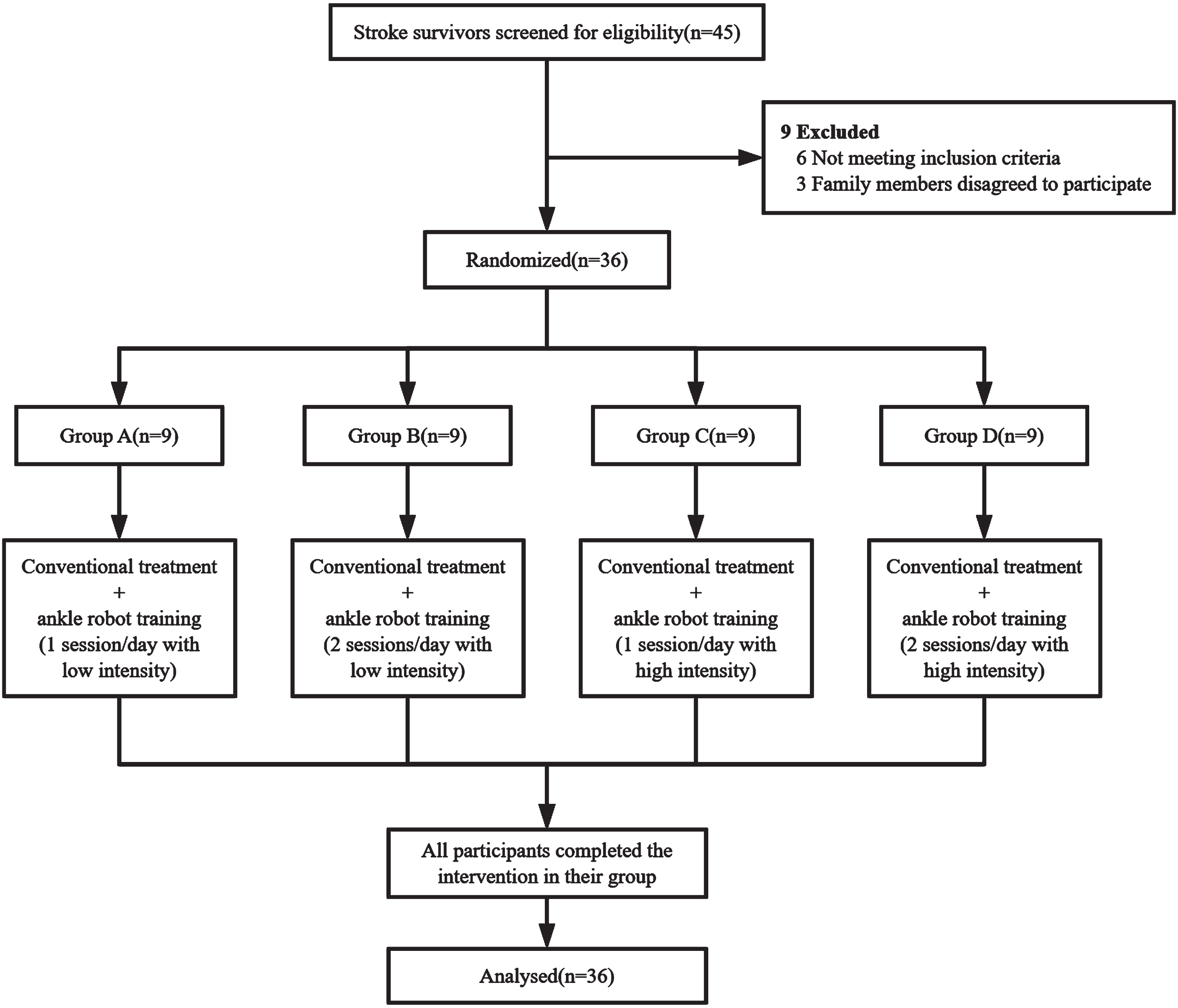
2.2Ankle intelligent rehabilitation robot
The Ankle Intelligent Rehabilitation Robot (Guangdong Minkai Medical Robotics Co., Ltd., Zhuhai, China) is a parallel robot configuration-based ankle robot for rehabilitation in the seated position (Liu et al., 2018). The device consists of a robot body, a chair, a control cabinet and a display system. The robot body has two symmetrical laminated platforms which can provide assistance for patients to exercise all three degrees of freedom (DOFs) including internal/external rotation, dorsiflexion/plantarflexion, and inversion/eversion movements to the ankle-foot complex (Fig. 3). Patients sit in the chair and their feet are placed on the platforms which allow them to use their able ankle joint to teach the affected ankle joint to perform ankle exercises under the guidance of a physical therapist. The included virtual reality software provides interactive game training with audio-visual feedback.
