Extrinsic feedback facilitates mental chronometry abilities in stroke patients
Abstract
BACKGROUND:
Motor imagery (MI) can serve as a treatment for stroke rehabilitation. MI abilities can be assessed by testing mental chronometry (MC) as the degree of conformity between imagined and real performance of a task. A good MC performance is supposed to indicate good MI capacities.
OBJECTIVE:
To explore if MC abilities can be modified by extrinsic feedback in stroke patients.
METHODS:
60 subacute stroke patients were randomized into three groups. MC was evaluated by executing a modified version of the Box and Block Test (BBT) mentally and in real before and after a training session. For Groups 1 and 2 the training consisted of repeated performance of the BBT in a mental and then a real version. The time needed to complete each task was measured. Only participants of Group 1 received feedback about how well mental and real performance matched. Group 3 executed the same number of BBTs but without MI.
RESULTS:
MC ability only improved in Group 1. The improvement lasted for at least 24 hours. In all groups, BBT real performance was improved post-training.
CONCLUSION:
External feedback was able to enhance MC capability which might be an approach for improving MI abilities.
1Introduction
Worldwide, stroke is the second leading cause of death and the most common cause of long-term disability (Stron et al., 2007). Up to 76% of patients are affected by paresis of the upper limb (Rathore et al., 2002). For many patients this leads to a reduction in quality of life (Liu et al., 2014) and thus becomes an important factor in rehabilitation. A large number of therapeutic methods have been developed in recent years to improve upper limb function after stroke (Maier et al., 2019; Veerbeek et al., 2014). In order to achieve optimal therapeutic effects, a high training intensity and a high number of repetitions is recommended (Krakauer et al., 2012).
Because of the severity of the motor impairment, numerous stroke patients have little or no opportunity of achieving high training intensity or a high degree of repetition. Mental Practice (MP) strategies might be particularly useful for these patients, since a high number of repetitions can be achieved without physical exhaustion and since mental training can be carried out anywhere and at any time. A recent Cochrane review on motor imagery training after stroke reported a beneficial effect of MP on upper extremity activity and impairment (Barclay et al., 2020). A more recent review concluded that MP is particularly effective when applied during the first 3 months after stroke and in patients with severe motor deficit (Stockley et al., 2021). However, issues as the best dosage and the most effective kind of MP are still unknown. MP usually involves motor imagery (MI) techniques: The patient imagines a movement without actually performing it. Major questions regarding MI are if all stroke patients are similarly capable of performing MI and if MI abilities can be trained and thus improved. To answer the latter question, it is necessary to measure MI abilities. Typically, MI can be subdivided in three categories: First, the vividness of imagery (measured by questionnaires) (e.g., Sakai, K., & Hosoi, Y., 2022; Suica et al., 2022) second, the ability to imagine movements in three-dimensional space (e.g., Johnson et al., 2002; Malouin et al., 2008) and third, the ability to imagine the temporal aspects of a movement (mental chronometry, MC). MC indicates the degree of congruence between the duration of the motor and mental execution of a movement. In healthy subjects, a high correlation between motor and mental execution time has been found (Bakker et al. 2007; Decety et al.). MC was found to predict the magnitude of neuroplastic adaptation following MI training (Yoxon et al., 2022). In recent years, MC has been applied in numerous stroke studies for assessment of motor imagery abilities (e.g., Braun et al., 2017; Liepert et al., 2016; Liepert et al., 2020; Malouin et al., 2012; Malouin et al., 2008; Oostra et al., 2015; Santoro et al., 2019).
It was shown to be a reliable tool for screening stroke patients' motor imagery capabilities (Malouin et al., 2008). Our own studies indicated that MC abilities were basically preserved in patients with a pure motor deficit and poorest in those with a severe somatosensory impairment (Liepert et al., 2016; Liepert et al., 2012).
In this study, we used MC not only to assess the patients' MI abilities but specifically to explore the question if extrinsic feedback can further improve MC abilities. Feedback can be categorized into two types: a knowledge of result (KR) and a knowledge of performance (KP). KR is feedback information about the outcome or goal of the movement and is a cognitive process (Salmoni et al., 1984). KP is feedback information about the movement pattern or kinematics used to achieve the goal (Chen et al., 2016; Schmidt & Lee, 2011).
Typically, KP feedback is provided during and KR feedback is given after a motor execution. In our study, we used KR feedback in combination with a MI task. To our knowledge, this is the first study that applies extrinsic feedback for improving MC.
