Exaggerated pressor response to static squats in Parkinson’s disease (PD) and healthy subjects is likely an individual trait, not influenced by whole body vibration (WBV)
Abstract
BACKGROUND:
Reduced muscle strength is one symptom of Parkinson’s disease (PD). Strength can be increased by strength training, which may cause exaggerated blood pressure (BP) rise. It is believed that exercises performed on vibrating platform can strengthen leg muscles without excessive BP increase.
OBJECTIVE:
To measure the pressor response to static exercises performed during whole body vibration in PD patients.
METHODS:
Twenty-four aged PD patients and twelve healthy young volunteers participated in the study. PD subjects performed six repetitions of deep-, semi-squat, and calves at vibration frequency of 30 Hz. Each 30 s exercise was followed by 30 s rest. The young volunteers performed two sessions of above-mentioned exercises with and without vibration. BP was measured continuously.
RESULTS:
In PD patients, the highest BP values were observed during deep squat; systolic blood pressure rose 10 mmHg in ‘weak responders’, and 50 mmHg in ‘strong responders’. This difference correlated with the rise in pulse pressure suggesting indirectly the role of stoke volume in individual response. In healthy subjects pressor response was also individually differentiated and not influenced by vibration.
CONCLUSION:
Deep and semi squat can evoke a strong cardiovascular response in some PD and healthy subjects. Low-magnitude vibrations likely did not affect pressor response.
1Introduction
The age-related decrease in the level of physical activity and muscle strength is more pronounced in people with Parkinson’s disease (PD) than in healthy subjects. Muscle weakness is considered one of the primary symptoms of PD (Koller & Kase, 1986). It is highly possible that muscle weakness can be one of the reasons that people with PD are three times more likely to sustain a hip fracture when compared to those without the condition (Genever et al., 2005).
The physical training, especially strength training, may be an effective strategy to delay muscle weakness. Such training enhanced leg muscle strength in PD patients (Silva-Batista et al., 2016). Strength training may pose heavy cardiovascular load, especially by increasing arterial pressure (MacDougall et al., 1985).
Application of sinusoidal whole body vibration (WBV) can serve as an alternative for strength training (Niewiadomski et al., 2005). WBV was found to increase muscle strength in young and old persons (Delecluse et al., 2003; Rees et al., 2008; Machado et al., 2010), in the elderly with sarcopenia (Wei et al., 2017), in patients with cerebral palsy (Ahlborg et al., 2006), and with chronic diseases (Pang, 2010).
Importantly, contrary to standard strength exercises, it seems that vibration exercise does not cause excessive pressor response in healthy people (Rittweger et al., 2000; Hazell et al., 2008; Robbins et al., 2014), in intensive care unit patients (Wollersheim et al., 2017), in persons with metabolic syndrome (Sá-Caputo et al., 2018), and in obese (Tamini et al., 2020). This kind of training may be a safe and effective exercise therapy also for elderly patients (Cochrane et al., 2008; Aoyama et al., 2019).
On the other hand, some publications demonstrated that the vibration exercises impose a moderate load on the cardiovascular system. Avelar et al. (2011) measured continuously heart rate (HR) in the elderly at rest and during the training session and observed an enhanced HR increase when squatting exercises were associated with whole body vibration. Similarly, addition of WBV to exercises enhanced mildly HR increase in persons with chronic stroke, however did not enhance the blood pressure response (Liao et al., 2015). Dipla et al. (2016) observed augmented HR during vibration in comparison to the situation when identical body position but without vibration was applied in lean and obese women. Moreover, when vibrations were applied, systolic (SBP) and diastolic blood pressure (DBP) increases were significantly enhanced, more in obese women than in lean ones. Dias & Polito (2015) did not observe the enhanced HR increase when vibrations were added to the exercises in sedentary healthy people; however, increases in SBP and DBP were also augmented. These studies prove that there is no uniform pattern of cardiovascular response to WBV, and there is no solid evidence confirming the cardiovascular safety of WBV.
We have completed a project in which 24 PD patients underwent a year of whole body vibration (WBV) training consisting of low amplitude sinusoidal vibrations combined with exercises, which suppressed the propagation of vibrations towards the upper body parts. After half of the year this training increased the maximal muscle strength of lower limbs by 17% (Niewiadomski et al., 2016). The question arose whether such training can pose heavy load on the cardiovascular system.
In papers mentioned above, the measurements were done before and after the intervention or in the chosen time points during the training session. In this study the advantage of continuous registration of arterial blood pressure was taken, what allowed for analysis of the time course of SBP and DBP changes during WBV session, specifically to detect their rapid and transient changes. Such changes, if existent, may be of importance for safety issue and such approach to gain insight into yet not examined problem of cardiovascular safety during WBV in PD patients was applied.
2Methods
2.1Subjects
Twenty four participants (12 men and 12 women) with PD were recruited to participate in the study.
Inclusion criteria were as follows: idiopathic PD, Hoehn & Yahr stage below 3, disease duration less than 10 years since disease diagnosis, men and women, age 55–75, lack of disorders that interfere with ability to perform static exercises on vibrating platform, willing to participate in exercise session performed on such platform.
The exclusion criteria were: venous thromboembolism, musculoskeletal disorders, implanted endoprosthesis, treated cardiovascular diseases including arrhythmias, neurodegenerative diseases except PD and involvement in intensive physical activity. Subjects’ details are given in Table 1. Additionally, twelve (1 man and 11 women) healthy persons were enrolled into the supplementary group. The details are given in Table 2.
Table 1
Physical characteristics of the PD subjects
Sex | N | Age [years] | Weight [kg] | Height [cm] | Duration of disease [years] | Hoehn & Yahr scale |
Men | 12 | 67 (5) | 85 (13) | 174 (6) | 5.4 (3.7) | 2.0 (0.5) |
Women | 12 | 68 (8) | 69 (11) | 161 (2) | 4.3 (3.1) | 2.1 (0.4) |
Data are shown as mean (SD).
Table 2
Physical characteristics of the subject from supplementary group
Sex | N | Age [years] | Weight [kg] | Height [cm] |
Man | 1 | 25 | 88 | 174 |
Women | 11 | 32 (14) | 65 (11) | 167 (7) |
Data are shown as mean (SD).
All participants provided written informed consent. The study was approved by the Ethical Committee of Warsaw Medical University in Warsaw.
