Comparison of posture and muscle stiffness between adolescent boys with haemophilia and age-matched healthy peers: An observational comparative study
Abstract
BACKGROUND:
Haemophilic arthropathy is likely to influence posture and muscle stiffness in adolescent male haemophilia patients (HP).
OBJECTIVE:
This study aimed to investigate the possible change in upright standing posture and stiffness of the superficial postural muscles in HP.
METHODS:
Twenty-two HP aged between 12 and 19 years and twenty-two healthy peers were included in the study. The photogrammetry was used to assess the upright standing posture from sagittal and frontal planes. Also, stiffness of the superficial postural muscles was evaluated using the myotonometry.
RESULTS:
A significant difference was found in the craniovertebral, lumbar lordosis, knee flexion-valgus, and ankle plantar flexion angles between the groups (
CONCLUSION:
This study revealed that the ankle, knee, lumbar, and craniovertebral segments’ posture angles were changed and the stiffness of the rectus femoris muscle decreased in HP. Postural alterations and reduced rectus femoris stiffness should be considered in management of the musculoskeletal complications of haemophilia.
1.Introduction
Haemophilia is a congenital bleeding disorder caused by a deficiency of the X-linked coagulation factors (factor VIII in haemophilia A and factor IX in haemophilia B). Bleeding into the synovial joints occurs repeatedly in patients with haemophilia [1]. Failure to prevent such hemorrhages causes destruction of articular cartilage, joint deterioration [1], balance impairment [2], and gait disorder [3, 4] and eventual worsening of quality of life [5, 6].
Posture, the attitude held by the body, is a strategy implemented by the human body to counter gravity and environmental forces in the most comfortable and economical fashion; therefore, stresses on the musculoskeletal system are minimal in the correct posture [7]. In some musculoskeletal diseases, joint disorders and muscular imbalance may cause alteration in posture [8, 9]. Posture disorders result in pain, deformity, or functional limitation in adolescents, and affect the spinopelvic and extremity alignment in adulthood [10, 11]. Misalignment of body segments can induce deterioration of the balance of pressure on the joints and cause the load on the joint to exceed natural limits at certain points [12, 13]. Impairment of the intra-articular load balance in haemophilia patients (HP) may increase the risk of bleeding in areas where the load is high [11]. Hmida et al. found that in patients with severe haemophilia some posture parameters differ in gait, but not in an upright position [14]. However, this study was performed with adult HP who had severe joint damage, and their lower extremity posture was not evaluated.
Changes in posture may be associated with the alteration in stiffness of agonist and antagonist muscles [15]. Muscle stiffness, a parameter of the viscoelastic characteristics of the muscle, is the ability of a muscle to withstand an external force that is attempting to change its shape. Stiffness is an objective parameter that reveals tissue status and can be used by clinicians as a reference in diagnosis and treatment [16, 17]. Muscle stiffness is a characteristic feature that affects segmental alignment or biomechanics [17, 18]. Therefore, it is necessary to determine the alteration in the stiffness of the postural muscles to reveal the possible reasons for a change in segmental alignment [18]. There is no study in the literature investigating possible differences in stiffness of the postural muscles in HP.
In HP, bleeding and musculoskeletal dysfunctions may become evident due to posture disorders [11, 19]. Such disorders result in a decrease in the quality of life of patients [5, 6] and in an economic burden [1]. There is a need for investigation of posture disorders and their underlying reasons, such as muscle stiffness [17, 18]. The primary aim of this study was to investigate the possible differences in sagittal-frontal posture during upright standing and stiffness of superficial postural muscles in adolescent male HP. The secondary purpose of the study was to determine the effects of muscle stiffness and joint health on posture. We hypothesized that upright standing posture and stiffness of the superficial postural muscles are altered in HP compared to age-matched healthy peers.
2.Materials and methods
2.1Study design and participants
This study used an observational and comparative design. The criteria for Strengthening the Reporting of Observational Studies in Epidemiology (STROBE) checklist were considered for reporting the study. This study was conducted between September 2021 and February 2022 at the Physical Medicine and Rehabilitation Outpatient Clinic of Cukurova University. The study was approved by the Non-Interventional Clinical Research Ethics Committee of Cukurova University (Decision No: 109/61, Approval Date: 05.03.2021) and the study protocol was registered in the clinicaltrials.gov database (ID: NCT05173129). All participants and their legal representatives were given written and verbal information before the study and a written consent was obtained. All procedures were conducted in accord with the Declaration of Helsinki (as revised in 2013).
HP recruited from the Cukurova Hemophilia Society were assigned to the haemophilia group, while randomly selected healthy adolescent boys from classmates of HP were assigned to the control group. HP were randomly enrolled in the study using computer generated numbers (randomizer org; available at https://www.randomizer.org/#randomize). Each patient of the Cukurova Hemophilia Society was numbered, and these numbers were entered into the computer program. Patients were invited to participate in the study according to a random order formed by the computer program until the power analysis-determined sample size was reached.
The study included adolescent boys diagnosed with severe-moderate haemophilia A or B and aged between 10 and 19 years. HP who were unable to stand without support due to haemophilic arthropathy, positive for FVIII or FIX inhibitor, had bleeding events in the previous two weeks, previous surgeries such as synovectomy or spine surgeries, spine diseases such as Scheuermann’s disease, and with mild haemophilia were excluded. In addition, boys diagnosed with a chronic musculoskeletal disease by an independent physician were excluded from the control group.
