Comparison between percutaneous short-segment fixation and percutaneous vertebroplasty in treating Kummell’s disease: A minimum 2-year follow-up retrospective study
Abstract
BACKGROUND:
Percutaneous kyphoplasty (PKP) or percutaneous short-segment fixation (PSSF) is often used to treat Kummell’s disease. However, it is not clear which treatment is better for patients.
OBJECTIVE:
To retrospectively compare the clinical efficacy of PVP and PSSF for the treatment of Kummell’s disease.
METHOD:
60 patients were involved in this research and the period of follow-up was at least 2 years. 27 of them were treated with PVP (Group I) and the rest who received PSSF (Group II). The visual analog scale (VAS) and radiographic indexes of each participant had been measured preoperatively as well as 1 week, 3 months, and 2 years postoperatively. Additionally, the Oswestry Disability Index (ODI) scores were assessed at the last time point.
RESULTS:
Comparing the two groups, no statistical significance was found among all parameters preoperatively. The time of operations and blood loss is less in Group I. At each time point after operation, the imaging indices in Group II are lower (
CONCLUSION:
For the treatment of Kummell’s disease, both PVP and PSSF have been found to be effective. PVP can provide rapid pain relief with a shorter operation time. However, in cases with severe kyphosis deformity, PSSF should be given priority.
1.Introduction
With the increasing proportion of the elderly individuals in the population, osteoporosis h has become a common condition worldwide [1, 2]. One of the complications of this disease is fragility compression fractures, with osteoporotic vertebral compression fractures (OVCFs) being the most prevalent type [3, 4]. Studies have shown that approximately 30% of OVCF cases do not respond adequately to standard conservative therapy [5, 6, 7, 8]. Subsequently, nonunion can be observed in 13.5% of the patients, and the presence of an intravertebral cleft (IVC) sign is reported in 7–13% of OVCF cases [9, 10, 11]. In addition, the prognosis for OVCFs with an intravertebral cleft (IVC) has been reported to be associated with Kummell’s disease (KD), which manifests as posttraumatic delayed vertebral collapses [5, 9].
KD is considered one complication and the final stage of OVCFs by Lim et al., since hyperflexion could also result in OVCFs and IVC might be seen in the chronic fractures [9, 14]. Some authors suggested that KD and OVCFs are two separate diseases for their different injury mechanisms and imaging characteristics [9, 12, 13]. Libicher et al. claimed that the appearance of the IVC sign specifically manifests the occurrence of osteonecrosis in vertebral compression fractures [14], although the relation between the IVC sign and KD is not clear.
According to previous analyses of the reasons for delayed healing or nonhealing in cases of osteonecrosis, blood supply plays a crucial role. Damage to blood vessels, either anatomical or mechanical, or pathology affecting the vessels, such as atherosclerosis or fat microembolism blockage caused by secondary fractures, can lead to compromised blood flow to the vertebral body [15]. This delay in neovascularization of the fractured vertebral body can ultimately result in nonunion or delayed healing of osteonecrosis. Moreover, factors such as Schmorl’s node, normal stress, lack of osteoblast, and low bone mineral density have also been identified as relevant contributors to nonhealing [16, 17].
There are multiple treatment options available for Kummell’s disease. Conservative therapy, including bed rest, bracing, nonsteroidal anti-inflammatory drug medication, and orthosis, is considered essential [18, 10]. For cases with kyphotic deformity, intractable pain, or neurologic deficits, aggressive surgical interventions are recommended [18, 10]. Among the surgical choices, percutaneous short-segment fixation (PSSF) and percutaneous vertebroplasty (PVP) have been widely accepted and utilized. PSSF is becoming increasingly popular due to its reduced surgical trauma [19]. Additionally, PVP has shown satisfactory clinical outcomes with less injury and significant pain relief in treating KD, although a consensus has not been reached yet. However, there is limited research on whether to choose PVP or PSSF for the treatment of KD. Our study aims to compare these two surgical approaches for KD, providing new insights into the treatment options for this condition.