Based on numerous studies that have demonstrated that KR and KP can improve motor performance in healthy subjects (Doma et al., 2022; Han et al., 2022; Sparrow & Summers, 1992; Zalech & Bujak, 2017) and stroke patients (Chen et al., 2016; Cirstea et al., 2006; Cirstea & Levin, 2007; Kim & Oh, 2019; Molier et al., 2010; Subramanian et al., 2010), we hypothesized that MC could be modified in a similar way.
2Methods
2.1Trial design
A single-blinded, randomized study in subacute post-stroke patients was performed. The primary outcome parameter was a change in MC, measured with a modified version of the Box and Block Test (BBT) (Mathiowetz et al., 1985). As secondary outcome parameter, motor execution of the BBT was used. The investigator (JS) conducting the assessment with the participants was blinded for the type of training. The investigators (JL, AS) performing the training were blinded for the assessment results.
The study protocol and informed consent process were approved by the local ethics committee of the University of Konstanz, Germany (3/2020). The study was conducted in accordance with the Declaration of Helsinki. All participants were informed regarding the experimental nature of the study. Written informed consent was obtained from all subjects. The trial was prospectively registered at Deutsches Register für Klinische Studien (DRKS) under registration number DRKS00020998.
Patient recruitments were started in June 2020 and, after reaching the estimated number of participants, terminated in April 2021.
2.2Subjects
A total of 60 subacute post-stroke patients with upper-limb hemiparesis were included in the study. Patients were recruited from the department of Neurorehabilitation (Kliniken Schmieder, Allensbach, Germany). Patients were randomly assigned either to intervention group 1 (IG 1), intervention group 2 (IG 2) or the control group (CG). Randomization was done by a third party otherwise not involved in the study.
The inclusion criteria for this study were the ability to understand the instructions, the willingness to participate, an ischemic or hemorrhagic stroke <6 months ago as proven by cranial computed tomography or magnetic resonance imaging of the brain and the ability to grasp and release blocks that are typically used in a BBT.
Exclusion criteria were defined as the inability to understand the instructions, aphasia, dementia, hemianopia, spatial neglect, anosognosia, other neurological diseases or severe other illnesses that could interfere with the ability to participate actively.
2.3Measures
For screening all patients underwent the same baseline assessments, including a neurological examination.
To determine possible cognitive limitations and detect neglect symptoms, the Montreal Cognitive Assessment (MoCa) (Nasreddine et al., 2005) and the line bisection test (Fujii et al., 1995) were carried out. Patients that fulfilled inclusion as well as exclusion criteria were then included in the study.
In order to determine MC and motor execution (ME) a modified version of the BBT was first performed mentally and then executed as a motor task. A stopwatch measured the time required for each type of performance. MI and ME were first performed for the affected hand and then for the non-affected hand. The MC was calculated using a ratio of (motor execution time - motor imagery time) / motor execution time. The reason for applying this ratio was to account for the influence of different ME times. For example, a difference of three seconds between MC and ME indicates a worse MC ability if ME takes 15 seconds than when it takes 30 seconds. In dependence on whether ME or MI time was shorter, the ratio could either be a positive or a negative value. Since we were exclusively interested in the absolute difference, all ratios were expressed as positive values. The closer to zero a ratio is, the higher is the congruency between MI and ME.
Immediately after the 30 minutes training and on the following day, the modified BBT was repeated as post- and follow-up test, respectively.
2.4Training
Intervention group 1 (IG 1, MC training with extrinsic feedback)
For the training session the BBT was used in the same way as described above. A total of 15 blocks was placed in the box, arranged in five rows of three blocks. The training took place with the affected side only. First, the patient should imagine grasping each block one by one and transporting it to the other side of the box as quickly as possible. The exercise started on a verbal start signal from the examiner and ended on a verbal stop signal from the patient. The time required was measured with a stopwatch. After the mental execution the motor execution followed, and the time needed was recorded as well. After the two rounds, the patient received feedback from the examiner to what extent the time duration of the mental and motor performance matched and was asked to adjust the mental performance accordingly in the next run.
Intervention group 2 (IG 2, MC training without feedback)
The training was exactly the same as in IG 1, but the patients received no feedback regarding the time difference between mental and motor execution.