2.2Protocol
PD patients performed a session consisting of six sequences; each sequence consisted of three types of static squats: calves (standing with heels slightly raised, knees slightly bent), deep squat (knee angle of ∼90°), and semi squat (knee angle of ∼120°). The subjects were familiar with the exercises. Each static squat was maintained for 30 s followed by 30 s of standing rest. Subjects were asked to breathe normally when they were remaining in static squat.
Simultaneously, during the 30 s periods of exercises patients were exposed to whole body vibration using a vibration platform constructed and located at Mossakowski Medical Research Institute. The vibration frequency of 30 Hz was applied. Vibrations were vertical. Root mean square value of acceleration in vertical axis was in the range 3–4 m/s2, what corresponds to the amplitude of 0.09 –0.11 mm. The magnitude of vibration used was safe as such magnitude could be used several hours per day without adverse effects (Muir et al., 2013).
The supplementary group performed two sessions of above described exercises, one with the vibration, as it was in case of PD patients, and the other one without to investigate the possible influence of vibration on cardiovascular response to the exercises performed. These two sessions were interspaced by one week.
2.3Measurement and data processing
Over one minute before the session, during the whole session, and over one minute after the session cessation the blood pressure was registered continuously and non-invasively with a Portapres (FMS, Amsterdam, The Netherlands). The finger cuff was placed on the finger on the left hand, while the right hand served as a support. Simultaneously, ECG and the signal from the accelerometer MMA7361L (Freescale Semiconductor, Austin, USA) placed on the vibration platform were recorded.
Analogue signals from these devices were digitized at a sampling rate of 200 Hz with WinAcq data acquisition system (Absolute Aliens Oy, Turku, Finland). The signals were analyzed using Sigman (free software, aimed to analyze polyphysiographic signals, developed by Krzysztof Cybulski and available at https://github.com/k-cybulski/sigman-project), the program conceived and developed in our laboratory. For every heart cycle SBP, DBP, pulse pressure (PP), and RR were determined. Heart rate was calculated from RR interval. The values of these parameters were averaged over each 30-second period of exercise or over each 30-second period of pause duration. Statistical evaluation was performed on these averages.
2.4Statistical analysis
Statistics were calculated using Statistica Software, (Version 10), Statsoft Polska. A two-way analysis of variance (ANOVA) for repeated measures was performed to evaluate the effect of exercise (3 levels: calves, deep squat, semi squat) and the effect of repetition (6 levels i.e. 6 repetitions of each exercise) on SBP, DBP, PP, and HR. A Newman-Keuls post hoc analysis was performed when significant factor effects or their interactions were detected. A value of p < 0.05 was accepted as the threshold of statistical significance.
In order to find out whether the pressor response to static exercises is individually different in PD patents, the linear regression was applied to find out whether individual magnitude of ΔSBP, calculated as average of 6 repetitions of deep squat was correlated with individual magnitude of ΔSBP, calculated as average of 6 repetitions of semi squat. ΔSBP for given repetition was calculated as a difference between baseline SBP; i.e. average of SBP measured during 30 seconds immediately before commencing first exercise, and SBP during given repetition, i.e. average of SBP measured during 30 seconds of given repetition. The linear regression between individual ΔSBP during deep squat performed with and without vibration in subjects from the supplementary group was also applied to find out whether the pressor responses to this exercise were individually different.
One-way ANOVA was applied to examine whether there exists significant differences in age, weight, height, duration of disease, Hoehn & Yahr scale, and basal SBP between four groups of PD subjects. The criteria of allotting particular subjects to the given group are described in Results section. Similarly, one-way ANOVA was performed to investigate significance of differences between four groups in mean ΔSBP, ΔDBP, ΔPP, and ΔHR changes obtained during deep squat. ΔDBP, ΔPP, and ΔHR were calculated in the analogous way as ΔSBP.
3Results
The blood pressure increased during exercises on the vibrating platform. The highest rise of blood pressure (Fig. 1a,b) was observed during deep squat and the lowest during calves. ANOVA showed significant effect of exercise (SBP: F(2,46) = 24.559, p < 0.001; DBP: F(2,46) = 25.874, p < 0.001); post hoc analysis revealed the significant differences between all three types of exercises. SBP increases, as well as, DBP increases were most pronounced during third repetition. It was confirmed by two way ANOVA which showed significant effect of repetition (SBP: F(5,115) = 2.396, p < 0.05; DBP: F(5,115) = 3.012, p < 0.05).
Fig. 1
The mean SBP (a), DBP (b), PP (c), and HR (d) during calves, deep squat, and semi squat during the training session. Data are shown as mean (SD).