Table 1
The fracture levels in both groups and follow-up situations
Items | PVP GI ( | PSSF GII ( | |
---|---|---|---|
Level | T7 | 3 | 1 |
T8 | 2 | 2 | |
T9 | 3 | 2 | |
T10 | 6 | 6 | |
T11 | 11 | 12 | |
T12 | 7 | 7 | |
L1 | 4 | 4 | |
L2 | 4 | 3 | |
L3 | 3 | 2 | |
L4 | 4 | 1 | |
L5 | 2 | 3 | |
Total | 49 | 43 | |
Death | 3 | 1 | |
Patients lost to follow-up | 19 | 9 | |
Final involved patients | 27 | 33 |
Note: 1. Fracture levels had been recorded. 2. The incidence rate of complications were calculated with the number of patients with complications divided by those received operations. 3. The final involved patients were those who finished complete 2-year follow-up. PVP GI: Percutaneous vertebroplasty Group I; PSSF GII: Percutaneous short-segment fixation Group II.
Table 2
The clinical features of patients involved
Items | PVP GI ( | PSSF GII ( | |
---|---|---|---|
ASA classification | |||
I | 6 | ||
II | 27 | ||
III | 20 | ||
IV | 7 | ||
V | 0 | ||
VI | 0 | ||
Sex | |||
Male | 12 | 12 | 1.00 |
Female | 15 | 21 | |
Age (Years) | 78.51 | 76.93 | 0.92 |
Duration of follow-up (m) | 26.00 | 25.75 | 0.47 |
Bone Density (T scores) | 0.94 | ||
BMI (kg/m | 23.51 | 22.66 | 0.16 |
Operation time (h) | 0.76 | 1.96 | 0.003 |
Blood loss (ml) | 5.38 | 6.95 | 0.75 |
Complications | 1 cement leakage (3.7%) | 1 fixation failure (3.0%) | |
Posterior decom-pression and fixation was adapted | Revision surgery was adapted |
Note: The description of statistics is Mean
2.Method
Figure 1.
A 68-year-old man was admitted to hospital for back pain. MRI showed low signal in both T1 and T2 images. b, CT showed IVC sign; c, X-ray showed vertebral compression; d, one week after operation; e, three months after operation; f, two years after operation.
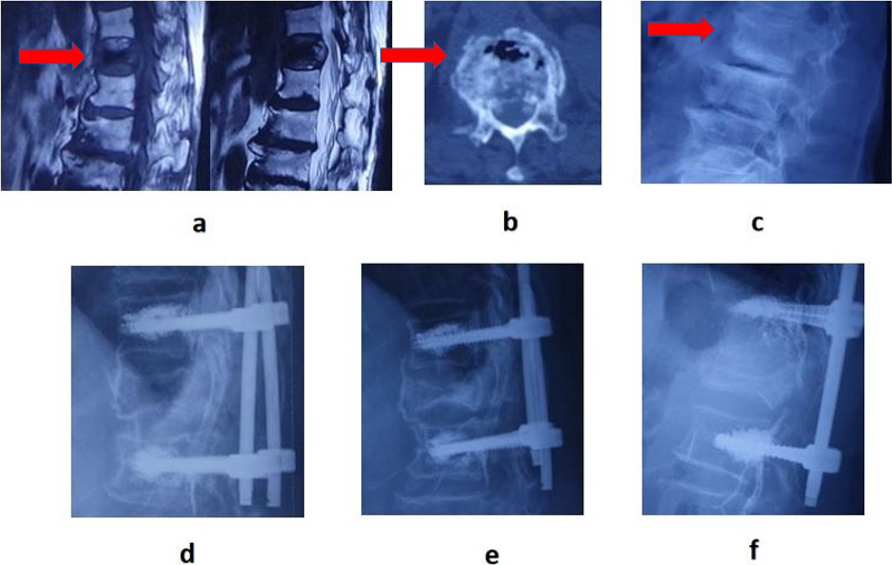
Figure 2.
A 83-year-old female patient with back pain was hospitalized, L3 vertebral body was compressed, and there was no lower limb nerve dysfunction. a, MRI showed low signals, in both T1 and T2 of the injured vertebrae with “IVC sign” of the vertebrae. X-ray and CT showed vertebral compression fractures b and c. Percutaneous vertebroplasty was performed one week after operation, three months after operation, and two years after operation d, e, f.