2.2Outcome measures
All participants were evaluated in different days between 9:00 and 10:00 am at local time. Sociodemographic and physical characteristics (age, height, weight, body mass index and educational status) of all participants were recorded. Type and severity of haemophilia, number of the target joints, treatment type, and joint health of the participants in the hemophilia group were also evaluated. Haemophilia Joint Health Score version 2.1 (HJHS) was used to assess the joint health. The HJHS which has high internal reliability (Cronbach’s
2.3Photogrammetry
This method is based on the analysis of two-dimensional (2D) photographic images of the whole body or alignment of the body segments using computer software [22]. Photogrammetry showed good-excellent interrater-reliability (interclass correlation coefficients: 0.84–0.99) for posture analysis of the pediatric population [23].
The data obtained in the measurement may vary depending on the type of software, camera height, participant-camera distance, and reference points [24]. We preferred the protocol developed by Belli et al. for the photogrammetry method [25]. Firstly, cylindrical type passive markers with a 0.5 cm2 surface area were attached to some anatomical reference points (processus mastoideus, acromion, superior angle of scapula, tuberculum majus, third-seventh cervical, first-seventh-twelfth thoracic, first-third-fifth lumbal vertebrae, spina iliaca anterior superior, spina iliaca posterior superior, trochanter major, lateral condyle of the femur, midpoint of the patella, lateral malleolus, midpoint of the ankle, and distal end of the fifth metatarsal bone) on the body of the participant wearing only tight-fitting sports short, using double-sided adhesive tape by an experienced physiotherapist. Furthermore, in order to form vertebral angles, a virtual straight line (external reference line) 33 cm posterior to the participant was drawn by software. The participant asked to stand on a fixed platform (5 cm high
Table 1
The posture angles formed with Kinovea® using landmarks [25]
Angle | How the angle was formed? |
---|---|
Sagittal plane | |
Craniovertebral angle | The angle was formed by connecting the sagittal straight line drawn parallel to the ground and the straight line that passed through the mastoid process and C7. |
Cervical lordosis angle | The angle was formed by connecting the straight line passed through the distal point of the occiput and C3 level point on the external reference line and the straight line passed through the C7 and C3 level point on the external reference line. |
Shoulder protraction angle | The angle was formed by connecting the sagittal straight line drawn parallel to the ground and the straight line that passed through the acromion and superior angle of scapula. |
Thoracic kyphosis angle | The angle was formed by connecting the straight line passed through the T1 and T6 level point on the external reference line and the straight line passed through the T12 and T6 level point on the external reference line. |
Lumbal lordosis angle | The angle was formed by connecting the straight line passed through the L1 and L3 level point on the external reference line and the straight line passed through the L5 and L3 level point on the external reference line. |
Sagittal pelvic tilt angle | The angle was formed by connecting the sagittal straight line drawn parallel to the ground and the straight line that passed through the SIAS-SIPS. |
Knee flexion angle | The angle was formed by connecting the straight line that passed through the trochanter major-lateral condyle of the femur and the straight line that passed through the lateral condyle of the femurr-lateral malleol. |
Ankle plantar flexion angle | The angle was formed by connecting the straight line that passed through the lateral condyle of femur – lateral malleol and the straight line that passed through the lateral lateral malleol-distal end of the fifth metatarsal bone. |
Frontal plane | |
Shoulder asymmetry angle | The angle was formed by connecting the frontal straight line drawn parallel to the ground and the straight line that passed through the left-right tuberculum majus. |
Pelvis asymmetry angle | The angle was formed by connecting the frontal straight line drawn parallel to the ground and the straight line that passed through the left-right SIASs. |
Knee valgus angle | The angle was formed by connecting the straight line that passed through the SIAS-midpoint of the patella and the straight line that passed through the midpoint of the patella- midpoint of the ankle. |
Abbreviations: C3, Third cervical vertebra; C7, Seventh cervical vertebra; T1, First thoracic vertebra; T6, Sixth thoracic vertebra; T12, Twelfth thoracic vertebra; L1, First lumbar vertebra; L3, Third lumbar vertebra; L5, Fifth lumbar vertebra; SIAS, Spina iliaca anterior superior; SIPS, Spina ilica posterior superior.
Figure 1.
Images taken with admission for analysis of posture parameters with Kinovea®; a) Spinopelvic posture angles in sagittal plane; grey angle for craniovertebral, red angle for cervical lordosis, yellow angle for thoracic kyphosis, green angle for lumbal lordosis, and blue angle for sagittal pelvic tilt; b) Extremity posture angles in sagittal plane; khaki angle for shoulder protraction, dark blue angle for knee flexion, and dark orange angle for ankle plantar flexion [calculated by the formula (angle obtained with Kinovea®)–90]; c) Posture angles in frontal plane; dark green angle for shoulder asymmetry, yellow angle for pelvis asymmetry, and pink angle for knee valgus.