Fig. 3
a Ankle intelligent rehabilitation robot, b Robot body.
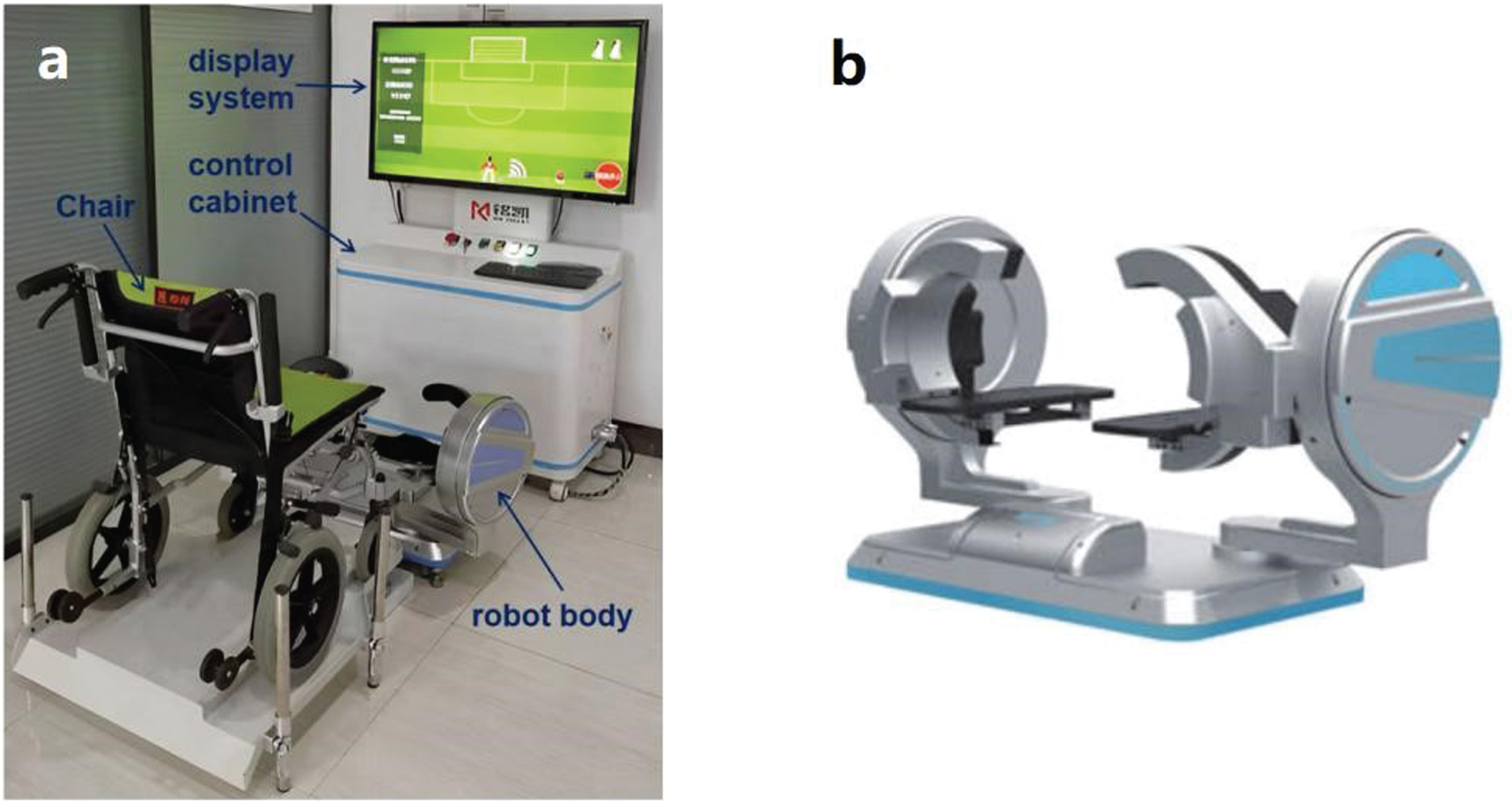
2.3Interventions
All subjects underwent a combination of conventional stroke rehabilitation programs and robot training. The conventional rehabilitation programs were conducted 4 hours/day and 5 days/week for 3 weeks. It consisted of physiotherapy, occupational therapy and physical therapy provided by allied healthcare workers.
Subjects were randomly assigned to four groups: Group A (n = 9), Group B (n = 9), Group C (n = 9), and Group D (n = 9) to receive robot-assisted ankle joint training of different frequencies and intensities (Fig. 1). The training parameters for each group were as follows: Group A received 1 session/day with low intensity, Group B received 2 sessions/day with low intensity, Group C received 1 session/day with high intensity, and Group D received 2 sessions/day with high intensity (Fig. 2). The duration of 1 session was 20 minutes and the resistance was 1Nm for low intensity and 5Nm for high intensity. This training was performed 5 days/week for 3 weeks using the Ankle Intelligent Rehabilitation Robot.