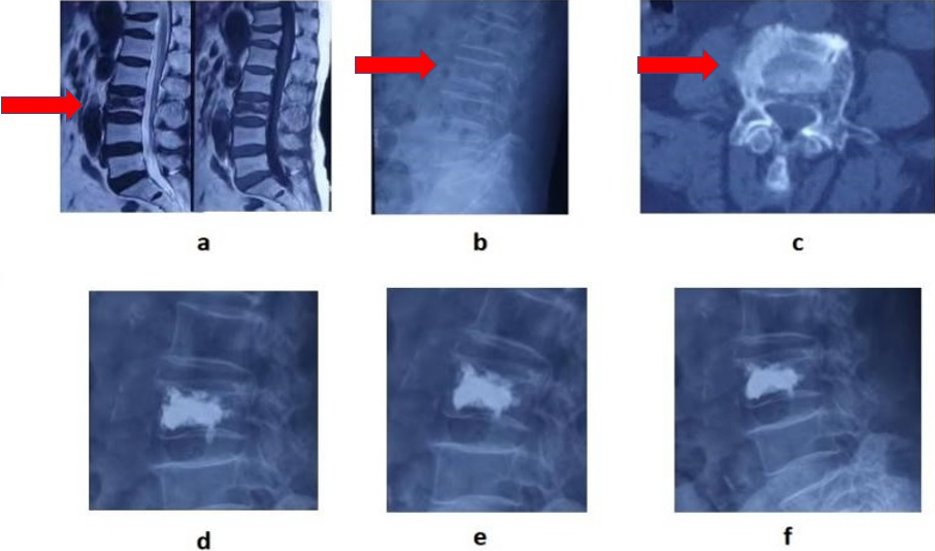
2.1Study design and patient selection
This study was a retrospective cohort study. 92 patients who suffered from Kummell’s disease were included in this study in total. Each patient received clinical and imaging assessments preoperatively and after surgeries. One of the indexes, American Society of Anesthesiology (ASA) classification was utilized as the grouping criterion. Six levels are included in this classification and higher grade presents higher perioperative risk. In this study, patients in III and higher Levels were divided into Group I who received PVP and the others in I and II Levels were included in Group II received PSSF (Table 2). All surgical procedures were performed by the same senior surgeon in the fourth orthopedics department of the First Affiliated Hospital of Harbin Medical University, China, between December 2016 and May 2020 and the period of follow-up was at least 2 years (Figs 1 and 2).
2.2Inclusion and exclusion criteria
Inclusion criteria: (1) Patients older than 65 years old, with back pain for more than 3 weeks; (2) T-score of the patient thoracolumbar vertebral body measured with dual-energy X-ray absorptiometry had to be less than
Exclusion criteria: (1) Follow-up less than 2 years, or patients passed away during the period; (2) Neurological deficits of lower extremities occurred due to the vertebral fracture compression; (3) Multiple adjacent vertebral bodies fractures, history of high-energy trauma or primary tumors; (4) Those patients underwent PSSF without bone cement screws.
This study was approved by the Institutional Review Broad of Harbin Medical University, Heilongjiang, China (No. IRB-AF/SC-04/02.0). All patients involved were well informed about the surgery they received and provided written informed consent. Serious adverse events during the trial were reviewed and reported every 6 months.
2.3Therapy
PVP was performed under X-ray fluoroscopy and the bone cement was inserted bilaterally. The other group underwent PSSF with bone cement screws. Antibiotics were adapted preventively and the anti-osteoporosis treatment was carried out postoperatively. Patients in both groups were asked to wear the brace for 6–8 weeks. For each participant in Groups I and II, a follow-up for at least 2 years was finished. During the period, clinical evaluations were done at the points of 1 week, 3 months, and 2 years after the surgical treatment. At the same time, spinal anteroposterior and lateral radiographs were also taken to assess the height loss and local kyphotic angle. For the patients who presented with leakage of cement during the period of follow-up, posterior decompression and fixation was adapted and internal fixation failure was dealt with via revision surgeries.