![Images taken with admission for analysis of posture parameters with Kinovea®; a) Spinopelvic posture angles in sagittal plane; grey angle for craniovertebral, red angle for cervical lordosis, yellow angle for thoracic kyphosis, green angle for lumbal lordosis, and blue angle for sagittal pelvic tilt; b) Extremity posture angles in sagittal plane; khaki angle for shoulder protraction, dark blue angle for knee flexion, and dark orange angle for ankle plantar flexion [calculated by the formula (angle obtained with Kinovea®)–90]; c) Posture angles in frontal plane; dark green angle for shoulder asymmetry, yellow angle for pelvis asymmetry, and pink angle for knee valgus.](https://content.iospress.com:443/media/bmr/2024/37-3/bmr-37-3-bmr230185/bmr-37-bmr230185-g001.jpg)
The 2D images were analyzed using the Kinovea® video analysis (version 0.8.15, Kinovea Open Source Project), which measures the angles formed by the lines traced from the previously labeled anatomic landmarks in order to identify and quantify the possible asymmetries and vertebral curvature angles. Eleven posture angles were obtained on the sagittal (craniovertebral, cervical lordosis, shoulder protraction, thoracic kyphosis, lumbar lordosis, pelvic tilt, knee flexion, and ankle plantar flexion angles) and frontal (shoulder asymmetry, pelvis asymmetry, and knee valgus angles) planes. Measurements were performed three times for each angle and the average value was recorded. To reduce the risk of bias for intra-rater analyses, three measurements were made one day apart on the same image. The angles obtained with Kinovea® using landmarks are summarized in Table 1 and shown in Fig. 1.
2.4Myotonometry
Myotonometry is based on MyotonPro® (Myoton AS, Tallinn, Estonia) a hand-held device that provides objective data regarding stiffness of superficial muscles and soft tissues. It is a reliable method for assessment of muscle stiffness in children (interrater reliabilities ranged from 0.74 to 0.99) [26].
MyotonPro® displays the myofascial stiffness by analyzing the oscillations of the myofascial tissue in response to the 5 short (15 ms) impulses, at 0.56 N force and frequency of 1 Hz applied by the probe of the device [12]. The device simultaneously calculates the stiffness by the manufacturer’s formula as follows:
(S: Stiffness, a1max: The maximum acceleration in the oscillatory wave occurred in the muscle, m: Probe mass,
The superficial muscles (cervical, thoracic and lumbar erector spinae, upper and middle trapezius, sternocleidomastoideum, pectoralis major, rectus abdominus, gluteus medius, adductor magnus, rectus femoris, biceps femoris, tibialis anterior, and gastriconemius), which play a role in the posture were evaluated [28]. Cervical, thoracic and lumbar erector spinae, middle trapezius, gluteus medius, rectus femoris, and tibialis anterior muscles were agonist, whereas upper trapezius, sternocleidomastoideum, pectoralis major, rectus abdominus, adductor magnus, biceps femoris, and gastriconemius were antagonist muscles for upright standing posture [29].
The participants were asked not to do moderate or vigorous physical activity before the measurement. Data were obtained during upright standing position and from the side (left or right) where sagittal posture analysis was performed. The probe of the MyotonPRO® device was placed vertically to the muscle fibers on the belly (previously marked by an experienced physiotherapist) of the muscle and three measurements were made for each muscle. Measurement was accepted as valid if there is less than a 5% variation between three taps [30]. The average of the three measurements was recorded on a data collection form. A higher value (N/m) indicates higher muscle stiffness [12, 16, 26].
2.5Statistical analysis
Statistical analyses were carried out using the Statistical Package for Social Sciences version 22.0 (SPSS Inc., Chicago, IL, USA). The distribution of the data was determined by visual (histograms and probability plots) and analytical methods (Shapiro-Wilks test). Normally distributed data were expressed as mean and standard deviation (SD), and categorical data as number and percent (%). Photogrammetry and myotonometry data were analyzed using unpaired
The intra-class correlation coefficient (ICC) was used to determine the reproducibility of the photogrammetry method. ICC values:
The required sample size was calculated using the G*Power Software (version 3.0.18; Heinrich-Heine-Universität Düsseldorf, Germany). The ‘t tests-difference between two independent means’ parameter was selected by accepting posture measurement as the primary assessment method. Based on the study performed by Hmida et al. [14] the effect size was determined at 0.88. We estimated a sample size of 22 participants for each group and 44 participants in total with a statistical power of 80% (1
3.Results
A total of 184 HP was enrolled to the study and all data of the 22 patients who met the inclusion criteria and 22 control subjects were analyzed (Fig. 2). No participant reported bleeding during assessments.