Randomization of participants was performed by an author who was not involved in the intervention. He/she used a computer random number generator to create the randomization sequence and constructed sealed envelopes containing the assignments. Due to the nature of the therapy, it was not possible to implement blinding for either the patients or the therapists.
Prior to the initial session, participant’s passive and active range of motion (PROM and AROM) of three DOFs were measured in the bilateral ankle joint in order to establish individual parameters for ankle exercises. In the training, participants sat in the treatment chair with their torso relaxed and facing the robotic display. The height of the chair was adjusted and they were assisted to secure their feet on the platforms. In a treatment session, patients underwent 5 minutes of flexibility training for the three DOFs followed by 5 minutes of low/high intensity ankle dorsiflexion training. Then, these two 5-minute-training were repeated.
In the first 5 minutes of flexibility training, subjects were asked to relax and follow the movement imposed by the robot in a slow pace continuously. The robot applied torque according to individual parameters to stretch calf muscles toward participant’s extreme limits. In this mode, training range of motion corresponded with the PROM of the affected ankle joint. The extreme limits could be adjusted by the research assistant when the robot detected restricted mobility.
In the second 5 minutes of ankle dorsiflexion training, it involved active resistance training. The affected ankle, led by the robot, synchronized its movements with the healthy ankle in a mirror-image fashion. Patients actively performed ankle dorsiflexion while resisting low/high-intensity resistance. The robot’s range of movement corresponded with the AROM of the healthy ankle and the extreme limits could be modified when the robot encountered restricted mobility.
2.4Outcome measures
Clinical assessments included pre-assessment (baseline, before the first exercise session) and post-assessment (after the last exercise session). All participants were evaluated for the PROM and AROM of dorsiflexion on the affected side using sensors, and their lower-extremity motor function was assessed using Fugl-Meyer Assessment for lower extremity (FMA-LE). All assessors who evaluated the participants were blinded to the intervention allocation.
The PROM and AROM were measured using two inertial sensors (LP-RESEARCH Inc., Tokyo, Japan) (Sun et al., 2017). Subjects were in a seated position with the knee at 90° of natural flexion and two sensors were attached to the middle of the lower affected limb (on the skin over the middle of the tibia at the anterior side) and to the top of the affected foot (on the skin over the middle cuneiform bone). Measurements were taken from the position midway between dorsiflexion and plantarflexion, i.e., at 0° of dorsiflexion. PROM was the range in which the subjects’ ankle was moved from a neutral position to maximum dorsiflexion until any resistance was felt, while AROM required the subjects to move the affected foot upward toward the calf as far as possible. All participants completed 3 sets of ankle PROM and AROM measurements with a 20s resting time between each measurement. The final PROM and AROM were the average of the 3 measurements.
The FMA-LE is designed to evaluate lower limbs motor functions including balance, sensation and joint function in hemiplegic post-stroke subjects (Sullivan et al., 2011). It consists of 7 assessing items. The maximum score is 34, and higher scores indicate better motor function of the lower limbs. All participants were assessed by three occupational therapists who were not involved in the intervention. The final FMA score was the average score of the three assessing scores to reduce bias.
2.5Statistical analysis
All data were analyzed using SPSS version 25 (IBM Corp., Armonk, NY, USA). Subject characteristics and outcome variables between groups using One-Way analysis of variance (ANOVA) or the Fisher Exact test. Because the disease duration did not conform to a normal distribution, the Wilcoxon rank-sum test was used. Normality and variance homogeneity of the data were assessed using the Shapiro-Wilk test and Levene’s test. Paired t-tests were used to compare the means of the pre-and post-treatment changes. Between-group differences in outcome variables after 3 weeks of training were analyzed using a general linear model including frequency (1 session/day or 2 sessions/day), intensity (low intensity or high intensity) as fixed factors, post-training PROM, AROM and FMA-LE as the dependent variable, and subjects as random factors. For all outcome variables, the interaction for frequency×intensity was tested. P < 0.05 was considered statistically significant.
3Results
A total of 45 stroke patients were screened for eligibility and 36 were included in our study. All subjects completed the study and no serious adverse event or important harm was reported (Fig. 2).
3.1Baseline
The average age of the study population was 54.92(10.61), and the majority (86.11%) of them were males. A summary of the demographic and clinical features of the subjects is shown in Table 1. There was no significant difference in age, gender, duration of stroke, side of hemiplegia, and stroke type at baseline in any of the groups (p > 0.05). Table 1 also demonstrates the outcome variables of baseline in four groups and no statistically significant difference was found in the baseline assessment.