Control group (CG, ME training only)
In this group, the patients only performed the motor execution of the BBT for half an hour. There was no mental execution.
The aim was to keep the number of motor executions per training session identical across the three groups. On average, IG 1 and IG 2 performed the motor execution of the BBT ten times and the CG eleven times.
As additional information, we were interested whether MC abilities were related to other individual or clinical aspects. Therefore, we correlated the initial MC performance of all participants with the factors age, gender, time since stroke, type of stroke (ischemic or hemorrhagic) and affected hemisphere (left or right). In addition, we compared MC abilities of the affected and the unaffected hand.
2.5Statistical analysis
The statistical evaluation was carried out with SPSS Statistics 29 (IBM Corp., Armonk, NY, USA).
Since the data were not normally distributed (determined by Shapiro Wilk Test), the statistical analyses were carried out with non-parametric tests. To test the differences in MC and ME between the three groups at the different measurement time points, the non-parametric Kruskal-Wallis test was used. To test for changes within each group over time (baseline –post –follow-up), the non-parametric Friedman test was performed. For comparison of affected and unaffected side at baseline, the Wilcoxon test was used. Subgroup analysis was performed with the Mann-Whitney U test. Correlations between MC ability and age and time since stroke were calculated using Spearman’s rank correlation analysis, and the correlation between MC ability and gender was determined using Bravais-Pearson correlation. Statistical significance was set at p < 0.05.
3Results
Sixty subacute stroke patients were included (Table 1).
Table 1
Demographic and clinical data (Abbreviations: m, male; f, female; r, right; l, left. Values are presented as mean±1 standard deviation)
Group | Age (years) | Gender (m/f) | Time since incident (weeks) | Diagnosis (Infarction/Bleeding) | Affected body side (r/l) |
IG 1 | 63±11 | 10/10 | 9±5 | 13/7 | 9/11 |
IG 2 | 66±11 | 17/3 | 9±5 | 13/7 | 9/11 |
CG | 68±11 | 9/11 | 10±5 | 17/3 | 10/10 |
3.1Mental chronometry
Prior to the training session, no significant difference between the three groups was found (p = 0.84). Immediately after the training, the MC ratio had dropped significantly in IG1 (p = 0.03) and remained unchanged in IG2 and CG (Fig. 1). Comparisons of the post-training MC ratios across the three groups indicated significant differences between IG1 and IG2 (p = 0.02) and IG1 and CG (p < 0.01) but no difference between IG2 and CG (p = 0.66). The