Table 2
The demographic characteristics of all subjects and the clinical characteristics of the haemophilia group
Haemophilia group ( | Control group ( | ||
---|---|---|---|
Age; (year) (mean | 16.4 | 16.0 | 0.577† |
Height; (cm) (mean | 170.6 | 166.6 | 0.269† |
Weight; (kg) (mean | 63.4 | 61.8 | 0.737† |
BMI; (kg/m2), (mean | 21.6 | 22.0 | 0.732† |
Education; | |||
Middle school High school University | 4 (18) 13 (59) 5 (23) | 6 (27) 11 (50) 5 (23) | 0.546‡ |
HJHS; (mean | |||
Elbow Knee Elbow Total | 1.3 | – | – |
Type of haemophilia; | |||
A/B | 20 (91)/2 (9) | – | – |
Severity; | |||
Severe/Moderate | 17 (77)/5 (23) | – | – |
Factor treatment; | |||
Prophylaxis/On-demand | 15 (68)/7 (32) | – | – |
Number of target joint; | |||
0 1 2 | 10 (45) 7 (32) 4 (18) 1 (5) | – | – |
Most affected joint | |||
Knee Ankle Elbow None | 7 (32) 3 (14) 2 (9) 10 (45) | – | – |
Abbreviations: sd, Standard deviation; min, Minimum; max, Maximum; BMI, Body mass index; HJHS, Haemophilia joint health score, †: Unpaired
Figure 2.
Study flowchart.
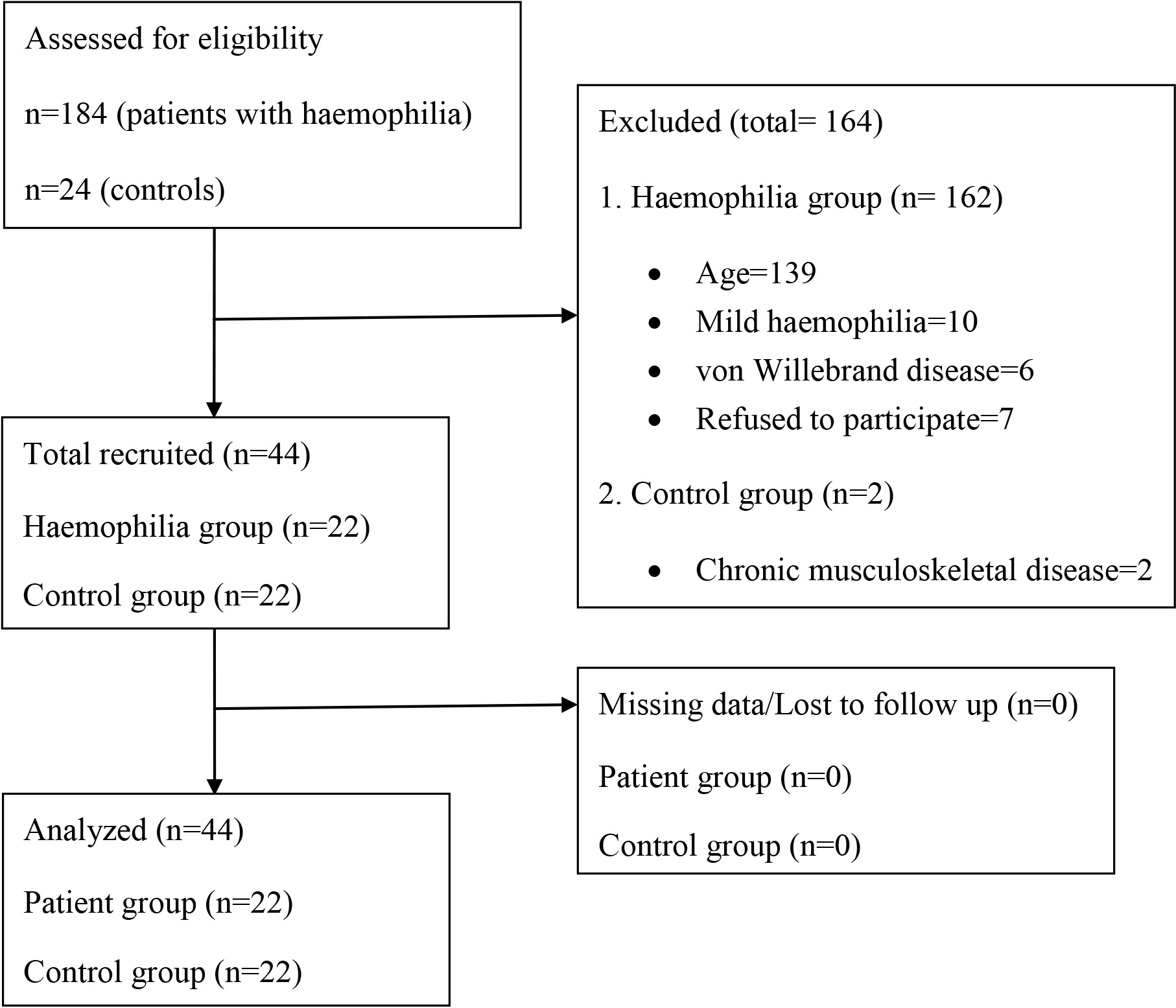
There was no significant difference between the groups in sociodemographic characteristics (
Table 3
Between-group comparison of the posture angles
Angle (degree) | Haemophilia group ( | Control group ( | Mean difference 95% (CI) | Effect size (Cohen d) | |
---|---|---|---|---|---|
Cervical lordosis angle | 17.5 | 19.2 | – | 0.064 | |
Craniovertebral angle | 50.4 | 61.5 | 1.47 | ||
Thoracic kyphosis angle | 53.7 | 53.1 | 0.6 ( | – | 0.785 |
Shoulder protraction angle | 35.1 | 39.8 |
| 0.076 | |
Shoulder asymmetry angle | 1.1 | 1.3 | – | 0.767 | |
Lumbar lordosis angle | 30.0 | 26.8 | 3.2 (0.5 to 5.9) | 0.71 | 0.021 |
Sagittal pelvic tilt angle | 15.6 | 15.2 | 0.4 ( | – | 0.462 |
Pelvic asymmetry angle | 1.5 | 1.2 | 0.3 ( | – | 0.729 |
Knee flexion angle | 173.3 | 179.1 | 1.20 | ||
Knee valgus angle | 175.9 | 179.8 | 1.19 | 0.001 | |
Ankle plantar flexion angle | 12.0 | 8.6 | 3.4 (0.2 to 6.5) | 0.64 | 0.043 |
Abbreviations: sd, Standard deviation; min, Minimum; max, Maximum, †: Unpaired
Regarding the reproducibility of the photogrammetry method, the 2D images of the whole body on the frontal and sagittal planes showed moderate to excellent mean ICC(s) (0.68 to 0.97) for all angles. During the upright standing posture measurement, craniovertebral, knee flexion-valgus angles were significantly lower; however, lumbar lordosis and ankle plantar flexion angles were significantly higher in the haemophilia group compared to the control group (
Table 4
Between-group comparison of the muscle stiffness
Muscle (N/m) | Haemophilia group ( | Control group ( | Mean difference 95% (CI) | Effect size (Cohen d) | |
---|---|---|---|---|---|
Cervical erector spinae | 288.9 | 304.3 | – | 0.444 | |
Sternocleidomastoideum | 200.2 | 192.4 | 7.8 ( | – | 0.514 |
Upper trapezius | 336.7 | 323.9 | 12.9 ( | – | 0.482 |
Middle trapezius | 261.0 | 282.2 | – | 0.179 | |
Thoracic erector spinae | 326.8 | 316.3 | 10.5 ( | – | 0.563 |
Pectoralis major | 274.1 | 262.1 | 12.0 ( | – | 0.653 |
Lumbar erector spinae | 269.2 | 301.4 | – | 0.186 | |
Rectus abdominus | 244.5 | 228.0 | 16.5 ( | – | 0.367 |
Gluteus medius | 246.3 | 259.4 | – | 0.508 | |
Adductor magnus | 269.3 | 254.6 | 14.7 ( | – | 0.185 |
Rectus femoris | 245.3 | 287.5 | 0.72 | ||