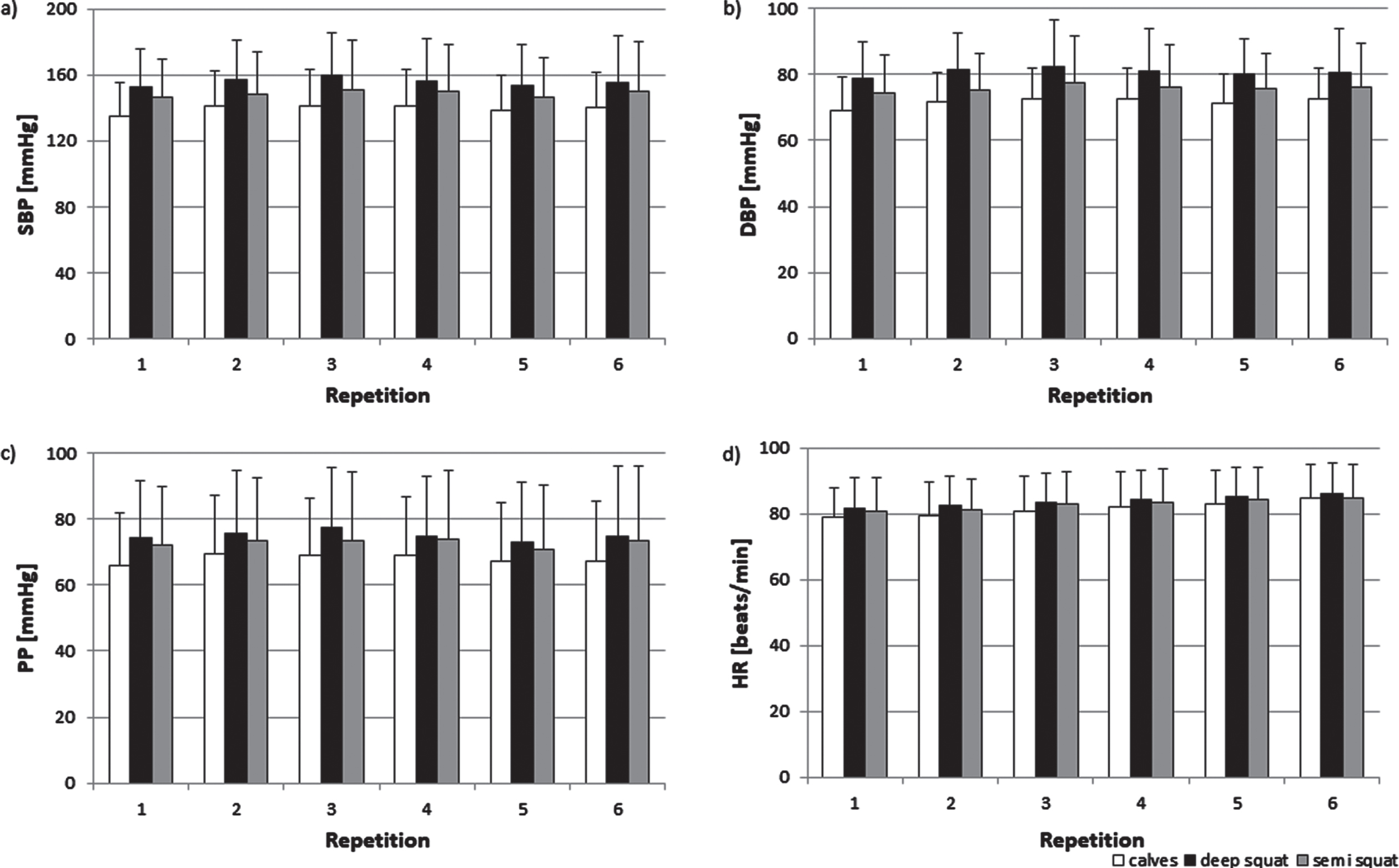
Magnitude of pulse pressure (PP) increase also depended on the exercise but did not change with subsequent repetition (Fig. 1c). These was confirmed by two-way ANOVA which showed only significant effect of exercise (F(2,46) = 18.2701, p < 0.001). The values during deep squats and semi squats were a significant higher than during calves (p < 0.001). There was a tendency towards (p = 0.08) higher PP values during deep squats than semi squats.
Furthermore, HR rose the most during deep squat and less during calves. In contrast to blood pressure, HR grew over the following repetitions (Fig. 1d). These was confirmed by two-way ANOVA which revealed significant effect of exercise (F(2,46) = 12.037, p < 0.001) and effect of repetitions (F(5,115) = 27.653, p < 0.001).
The magnitude of blood pressure response to the exercises was individually differentiated. The higher the increase of SBP (ΔSBP) values obtained during deep squat the higher SBP increase observed during semi squat in a given subject (r = 0.93; p < 0.001, Fig. 2). The ΔSBP during deep and semi squats did not correlate with the basal SBP registered before the training session, what suggests that the individual pressor response does not depend on resting systolic blood pressure.
Fig. 2
The correlation between ΔSBP during semi and deep squats in 24 PD subjects.
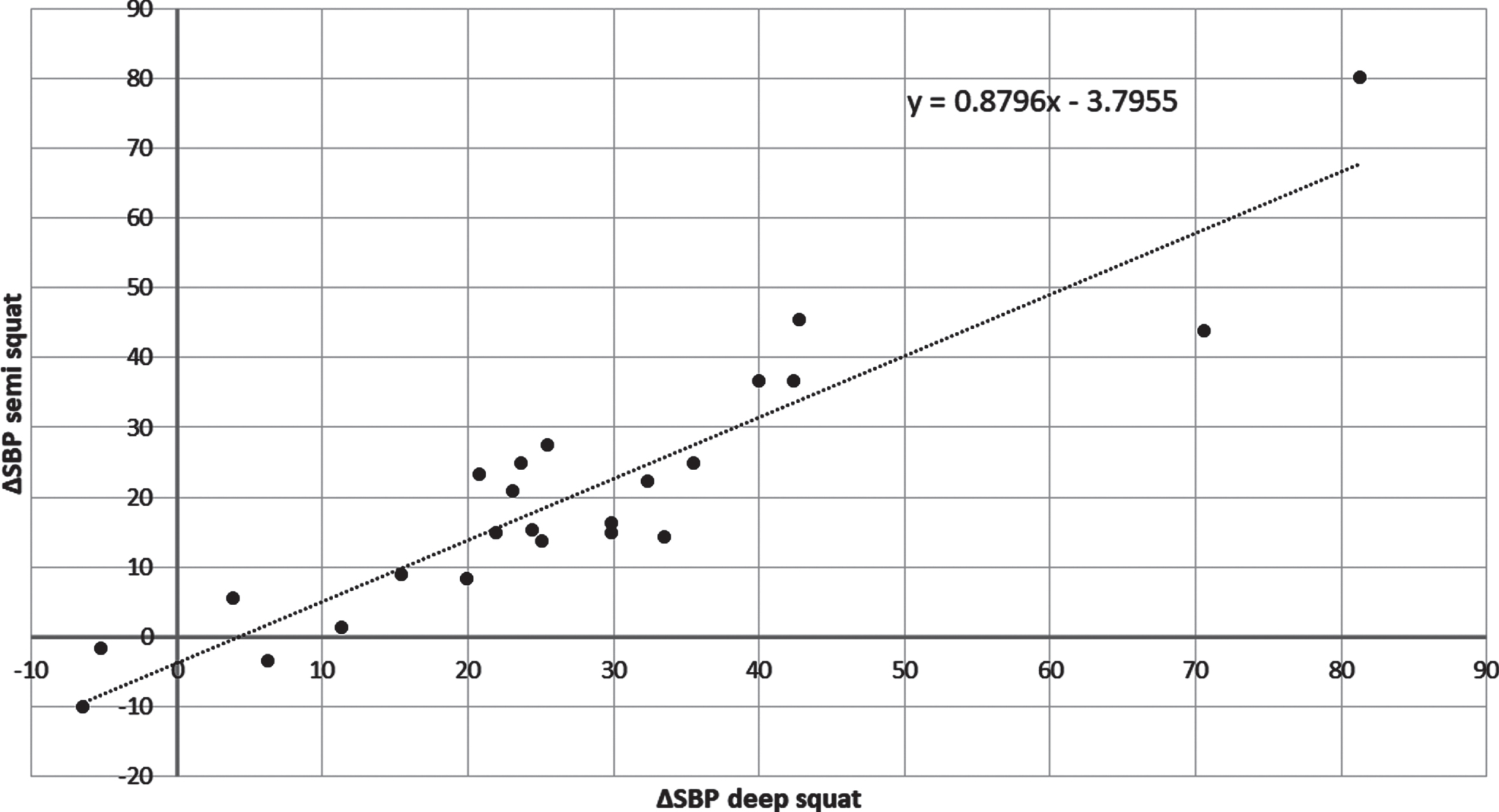
Figure 2 suggests that there are individual differences in ΔSBP rise caused by exercises. The question was whether ’strong responders‘ are characterized by a specific pressor response to exercises different from that of ‘weak responders’. To answer this the subjects were ordered according to the magnitude of SBP increase observed during the first deep squat and divided into 4 equal groups (6 persons per group), from ‘weak responders’, upwards through ‘intermediate’, to ‘strong responders’.