follow-up measurement of IG1 showed an unchanged MC ratio compared to the post-training result and still a significant difference compared to baseline (p = 0.02). For IG2 and CG MC ratios at follow-up remained stable compared to the post-training results. Comparisons across the three groups at follow-up showed a significant difference between IG1 and CG (p < 0.01) and a non-significant trend between IG1 and IG2 (p = 0.07) and no difference between IG2 and CG (p = 0.81).
Fig. 1
MC ratios of the 3 groups at baseline (base), after the training session (post) and on the following day (follow-up) (Abbreviations: IG 1, intervention group 1; IG 2, intervention group 2; CG, control group).
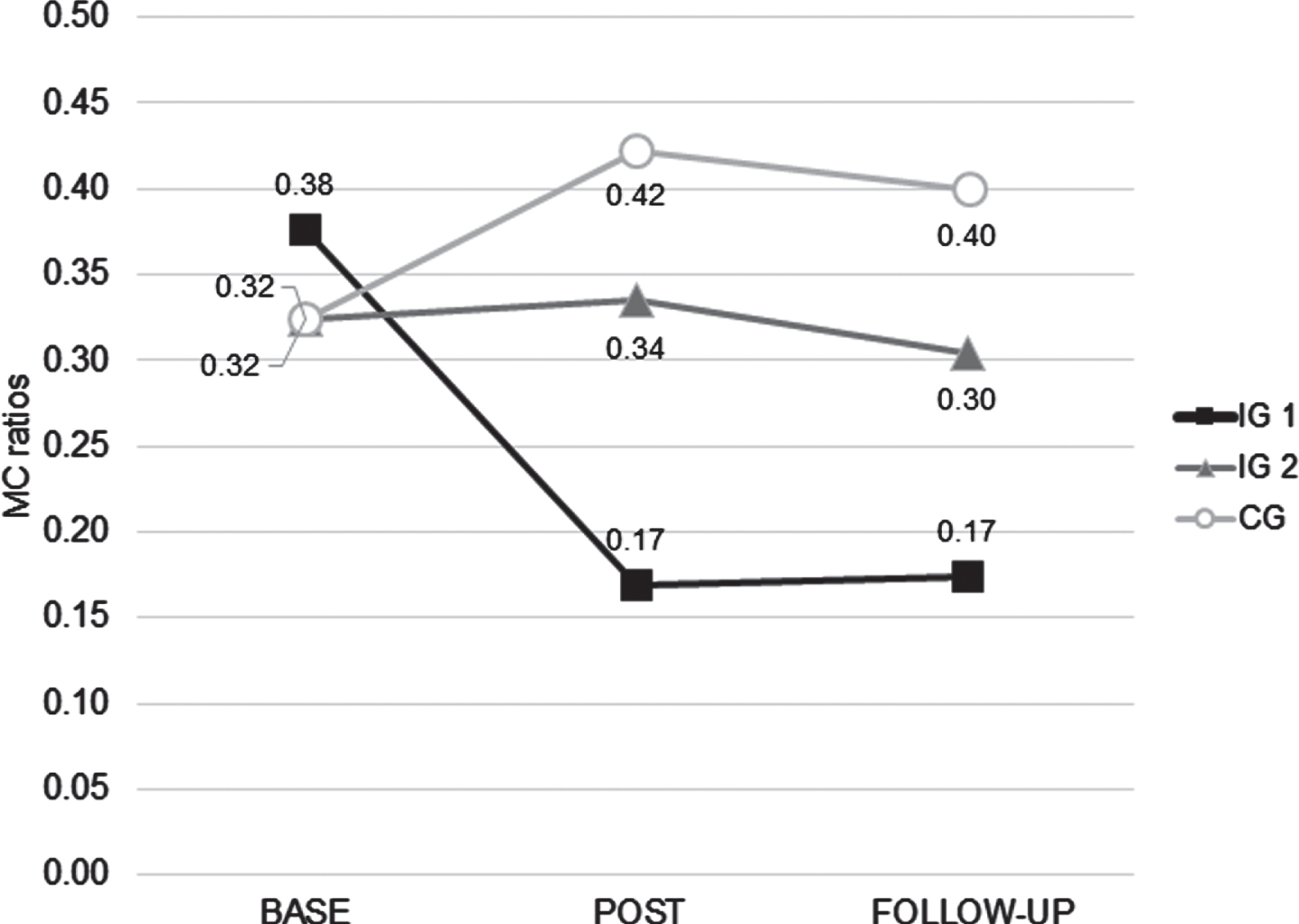
3.2Motor execution
Prior to the training session, execution of the BBT with the affected hand was somewhat slower in the CG (Table 2). However, there was no significant difference (p = 0.23) across the three groups. Immediately after the training BBT execution was significantly faster in all groups in comparison to the baseline performance (IG 1: p < 0.01, IG 2: p < 0.01, CG: p < 0.01). There was no significant difference across the three groups post-training (p = 0.14).
Table 2
BBT execution results of the affected hand (measured in seconds and expressed as mean±standard deviation)
Baseline | Post-training | Follow-up | |
IG 1 | 29.8±11.8 | 24.5±10.9 | 24.6±10.0 |
IG 2 | 33.0±11.9 | 31.0±18.9 | 29.2±10.7 |
CG | 41.4±23.9 | 36.3±23.4 | 34.0±18.7 |
At follow-up BBT performance was comparable to the post-training results. There was neither a significant difference within each group when comparing post-training and follow-up nor across the groups at the follow-up measurement (p = 0.18).
3.3Affected versus unaffected side at baseline
Irrespective of the group allocation we compared MC ability and ME of the affected side and the unaffected side at baseline for all participants (n = 60). In terms of MC ability, there was a significant difference (p < 0.001) between the two body sides, with MC being worse on the affected side (MC ratio mean: 0.34±0.26) than on the unaffected side (MC ratio mean: 0.31±0.28). Similarly, the comparison of ME showed a significant difference (p < 0.001) between the affected (ME mean: 34.7±15.8 seconds) and the unaffected side (ME mean: 14.6±2.8 seconds).
3.4Subgroup analyses
3.4.1Left versus right hemispheric stroke
Our three patient groups were well-balanced with respect to right and left hemispheric strokes. Since Malouin et al. (2012) had reported a poorer MC capability in right hemispheric stroke patients we analyzed our data by comparing left and right hemispheric strokes.