2.4Outcome parameters
The patients’ history, radiographs, operation records, and situations in the follow-up period were carefully reviewed and sorted out. The indexes that indicated the therapeutic efficiency included: (1) Visual analogue scale (VAS) scores; (2) Oswestry Disability Index (ODI). The VAS scores ranging from 0 to 10 describe the degree of the pain and higher scores represent severer pain [20, 21, 22]. The quality of the patients’ life was reflected by the ODI scores, which include nine dimensions in everyday life and there are six degrees in each aspect ranging from 0 to 5 [20, 21, 22]. Radiologically, the fractured vertebral height (FVH) ratio, the local kyphotic angle (LKA) and the vertebral wedge angle (VWA) were measured to assess patients in both groups [21, 22, 23]. FVH was measured at the maximal compressed point. The value of the FVH ratio was calculated as follows: [The normal vertebral height (NVH)
Table 3
Comparison between pre- and 1 w post-operative VAS, LKA, VWA and FVHr intra-group
Groups | Pre-scores (Mean | 3 w post-scores (Mean | ||
VAS | PVP GI | 7.78 | 3.00 | |
PSSF GII | 7.78 | 4.33 | ||
LKA ( | PVP GI | 11.65 | 10.80 | 0.54 |
PSSF GII | 12.18 | 6.55 | ||
VWA ( | PVP GI | 14.19 | 13.21 | 0.71 |
PSSF GII | 14.35 | 8.72 | ||
FVHr (%) | PVP GI | 23.88 | 16.37 | |
PSSF GII | 26.34 | 10.44 |
Note: VAS and ODI recorded are the actual scores. The range is in brackets. (
2.5Statistical analysis
The distribution of demographic characteristics and clinical data were compared between cases and controls using the Chi-square test or Fisher exact test or T-test as appropriate. Univariate and multivariate unconditional logistic regression was used to estimate crude and adjusted odds ratios (ORs) and 95% confidence intervals (CIs). The statistical analyses were carried out using SAS version 9.1.3 software (SAS Institute, Cary, North Carolina, USA). All reported P values were two-sided, with
3.Results
3.1Clinical features of the patients involved
Among the total of 92 participants, 49 patients were included in Group I, who underwent percutaneous vertebral augmentation, while the remaining 43 patients were assigned to Group II, who underwent percutaneous short-segment fixation, based on the grouping criterion. During the follow-up period, 32 patients were excluded from the study, with 4 patients in severe status having passed away, and 28 patients lost to follow-up. Consequently, the final analysis included a total of 60 patients, with 27 patients in Group I and 33 patients in Group II, as indicated in Table 1.
Besides, the basic information on eight aspects of each patient has been collected: gender, age, duration of follow-up, Bone Density, Body Mass Index, operation time, blood loss and complications (Table 2). The operation time in Group I is 0.76
3.2Therapeutic effectiveness analysis
We conducted a therapeutic effectiveness analysis by comparing the outcomes one week after the operation with those preoperatively (Table 3). In Group I, the VAS decreased from 7.78
Table 4
Comparison of VAS, ODI and corrections between GI and GII
Items | PVP GI ( | PSSF GII ( | |
---|---|---|---|
Vas pre | 7.78 | 7.78 | 0.97 |
Vas 1 w post- | 3.00 | 4.33 | 0.03 |
Correction | 4.78 | 3.45 | 0.04 |
Vas 3 m post- | 2.59 | 3.36 | 0.008 |
Correction | 5.19 | 4.42 | 0.031 |
Vas 2 y post- | 1.59 | 2.24 | 0.067 |
Correction | 6.18 | 5.54 | 0.34 |
ODI pre (%) | 76.46 | 74.34 | 0.87 |
ODI 2 y post- (%) | 30.37 | 23.50 | |
Correction (%) | 46.09 | 50.84 | 0.029 |
Note: The description of statistics are Mean
Table 5
Comparison of radiographic indexes between GI and GII
Items of LKA ( | PVP GI ( | PSSF GII ( | |
---|---|---|---|
LKA ( | 11.65 | 12.18 | 0.83 |
LKA ( | 10.80 | 6.56 | 0.01 |
Correction ( | 0.86 | 5.62 | 0.01 |
LKA ( | 10.20 | 6.82 | 0.024 |
Correction ( | 1.46 | 5.37 | |
LKA ( | 10.89 | 6.92 | 0.038 |
Correction ( | 0.77 | 5.26 | 0.017 |
VWA ( | 14.19 | 14.35 | 0.92 |
VWA ( | 13.21 | 8.72 | 0.024 |
Correction ( | 0.98 | 5.62 | 0.018 |
VWA ( | 13.34 | 8.58 | 0.033 |
Correction ( | 0.85 | 5.77 | |
VWA ( | 13.48 | 8.31 | 0.011 |
Correction ( | 0.71 | 6.04 | |
FVHr (%) pre | 23.88 | 26.34 | 0.35 |
FVHr (%) 1 w post- | 16.37 | 10.44 | 0.029 |
Correction (%) | 7.51 | 15.89 | |
FVHr (%) 3 m post- | 19.42 | 11.10 | 0.043 |
Correction (%) | 4.46 | 15.24 | |
FVHr (%) 2 y post- | 18.49 | 12.69 | 0.011 |
Correction (%) | 5.39 | 13.65 | 0.01 |
Note: The description of statistics is Mean
3.3Comparison between two methods
Statistical differences in VAS and ODI scores between both groups were not observed before surgical treatments. Similarly, there are no significant differences in VAS and the correction values in 2 years follow-up (
4.Discussion
KD is a clinical condition characterized by painful progressive angular kyphosis due to delayed vertebral body collapse after minor spinal trauma. The choice of surgical approach for the treatment of KD lacks uniformity. While both surgical procedures have been shown to be effective, they differ in certain aspects. PVP could achieve more rapid pain relief with less operation time, even if outcomes in both groups are similar in the long term. In contrast, PSSF demonstrated better kyphosis correction and vertebral height restoration. Based on these results, our findings suggest that PVP is more suitable for KD patients with severe pain, while PSSF is the preferred option for those with serious kyphosis.