Table 1
Demographic characteristics and clinical assessment of PROM, AROM and FMA-LE at baseline
Characteristics | Group A (n = 9) | Group B (n = 9) | Group C (n = 9) | Group D (n = 9) | p-value |
Age, mean (SD), years | 57.11(9.37) | 53.22(9.65) | 53.67(14.03) | 55.67(10.16) | 0.864 |
Gender, n, male/female | 8/1 | 7/2 | 7/2 | 9/0 | 0.465 |
Stroke type, n, ischemic/hemorrhagic | 6/3 | 3/6 | 5/4 | 6/3 | 0.440 |
Stroke duration before screening, mean (SD), days | 126.14(48.15) | 131.29(86.29) | 117.43(94.20) | 103.25(67.40) | 0.897 |
Affected limb, n, left/right | 2/7 | 3/6 | 4/5 | 4/5 | 0.871 |
PROM, mean (SD) | 25.11(3.17) | 24.94(6.63) | 26.82(4.25) | 26.90(5.81) | 0.766 |
AROM, mean (SD) | 6.97(3.70) | 7.96(3.41) | 8.73(3.79) | 8.83(3.86) | 0.689 |
FMA-LE, mean (SD) | 17.33(3.87) | 16.07(5.02) | 17.31(3.68) | 16.89(5.36) | 0.740 |
PROM: passive range of motion; AROM: active range of motion; FMA-LE: Fugl-Meyer Assessment for lower extremity.
3.2Comparison of PROM in four groups
After 3 weeks of treatment, the PROM of dorsiflexion improved in all four groups compared to pre-treatment (p < 0.05) (Table 2). A significant difference was observed in two frequency levels (F = 15.327, p = 0.00, eta2 = 0.324) while there was no significant difference between the two intensity levels (F = 1.476, p = 0.233, eta2 = 0.044). In addition, no significant interaction effect was found in frequency×intensity (F = 0.017, p = 0.896, eta2 = 0.001). Among subjects who received the same level of intensity training, patients who received 2 sessions/day showed more significant improvement in dorsiflexion PROM than those who received 1 session/day (Table 3).
Table 2
Clinical assessment of PROM, AROM and FMA-LE at baseline (Pre) and the changes after the ankle robot training (Post)
Outcome Measures | Group A (n = 9) | Group B (n = 9) | Group C (n = 9) | Group D (n = 9) | p-valuea |
PROM | |||||
Pre | 25.11(3.17) | 24.94(6.63) | 26.82(4.25) | 26.90(5.81) | 0.766 |
Post | 28.42(3.51)* | 34.40(3.25)* | 30.41(4.70)* | 36.56(4.69)* | 0.003 |
AROM | |||||
Pre | 6.97(3.70) | 7.96(3.41) | 8.73(3.79) | 8.83(3.86) | 0.689 |
Post | 9.17(2.64)* | 13.87(4.17)* | 11.93(3.76)* | 16.90(4.12)* | 0.001 |
FMA-LE | |||||
Pre | 17.33(3.87) | 16.07(5.02) | 17.31(3.68) | 16.89(5.36) | 0.740 |
Post | 19.19(2.52)* | 24.14(3.21)* | 22.66(2.39)* | 27.36(3.45)* | 0.000 |
Values are mean (SD). *p < 0.05, significance level of differences with-in groups between Pre and Post using the t-test. a Significance level of differences between four groups using One-Way ANOVA. PROM: passive range of motion; AROM: active range of motion; FMA-LE: Fugl-Meyer Assessment for lower extremity.
Table 3
Results of ANOVA of frequency and intensity on PROM, AROM and FMA-LE after 3 weeks of intervention
Low intensity | High intensity | Frequency | Intensity | Frequency×Intensity interaction | |||||||
F | p | eta2 | F | p | eta2 | F | p | eta2 | |||
PROM | |||||||||||
1 session/day | 28.42 (3.51) | 30.41 (4.70) | 15.327 | 0.000 | 0.324 | 1.476 | 0.233 | 0.044 | 0.017 | 0.896 | 0.001 |
2 sessions/day | 34.40 (3.25) | 36.56 (4.69) | |||||||||
AROM | |||||||||||
1 session/day | 9.17 (2.64) | 11.93 (3.76) | 5.443 | 0.026 | 0.145 | 15.144 | 0.000 | 0.321 | 0.013 | 0.912 | 0.000 |
2 sessions/day | 13.87 (4.17) | 16.90 (4.12) | |||||||||
FMA-LE | |||||||||||
1 session/day | 19.19 (2.52) | 22.66 (2.39) | 24.473 | 0.000 | 0.433 | 11.736 | 0.002 | 0.268 | 0.018 | 0.894 | 0.001 |
2 sessions/day | 24.14 (3.21) | 27.36 (3.45) |
PROM: passive range of motion; AROM: active range of motion; FMA-LE: Fugl-Meyer Assessment for lower extremity.