The characteristic of these groups is given in Table 3. Analysis showed no significant differences among groups in age, weight, height, duration of disease, H&Y scale, and basal SBP. Numbers of females and men in the groups were different, however, fairly similar in Group 1 and 4.
Table 3
Characteristics of four groups of PD patients
Group | Sex | Age [years] | Weight [kg] | Height [cm] | Duration of disease [years] | Hoehn &Yahr scale | Basal SBP [mmHg] |
1 | 4M, 2F | 67 (8) | 86 (20) | 174 (11) | 5.2 (4.7) | 1.8 (0.4) | 136 (16) |
2 | 2M, 4F | 68 (4) | 74 (13) | 165 (11) | 5.5 (3.9) | 2.0 (0.4) | 134 (21) |
3 | 1M, 5F | 67 (10) | 70 (10) | 161 (5) | 3.8 (2.1) | 2.1 (0.5) | 116 (15) |
4 | 4M, 1F | 69 (3) | 77 (9) | 170 (2) | 5.0 (2.8) | 2.3 (0.4) | 129 (26) |
The division into groups was based on the SBP reaction observed during the first deep squat of the training session. The basal SBP is the mean of SBP values registered during 30 s before commencing exercise. M - male, F –female. Data are shown as mean (SD).
The response to deep squat in four groups have been analysed. In case of ΔSBP, there was significant effect of group (F(3,20) = 8,18687, p < 0.001). Post hoc analysis revealed significant higher ΔSBP values in group 3 (p < 0.005) and 4 (p < 0.001) when compared to group 1. There was also a tendency to higher values in group 4 in comparison to group 3 (p = 0.07). The occurrence of this difference is obvious as it is a consequence of the way the population was divided in 4 groups. However, it should be mentioned that the groups where divided according to SBP values obtained during the first squat, and here the results being mean of 6 ΔSBP readouts noted during all deep squat exercises are reported. Although there is a clear increase of ΔDBP along the groups, surprisingly the effect of group was not statistically significant. However, there was a significant effect of the group in the case of ΔPP (F(3,20) = 10,31352, p < 0.001). Similarly to ΔSBP, the values of ΔPP in groups 3 and 4 were significantly greater than in group 1 (p < 0.05, p < 0.001, respectively). A tendency to higher values in group 4 when compared to group 3 was also noted (p = 0.06). The effect of group was not significant in the case of ΔHR (Fig. 3).
Fig. 3
The mean ΔSBP, ΔDBP, ΔPP, ΔHR during deep squat in four subjects’ groups. The values shown are averaged readouts from 6 repetition of 30-sec. lasting exercise. Description of groups is given in Results. *p < 0.05, ***p < 0.001.
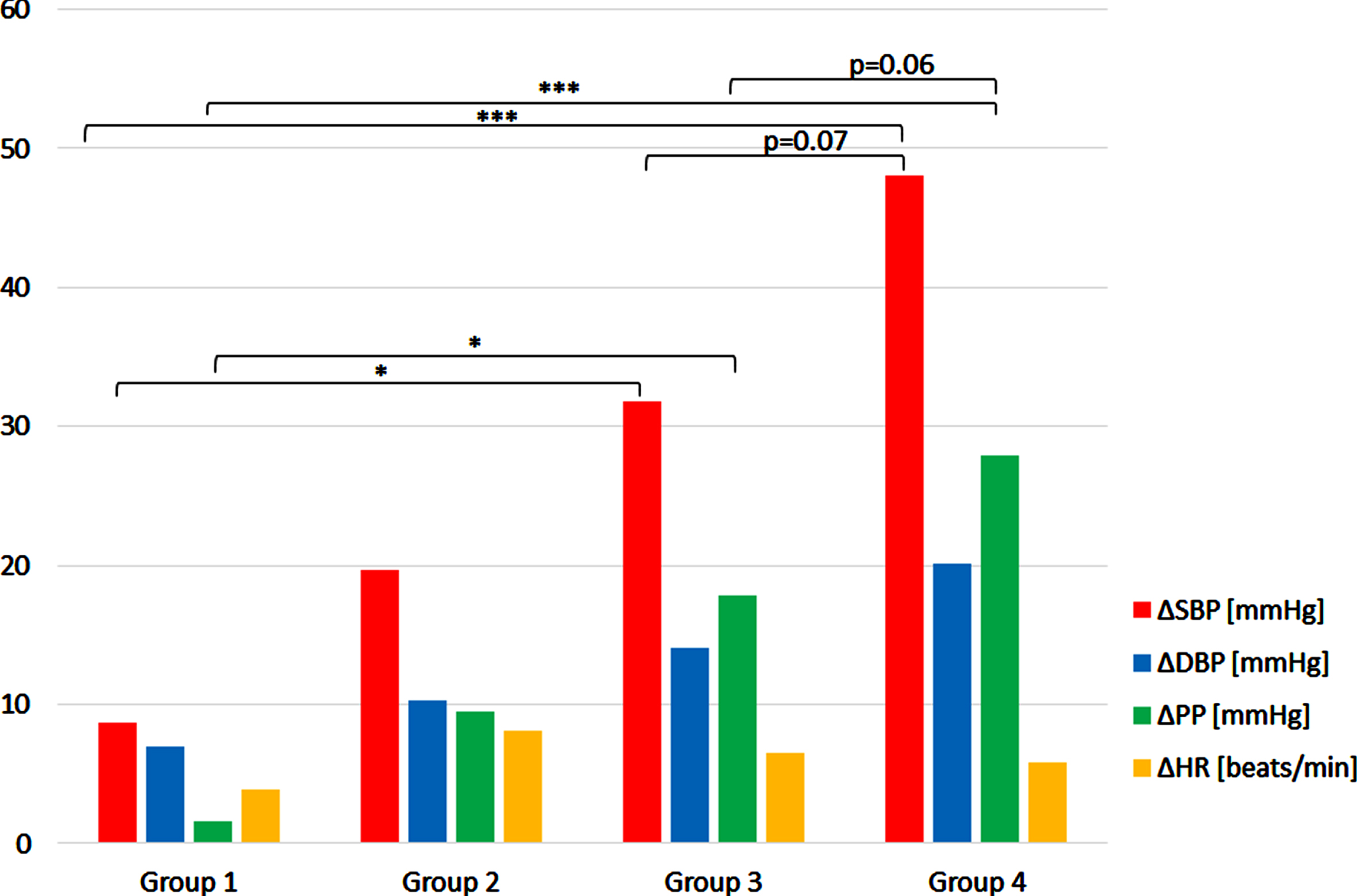
There is a clear difference in the pressure response in deep squat between ‘weak responders’ (group 1) and ‘strong responders’ (group 4), not only in the magnitude of ΔSBP and ΔPP changes, but also in the roles played by diastolic and pulse pressures changes in the pressor response.
