Pharmacological management of sleep after traumatic brain injury
Abstract
Sleep-wake disturbances (SWD) are prevalent in the traumatic brain injury (TBI) population and may exacerbate related neurobehavioral impairments. As such, it is important to recognize and treat SWD early to allow for optimal cognitive recovery following a TBI. A number of medications are currently available for treatment of SWD. However, most research in this area has historically focused on neurologically intact populations. This article reviews key pharmacologic treatment principles and agents to consider for use in the treatment of TBI-related SWD. In addition, it highlights available research literature that has examined the use of sleep medications in this unique population.
1Introduction
Sleep-wake disturbances (SWD) following traumatic brain injury (TBI) are well documented. Examples of SWD may include excessive daytime sleepiness, increased sleep need per 24 hours, insomnia, hypersomnia, and narcolepsy. Estimates of the frequency of SWD following TBI range from 36–70% (Matthias, & Alvaro 2012). A meta-analysis from 2012 included 21 sleep studies involving mild to severe TBI subjects (Matthias, & Alvaro 2012). 51% of subjects with a TBI were found to have a SWD. Kempf prospectively studied mild to severe TBI subjects at six months and three years following their injury. SWD was found in 72% of subjects six months after injury and in 67% of subjects three years after injury with 14 subjects lost to follow-up (Kempf et al., 2010). Interestingly, no association with severity of brain injury and SWD was noted. The most prevalent SWD six months after injury was excessive daytime sleepiness and/or fatigue (55%) and insomnia was only noted in 5% of subjects.
Impaired sleep may exacerbate neurobehavioral impairments commonly seen following TBI, such as agitation, depression, anxiety, slowed processing speed, attention, and memory. Bloomfield noted a positive association between “good” sleepers and sustained attention in a study examining primarily severe TBI subjects. Using a Pittsburgh Sleep Quality Index cut-off of 6, 52% of participants were categorized as a poor sleeper and 48% as a good sleeper. The poor sleep group demonstrated significantly poorer sustained attention compared to the good sleep group (Bloomfield, Espie, & Evans 2010). Given the frequency of SWD in the TBI population and its potential impact on neurobehavioral impairments, it is important to monitor for this condition and optimize sleep-wake cycle regulation early in the recovery process.
1.1Clinical significance
TBI is a significant cause of disability in the United States and worldwide. An estimated 1.6–3 million TBIs occur in the United States each year. Insomnia, fatigue, and sleepiness are the most frequent patient complaints following TBI (Viola-Saltzman, & Watson 2012). As mentioned above, multiple studies have suggested that there is no association between the severity of TBI and likelihood of developing SWD. The cause and effect relationship between SWD following TBI and fatigue, mood disorder, anxiety, and neurobehavioral impairments is uncertain, but frequently associated with one another. It is well recognized that minimizing sleep-wake cycle disturbance is associated with improvement in fatigue levels, improved mood, decreased levels of anxiety, and improvement in cognitive functioning. In summary, SWD are common following TBI and are associated with related neurobehavioral impairments. Therefore, it is important that those who provide care for TBI patients screen for SWD, recognize related neurobehavioral impairments, and understand evidence based treatment options.
2Treatment principles
The treatment of SWD after TBI usually requires a multifactorial approach. First-line treatment should focus on non-pharmacological interventions. Sleep hygiene can be optimized through key behavioral and environmental practices including establishing a regular bedtime routine, creating a restful bedroom environment with minimal light and ideal ambient temperature, minimizing daytime napping, limiting evening caffeine and alcohol intake, and avoiding late night screen-time. In addition to sleep hygiene, other non-pharmacological treatments have been supported by the literature. Cognitive behavioral therapy (CBT) may be a safe and effective therapy for SWD. In a randomized controlled trial evaluating the efficacy of adapted CBT for TBI-related SWD, CBT recipients reported greater sleep quality compared to individuals who received usual treatment only (Nguyen et al., 2017). Acupuncture has also been found to have a beneficial effect on the perception of sleep or sleep quality in TBI survivors with insomnia (Zollman et al., 2012). Finally, bright light therapy can regulate sleep-wake cycles through targeted exposure to short wavelength blue light in the morning hours. In a study examining 28 individuals who had sustained a mild TBI, blue light exposure led to an earlier bedtime and rise time as well as lower daytime sleepiness compared to placebo (Killgore et al., 2017). It is also important to assess for sleep-related breathing disorders such as obstructive sleep apnea (OSA), which occurs with increased frequency after TBI. Some studies suggest the prevalence of post-TBI OSA may be as high as 25–35% (Castriotta et al., 2007 and Webster et al., 2001). Continuous Positive Airway Pressure (CPAP) and Bilevel Positive Airway Pressure (BiPAP) should be initiated for the treatment of such disorders. Other treatments may also include weight loss and adjustments in body positioning during sleep, mandibular advancement devices, or surgical interventions.