32 patients with right hemispheric stroke and 28 with left hemispheric stroke were included in the study. MC of the affected hand did not show significant differences between the right- and left-hemispherically affected patients, neither at baseline (p = 0.38) nor post-training (p = 0.87) nor at follow-up test (p = 0.85). Similar results were found for MC of the unaffected hand (baseline: p = 0.32, post-training: p = 0.80, follow-up: p = 0.78).
3.4.2Ischemic stroke versus hemorrhage
43 patients had suffered a cerebral ischemia and 17 a cerebral hemorrhage. MC of the affected hand showed no significant difference between the patients with infarction and those with hemorrhage (baseline test: p = 0.86, post-training: p = 0.83, follow-up test: p = 0.63).
3.4.3Correlation of MC ability with age
There was no significant correlation between the MC ability for the affected side of the body at baseline and the patients’ age (rs = –0.2; p = 0.89, n = 60).
3.4.4Correlation of MC ability with gender
There was no significant correlation between the MC ability for the affected side of the body at baseline and the patients’ gender (rp = 0.17; p = 0.19; n = 60).
3.4.5Correlation of MC ability with time since stroke
There was no significant correlation between the MC ability for the affected side of the body at baseline and the time since stroke (rs = 0.18; p = 0.18; n = 60).
4Discussion
This study demonstrated that provision of external feedback improved the capability of MC in subacute stroke patients even within a single training session. This improvement was still present 24 hours later. To our knowledge, this is the first demonstration of MC abilities being modified by knowledge of result feedback. Our results indicate that the MC improvement can develop within 30 minutes of training and that it outlasts the training for at least one day. Knowledge of result feedback has a similar effect on MC improvement as it had on ME in other studies (Chen et al., 2016; Cirstea et al., 2006; Cirstea & Levin, 2007; Kim & Oh, 2019; Molier et al., 2010; Subramanian et al., 2010).
A basic assumption is that persons with good MC abilities benefit to a greater extend from MI training than persons with poor MC abilities. If this is true, then MI training should include exercises with MC elements and the possibility to provide feedback about the MC performance.
ME was similarly affected across the three groups, indicating that the groups were relatively homogeneous regarding their motor performance. In all groups, BBT execution improved to a similar extend. Presumably, this is due to the same number of execution trials across the groups. It also indicates that a single 30 minutes session of MP is not sufficient to modulate motor performance. However, we did not expect such a performance change since, typically, MI training is provided for about 20–30 sessions in order to improve motor function (e.g. Barclay et al., 2020; Page et al., 2011).
The comparison of MC for the affected and the unaffected hand demonstrated a small but significant difference in favor of the unaffected hand. The result suggests that it was easier for patients to imagine movements with the unaffected hand and that patients had a more reliable internal representation of its function. We hypothesize that a less frequent use of the affected hand in activities of daily living could have contributed to this difference. However, we cannot exclude the possibility that this finding might have been biased by the sequence since patients always performed motor imagery and motor execution first with the affected, then with the unaffected hand. Thus, when performing MC with the unaffected hand, patients had already been more familiar with the task.
Our results also demonstrated that there was no correlation between MC ability and age, gender and time since stroke. Thus, neither age nor gender seem to have a relevant influence on MC. In healthy subjects, a worse performance of MC had been found in a group of elderly subjects (mean age: 67 years) as compared to younger individuals (mean age 42 years) (Greiner et al., 2014). Thus, in general an age-related influence on MC abilities is probable. We hypothesize that, in this study, the age range was too small to detect age-related differences since the majority (44 patients) was between 60 and 85 years old.
Time since stroke did not seem to be a relevant factor. However, one has to keep in mind that all of our patients were within the first 6 months after stroke. Thus, we cannot exclude that time after stroke becomes more relevant if patients in the chronic stage had been included.
Our analyses also indicated that the type of stroke (ischemia versus hemorrhage) had no influence on MC abilities. Nor did we find a difference between right-hemispheric and left-hemispheric lesions. This result is in contrast to Malouin et al. (2012) who reported that patients with right-hemispheric lesions presented more frequently a temporal incongruence with longer movement times during imagination and displayed greater visuospatial working memory deficits. It is currently unclear why our results do not correspond to those of Malouin et al. (2012). We hypothesize that individual patients’ characteristics, e.g. the lesion locations, were different between the patient groups of the two studies.