Figure 3 shows that in the ‘weak responders’ rise of pulse pressure (ΔPP) contributes less to increase in systolic pressure (ΔSBP) during deep squat than the rise of diastolic pressure (ΔDBP). In ‘intermediate’ (2 and 3) groups ΔPP contribution to rise in systolic pressure gradually increases and peaks in ‘strong responders’ group. The percent increase of PP vs. SBP was 3% vs. 6% in ‘weak responders’; 46% vs. 37% in strong responders. Thus, the greater ΔSBP evoked by deep squat, the greater the role of ΔPP in this response. The 5% rise in HR may play a role in above mentioned 3% SBP increase in ‘weak responders’. However, the contribution to 37% SBP increase in ‘strong responders’ seems to be marginal as HR increased only 7%.
These results suggested the dominant role of type of exercise in PD patients on the magnitude of cardiovascular response, the role of vibration seemed questionable. As may be seen in Fig. 4 the presence of vibrations did not increase ΔSBP in healthy subjects. In this group significant correlation (r = 0.90, p < 0.001) between ΔSBP during deep squat with and without vibration was found (Fig. 4). This proves that the magnitude of the pressor response to deep squat is individually differentiated and reproducible.
Fig. 4
The correlation between ΔSBP during deep squats performed without and with whole body vibration (WVB) in 10 healthy subjects.
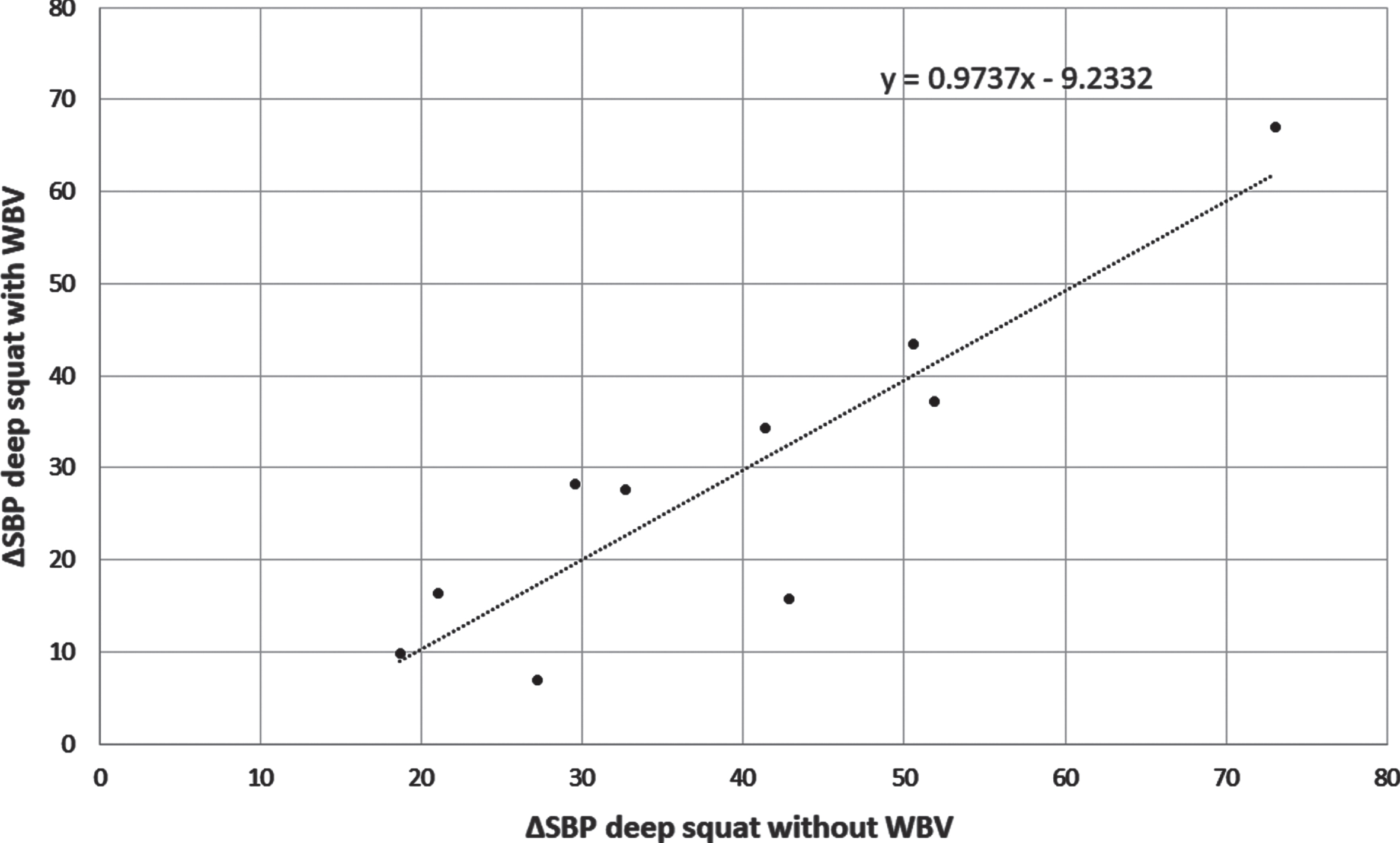
4Discussion
The cardiovascular response of PD patients to static exercises performed with the lower limbs, while standing on the vibration platform have been examined. This study showed that the magnitude of pressor response depended on the form of lower limb static exercise, being the highest during deep squat, intermediate during semi squat, and the lowest during calves exercise. This observation suggests, that of primary importance to the magnitude of pressor response was the form of static exercise performed with lower limbs.