3.3Comparison of AROM in four groups
After 3 weeks of treatment, dorsiflexion AROM improved in all four groups compared to pre-treatment (p < 0.05) (Table 2). There was a significant difference between the two levels of intensity (F = 15.144, p = 0.000, eta2 = 0.321) and frequency (F = 5.443, p = 0.026, eta2 = 0.145), with no significant interaction effect between frequency×intensity (F = 0.013, p = 0.912, eta2 = 0.000). Patients who received 2 sessions/day with high intensity robot training showed a significant improvement in dorsiflexion AROM compared to those who received 1 session/day with low intensity, 2 sessions/day with low intensity and 1 session/day with high intensity, suggesting a superimposed effect of frequency combined with intensity (Table 3).
3.4Comparison of FMA-LE in four groups
After 3 weeks of treatment, all 4 groups showed an improvement in FMA-LE scores compared to pre-treatment (p < 0.05) (Table 2). There was a significant difference between the two levels of frequency (F = 24.47, p = 0.000, eta2 = 0.433) and intensity (F = 11.74, p = 0.002, eta2 = 0.268), with no significant interaction between frequency×intensity (F = 0.018, p = 0.894, eta2 = 0.001). Patients who received high intensity robotic training at 2 sessions/day showed a significant improvement in FMA-LE compared to the other 3 groups, suggesting a superimposed effect of frequency combined with intensity (Table 3).
4Discussion
This study aims to explore the optimal training scheme with Ankle Intelligent Rehabilitation Robot to guide its clinical application. Previous investigations of ankle robots in subjects with post-stroke foot drop primarily focused on robot development and validating their clinical efficacy. To our knowledge, this is the first study to compare the impact of trainings with different frequencies and intensities using an ankle robot in individuals with post-stroke foot drop. When training at the same level of intensity, patients who received 2 sessions/day training had better improvement in dorsiflexion PROM of the affected ankle than those who received 1 session/day. In addition, patients who received 5Nm and 2 sessions/day of robotic training showed more significant improvement in both AROM and FMA-LE compared to the other 3 groups.
A number of previous studies confirmed that passive stretching was effective in expanding the ankle range of motion by reducing the triceps surae muscle spasticity (Zhao et al., 2011; Freitas et al., 2015; Lecharte et al., 2020). Our study further explored the efficacy of passive stretching, utilizing robotic equipment that provided “flexibility training”. Our findings revealed that a higher frequency of flexibility training proved more effective in enhancing ankle dorsiflexion PROM. This can be explained as large repetitions of passive training may be more effective in relieving muscle tension and stiffness, enhancing muscle elasticity and flexibility, and improving neuromuscular coordination, which was more helpful in reducing muscle spasticity and increasing PROM. A study of Thibaut et al. (2018) had similar results in traumatic brain injury patients with disorders of consciousness. They identified a negative correlation between the frequency of stretching and the severity of spasticity. They also found that subjects who stretched more than 4 sessions per week experienced better relief muscle spasticity compared to those who stretched less than 4 sessions. However, the work that has been done on the effect of stretching frequency on muscle spasticity after stroke is scarce. Our findings may provide some insights for the development of training programs to improve spasticity in patients with stroke.
The intensity of resistance, on the other hand, was found not significantly meaningful for improving PROM in this study. This was probably because the low/high intensity in this study was applied in ankle dorsiflexion training, which was active resistance training. The intensity of the resistance mainly focuses on muscle strength and muscle mass gains and is not closely related to the reduction of muscle spasticity (Lasevicius et al., 2018).
Our results also showed that both the frequency and intensity of robotic training had a significant effect on improving ankle dorsiflexion AROM, with a superimposed effect observed between the two factors. Specifically, 2 sessions/day with high intensity training exhibited greater effectiveness. One plausible explanation was that the higher frequency of robotic training strengthened the dorsiflexors, which in turn improved the ankle AROM. A meta-analysis conducted by Grgic et al. (2018) found that increasing the frequency of resistance training could significantly enhance muscle strength gains and this was observed when comparing resistance training programs with different frequencies (1, 2, 3, and ≥4 days per week) in healthy adults. In addition, a study utilizing electromyography (EMG) also confirmed a greater muscular activation in high- versus low-intensity, suggesting that the full spectrum of motor units may not be fully stimulated when training at a lower intensity (Schoenfeld et al., 2014). Furthermore, from a neurological perspective, engaging in high-intensity repetitive training could enhance neuroplasticity and facilitate motor relearning in stroke patients (Langhorne et al., 2009; French et al., 2016). Leech et al. (2017) also discovered a positive correlation between exercise intensity and serum concentration of brain-derived neurotrophic factor (BDNF), which served as a peripheral blood indicator for nerve remodeling. The elevated levels of BDNF suggested that higher intensity rehabilitation treatments could facilitate more effective nerve remodeling. Thus, this superimposed effect of frequency and intensity may have a greater ameliorative effect on ankle dorsiflexion AROM.