Our research findings indicate that, at the 1-week follow-up, both PVP and PSSF provided satisfactory pain relief when the same group of patients was longitudinally compared. Thus, we have confidence in the reliability and effectiveness of both surgical procedures. The dissipation of heat during the solidification of bone cement is a critical mechanism that plays a key role in nerve inactivation and immediate stabilization. Furthermore, PSSF enhances stability, effectively alleviating pain, and improving overall quality of life.
When comparing both operations, PVP could achieve quicker pain relief in a short period (lower VAS at 1 week postoperatively). Possibly, the non-fusion in the fractured vertebral body during PSSF significantly contributes to quicker pain relief. In the long-term, patients in Group II could experience improved health (greater correction of ODI at 2-year follow-up) and comparable pain remission. This improvement might be attributed to better kyphosis correction and vertebral height restoration. Regarding imaging evaluation, PSSF is more affirmable than PVP in both the short and long term due to its superior reduction capabilities.
KD is often accompanied by chronic pain, which is considered one of the special circumstances of OVCFs. This pain often presents with the IVC sign [14], a specific sign that strongly suggests the presence of osteonecrosis [24]. In order to implement the therapeutic strategies, surgical interventions have been performed. Among these methods, both PVP and PSSF have been reported as solutions for KD, resulting in significant improvement [25, 10].
As a minimally invasive treatment, PVP should be considered for persistent pain or progressive vertebral collapse [9]. The results of the study conducted by Ate?et al. showed significant improvements in VAS and ODI scores in both PVP and percutaneous kyphoplasty (PKP) groups during the mean 26-month follow-up, which is consistent with our study [25]. PKP was found to be more effective for correcting kyphosis. Another team also reported that apparent VAS improvements appeared immediately after the PVP and our study indirectly supports their conclusion (VAS scores significantly decreased in PVP at the point of 3 weeks). Similarly, there were no statistically significant differences in local kyphotic angle, but intravertebral instability improved greatly. Moreover, PVP may also resolve delayed neurologic deficits (DND) following OVCFs with IVC, which may be a result of intravertebral instability [5].
According to some scholars, a fibrocartilaginous membrane may form in a fractured vertebral body with IVC sign. This might drive polymethyl methacrylate (PMMA) into the spinal canal, thereby limiting the applicability of PVP in OVCFs with IVC [10]. In contrast, Krauss et al. argued that during PVP, the leakage of PMMA could be reduced due to the presence of the fibrocartilaginous membrane [26]. However, many authors reported cement leakage into the spinal canal in OVCF with IVC. The possible causes include the presence of an IVC sign, a viscosity of injected cement, injection volume and velocity, and the morphology of the fractured vertebral body [27]. In our study, cement (PMMA) leakage occurred in 1 patient, with a rate of 3.7% (1 out of 27), which is lower than the rate reported in previous studies for new OVCFs (10%) [28]. Therefore, we speculated that leakage and obsolete OVCFs are not significantly related. Besides, internal fixation failure was observed in one patient in Group II (1 out of 33, 3.0%), and the incidence rate of complications in both groups was similar.
Vertebral recollapses are another PVP postoperative complication and the incidence is high [29, 30]. It is reported that recollapses are related to the appearance of osteonecrosis [10]. Recollapses would further aggravate the kyphosis deformity. Therefore, PVP is not recommended in the treatment of OVCFs with IVC signs [30]. However, no recollapses were observed in our research. Maybe the proper vertebral height correction for OVCFs and inadequate follow-up period were the possible factors.