Once non-pharmacological interventions have been utilized, there may be further indication to initiate a pharmacologic agent to promote sleep. It is important to acknowledge that most research regarding the side effects of sleep medications has focused on neurologically intact populations and little evidence is available regarding the tolerance of side effects in a TBI population. Therefore, as a general principle, it is usually recommended to start at low medication dosages and titrate slowly to monitor for any potential adverse effects.
Another key principle is to minimize the use of sleep medications that may have adverse effects on cognition. Benzodiazepines exert effects on gamma-aminobutyric acid-A (GABA-A), the primary inhibitory neurotransmitter throughout the brain. Due to this mechanism of action, benzodiazepines may have the potential to impair long-term neurological recovery and cause daytime sedation and anterograde amnesia (Goldstein 1995; Schallert, Hernandez, & Barth 1986). In addition, this class of medications carries risk for dependency and abuse. Several studies have found an association with benzodiazepine use and cognitive impairment in neurologically intact populations. In a study that compared a group of long-term benzodiazepine users with six months of abstinence to a group with anxiety that had never been treated with benzodiazepines, lower scores were noted on several memory scales and a visual spatial learning test (Malec, Ivnik, & Hinkedly 1991). A meta-analysis reported that long-term benzodiazepine users made some improvement in cognitive functions following a period of abstinence, but they continued to score lower than controls with no history of benzodiazepine exposure (Barker et al., 2005). To date, the impact of benzodiazepines on cognitive function in the TBI population has not been reported. Although benzodiazepines have been shown to decrease time to sleep onset, decrease nocturnal awakenings, and increase total sleep time, benzodiazepines undesirable side effect profile makes their routine use not recommended in the TBI population (Flanagan, Greenwald, & Wieber 2007).
Tricyclic antidepressants and some H1 antagonists, such as diphenhydramine, have anticholinergic properties that can have detrimental cognitive side effects such as memory impairment and altered mental status. Their use should be cautioned in the TBI population – particularly in those with severe injury. The relationship between anticholinergic medications and cognitive impairment has been well documented. In a review of 27 studies, which used serum anticholinergic assay as the main method to determine anticholinergic activity, all but two studies found an association between the anticholinergic activity of medications and either delirium or cognitive impairment (Campbell et al., 2009). These studies included neurologically intact populations. Caution should also be given to the use of tricyclic antidepressants in the moderate to severe TBI population given their ability to lower the seizure threshold (Flanagan, Greenwald, & Wieber 2007). In the mild TBI population, consideration may be given to the use of nortriptyline and amitriptyline – especially if one is suffering from posttraumatic headaches, as these agents are commonly accepted agents for the management of posttraumatic headaches. In addition Doxepin, another tricyclic antidepressant, promotes sleep maintenance and does not exert anticholinergic side effects at low dosages.
3Pharmacologic agents
There are several pharmacologic agents that may be utilized for the treatment of TBI-related SWD.