The 2 sessions/day with high intensity robotic training was also found effective in improving FMA-LE scores. Ankle dorsiflexion plays a key role in the motor function of lower limbs and affects activities of daily life such as walking. Training with higher frequency and intensity in rehabilitation could lead to better improvements in PROM and AROM of ankle dorsiflexion which allowed for more effective gait training. This contributed to the improvement of lower limb motor function. Similar findings can be found in Forrester et al.’s (2013) study. They applied a seated ankle robot training device for patients with stroke which could successfully improve ankle-foot movement angles and increase walking speeds, thus enhancing lower limb motor function.
There is a growing trend towards incorporating robots into traditional rehabilitation practice. Ankle rehabilitation robots can play an important role due to their distinctive features, including repetitive and safe sensory motor stimulation, precise and effective motor training patterns, and the provision of objective and accurate motor feedback (Zhang et al., 2013; Payedimarri et al., 2022). The robotic device utilized in this study was developed based on the mirror neuron theory. In addition to offering passive activities, a distinctive combined training mode was developed, involving both the healthy and affected side. In this mode, the healthy side drives the affected side to perform repeated active ankle dorsiflexion movements (Liu et al., 2018). This approach enables patients to engage in coordinated and controlled movements of both ankles, facilitating biomechanical balance in the muscle groups surrounding both feet. Furthermore, the incorporation of virtual reality training, utilizing computer games, offers visual and auditory feedback, effectively enhancing patient motivation during the training sessions (Kayabinar et al., 2021). To ensure optimal therapeutic outcomes, it is essential to establish clear protocols guiding its clinical application. Our findings can provide evidence for policymakers when formulating such a protocol. The results are not only applicable to the device but can also be relevant and valuable for the implementation of similar ankle robotic devices in clinical settings.
This study has several limitations. First, the small sample size of participants might limit the results. Second, we only used PROM, AROM and FMA-LE to evaluate the ankle function and lack of measurements for relevant muscle (e.g., tibialis anterior and triceps surae) kinematics and kinetics. The assessment of balance function and gait was also lacking. Third, we have only compared two levels of frequency and intensity of robot training, while other levels have not been investigated. Additionally, the training duration was 20 minutes for all four protocols, and the effect of different training durations was not studied. In future research, we will conduct a multi-centre study containing a larger sample size for further verification. More assessment metrics will be added for a multi-faceted study such as the use of EMG to collect information regarding muscle activation around the ankle joint and the evaluation of balance and gait to assess overall motor function. In addition, the effect of different training durations and levels of frequency and intensity will be compared to explore a more effective robot training programme.
5Conclusion
Utilizing an Ankle Intelligent Rehabilitation Robot, this study explored the optimal solution for quantitative exercise training of stroke patients with foot drop for the needs of clinical rehabilitation. In light of this study, we suggest that the frequency of 2 sessions/day ankle robot training could be used to expand PROM and the frequency of 2 sessions/day and the intensity of 5Nm to train could be used to improve AROM and lower limb motor function. In addition, the findings of this study can serve as valuable references for the clinical application of other ankle joint robotic devices.
Acknowledgments
The authors would like to thank all researchers and participants involved in this study. They furthermore express their gratitude to Guangdong Mingkai Medical Robot Co., Ltd., for developing the Ankle Intelligent Rehabilitation Robot and guiding the use of the equipment.
Conflict of interest
The authors declare no competing interests.
Funding
This study was supported by the Emergency Clinical Research Project on New Coronavirus Infection at Shenzhen Second People’s Hospital (2023xgyj3357006), Guangdong Medical Research Foundation (A2021169), and a grant from the Sanming Project of Medicine in Shenzhen (SZSM202111010).
Ethics statement
This study was registered at the Chinese Clinical Trial Registry (ID number: ChiCTR2000034479). The research protocol was approved by the Ethical Committee of Shenzhen Second People’s Hospital, China (approval number: 20200601016-XZ01-FS01). The study was designed following the principles of the Declaration of Helsinki. All subjects provided written informed consent.