Biceps femoris | 287.9 | 267.8 | 20.2 ( | – | 0.192 |
Tibialis anterior | 602.3 | 616.8 | – | 0.656 | |
Gastriconemius | 383.9 | 408.3 | – | 0.250 |
Abbreviations: N/m, Newton/meter; sd, Standard deviation; min, Minimum; max, Maximum, †: Unpaired
The stiffness of the rectus femoris was significantly lower with a medium effect size (
The stepwise multiple regression analysis demonstrated that there was no association between potential confounders and the significant difference in the dependent variables (
4.Discussion
This study aimed to compare upright standing posture and muscle stiffness between HP and age-matched healthy peers. It showed an increased forward tilt of the head, reduced lumbar lordosis curvature, a misalignment in knee flexion valgus and ankle plantar flexion in HP. Stiffness of the rectus femoris muscle was lower in the HP than in the controls Deterioration of joint health and the reduction in rectus femoris stiffness could cause differences in craniocervical and knee flexion-valgus angles. Worsening joint health causes a change in ankle posture in the form of plantar flexion.
Table 5
Linear regression analyses of rectus femoris stiffness and haemophilia joint health score with posture angles
Craniovertebral angle | Lumbar lordosis angle | Knee flexion angle | Knee valgus angle | Ankle plantar flexion angle | |||||||||||
---|---|---|---|---|---|---|---|---|---|---|---|---|---|---|---|
B(SE) | ß |
| B(SE) | ß |
| B(SE) | ß |
| B(SE) | ß |
| B(SE) | ß |
| |
Model 1 | |||||||||||||||
RF stiffness | 0.09 (0.02) | 0.54 | 0.001 | 0.396 | 0.02 (0.01) | 0.03 | 0.045 | 0.02 (0.01) | 0.04 | 0.007 | 0.483 | ||||
Model 2 | |||||||||||||||
RF stiffness | 0.09 (0.02) | 0.54 | 0.001 | 0.396 | 0.02 (0.01) | 0.03 | 0.045 | 0.02 (0.01) | 0.04 | 0.005 | 0.475 | ||||
HJHS total | 0.048 | 0.154 | 0.004 | 0.041 | 0.034 | ||||||||||
Note: | R2= 0.29 for model 1 | R2= 0.02 for model 1 | R2= 0.12 for model 1 | R2= 0.16 for model 1 | R2= 0.01 for model 1 | ||||||||||
Abbreviations: B, unstandardized coefficients; SE, standard error;
It is widely known that joint degeneration, muscular imbalance and proprioceptive disorders that are seen in musculoskeletal diseases give rise to various changes in static erect posture [8, 9]. A guideline published for managing musculoskeletal complications of haemophilia reported that HP often have postural changes characterized by flexion deformities in the extremities [34]. Similarly, Boccalandro et al. found alterations in static upright posture in pediatric HP and reported that balance was adversely affected due to postural changes [11]. Surprisingly, a recent study found no significant change in spinal posture in adult HP (with a relatively high level of joint damage; mean HJHS total of 23.5 points) compared to their healthy peers [14]. The misalignment in the lower extremity in our study is consistent with the haemophilia guideline. In addition, similarly to Boccalandro et al. [11], we determined misalignment in spinal segments. From a biomechanical perspective, the joints of the lower extremities serve as a basis for spine posture [35]. Therefore, we hypothesized that recurrent bleeding in the knee and ankle joints results in significant changes in the alignment of the joints and that this may be a reason for altered spinal posture in HP.