3.1Nonbenzodiazepine GABA-A agonists
Despite a paucity of evidence for use in the TBI population, hypnotics are one of the most frequently prescribed classes of medications in the acute rehabilitation TBI population. In a sample of 2,130 TBI patients (complicated mild, moderate or severe), hypnotic agents were prescribed for 30% of the patients (Hammond et al., 2015). The most commonly prescribed hypnotics were nonbenzodiazepine gamma-aminobutyric acid-A (GABA-A) agonists, with zolpidem being the most prescribed medication in this class followed by eszopiclone and zaleplon. These agents bind more selectively to subtypes of GABA-A receptors, which results in less cognitive side effects and lower risk for dependence and abuse compared to traditional benzodiazepine GABA-A agonists. Zolpidem and zaleplon have relatively short half-lives (2.5 hours and 1 hour, respectively) and are most effective for facilitating sleep onset compared to longer acting agents such as controlled-released zolpidem and eszopiclone which can promote both sleep onset and maintenance (Minkel & Krystal, 2013). Given the short half-life of nonbenzodiazepine GABA-A agonists, the expectation is that there would be minimal next day residual effects when compared to the benzodiazepine GABA-A agonists (alprazolam has a half life 9–16 hours). There has been little research on the use of nonbenzodiazepine GABA-A agonists in the TBI population. Li compared the effects of lorazepam and zopiclone (no longer available in the United States; however, its stereoisomer, eszopiclone is commonly used) in patients with stroke or brain injury in a double-blind cross-over trial. Twenty individuals were randomized to either group. There was no difference in scores on the Mini-Mental State examination, although this is known to be an insensitive measure for cognitive impairment (Li, Shan, & Ashworth 2004). In neurologically intact populations, the nonbenzodiazapine GABA-A agonists have generally been found to cause temporary memory impairment that resolves within 6–8 hours of administration (Larson, & Zollman 2010). Other common adverse effects to monitor for with use of nonbenzodiazapine GABA-A agonists include headache, dizziness, and nausea. A multicenter, randomized, crossover study investigated the efficacy of eszopiclone 1–3 mg or zolpidem 10 mg compared to placebo using latency to persistent sleep as measured by polysomnography as its primary endpoint. This study involved a neurologically intact population. There was a statistically significant difference for all treatment doses of eszopiclone and zolpidem compared to placebo in decreasing latency to persistent sleep. Of note, there was also a statistically significant difference in central nervous system adverse events in the eszopiclone and zolpidem groups compared to placebo (Erman et al., 2008).
3.2Melatonin receptor agonists