The presence of persistent pain, neurologic deficit, or progressive kyphosis indicates the need for decompression and fixation in patients with obsolete OVCFs [18]. Both anterior and posterior techniques can provide satisfactory pain relief and height improvement. For kyphosis correction, the anterior approach is preferred, except that it requires longer operative time, causes more damage, and results in higher blood loss [19, 31]. It is remarkable that larger surgical procedures carry higher risks for elderly patients with comorbidities, potentially jeopardizing their lives in some situations. Consequently, anterior approaches, such as vertebral body replacement, are limited. On the contrary, modified surgeries that aim for minimal injury have been reported more frequently. Short segmental pedicle screw fixation combined with vertebroplasty has been shown to be more effective in treating OVCFs with neurological deficits than posterior decompression and fixation alone [31]. To reduce perioperative injuries, percutaneous pedicle screws are demonstrated to apply and effectively restore normal physiological load transmission of the spine [32]. Wu et al. [33] concluded that percutaneous vertebroplasty with a lateral opening trocar is safe and effective in avoiding cement leakage, improving cement distribution, and restoring vertebral height compared to conventional PVP. The results of this study also demonstrate the advantages of the modified PVP.
In conclusion, according to this study, patients with significant pain may benefit more from PVP. However, for patients with severe kyphotic deformities or those who experience worsened deformity during the procedure, PSSF may be a more effective option for restoring vertebral height, correcting the kyphotic angle, and improving long-term quality of life.
There are still some defects and limitations in our research. First, participants in Group I were less likely to wear the physical brace strictly due to the reduced pain experienced. Furthermore, the use of painkillers during the follow-up period may have affected the VAS scores of participants of both groups. Additionally, our study suffered from inadequate sample sizes in both groups, a short follow-up period, and its retrospective nature. These factors contribute to potential biases in the study results. Therefore, future research should include multi-center, larger sample sizes, longer follow-up periods, and randomized controlled trials (RCTs) to obtain more accurate and reliable conclusions.
5.Conclusion
For the treatment of Kummell’s disease, both PVP and PSSF operations have shown efficacy. PVP is associated with faster pain relief and shorter operation time. However, in the presence of severe kyphosis deformity, PSSF should be prioritized. To achieve optimal clinical outcomes, individual patient characteristics and realistic treatment options must be taken into consideration.
Funding
Postdoctor Science Foundation of Heilongjiang Province (2022).
Ethics statement
The study protocol was approved by the Ethics Committee of First Affiliated Hospital of Harbin Medical University Hospital (No. IRB-AF/SC-04/02.0). All patients involved were well informed about the surgery they received and provided written informed consent.
Author contributions
ZY and JG contributed to the formulating of the hypothesis and study design. XY and TS contributed to the collection of the data. XL and YH were responsible for the statistical analysis. HS and WT wrote the manuscript and handled the correspondence. ZZ and LY helped perform the analysis with constructive discussions. All authors read and approved the final version of the manuscript.
Acknowledgments
The authors have no acknowledgments.
Conflict of interest
None to report.
References
[1] | Johnell O, Kanis JA. An estimate of the worldwide prevalence and disability associated with osteoporotic fractures. Osteoporos Int. (2006) ; 17: (12): 1726-1733. doi: 10.1007/s00198-006-0172-4. |