5Conclusion
Our study demonstrated that, in subacute stroke patients, MC abilities can by optimized by knowledge of results feedback. Future studies should explore if an improved MC leads to better results in MP exercises.
Conflict of interest
The authors have no conflicts of interest to declare.
Acknowledgments
The authors thank C. Salzmann, P. Theobald, L. Zeilhofer and T. Loewner for organizational support during the study.
Ethics statement
The study protocol and informed consent process were approved by the local ethics committee of the University of Konstanz, Germany (approval number: 3/2020). The study was conducted in accordance with the Declaration of Helsinki. All participants were informed regarding the experimental nature of the study. Written informed consent was obtained from all subjects. The trial was prospectively registered at Deutsches Register für Klinische Studien (DRKS) under registration number DRKS00020998.
Funding
The authors report no funding.
References
1 | Bakker, M. , Lange, F. P. , de, Stevens, J. A. , Toni, I. , Bloem, B. R. ((2007) ). Motor imagery of gait: A quantitative approach. Experimental Brain Research 179: (3), 497–504. https://doi.org/10.1007/s00221-006-0807-x |
2 | Barclay, R. E. , Stevenson, T. J. , Poluha, W. , Semenko, B. , Schubert, J. ((2020) ) Mental practice for treating upper extremity deficits in individuals with hemiparesis after stroke. The Cochrane Database of Systematic Reviews, 5: (5), CD005950. https://doi.org/10.1002/14651858.CD005950.pub5 |
3 | Braun, N. , Kranczioch, C. , Liepert, J. , Dettmers, C. , Zich, C. , Büsching, I. , Debener, S. ((2017) ). Motor Imagery Impairment in Postacute Stroke Patients. Neural Plasticity, 2017: , 4653256. https://doi.org/10.1155/2017/4653256 |
4 | Chen, J. L. , Fujii, S. , Schlaug, G. ((2016) ). The use of augmented auditory feedback to improve arm reaching in stroke: A case series. Disability and Rehabilitation 38: (11), 1115–1124. https://doi.org/10.3109/09638288.2015.1076530 |
5 | Cirstea, C. M. , Ptito, A. , Levin, M. F. ((2006) ). Feedback and cognition in arm motor skill reacquisition after stroke. Stroke 37: (5), 1237–1242. https://doi.org/10.1161/01.STR.0000217417.89347.63 |
6 | Cirstea, M. C. , Levin, M. F. ((2007) ). Improvement of arm movement patterns and endpoint control depends on type of feedback during practice in stroke survivors. Neurorehabilitation and Neural Repair 21: (5), 398–411. https://doi.org/10.1177/1545968306298414 |
7 | Decety, J. , Jeannerod, M. , Prablanc, C. ((1989) ). The timing of mentally represented actions. Behavioural Brain Research, 34: , 35–42. https://citeseerx.ist.psu.edu/viewdoc/download?doi=10.1.1.212.3957&rep=rep1&type=pdf |
8 | Doma, K. , Engel, A. , Connor, J. , Gahreman, D. ((2022) ). Effects of Knowledge of Results and Change-Oriented Feedback on Swimming Performance. International Journal of Sports Physiology and Performance 17: (4), 556–561. https://doi.org/10.1123/ijspp.2021-0227 |
9 | Fujii, T. , Fukatsu, R. , Yamadori, A. , Kimura, I. ((1995) ). Effect of age on the line bisection test. Journal of Clinical and Experimental Neuropsychology 17: (6), 941–944. https://doi.org/10.1080/01688639508402443 |
10 | Greiner, J. , Schoenfeld, M. A. , Liepert, J. ((2014) ). Assessment of mental chronometry (MC) in healthy subjects. Archives of Gerontology and Geriatrics 58: (2), 226–230. https://doi.org/10.1016/j.archger.2013.09.003 |
11 | Han, Y. , Syed Ali, S. K. B. , Ji, L. ((2022) ). Feedback for Promoting Motor Skill Learning in Physical Education: A Trial Sequential Meta-Analysis. International Journal of Environmental Research and Public Health 19: (22). https://doi.org/10.3390/ijerph192215361 |