Melatonin is an endogenous hormone that plays a key role in the internal timing of biological rhythms including the regulation of sleep-wake cycles. One study found that patients with TBI showed 42% less melatonin production overnight compared with healthy controls (Grima et al., 2016). Evidence suggests that exogenous melatonin supplementation may help reset the body’s sleep-wake cycles and improve daytime alertness (Kemp et al., 2004). A randomized, double-blinded, cross-over trial compared melatonin 5 mg to amitriptyline 25 mg in a TBI population (Minkel, & Krystal 2013). For either medication, there was no difference in sleep latency, duration, quality, or daytime alertness compared to baseline. Patients on melatonin, however, reported improved daytime alertness compared to baseline.
Fig. 1.
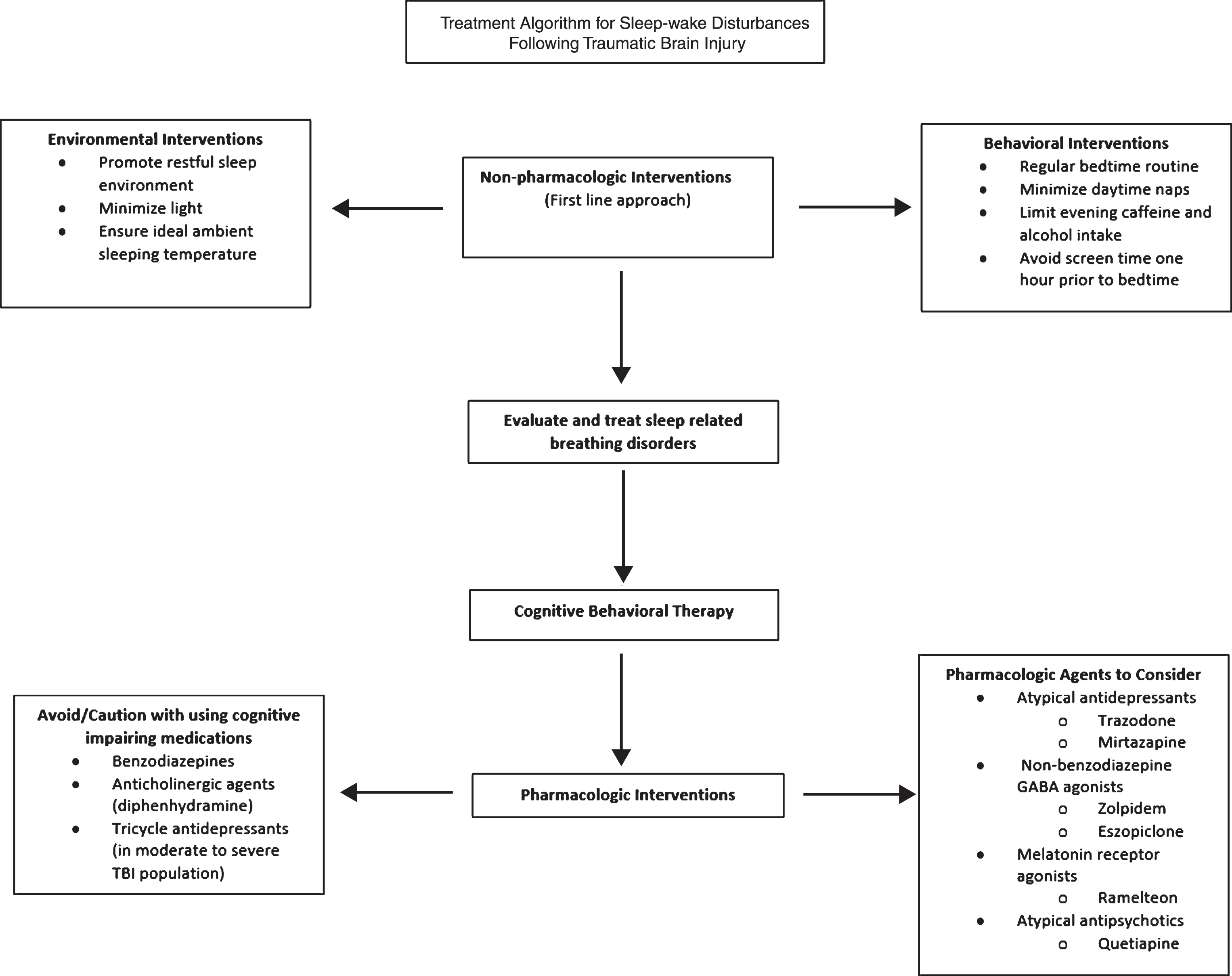
Ramelteon is a melatonin receptor agonist approved by the Food and Drug Administration for the treatment of insomnia with sleep onset abnormalities. Preliminary evidence from a double-blind placebo-controlled trial of 13 individuals with mild to severe TBI demonstrated improvements in total sleep time and some aspects of cognitive functioning following a 3-week trial of ramelteon at a dose of 8 mg compared to placebo (Lequerica et al., 2015). Interestingly, the subjects had increased sleep onset latency with ramelteon compared to placebo. Ramelteon is an intriguing pharmacologic choice for the TBI population given its low side effect profile and limited potential to exacerbate neurobehavioral deficits. While its side effects have not been studied in a TBI population, in a geriatric population it did not appear to influence middle of the night balance deficits or morning after word list recall (Erman et al., 2006).
3.3Antidepressants
Antidepressants are often used in the off-label treatment of SWD. Trazodone is an atypical antidepressant medication commonly utilized to promote sleep maintenance following a TBI. At lower doses, it acts as an effective hypnotic agent by blocking 5-hydroxytryptamine HT2A receptors and H1 histamine and α1 adrenergic receptors (Stahl, 2009). There are no clinical trials to date that have studied trazodone for sleep-wake cycle regulation in the TBI population. A recent meta-analysis included seven randomized placebo controlled trials (429 subjects) investigating the efficacy of trazodone for treatment of insomnia in a neurologically intact population. The meta-analysis found that trazodone was effective in decreasing the number of early awakenings, but there was no significant improvement in sleep efficiency and other objective measures of sleep (Yi et al., 2018). And while cognitive performance on trazodone has not been studied in a TBI population, in a double-blind, randomized design, trazodone did not influence cognitive performance in a depressed population (Fudge et al., 1990). Common adverse effects to monitor for with use of trazodone include orthostatic hypotension, headache, nausea, dry mouth, and more rarely priapism.