It was found that the magnitude of cardiovascular reaction to exercises performed by PD patients was individually different. This statement was supported by the observation that the persons who had the high response to deep squat had also the high response to semi squat and even to calves, whereas those with low response to one form of exercise had also a low response to the two other forms, what may be seen in Fig. 2. Moreover, it was found that the pressor response in young, healthy subjects had the individual character similarly as in PD patients, and in addition the presence of vibrations did not influence this response (Fig. 4). The lack of effect of vibration on circulatory response, in contrast to some other studies, may stem from the comparatively small amplitude of vibration applied in this study. Though vibration likely did not effect pressor response, the possibility that WBV contributed to increase of lower limbs’ strength in PD patients, observed by us (Niewiadomski et al., 2016), cannot be excluded as the exercises applied to PD patients, where always combined with WBV.
As shown in Fig. 3, the average increase of SBP in the strong responders group (encompassing 25% of examined PD patients) was nearly 50 mmHg, whereas in the weak responders group (also encompassing 25% of examined PD patients) SBP increase was merely 10 mmHg. Of note is the observation that the maximal SBP when performing deep squat amounted to 215 mmHg. Therefore, when performing deep and semi squats the individual pressor response to these exercises should be examined prior to their application as a training modality.
The exaggerated pressor response is not driven by an excessive HR rise, rather it is related to a change in PP. The greater SBP rise during deep squat (9, 20, 32, and 48 mmHg), the greater PP increase (2, 10, 18, and 28 mmHg) in group 1, 2, 3, and 4, respectively. A similar relation between SBP and PP increases occur during semi squat.
Contrary to the general assumption that blood pressure response to exercise, and specifically to static exercise, is blunted in PD patients (reviewed by Sabino-Carvalho & Vianna, 2020), our study has shown that static effort of short duration performed with lower limbs may cause considerable increase of arterial pressure. Our results do not contradict above assumption, insofar blunted pressor response is ascribed to diminished stimulation and/or effectiveness of sympathetic nervous system.
Static exercise best describes situation of static squats, applied by us in this study. An important feature of these static squats was their duration; 30 seconds. It is because cardiovascular reaction to static exercise has two phases.
The first phase of cardiovascular reaction to static exercise is centrally mediated, acts as long as muscles are volitionally contracted and accelerates HR due to inhibition of parasympathetic nervous system (PNS). PNS inhibition is believed to be caused by central command, i.e. neural signals from the brain structures, responding to an individual’s sense of effort (Williamson et al., 2006).
Mark et al. (1985) showed that, during the first minute of 2 minutes lasting handgrip (HG), muscle sympathetic nervous activity (MSNA) remains at basal level, whereas HR attains its maximal level and arterial blood pressure attains half its maximal level. This observation was confirmed by Seals & Enoka (1989), who showed that after first minute of isometric handgrip performed at 30% of maximal voluntary contraction (30% MVC) to exhaustion, MSNA remained at baseline, HR almost reached its maximal level and mean arterial pressure (MAP) clearly increased, though this first minute rise was a fraction of final (i.e. end-HG) MAP level, which was reached after several minutes of HG.
In second phase of HG performed at least with 30% MVC pressor response relays predominantly on increased sympathetic activity. This increase is believed to be caused by gradual accumulation of exercise metabolites, if the force of contraction is strong enough to prevent blood outflow from contracted muscles. These metabolites, notably lactic acid, excites acid sensitive sensors what in turn activates group IV afferent neurons, what leads to excitation of sympathoexcitatory neurons in the rostral ventrolateral medulla (Potts, 2006). The time needed for accumulation of metabolites explains, why during HG, MSNA starts to increase with ca. one minute delay after HG commencement. Also supporting the role of accumulated metabolites in stimulating sympathetic nervous system is the observation, that when contracted arm is occluded with cuff short before the end of HG and in consequence, muscle metabolites remain entrapped in the muscle, then MSNA and arterial pressure remain elevated, though HR may decline (Mark et al., 1985).
It was shown (Sabino-Carvalho et al., 2019) that in the first phase of cardiovascular response to HG, rise of MAP was greater in PD than in young healthy subjects but slightly less than in older, age matched, healthy ones. This was not explainable by differences in heart rate increases, as they were incompatible with the pattern of MAP increases. However, in the second phase of HG Sabino-Carvalho et al. (2018) found in PD patients attenuated pressor response, which was maintained also during postexercise ischemia.
As mentioned above, the initial blood pressure increase during static exercise is believed to be caused by HR increase caused by inhibition of parasympathetic tone. Such mechanism may be operating in ‘weak responders’ because small percent increase in SBP is paralleled by small increase in HR. It is unlikely that this mechanism solely explains the SBP in ‘strong responders’, as percent increase of SBP by far exceeds that of HR.
We hypothesize that there exists yet another mechanism, capable of producing considerable rise of arterial pressure in some PD patients as well as in some young healthy persons. This mechanism acts by increasing pulse pressure. We think that most likely reason of pulse pressure increase is an increase of stroke volume (Dart & Kingwell, 2001). Notably, such increase of stroke volume, if this is the right supposition, should depend on the kind of squat; the heavier the effort, the greater stroke volume increase. Further, we think it possible that mechanical compression of visceral organs during squats may direct some blood from splanchnic bed into central circulation. Shoemaker et al. (2007) found that rise of arterial pressure during handgrip was partly due to stroke volume increase and that this was accompanied by reduction of portal vein diameter. However, this diameter reduction was likely of neurogenic nature as subjects were in supine position, so any external mechanical action on visceral structures was unlikely and reduction occurred first in second minute of effort, suggesting sympathetic driven vasoconstriction.