12 | Johnson, S. H. , Sprehn, G. , Saykin, A. J. ((2002) ). Intact motor imagery in chronic upper limb hemiplegics: Evidence for activity-independent action representations. Journal of Cognitive Neuroscience 14: (6), 841–852. https://doi.org/10.1162/089892902760191072 |
13 | Kim, D. K. , Oh, D. W. ((2019) ). Repeated Use of 6-min Walk Test with Immediate Knowledge of Results for Walking Capacity in Chronic Stroke: Clinical Trial of Fast versus Slow Walkers. Journal of Stroke and Cerebrovascular Diseases: The Official Journal of National Stroke Association 28: (11). https://doi.org/10.1016/j.jstrokecerebrovasdis.2019.104337 |
14 | Krakauer, J. W. , Carmichael, S. T. , Corbett, D. , Wittenberg, G. F. ((2012) ). Getting neurorehabilitation right: What can be learned from animal models? Neurorehabilitation and Neural Repair, 26: (8), 923–931. https://doi.org/10.1177/1545968312440745 |
15 | Liepert, J. , Büsching, I. , Sehle, A. , Schoenfeld, M. A. ((2016) ). Mental chronometry and mental rotation abilities in stroke patients with different degrees of sensory deficit. Restor Neurol Neurosci 34: (6), 907–914. https://doi.org/10.3233/RNN-160640 |
16 | Liepert, J. , Greiner, J. , Nedelko, V. , Dettmers, C. ((2012) ). Reduced upper limb sensation impairs mental chronometry for motor imagery after stroke: Clinical and electrophysiological findings. Neurorehabilitation and Neural Repair 26: (5), 470–478. https://doi.org/10.1177/1545968311425924 |
17 | Liepert, J. , Stürner, J. , Büsching, I. , Sehle, A. , Schoenfeld, M. A. ((2020) ). Effects of a single mental chronometry training session in subacute stroke patients - a randomized controlled trial. BMC Sports Science, Medicine & Rehabilitation, 12: , 66. https://doi.org/10.1186/s13102-020-00212-w |
18 | Liu, H. , Song, L. , Zhang, T. ((2014) ). Mental practice combined with physical practice to enhance hand recovery in stroke patients. Behavioural Neurology, 2014: , 876416. https://doi.org/10.1155/2014/876416 |
19 | Maier, M. , Ballester, B. R. , Verschure, P. F. M. J. ((2019) ). Principles of Neurorehabilitation After Stroke Based on Motor Learning and Brain Plasticity Mechanisms. Frontiers in Systems Neuroscience, 13: , 74. https://doi.org/10.3389/fnsys.2019.00074 |
20 | Malouin, F. , Richards, C. L. , Durand, A. ((2012) ). Slowing of motor imagery after a right hemispheric stroke. Stroke Research and Treatment, 2012: , 297217. https://doi.org/10.1155/2012/297217 |
21 | Malouin, F. , Richards, C. L. , Durand, A. , Doyon, J. ((2008) ). Reliability of mental chronometry for assessing motor imagery ability after stroke. Archives of Physical Medicine and Rehabilitation 89: (2), 311–319. https://doi.org/10.1016/j.apmr.2007.11.006 |
22 | Mathiowetz, V. , Volland, G. , Kashman, N. , Weber, K. ((1985) ). Adult Norms for the Box and Block Test of Manual Dexterity. The American Journal of Occupational Therapy 39: (6), 386–391. http://ajot.aota.org on 04/07/2020 Terms of use: http://AOTA.org/terms |
23 | Molier, B. I. , Van Asseldonk, E. H. F. , Hermens, H. J. , Jannink,M. J. ((2010) ). Nature, timing, frequency and type of augmented feedback; does it influence motor relearning of the hemiparetic arm after stroke? A systematic review. Disability and Rehabilitation 32: (22). https://doi.org/10.3109/09638281003734359 |
24 | Nasreddine, Z. S. , Philips, N. A. , Bédirian, V. , Charbonneau, S. , Whitehead, V. , Collin, I. , Cummings, J. L. , Chertkow, H. ((2005) ). The Montreal Cognitive Assessment, MoCA: a brief screening tool for mild cognitive impairment. Journal of the American Geriatrics Society 53: (4), 695–699. |
25 | Oostra, K. M. , Oomen, A. , Vanderstraeten, G. , Vingerhoets, G. ((2015) ). Influence of motor imagery training on gait rehabilitation in sub-acute stroke: A randomized controlled trial. Journal of Rehabilitation Medicine 47: (3), 204–209. https://doi.org/10.2340/16501977-1908 |