Another atypical antidepressant, mirtazapine, is believed to have sleep promoting effects through antagonism of 5HT2 and 5HT3 serotonin, H1 histamine and α1 adrenergic receptors (Minkel & Krystal 2013). Due to the common side effect of weight gain, low dose mirtazapine may be considered for use in the treatment of patients with SWD who have concomitant mood and appetite disturbances. It should be noted that at high doses, mirtazapine can have an activating effect and contribute to poor sleep. Although Mirtazapine has not been studied in the TBI population, there is some evidence to support its efficacy for treatment of insomnia in a population with major depressive disorder. Mirtazapine was compared to Venlafaxine in an eight week randomized double blinded study using polysomnography to evaluate for objective changes in sleep parameters. Polysomnography studies were obtained at baseline, one, two, and eight weeks following medication initiation. At two weeks, the mirtazapine group was noted to have statistically significant improvements in sleep latency, sleep efficiency, and wake after sleep onset; whereas, the venlafaxine group did not – even by eight weeks (Miljatovic, 2012).
3.4Other agents
There are less traditional sleep agents that may be considered for use in TBI patients with SWD and other coexistent conditions. Atypical antipsychotics, such as low dose quetiapine and olanzapine, may have utility in patients with SWD and agitation. However, caution should be used with administration of antipsychotics in the TBI population due to the risk for altered cognition and extrapyramidal symptoms associated with dopamine blockade. Quetiapine is more commonly used than olanzapine for isolated SWD in the TBI population given that it is a potent histamine H1 antagonist and is the least potent dopamine antagonist of the atypical antipsychotics. In a healthy population, quetiapine 50 mg was compared to mirtazapine 7.5 mg in a double-blind, crossover, placebo-controlled trial (Karsten, Hagenauw, & Kamphuis 2017). Residual effects of next day alertness and cognitive functioning were examined. Sleep was assessed using polysomnography. Both mirtazapine and quetiapine increased total sleep time by 30 minutes and decreased awakenings by 35–40%. However, quetiapine increased the duration of non-rapid eye movement sleep, stage N2; whereas, mirtazapine mainly increased deep sleep stage N3. Both quetiapine and mirtazapine caused daytime sleepiness and decreased sustained attention when compared to placebo.
Other less traditional sleep agents that may be considered for use in the treatment of TBI-related SWD include anticonvulsants such as gabapentin when concomitant neuropathic pain is present. The efficacy of gabapentin for treatment of insomnia is questionable. Efficacy for insomnia treatment has not been studied in a TBI population. A small (22 subjects) randomized double-blind placebo-controlled trial studying gabapentin compared to placebo in an alcohol dependent population did not find a statistically significant improvement in subjective sleep quality nor in objective measures of polysomnography after six weeks of treatment at a dose of 1500 mg at bedtime (Brower et al., 2008).
4Conclusion
Sleep wake disturbance is a common finding in the TBI population and can be seen in the full spectrum of injury – from mild to severe TBI. Sleep wake disturbances have been associated with neurobehavioral deficits and poorer performance on cognitive testing. Non-pharmacological treatment, such as implementation of sleep hygiene practices and cognitive behavioral therapy, may be effective in helping to regulate SWD in TBI. However in the acute stages of recovery from severe TBI, implementation of such strategies is limited due to impaired cognition. Pharmacologic intervention for regulation of SWD is reasonable in the TBI population as improved sleep may help lessen daytime sleepiness, fatigue, neurobehavioral impairments, and improve aspects of cognition. Caution must be used with the use of pharmacologic agents in the treatment of SWD in the TBI population. In particular, use of benzodiazepine GABA-A agonists should be avoided in the TBI population due to potential for contributing to impaired cognition and potential for impairing neurological recovery. There is less concern with use of the non-benzodiazepine GABA-A agonists given their shorter half-lives, but further studies need to be performed in the TBI population as transient memory impairment has been reported in a healthy population. Overall, there is a paucity of research for the use of sleep promoting agents in the TBI population. Studies are needed to document efficacy of pharmacotherapy for sleep-wake cycle regulation in the TBI population and tolerance of side effects – most notably their influence on cognition and neurobehavioral impairments.
Conflict of interest
The authors do not have any conflict of interest to disclose.