Changes in the viscoelastic properties of the muscle affect joint mobility, muscle flexibility, posture, and functionality [12, 36]. The literature has studies addressing the change in the viscoelastic properties of muscles in people with neuromuscular and orthopedic diseases or in healthy individuals in [12, 17, 36]. Chleboun et al. found a positive correlation between muscle volume and passive muscle stiffness [37]. Considering this finding, muscle atrophy is likely associated with a decrease in passive stiffness. In contrast, a previous study found increased passive stiffness of the calf muscles in patients with haemophilic ankle arthropathy [38]. Muscle atrophy is a typical symptom of haemophilic arthropathy [1]. Researchers have hypothesized that fibrosis of periarticular tissues due to repeated joint bleeding may increase muscle stiffness [38]. In our study, like that of Chleboun et al. [37], we found a significant decrease in stiffness of the rectus femoris muscle, which controls the knee. The change in stiffness of the rectus femoris muscle can be explained by arthrogenic muscle inhibition [39]. Knee hemarthrosis triggers a reflex inhibition mechanism that reduces the quadriceps activity, thus diminishing tone and stiffness [39]. No significant change was determined in the stiffness of the superficial spinal muscles in HP. This finding suggests that the stiffness of the superficial spinal muscles does not play a key role in the changes in spinal posture in HP.
4.1Limitations
The present study has some limitations regarding data collection. First of all, stiffness of the deep muscles affecting the posture, especially the iliopsoas muscle, which is known to bleed frequently in HP [40], cannot be measured with MyotonPRO® device [27]. Therefore, we were unable to determine the changes in the stiffness of the deep postural muscles. The second limitation is that we did not use other advanced techniques, such as ultrasound imaging or magnetic resonance elastography, to determine whether differences in fat infiltration/densification and fibrosis of superficial muscles contributed to the observed variability of muscle stiffness [12]. Third, since the frontal plane alignment of the spine cannot be assessed using the photogrammetry method [41], we could not perform a postural analysis from the posterior side of the participant.
5.Conclusion
In adolescent HP, hemarthrosis can lead to misalignment in the ankle, knee, lumbar, and craniovertebral segments and a decrease in the stiffness of the rectus femoris muscle. Posture assessment should be part of the examination in musculoskeletal complications of haemophilia, and any change in the stiffness of the rectus femoris should also be considered in the management of postural disorders in HP. Approaches to improve joint health and rectus femoris muscle stiffness may be among the methods that can be used by clinicians to prevent postural disorders in HP.
Author contributions
Volkan Deniz: Conceptualization, data curation, formal analysis, supervision, writing, and editing. Nevin Atalay Guzel: Data collection, data analysis, and writing. Bayram Kelle: Data curation, supervision, writing-review, and editing. Ali Bulent Antmen: Data curation, supervision, writing-review, and editing.
Data availability statement
The data supporting the findings of the present study are available from the corresponding author upon reasonable request.
Ethical approval
The study was conducted in accordance with the Declaration of Helsinki (as revised in 2013) and approved by the Non-Interventional Clinical Research Ethics Committee of Cukurova University (Decision No: 109/61, Approval Date: 05.03.2021).
Funding
The authors report no funding.
Informed consent
All participants and their legal representatives provided signed informed consent for their inclusion in the research.
Acknowledgments
We want to thank Mr. Ali Ekber Daghan, manager of Cukurova Hemophilia Society, for his support in the recruitment process of patients.
Conflict of interest
The authors declare that they have no conflict of interest.