References
1 | Alvarez-Perez, M. G. , Garcia-Murillo, M. A. , Cervantes-Sánchez, J. J. ((2020) ). Robot-assisted ankle rehabilitation: A review. Disabil Rehabil Assist Technol, 15: , 394–408. |
2 | Brewer, L. , Horgan, F. , Hickey, A. , Williams, D. ((2013) ). Stroke rehabilitation: Recent advances and future therapies. Qjm, 106: , 11–25. |
3 | de Haart, M. , Geurts, A. C. , Huidekoper, S. C. , Fasotti, L. , van Limbeek, J. ((2004) ). Recovery of standing balance in postacute stroke patients: A rehabilitation cohort study. Arch Phys Med Rehabil, 85: , 886–895. |
4 | Fernandez-Gonzalez, P. , Molina-Rueda, F. , Cuesta-Gomez, A. , Carratala-Tejada, M. , Miangolarra-Page, J. C. ((2016) ). Instrumental gait analysis in stroke patients. Revista de Neurologia, 63: , 433–439. |
5 | Forrester, L. W. , Roy, A. , Goodman, R. N. , Rietschel, J. , Barton, J. E. , Krebs, H. I. , Macko, R. F. ((2013) ). Clinical application of a modular ankle robot for stroke rehabilitation. NeuroRehabilitation, 33: , 85–97. |
6 | Freitas, S. R. , Vilarinho, D. , Rocha Vaz, J. , Bruno, P. M. , Costa, P. B. , Mil-homens, P. ((2015) ). Responses to static stretching are dependent on stretch intensity and duration. Clinical Physiology and Functional Imaging, 35: , 478–484. |
7 | French, B. , Thomas, L. H. , Coupe, J. , McMahon, N. E. , Connell, L. , Harrison, J. , Sutton, C. J. , Tishkovskaya, S. , Watkins, C. L. ((2016) ). Repetitive task training for improving functional ability after stroke. The Cochrane Database of Systematic Reviews, 11: , Cd006073. |
8 | Grgic, J. , Schoenfeld, B. J. , Davies, T. B. , Lazinica, B. , Krieger, J. W. , Pedisic, Z. ((2018) ). Effect of resistance training frequency on gains in muscular strength: A systematic review and meta-analysis. Sports Medicine (Auckland, N.Z.), 48: , 1207–1220. |
9 | Hussain, S. , Jamwal, P. K. , Ghayesh, M. H. ((2016) ). Single joint robotic orthoses for gait rehabilitation: An educational technical review. Journal of Rehabilitation Medicine, 48: , 333–338. |
10 | Hussain, S. , Jamwal, P. K. , Vliet, P. V. , Brown, N. A. T. ((2021) ). Robot assisted ankle neuro-rehabilitation: State of the art and future challenges. Expert Review of Neurotherapeutics, 21: , 111–121. |
11 | Jamwal, P. K. , Hussain, S. , Xie, S. Q. ((2015) ). Three-stage design analysis and multicriteria optimization of a parallel ankle rehabilitation robot using genetic algorithm. IEEE Transactions on Automation Science and Engineering, 12: , 1433–1446. |
12 | Kayabinar, B. , Alemdaroğlu-Gürbüz, İ. , Yilmaz, Ö. ((2021) ). The effects of virtual reality augmented robot-assisted gait training on dual-task performance and functional measures in chronic stroke: A randomized controlled single-blind trial. Eur J Phys Rehabil Med, 57: , 227–237. |
13 | Langhorne, P. , Coupar, F. , Pollock, A. ((2009) ). Motor recovery after stroke: A systematic review. The Lancet Neurology, 8: , 741–754. |
14 | Lasevicius, T. , Ugrinowitsch, C. , Schoenfeld, B. J. , Roschel, H. , Tavares, L. D. , De Souza, E. O. , Laurentino, G. , Tricoli, V. ((2018) ). Effects of different intensities of resistance training with equated volume load on muscle strength and hypertrophy. Eur J Sport Sci, 18: , 772–780. |
15 | Lecharte, T. , Gross, R. , Nordez, A. , Le Sant, G. ((2020) ). Effect of chronic stretching interventions on the mechanical properties of muscles in patients with stroke: A systematic review. Annals of Physical and Rehabilitation Medicine, 63: , 222–229. |
16 | Leech, K. A. , Hornby, T. G. ((2017) ). High-intensity locomotor exercise increases brain-derived neurotrophic factor in individuals with incomplete spinal cord injury. Journal of Neurotrauma, 34: , 1240–1248. |
17 | Liu, Q. , Wang, C. , Long, J. J. , Sun, T. , Duan, L. , Zhang, X. , Zhang, B. , Shen, Y. , Shang, W. , Lin, Z. , Wang, Y. , Xia, J. , Wei, J. , Li, W. , Wu, Z. ((2018) ). Development of a new robotic ankle rehabilitation platform for hemiplegic patients after stroke. Journal of Healthcare Engineering, 2018: , 3867243. |