[2] | Marcia S, Muto M, Hirsch JA, Chandra RV, Carter N, Crivelli P, Piras E, Saba L. What is the role of vertebral augmentation for osteoporotic fractures? A review of the recent literature. Neuroradiology. (2018) ; 60: (8): 777-783. doi: 10.1007/s00234-018-2042-0. |
[3] | Ettinger B, Black DM, Nevitt MC, et al. Contribution of vertebral deformities to chronic back pain and disability. The study of osteoporotic fractures research group. J Bone Miner Res. (1992) ; 7: (4): 449-456. doi: 10.1002/jbmr.5650070413. |
[4] | Nevitt MC, Ettinger B, Black DM, et al. The association of radiographically detected vertebral fractures with back pain and function: a prospective study. Ann Intern Med. (1998) ; 128: (10): 793-800. doi: 10.7326/0003-4819-128-10-199805150-00001. |
[5] | Nakamae T, Fujimoto Y, Yamada K, Takata H, Shimbo T, Tsuchida Y. Percutaneous vertebroplasty for osteoporotic vertebral compression fracture with intravertebral cleft associated with delayed neurologic deficit. Eur Spine J. (2013) ; 22: (7): 1624-1632. doi: 10.1007/s00586-013-2686-8. |
[6] | Kim DH, Vaccaro AR. Osteoporotic compression fractures of the spine; current options and considerations for treatment. Spine J. (2006) ; 6: (5): 479-487. doi: 10.1016/j.spinee.2006.04.013. |
[7] | Melton LJ 3rd, Kan SH, Frye MA, Wahner HW, O’Fallon WM, Riggs BL. Epidemiology of vertebral fractures in women. Am J Epidemiol. (1989) ; 129: (5): 1000-1011. doi: 10.1093/oxford.journals.aje.a115204. |
[8] | Suzuki N, Ogikubo O, Hansson T. The course of the acute vertebral body fragility fracture: its effect on pain, disability and quality of life during 12 months. Eur Spine J. (2008) ; 17: (10): 1380-1390. doi: 10.1007/s00586-008-0753-3. |
[9] | Lim J, Choi SW, Youm JY, Kwon HJ, Kim SH, Koh HS. Posttraumatic delayed vertebral collapse: Kummell’s disease. J Korean Neurosurg Soc. (2018) ; 61: (1): 1-9. doi: 10.3340/jkns.2017.0505.010. |
[10] | Lee SH, Kim ES, Eoh W. Cement augmented anterior reconstruction with short posterior instrumentation: a less invasive surgical option for Kummell’s disease with cord compression. J Clin Neurosci. (2011) ; 18: (4): 509-514. doi: 10.1016/j.jocn.2010.07.139. |
[11] | Venmans A, Klazen CA, Lohle PN, Mali WP, van Rooij WJ. Natural history of pain in patients with conservatively treated osteoporotic vertebral compression fractures: results from VERTOS II. AJNR Am J Neuroradiol. (2012) ; 33: (3): 519-521. doi: 10.3174/ajnr.A2817. |
[12] | Matzaroglou C, Georgiou CS, Panagopoulos A, Assimakopoulos K, Wilke HJ, Habermann B, Panos G, Kafchitsas K. Kümmell’s Disease: Clarifying the Mechanisms and Patients’ Inclusion Criteria. Open Orthop J. (2014) ; 8: : 288-297. doi: 10.2174/1874325001408010288. |
[13] | Young WF, Brown D, Kendler A, Clements D. Delayed post-traumatic osteonecrosis of a vertebral body (Kummell’s disease). Acta Orthop Belg. (2002) ; 68: (1): 13-19. |
[14] | Libicher M, Appelt A, Berger I, Baier M, Meeder PJ, Grafe I, Dafonseca K, Nöldge G, Kasperk C. The intravertebral vacuum phenomen as specific sign of osteonecrosis in vertebral compression fractures: results from a radiological and histological study. Eur Radiol. (2007) ; 17: (9): 2248-2252. doi: 10.1007/s00330-007-0684-0. |
[15] | Kim YC, Kim YH, Ha KY. Pathomechanism of intravertebral clefts in osteoporotic compression fractures of the spine. Spine J. (2014) ; 14: (4): 659-666. doi: 10.1016/j.spinee.2013.06.106. |
[16] | D’Ippolito G, Schiller PC, Ricordi C, Roos BA, Howard GA. Age-related osteogenic potential of mesenchymal stromal stem cells from human vertebral bone marrow. J Bone Miner Res. (1999) ; 14: (7): 1115-1122. doi: 10.1359/jbmr.1999.14.7.1115. |
[17] | Benedek TG, Nicholas JJ. Delayed traumatic vertebral body compression fracture; part II: pathologic features. Semin Arthritis Rheum. (1981) ; 10: (4): 271-277. doi: 10.1016/0049-0172(81)90004-4. |
[18] | Chen L, Dong R, Gu Y, Feng Y. Comparison between balloon kyphoplasty and short segmental fixation combined with vertebroplasty in the treatment of Kümmell’s disease. Pain Physician. (2015) ; 18: (4): 373-381. |