In our case, arterial pressure rose within few heartbeats after assuming squat position, what is compatible with rapid compression of splanchnic blood reservoirs and time needed to transfer increased volume of blood from periphery to systemic arteries. It is also plausible that the impact of lower trunk muscles upon visceral organs is lesser with lighter effort, thus driving less blood from them and producing smaller increase of stroke volume.
Our subjects presented continuum of PP increases, from small to conspicuous, thus it is likely that above mentioned mechanism and heart rate driven increase of arterial pressure operate simultaneously, albeit the contribution of blood translocation from visceral reservoir is individually different. Furthermore, this mechanism may be operative in strength training performed with lower limbs, as it may cause compression of visceral organs. This may be relevant for PD patients, as strength training is a recommended element of their rehabilitation (Paolucci et al., 2020).
In this study, thanks to continuous measurement of arterial pressure, we were able to see the rapid rise in PP immediately at the beginning of exercise. This rapid and transient appearance, combined with present knowledge of pressor response to static efforts allowed us to formulate the hypothesis on mechanical origin of these form of arterial pressure changes.
In this context, it is evident, that our study is deficient on lack of reliable stroke volume measurement and estimate of splanchnic blood volume. The device used by us, Portapres provides theoretically stroke volume data, however due to doubts concerning reliability of these stroke volume estimates, based on our own experience and on that of the others (Dyson et al., 2010), we abstained to publish these results.
5Conclusions
Based on this study, it is believed that the magnitude of pressor response to deep squat and semi squat in PD patients and healthy persons is an individual trait, increase of SBP ranges from 10 mm Hg in weak responders to 50 mm Hg in strong responders. This difference in SBP rise is accompanied by difference in PP increase, lowest in weak responders and highest in strong responders, what indirectly suggests the role of SV in individual response. Exaggerated pressor response may pose some load on cardiovascular system, therefore arterial pressure should be measured when performing these exercises.
Conflict of interest
The authors certify that there is no conflict of interest with any financial organization regarding the material discussed in the manuscript.
Acknowledgments
Special thanks go to all research participants for their time and engagement.
Funding
This research was funded by a grant from the National Science Centre, Poland (2011/01/D/NZ7/04405).
References
1 | Ahlborg, L. , Andersson, C. , & Julin, P. ((2006) ) Whole-body vibration training compared with resistance training: Effect on spasticity, muscle strength and motor performance in adults with cerebral palsy. Journal of Rehabilitation Medicine 38: , 302–308. |
2 | Aoyama, A. , Yamaoka-Tojo, M. , Obara, S. , Shimizu, E. , Fujiyoshi, K. , Noda, C. , Matsunaga, A. , & Ako, J. ((2019) ) Acute effects of whole-body vibration training on endothelial function and cardiovascular response in elderly patients with cardiovascular disease. International Heart Journal 60: , 854–861. |
3 | Avelar, N. C. , Simão, A. P. , Tossige-Gomes, R. , Neves, C. D. , Mezencio, B. , Szmuchrowski, L. , Coimbra, C. C. , & Lacerda, A. C. ((2011) ) Oxygen consumption and heart rate during repeated squatting exercises with or without whole-body vibration in the elderly. The Journal of Strength Conditioning Research 25: , 3495–3500. |
4 | Cochrane, D. J. , Sartor, F. , Winwood, K. , Stannard, S. R. , Narici, M. V. , & Rittweger, J. ((2008) ) A comparison of the physiologic effects of acute whole-body vibration exercise in young and older people. Archives of Physical Medicine and Rehabilitation 89: , 815–821. |
5 | Dart, A. M. , & Kingwell, B. A. ((2001) ) Pulse pressure–a review of mechanisms and clinical relevance. Journal of the American College of Cardiology 37: , 975–984. |
6 | Delecluse, C. , Roelants, M. , & Verschueren, S. ((2003) ) Strength increase after whole-body vibration compared with resistance training. Medicine & Science in Sports Exercise 35: , 1033–1041. |
7 | Dias, T. , & Polito, M. ((2015) ) Acute cardiovascular response during resistance exercise with whole-body vibration in sedentary subjects: A randomized cross-over trial. Research in Sports Medicine 23: , 253–264. |
8 | Dipla, K. , Kousoula, D. , Zafeiridis, A. , Karatrantou, K. , Nikolaidis, M. G. , Kyparos, A. , Gerodimos, V. , & Vrabas, I. S. ((2016) ) Exaggerated haemodynamic and neural responses to involuntary contractions induced by whole-body vibration in normotensive obese versus lean women. Experimental Physiology 101: , 717–730. |
9 | Dyson, K. S. , Shoemaker, J. K. , Arbeille, P. , & Hughson, R. L. ((2010) ) Modelflow estimates of cardiac output compared with Doppler ultrasound during acute changes in vascular resistance in women. Experimantal Physiology 95: , 561–568. |
10 | Genever, R. W. , Downes, T. W. , & Medcalf, P. ((2005) ) Fracture rates in Parkinson’s disease compared with age- and gender-matched controls: A retrospective cohort study. Age Ageing 34: , 21–24. |
11 | Hazell, T. J. , Thomas, G. W. , Deguire, J. R. , & Lemon, P. W. ((2008) ) Vertical whole-body vibration does not increase cardiovascular stress to static semi-squat exercise. European Journal of Applied Physiology 104: , 903–908. |
12 | Koller, W. , & Kase, S. ((1986) ) Muscle strength testing in Parkinson’s disease. European Neurology 25: , 130–133. |
13 | Liao, L. R. , Ng, G. Y. , Jones, A. Y. , & Pang, M. Y. ((2015) ) Cardiovascular stress induced by whole-body vibration exercise in individuals with chronic stroke. Physical Therapy 95: , 966–977. |