18 | Mao, Y. R. , Zhao, J. L. , Bian, M. J. , Lo, W. L. A. , Leng, Y. , Bian, R. H. , Huang, D. F. ((2022) ). Spatiotemporal, kinematic and kinetic assessment of the effects of a foot drop stimulator for home-based rehabilitation of patients with chronic stroke: A randomized clinical trial. Journal of Neuroengineering and Rehabilitation, 19: , 56. |
19 | Nikamp, C. D. M. , van der Palen, J. , Hermens, H. J. , Rietman, J. S. , Buurke, J. H. ((2018) ). The influence of early or delayed provision of ankle-foot orthoses on pelvis, hip and knee kinematics in patients with sub-acute stroke: A randomized controlled trial. Gait & Posture, 63: , 260–267. |
20 | Park, D. , Lee, J. H. , Kang, T. W. , Cynn, H. S. ((2019) ). Four-week training involving ankle mobilization with movement versus static muscle stretching in patients with chronic stroke: A randomized controlled trial. Topics in Stroke Rehabilitation, 26: , 81–86. |
21 | Payedimarri, A. B. , Ratti, M. , Rescinito, R. , Vanhaecht, K. , Panella, M. (2022). Effectiveness of platform-based robot-assisted rehabilitation for musculoskeletal or neurologic injuries: A systematic review. Bioengineering (Basel, Switzerland), 9. |
22 | Peishun, C. , Haiwang, Z. , Taotao, L. , Hongli, G. , Yu, M. , Wanrong, Z. ((2021) ). Changes in gait characteristics of stroke patients with foot drop after the combination treatment of foot drop stimulator and moving treadmill training. Neural Plasticity, 2021: , 9480957. |
23 | Renfrew, L. M. , Paul, L. , McFadyen, A. , Rafferty, D. , Moseley, O. , Lord, A. C. , Bowers, R. , Mattison, P. ((2019) ). The clinical- and cost-effectiveness of functional electrical stimulation and ankle-foot orthoses for foot drop in Multiple Sclerosis: A multicentre randomized trial. Clin Rehabil, 33: , 1150–1162. |
24 | Schoenfeld, B. J. , Contreras, B. , Willardson, J. M. , Fontana, F. , Tiryaki-Sonmez, G. ((2014) ). Muscle activation during low- versus high-load resistance training in well-trained men. European Journal of Applied Physiology, 114: , 2491–2497. |
25 | Sullivan, K. J. , Tilson, J. K. , Cen, S. Y. , Rose, D. K. , Hershberg, J. , Correa, A. , Gallichio, J. , McLeod, M. , Moore, C. , Wu, S. S. , Duncan, P. W. ((2011) ). Fugl-Meyer assessment of sensorimotor function after stroke: Standardized training procedure for clinical practice and clinical trials. Stroke, 42: , 427–432. |
26 | Sun, T. , Li, H. , Liu, Q. , Duan, L. , Li, M. , Wang, C. , Liu, Q. , Li, W. , Shang, W. , Wu, Z. , Wang, Y. ((2017) ). Inertial sensor-based motion analysis of lower limbs for rehabilitation treatments. Journal of Healthcare Engineering, 2017: , 1949170. |
27 | Thibaut, A. , Wannez, S. , Deltombe, T. , Martens, G. , Laureys, S. , Chatelle, C. ((2018) ). Physical therapy in patients with disorders of consciousness: Impact on spasticity and muscle contracture. NeuroRehabilitation, 42: , 199–205. |
28 | Yoo, D. , Son, Y. , Kim, D. H. , Seo, K. H. , Lee, B. C. ((2018) ). Technology-assisted ankle rehabilitation improves balance and gait performance in stroke survivors: A randomized controlled study with 1-month follow-up. IEEE Trans Neural Syst Rehabil Eng, 26: , 2315–2323. |
29 | Zhai, X. , Wu, Q. , Li, X. , Xu, Q. , Zhang, Y. , Fan, S. , Zhang, L.Q. , Pan, Y. ((2021) ). Effects of robot-aided rehabilitation on the ankle joint properties and balance function in stroke survivors: A randomized controlled trial. Front Neurol, 12: , 719305. |
30 | Zhang, M. , Davies, T. C. , Xie, S. ((2013) ). Effectiveness of robot-assisted therapy on ankle rehabilitation–a systematic review. J Neuroeng Rehabil, 10: , 30. |
31 | Zhao, H. , Wu, Y. N. , Hwang, M. , Ren, Y. , Gao, F. , Gaebler-Spira, D. , Zhang, L. Q. ((2011) ). Changes of calf muscle-tendon biomechanical properties induced by passive-stretching and active-movement training in children with cerebral palsy. Journal of Applied Physiology (Bethesda, Md. : 1985), 111: , 435–442. |