References
[1] | Srivastava A, Brewer AK, Mauser-Bunschoten EP, et al. Treatment Guidelines Working Group on Behalf of The World Federation of Hemophilia. Guidelines for the management of hemophilia. Haemophilia. (2013) Jan; 19: (1): e1-47. doi: 10.1111/j.1365-2516.2012.02909.x. |
[2] | Tat NM, Tat AM, Oner AF, et al. Static postural balance evaluation and an investigation of the relationship with joint health in children with severe haemophilia: a controlled cross-sectional study. Haemophilia. (2021) Mar; 27: (2): e245-e252. doi: 10.1111/hae.14240. |
[3] | Fouasson-Chailloux A, Maugars Y, Vinatier C, et al. Clinical relevance of 3D gait analysis in patients with haemophilia. Haemophilia. (2018) ; 24: (5): 703-710. doi: 10.1111/hae.13563. |
[4] | Deniz V, Guzel NA, Lobet S, et al. Effects of a supervised therapeutic exercise program on musculoskeletal health and gait in patients with haemophilia: A pilot study. Haemophilia. (2022) Jan; 28: (1): 166-175. doi: 10.1111/hae.14444. |
[5] | Deniz V, Guzel NA. Do therapeutic exercises improve kinesophobia and health-related quality of life in adult hemophilia patients? A randomized controlled trial. International Journal of Disabilities Sports and Health Sciences. (2020) ; 3: (1): 11-19. doi: 10.33438/ijdshs.690280. |
[6] | Taha MY, Hassan MK. Health-related quality of life in children and adolescents with hemophilia in Basra, Southern Iraq. J Pediatr Hematol Oncol. (2014) Apr; 36: (3): 179-84. doi: 10.1097/MPH.0000000000000117. |
[7] | Woollacott M, Shumway-Cook A. Attention and the control of posture and gait: a review of an emerging area of research. Gait Posture. (2002) Aug; 16: (1): 1-14. doi: 10.1016/s0966-6362(01)00156-4. |
[8] | Al-Bayati Z, Coskun Benlidayi I, Gokcen N. Posture of the foot: Don’t keep it out of sight, out of mind in knee osteoarthritis. Gait Posture. (2018) Oct; 66: : 130-134. doi: 10.1016/j.gaitpost.2018.08.036. |
[9] | Saito ET, Akashi PM, Sacco Ide C. Global body posture evaluation in patients with temporomandibular joint disorder. Clinics (Sao Paulo). (2009) ; 64: (1): 35-9. doi: 10.1590/s1807-59322009000100007. |
[10] | Araújo FA, Simões D, Silva P, et al. Sagittal standing posture and relationships with anthropometrics and body composition during childhood. Gait Posture. (2019) Sep; 73: : 45-51. doi: 10.1016/j.gaitpost.2019.07.130. |
[11] | Boccalandro E, Pasta G, Mannucci PM, et al. Integrated postural analysis in children with haemophilia. Haemophilia. (2014) Mar; 20: (2): 263-7. doi: 10.1111/hae.12369. |
[12] | Kocur P, Tomczak M, Wiernicka M, et al. Relationship between age, BMI, head posture and superficial neck muscle stiffness and elasticity in adult women. Sci Rep. (2019) Jun 11; 9: (1): 8515. doi: 10.1038/s41598-019-44837-5. |
[13] | Jain R, Meena ML, Sain MK, Dangayach GS. Impact of posture and upper-limb muscle activity on grip strength. Int J Occup Saf Ergon. (2019) Dec; 25: (4): 614-620. doi: 10.1080/. |
[14] | Hmida J, Hilberg T, Krüger S, et al. Changes of static and dynamic spine alignment in patients with severe haemophilia. Haemophilia. (2021) Nov; 27: (6): e721-e729. doi: 10.1111/hae.14406. |
[15] | Levi D, Springer S, Parmet Y, Ovadia D, Ben-Sira D. Acute muscle stretching and the ability to maintain posture in females with adolescent idiopathic scoliosis. J Back Musculoskelet Rehabil. (2019) ; 32: (4): 655-662. doi: 10.3233/BMR-181175. |
[16] | Lohr C, Braumann KM, Reer R, et al. Reliability of tensiomyography and myotonometry in detecting mechanical and contractile characteristics of the lumbar erector spinae in healthy volunteers. Eur J Appl Physiol. (2018) ; 118: (7): 1349-59. doi: 10.1007/s00421-018-3867-2. |
[17] | Li Y, Yu J, Zhang J, et al. Quantifying the stiffness of lumbar erector spinae during different positions among participants with chronic low back pain. PLoS ONE. (2022) ; 17: (6): e0270286. doi: 10.1371/journal.pone.0270286. |
[18] | Creze M, Bedretdinova D, Soubeyrand M, et al. Posture-related stiffness mapping of paraspinal muscles. J Anat. (2019) Jun; 234: (6): 787-799. doi: 10.1111/joa.12978. |
[19] | Barrett JM, McKinnon C, Callaghan JP. Cervical spine joint loading with neck flexion. Ergonomics. (2020) Jan; 63: (1): 101-108. doi: 10.1080/00140139.2019.1677944. |
[20] | St-Louis J, Abad A, Funk S, Tilak M, Classey S, Zourikian N, McLaughlin P, Lobet S, Hernandez G, Akins S, Wells AJ, Manco-Johnson M, John J, Austin S, Chowdhary P, Hermans C, Nugent D, Bakeer N, Mangles S, Hilliard P, Blanchette VS, Feldman BM. The Hemophilia Joint Health Score version 21. Validation in Adult Patients Study: A multicenter international study. Res Pract Thromb Haemost. (2022) Mar 25; 6: (2): e12690. doi: 10.1002/rth2.12690. |