14 | MacDougall, J. D. , Tuxen, D. , Sale, D. G. , Moroz, J. R. , & Sutton, J. R. ((1985) ) Arterial blood pressure response to heavy resistance exercise. Journal of Applied Physiology 58: , 785–790. |
15 | Machado, A. , García-López, D. , González-Gallego, J. , & Garatachea, N. ((2010) ) Whole-body vibration training increasesmuscle strength and mass in older women: A randomized-controlledtrial. Scandinavian Journal of Medicine & Science in Sports 20: , 200–207. |
16 | Mark, A. L. , Victor, R. G. , Nerhed, C. , & Wallin, B. G. ((1985) ) Microneurographic studies of the mechanisms of sympathetic nerve responses to static exercise in humans. Circulation Research 57: , 461–469. |
17 | Muir, J. , Kiel, D. P. , & Rubin, C. T. ((2013) ) Safety and severity of accelerations delivered from whole body vibration exercise devices to standing adults. Journal of Science and Medicine in Sport 16: , 526–531. |
18 | Niewiadomski, W. , Strasz, A. , Karbowniczek, A. , Gasiorowska, A. , Żyliński, M. , Pariaszewska, K. , Cybulski, G. , Palasz, E. , & Niewiadomska, G. ((2016) ) Changes in maximum static force of kneeextensors caused by prolonged whole body vibration training inParkinson Disease patients. Parkinsonism & Related Disorders 22: (Supplement 2), e67. |
19 | Niewiadomski, W. , Cardinale, M. , Gasiorowska, A. , Cybulski, G. , Karuss, B. , & Strasz, A. ((2005) ) Could vibration training be an alternative to resistance training in reversing sarcopenia? Journal of Human Kinetics 14: , 3–20. |
20 | Pang, M. Y. ((2010) ) Whole body vibration therapy in fracture prevention among adults with chronic disease. World Journal of Orthopedics 1: , 20–25. |
21 | Paolucci, T. , Sbardella, S. , La Russa, C. , Agostini, F. , Mangone, M. , Tramontana, L. , Bernetti, A. , Paoloni, M. , Pezzi, L. , Bellomo, R. G. , Santilli, V. , & Saggini, R. ((2020) ) Evidence of rehabilitative impact of progressive resistance training (PRT) programs in parkinson disease: An umbrella review. Journal of Parkinson’s Disease 2020: , 9748091. |
22 | Potts, J. T. ((2006) ) Inhibitory neurotransmission in the nucleus tractus solitarii: Implications for baroreflex resetting during exercise. Experimental Physiology 91: , 59–72. |
23 | Rees, S. S. , Murphy, A. J. , & Watsford, M. L. ((2008) ) Effects of whole-body vibration exercise on lower-extremity muscle strength and power in an older population: A randomized clinical trial. Physical Therapy 88: , 462–470. |
24 | Rittweger, J. , Beller, G. , & Felsenberg, D. ((2000) ) Acute physiological effects of exhaustive whole-body vibration exercise in man. Clinical Physiology 20: , 134–142. |
25 | Robbins, D. , Yoganathan, P. , & Goss-Sampson, M. ((2014) ) The influence of whole body vibration on the central and peripheral cardiovascular system. Clinical Physiology and Functional Imaging 34: , 364–369. |
26 | Sabino-Carvalho, J. L. , Samora, M. , Teixeira, A. L. , Daher, M. , & Vianna, L. C. ((2019) ) Circulatory responses at the onset of handgrip exercise in patients with Parkinson’s disease. Experimental Physiology 104: , 793–799. |
27 | Sabino-Carvalho, J. L. , Teixeira, A. L. , Samora, M. , Daher, M. , & Vianna, L. C. ((2018) ) Blunted cardiovascular responses to exercise in Parkinson’s disease patients: Role of the muscle metaboreflex. Journal of Neurophysiology 120: , 1516–1524. |
28 | Sabino-Carvalho, J. L. , & Vianna, L. C. ((2020) ) Altered cardiorespiratory regulation during exercise in patients with Parkinson’s disease: A challenging non-motor feature. SAGE Open Medicine 8: , 2050312120921603. |
29 | Sá-Caputo, D. C. , Paineiras-Domingos, L. L. , Oliveira, R. , Neves, M. F. T. , Brandão, A. , Marin, P. J. , Sañudo, B. , Furness, T. , Taiar, R. , & Bernardo-Filho, M. ((2018) ) Acute effectsof whole-body vibration on the pain level, flexibility, andcardiovascular responses in individuals with metabolic syndrome. Dose Response 16: , 1559325818802139. |
30 | Seals, D. R. , & Enoka, R. M. ((1989) ) Sympathetic activation is associated with increases in EMG during fatiguing exercise. Journal of Applied Physiology 66: , 88–95. |
31 | Shoemaker, J. K. , Mattar, L. , Kerbeci, P. , Trotter, S. , Arbeille, P. , & Hughson, R. L. ((2007) ) WISE 2005: Stroke volume changes contribute to the pressor response during ischemic handgrip exercise in women. Journal of Applied Physiology (1985) 103: , 228–233. |
32 | Silva-Batista, C. , Corcos, D. M. , Roschel, H. , Kanegusuku, H. , Gobbi, L. T. , Piemonte, M. E. , Mattos, E. C. , DE Mello, M. T. , Forjaz, C. L. , Tricoli, V. , & Ugrinowitsch, C. ((2016) ) Resistance training with instability for patients with Parkinson’s disease. Medicine & Science in Sports & Exercise 48: , 1678–1687. |
33 | Tamini, S. , De Micheli, R. , Tringali, G. , Bernardo-Filho, M. , & Sartorio, A. ((2020) ) Acute effects of whole-body vibration exercises at 2 different frequencies versus an aerobic exercise on some cardiovascular, neuromotor and musculoskeletal parameters in adult patients with obesity. Dose Response 18: , 1559325820965005. |
34 | Wei, N. , Pang, M. Y. , Ng, S. S. , & Ng, G. Y. ((2017) ) Optimal frequency/time combination of whole-body vibration training for improving muscle size and strength of people with age-related muscle loss (sarcopenia): A randomized controlled trial. Geriatrics & Gerontology International 17: , 1412–1420. |
35 | Williamson, J. W. , Fadel, P. J , & Mitchell J. H. ((2006) ) New insights into central cardiovascular control during exercise in humans: A central command update. Experimantal Physiology 91: , 51–58. |
36 | Wollersheim, T. , Haas, K. , Wolf, S. , Mai, K. , Spies, C. , Steinhagen-Thiessen, E. , Wernecke, K. D. , Spranger, J. , & Weber-Carstens, S. ((2017) ) Whole-body vibration to prevent intensive care unit-acquired weakness: Safety, feasibility, and metabolic response. Critical Care 21: , 9. |