[19] | Park SJ, Kim HS, Lee SK, Kim SW. Bone cement-augmented percutaneous short segment fixation: an effective treatment for Kummell’s disease? J Korean Neurosurg Soc. (2015) ; 58: (1): 54-9. doi: 10.3340/jkns.2015.58.1.54. |
[20] | Buchbinder R, Johnston RV, Rischin KJ, Homik J, Jones CA, Golmohammadi K, Kallmes DF. Percutaneous vertebroplasty for osteoporotic vertebral compression fracture. Cochrane Database Syst Rev. (2018) ; 4: (4): CD006349. doi: 10.1002/14651858.CD006349.pub3. |
[21] | Hu X, Ma W, Chen J, Wang Y, Jiang W. Posterior short segment fixation including the fractured vertebra combined with kyphoplasty for unstable thoracolumbar osteoporotic burst fracture. BMC Musculoskelet Disord. (2020) ; 21: (1): 566. doi: 10.1186/s12891-020-03576-9. |
[22] | Zhong W, Liang X, Luo X, Quan Z. Vertebroplasty and vertebroplasty in combination with intermediate bilateral pedicle screw fixation for OF4 in osteoporotic vertebral compression fractures: a retrospective single-Centre cohort study. BMC Surg. (2019) ; 19: (1): 178. doi: 10.1186/s12893-019-0646-x. |
[23] | Lin CL, Chou PH, Fang JJ, Huang KY, Lin RM. Short-segment decompression and fixation for thoracolumbar osteoporotic fractures with neurological deficits. J Int Med Res. (2018) ; 46: (8): 3104-3113. doi: 10.1177/0300060518772422. |
[24] | Maldague BE, Noel HM, Malghem JJ. The intravertebral vacuum cleft: a sign of ischemic vertebral collapse. Radiology. (1978) ; 129: (1): 23-29. doi: 10.1148/129.1.23. |
[25] | Ateş A, Gemalmaz HC, Deveci MA, Şimşek, Çetin E, Şenköylü. Comparison of effectiveness of kyphoplasty and vertebroplasty in patients with osteoporotic vertebra fractures. Acta Orthop Traumatol Turc. (2016) ; 50: (6): 619-622. doi: 10.1016/j.aott.2016.10.002. |
[26] | Krauss M, Hirschfelder H, Tomandl B, Lichti G, Bär I. Kyphosis reduction and the rate of cement leaks after vertebroplasty of intravertebral clefts. Eur Radiol. (2006) ; 16: (5): 1015-1021. doi: 10.1007/s00330-005-0056-6. |
[27] | Nieuwenhuijse MJ, Van Erkel AR, Dijkstra PD. Cement leakage in percutaneous vertebroplasty for osteoporotic vertebral compression fractures: identification of risk factors. Spine J. (2011) ; 11: (9): 839-848. doi: 10.1016/j.spinee.2011.07.027. |
[28] | Cao P, Hao W, Zhang L, Zhang Q, Liu X, Li M. Safety and efficacy studies of vertebroplasty with dual injections for the treatment of osteoporotic vertebral compression fractures: preliminary report. Acad Radiol. (2020) ; 27: (8): e224-e231. doi: 10.1016/j.acra.2019.09.023. |
[29] | Yu WB, Jiang XB, Liang D, Xu WX, Ye LQ, Wang J. Risk factors and score for recollapse of the augmented vertebrae after percutaneous vertebroplasty in osteoporotic vertebral compression fractures. Osteoporos Int. (2019) ; 30: (2): 423-430. doi: 10.1007/s00198-018-4754-8. |
[30] | Ma YH, Tian ZS, Liu HC, Zhang BY, Zhu YH, Meng CY, Liu XJ, Zhu QS. Predictive risk factors for recollapse of cemented vertebrae after percutaneous vertebroplasty: a meta-analysis. World J Clin Cases. (2021) ; 9: (12): 2778-2790. doi: 10.12998/wjcc.v9.i12.2778. |
[31] | Zhang GQ, Gao YZ, Zheng J, Luo JP, Tang C, Chen SL, Wang HQ, Liu K, Xie RG. Posterior decompression and short segmental pedicle screw fixation combined with vertebroplasty for Kümmell’s disease with neurological deficits. Exp Ther Med. (2013) ; 5: (2): 517-522. doi: 10.3892/etm.2012.833. |
[32] | Sahai N, Faloon MJ, Dunn CJ, Issa K, Sinha K, Hwang KS, Emami A. Short-segment fixation with percutaneous pedicle screws in the treatment of unstable thoracolumbar vertebral body fractures. Orthopedics. (2018) ; 41: (6): e802-e806. doi: 10.3928/01477447-20180912-05. |
[33] | Wu XF, Ping Y, Zeng XQ, Feng Y, Wang Z, Li T, Wu DJ. Percutaneous vertebroplasty with side-opening cannula or front-opening cannula in the treatment of Kummell Disease? Orthop Surg. (2020) ; 12: (4): 1190-1198. doi: 10.1111/os.12730. |