26 | Page, S. J. , Dunning, K. , Hermann, V. , Leonard, A. , Levine, P. ((2011) ). Longer versus shorter mental practice sessions for affected upper extremity movement after stroke: A randomized controlled trial. Clinical Rehabilitation 25: (7), 627–637. https://doi.org/10.1177/0269215510395793 |
27 | Rathore, S. S. , Hinn, A. R. , Cooper, L. S. , Tyroler, H. A. , Rosamond, W. D. ((2002) ). Characterization of incident stroke signs and symptoms: Findings from the atherosclerosis risk in communities study. Stroke 33: (11), 2718–2721. https://doi.org/10.1161/01.str.0000035286.87503.31 |
28 | Sakai, K. , Hosoi, Y. ((2022) ). Relationship between the vividness of motor imagery and physical function in patients with subacute hemiplegic stroke: A cross-sectional preliminary study. Brain Injury 36: (1), 121–126. |
29 | Salmoni, A. W. , Schmidt, R. A. , Walter, C. B. ((1984) ). Knowledge of Results and Motor Learning: A Review and Critical Reappraisal. Psychological Bulletin 95: (3), 355–386. |
30 | Santoro, S. , Lo Buono, V. , Corallo, F. , Cartella, E. , Micchia, K. , Palmeri, R. , Arcadi, F. A. , Bramanti, A. , Marino, S. ((2019) ). Motor imagery in stroke patients: A descriptive review on a multidimensional ability. International Journal of Neuroscience 129: (8), 821–832. https://doi.org/10.1080/00207454.2019.1567509 |
31 | Schmidt, R. A. , Lee, T. D. (2011). Motor Control and Learning: A Behavioral Emphasis (5th version). Human Kinetics. |
32 | Sparrow, W. A. , Summers, J. J. ((1992) ). Performance on Trials without Knowledge of Results (KR) in Reduced Relative Frequency Presentations of KR. Journal of Motor Behavior 24: (2), 197–209. https://doi.org/10.1080/00222895.1992.9941615 |
33 | Stockley, R. C. , Jarvis, K. , Boland, P. , Clegg, A. J. ((2021) ). Systematic Review and Meta-Analysis of the Effectiveness of Mental Practice for the Upper Limb After Stroke: Imagined or Real Benefit? Archives of Physical Medicine and Rehabilitation, 102: (5), 1011–1027. https://doi.org/10.1016/j.apmr.2020.09.391 |
34 | Stron, K. , Mathers, C. , Bonita, R. ((2007) ). Preventing stroke: saving lives around the world. Lancet Neurol, 6: , 182–187. https://doi.org/10.1016/S1474-4422(07)70031-5 |
35 | Subramanian, S. K. , Massie, C. L. , Malcolm, M. P. , Levin, M. F. ((2010) ). Does provision of extrinsic feedback result in improved motor learning in the upper limb poststroke? A systematic reviewof the evidence. Neurorehabilitation and Neural Repair 24: (2), 113–124. https://doi.org/10.1177/1545968309349941 |
36 | Suica, Z. , Behrendt, F. , Gäumann, S. , Gerth, U. , Schmidt-Trucksäss, A. , Ettlin, T. , Schuster-Amft, C. ((2022) ). Imagery ability assessments: A cross-disciplinary systematic review and quality evaluation of psychometric properties. BMC Medicine, 20: (1), 166. https://doi.org/10.1186/s12916-022-02295-3 |
37 | Veerbeek, J. M. , van Wegen, E. , van Peppen, R. , van der Wees, P. J. , Hendriks, E. , Rietberg, M. , Kwakkel, G. ((2014) ). What is the evidence for physical therapy poststroke? A systematic review and meta-analysis. PloS One, 9: (2), e87987. https://doi.org/10.1371/journal.pone.0087987 |
38 | Yoxon, E. , Brillinger, M. , Welsh, T. N. ((2022) ). Behavioural indexes of movement imagery ability are associated with the magnitude of corticospinal adaptation following movement imagery training. Brain Research, 1777: , 147764. https://doi.org/10.1016/j.brainres.2021.147764 |
39 | Zalech, M. , Bujak, Z. ((2017) ). Precision of verbal feedback affects accuracy of motor skill performance. The Journal of Sports Medicine and Physical Fitness 58: (4), 435–441. https://europepmc.org/article/med/28181775 |