[21] | Feldman BM, Funk SM, Bergstrom B-M, et al. Validation of a new pediatric joint scoring system from the International Hemophilia Prophylaxis Study Group: validity of the hemophilia joint health score. Arthritis Care Res (Hoboken). (2011) ; 63: (2): 223-230. doi: 10.1002/acr.20353. |
[22] | Singla D, Veqar Z. Methods of postural assessment used for sports persons. J Clin Diagn Res. (2014) Apr; 8: (4): LE01-4. doi: 10.7860/JCDR/2014/6836.4266. |
[23] | Santos MM, Silva MPC, Sanada LS, et al. Photogrammetric postural analysis on healthy seven to ten-year-old children:interrater reliability. Rev Bras Fisioter. (2009) ; 13: (4): 350-5. doi: 10.1590/S1413-35552009005000047. |
[24] | Singla D, Veqar Z, Hussain ME. photogrammetric assessment of upper body posture using postural angles: A literature review. J Chiropr Med. (2017) Jun; 16: (2): 131-138. doi: 10.1016/j.jcm.2017.01.005. |
[25] | Belli JF, Chaves TC, de Oliveira AS, et al. Analysis of body posture in children with mild to moderate asthma. Eur J Pediatr. (2009) Oct; 168: (10): 1207-16. doi: 10.1007/s00431-008-0911-y. |
[26] | Aarrestad DD, Williams MD, Fehrer SC, et al. Intra- and interrater reliabilities of the Myotonometer when assessing the spastic condition of children with cerebral palsy. J Child Neurol. (2004) Nov; 19: (11): 894-901. doi: 10.1177/08830738040190110801. |
[27] | Myoton PRO: Methods of measurement.Tallinn, Estonia [Updated 2016; Cited Feb 23, 2022]. Available from: www.myoton.com/technology. |
[28] | Kuo AD, Zajac FE. A biomechanical analysis of muscle strength as a limiting factor in standing posture. J Biomech. (1993) ; 26: (1): 137-50. doi: 10.1016/0021-9290(93)90085-s. |
[29] | Chiba R, Takakusaki K, Ota J, et al. Human upright posture control models based on multisensory inputs; in fast and slow dynamics. Neuroscience research. (2016) Mar 1; 104: : 96-104. doi: 10.1016/j.neures.2015.12.002. |
[30] | Llurda-Almuzara L, Pérez-Bellmunt A, López-de-Celis C, et al. Normative data and correlation between dynamic knee valgus and neuromuscular response among healthy active males: a cross-sectional study. Sci Rep. (2020) Dec 2; 10: (1): 17206. doi: 10.1038/s41598-020-74177-8. |
[31] | Ferreira JC, Patino CM. Subgroup analysis and interaction tests: why they are important and how to avoid common mistakes. J Bras Pneumol. (2017) May-Jun; 43: (3): 162. doi: 10.1590/S1806-37562017000000170. |
[32] | Koo TK, Li MY. A guideline of selecting and reporting intraclass correlation coefficients for reliability research. J Chiropr Med. (2016) ; 15: (2): 155-63. doi: 10.1016/j.jcm.2016.02.012. |
[33] | Miranda APOC, Penha PJ, Pereira LG, et al. Influence of Sex and Body Mass Index on the Thoracic Kyphosis and Lumbar Lordosis. J Manipulative Physiol Ther. (2022) Sep; 45: (7): 508-514. doi: 10.1016/j.jmpt.2022.12.001. |
[34] | Forsyth A, Blamey G, Lobet S, et al. Practical Guidance for Non-Specialist Physical Therapists Managing People with Hemophilia and Musculoskeletal Complications. Health. (2020) ; 12: : 158-179. doi: 10.4236/health.2020.122014. |
[35] | McGregor AH, Hukins DWL. Lower limb involvement in spinal function and low back pain. J Back Musculoskelet Rehabil. (2009) ; 22: (4): 219-222. doi: 10.3233/BMR-2009-0239. |
[36] | Gustafson JA, Anderton W, Sowa GA, et al. Dynamic knee joint stiffness and contralateral knee joint loading during prolonged walking in patients with unilateral knee osteoarthritis. Gait Posture. (2019) Feb; 68: : 44-49. doi: 10.1016/j.gaitpost.2018.10.032. |
[37] | Chleboun GS, Howell JN, Conatser RR, Giesey JJ. The relationship between elbow flexor volume and angular stiffness at the elbow. Clin Biomech (Bristol, Avon). (1997) Sep; 12: (6): 383-392. doi: 10.1016/s0268-0033(97)00027-2. |
[38] | Lobet S, Cartiaux O, Peerlinck K, Henrard S, Hermans C, Detrembleur C, Deschamps K. Assessment of passive musculoarticular ankle stiffness in children, adolescents and young adults with haemophilic ankle arthropathy. Haemophilia. (2018) May; 24: (3): e103-e112. doi: 10.1111/hae.13467. |
[39] | Rice DA, McNair PJ. Quadriceps arthrogenic muscle inhibition: neural mechanisms and treatment perspectives. Semin Arthritis Rheum. (2010) Dec; 40: (3): 250-66. doi: 10.1016/j.semarthrit.2009.10.001. |
[40] | Kaçar D, Ergun O, Kara A, et al. Percutaneous Drainage of an Iliopsoas Hematoma in a Hemophilia Patient. J Pediatr Hematol Oncol. (2020) Oct; 42: (7): e683-e685. doi: 10.1097/MPH.0000000000001503. |
[41] | Penha PJ, Penha NLJ, De Carvalho BKG, et al. Posture Alignment of Adolescent Idiopathic Scoliosis: Photogrammetry in Scoliosis School Screening. J Manipulative Physiol Ther. (2017) Jul-Aug; 40: (6): 441-451. doi: 10.1016/j.jmpt.2017.03.013. |