References
1 | Barker, M. , Greenwood, K. , Jackson, M. , & Crowe, S. ((2005) ). An evaluation of persisting cognitive effects after withdrawal from long-term benzodiazepine use. J Int Neuropsychol Soc, 11: (3), 281–289. |
2 | Bloomfield, I. , Espie, C. , & Evans, J. ((2010) ). Do sleep difficulties exacerbate deficits in sustained attention following traumatic brain injury? J of the International Neuropsychological Society, 16: (1), 17–25. |
3 | Brower, K. , Kim, H. , Strobbe, S. , Karam-Hage, M. , Consens, F. , & Zucker, R. ((2008) ). A randomized double-blind pilot trial of gabapentin versus placebo to treat alcohol dependence and comorbid insomnia. Alcoholism: Clinical and Experimental Research, 32: (8), 1429–1438. |
4 | Campbell, N. , Malaz, M. , Limbil, T. , Ott, C. , Fox, C. , Maidment, I. , Schubert, C. , Munger, S. , Fick, D. , Miller, D. , & Gulati, R. ((2009) ). The cognitive impact of anticholinergics: A clinical review. Clinical Interventions in Aging, 4: (1), 225–233. |
5 | Castriotta, R. J. , Wilde, M. C. , Lai, J. M. , Atanasov, S. , Masel, B. E. , & Kuna, S. T. ((2007) ). Prevalence and Consequences of Sleep Disorders in Traumatic Brain Injury. Journal of Clinical Sleep Medicine: JCSM: Official Publication of the American Academy of Sleep Medicine, 3: (4), 349–356. |
6 | Erman, M. , Seiden, D. , Zammit, G. , Sainati, S. , & Zhang, J. ((2006) ). An efficacy, safety, and dose-response study of ramelteon in patients with chronic primary insomnia. Sleep Medicine, 7: (1), 17–24. |
7 | Erman, M. , Zammit, G. , Rubens, R. , Schaefer, K. , Wessel, T. , Amato, D. , Caron, J. , & Walsh, J. ((2008) ). A polysomnographic placebo-controlled evaluation of the efficacy and safety of eszopiclone relative to placebo and zolpidem in the treatment of primary insomnia. Journal of Clinical Sleep Medicine, 4: (3), 229–234. |
8 | Flanagan, S. R. , Greenwald, B. , & Wieber, S. , ((2007) ). Pharmacological treatment of insomnia for individuals with brain injury. J Head Trauma Rehabil, 22: (1), 67–70. |
9 | Fudge, J. , Perry, P. , Garvey, M. , & Kelly, M. ((1990) ). A comparison of the effect of fluoxetine and trazodone on the cognitive functioning of depressed outpatients. J Affect Disorders, 18: (4), 275–280. |
10 | Goldstein, L. B. ((1995) ). Prescribing of potentially harmful drugs to patients admitted to hospital after head injury. J Neurol Neurosurg Psychiatr, 58: (6), 753–755. |
11 | Grima, N. A. , Ponsford, J. L. , St Hilaire, M. A. , Mansfield, D. , & Rajaratnam, S. M. ((2016) ). Circadian melatonin rhythm following traumatic brain injury. Neurorehabil Neural Repair, 30: (10), 972–977. |
12 | Hammond, F. M. , Barrett, R. S. , Shea, T. , Seel, R. T. , McAlister, T. W. , Kaelin, D. ,… & Horn, S. D. ((2015) ). Psychotropic Medication Use during Inpatient Rehabilitation for Traumatic Brain Injury. Archives of Physical Medicine and Rehabilitation, 96: (80), S256–S273. |
13 | Karsten, J. , Hagenauw, L. , & Kamphuis, J. ((2017) ). Low doses of mirtazapine or quetiapine for transient insomnia: A randomized, double-blind, cross-over placebo-controlled trial. J of Psychopharmacology, 31: (3), 327–337. |
14 | Kemp, S. , Biswas, R. , Neumann, V. , & Coughlan, A. ((2004) ). The value of melatonin for sleep disorders occurring post-head injury: A pilot RCT. Brain Inj 18: (9), 911–919. |
15 | Kempf, J. , Werth, E. , Kaiser, P. , Bassetti, C. , & Baumann, C. ((2010) ). Sleep-wake disturbances 3 years after traumatic brain injury. J of Neurology, Neurosurgery, & Psychiatry, 81: (12), 1402–1405. |
16 | Killgore, W. D. , Shane, B. R. , Vanuk, J. R. , Franco, J. , Castellanos, A. , Millan, M. , Grandner, M. A. , & Bajaj, S. ((2017) ). Short Wavelength Light Therapy Facilitates Recovery From Mild Traumatic Brain Injury. Sleep, 40: (1), A426–A427. |
17 | Larson, E. , & Zollman, F. ((2010) ). The effect of sleep medications on cognitive recovery from traumatic brain injury. J of Head Trauma Rehabilitation, 25: (1), 61–67. |
18 | Lequerica, A. , Jasey, N. , Portelli Tremont, J. N. , & Chiaravalloti, N. D. ((2015) ). Pilot study on the effect of Ramelteon on sleep disturbance after traumatic brain injury: Preliminary evidence from a clinical trial. Arch Phys Med Rehabil, 96: (10), 1802–1809. |
19 | Li, P. , Shan, R. , & Ashworth, N. ((2004) ). Comparison of lorazapam and zopiclone for insomnia in patients with stroke and brain injury. Am J Phys Med Rehabil, 83: (1), 421–427. |
20 | Malec, J. , Ivnik, R. , & Hinkedly, N. ((1991) ). Visual spatial learning test. Psychcol Assess, 3: (1), 82–88. |
21 | Matthias, J. , & Alvaro, P. ((2012) ). Prevalence of sleep disturbance, disorders, and problems following traumatic brain injry: A meta-analysis. Sleep Medicine, 13: (7), 898–905. |
22 | Miljatovic, A. ((2012) ). Comparative effects of venlafaxine and mirtazapine on sleep physiology measures in patients with major depressive disorder and insomnia. European Psychiatry, 27: (S1). |
23 | Minkel, J. , & Krystal, A. ((2013) ). Optimizing the Pharmacologic Treatment of Insomnia: Current Status and Future Horizons. Sleep Med Clin, 8: (3), 333–350. |
24 | Nguyen, S. , McKay, A. , Wong, D. , Rajaratnam, S. M. , Spitz G , Williams, G. , Mansfield, D. , & Ponsford, J. L. ((2017) ). Cognitive Behavior Therapy to Treat Sleep Disturbance and Fatigue After Traumatic Brain Injury: A Pilot Randomized Controlled Trial. Arch Phys Med Rehabil, 98: (8), 1508–1817. |
25 | Pandi-Perumal, S. , Srinivasan, V. , Spence, D. , & Cardinali, D. ((2007) ). Role of the melatonin system in the control of sleep: Therapeutic implications. CNS Drugs, 21: , 995–1018. |
26 | Pevet, P. & Challet, E. , ((2011) ). Melatonin: Both master clock output and internal time-giver in the circadian clocks network. J Physiol Paris, 105: , 170–182. |
27 | Schallert,T. , Hernandez, T. D. , & Barth, T. M. ((1986) ). Recovery of function after brain damage: Severe and chronic disruption by diazepam. Brain Res, 379: (1), 104–111. |
28 | Stahl, S. M. ((2009) ). Mechanism of action of trazodone: A multifunctional drug. CNS Spectr, 14: (10), 536–546. |
29 | Viola-Saltzman, M. , & Watson, N. F. ((2012) ). Traumatic Brain Injury and Sleep Disorders. Neurologic Clinics, 30: (4), 1299–1312. |
30 | Webster, J. B. , Bell, K. R. , Hussey, J. D. , Natale, T. K. , Lakshminarayan S. ((2001) ). Sleep apnea in adults with traumatic brain injury: A preliminary investigation. Arch Phys Med Rehabil, 82: , 316–321. |
31 | Yi, X. , Ni, S. , Ghadami, M. , Meng, H. , Chen, M. , Kuang, L. , Zhang, Y. , & Zhou, X. ((2018) ). Trazodone for the treatment of insomnia: A meta-analysis of randomized placebo-controlled trials. Sleep Medicine, 45: , 25–32. |
32 | Zollman, F. S. , Larson, E. B. , Wasek-Throm, L. K. , Cyborski, C. M. , & Bode, R. K. ((2012) ). Acupuncture for Treatment of Insomnia in Patients With Traumatic Brain Injury: A Pilot Intervention Study. Journal of Head Trauma Rehabilitation, 27: (2), 135–142. |