Glycoprotein IIB-IIIA inhibitor, monafram decelerate the early phase of red blood cells aggregation
Abstract
It is commonly known that red blood cell (RBC) tendency to reversibly aggregate contribute to the unique flow properties of blood. The fibrinogen-induced aggregation is primarily based on non-specific processes. Nevertheless, recent investigations disclosed a possibility of the specific fibrinogen-RBC binding, however, its role in RBC aggregation remains unclear. We conducted a study to determine whether this specific interaction is essential for natural RBC aggregation. The influence of glycoprotein (GP) IIb-IIIa inhibitor, monafram, on RBC aggregation was compared in RBC suspensions and in whole blood samples from healthy volunteers and, in addition, from patients with systemic lupus erythematosus (SLE) using laser-light backscattering detection and optical tweezers. The effect of monafram on normal RBC aggregation was detectable only in the early phase of intercellular interactions and it involved a deceleration of the aggregation, which was equally expressed in native blood samples and RBC suspensions based on platelet-free plasma. Additionally, in SLE patients, monafram weakened the strength of paired RBC aggregates in platelet-free suspensions. In healthy subjects, strengthened aggregates were found mainly in platelet-containing bulk RBC suspensions. These findings suggest that normal specific fibrinogen-RBC binding could lead to early accelerated formation of RBC aggregates, which could increase possible beneficial effects of reversible RBC aggregation. The strength of RBC aggregates could be modified by platelets in normal subjects and by specific fibrinogen-RBC binding in SLE patients.
1Introduction
The reversible aggregation of red blood cells is a well-known determinant of hemorheological properties and microcirculation, especially under pathological conditions [2, 3, 11]. So, investigation of mechanisms of RBC aggregation continues to be of the primary scientific and clinical interest, and yet it is far from being resolved [16]. RBCs are considered to interact with each other due to intercellular bridges of polymer molecules [8] or osmotic forces [14], and, according to both explanations, RBC aggregation mechanisms are nonspecific. Nevertheless, it was shown that a number of RBC-bonded fibrinogen molecules, as well as bonding force, can be reduced by suppression of specific fibrinogen binding with RGD-mimetic inhibitors, which had also been found to suppress the aggregation of rats’ RBCs in human fibrinogen solution [6, 13]. The experimental design of these studies, based on the use of suspensions of washed RBCs, has prevented possible artifacts and allows considering the specific fibrinogen binding as an additional determinant of RBC aggregation. The unknown fibrinogen receptors on RBC surface could be attractive targets for hyperaggregation therapy, however, the problem of their real value in RBC intercellular interactions in the whole blood is still opened. In order to take a step closer to solving this problem, we estimated the contribution of the specific mechanism of this process in the kinetics of RBC aggregation and disaggregation in whole blood and red blood cell suspensions.
2Materials and methods
2.1Subjects
Heparinized (5 IU/ml) venous blood samples (6 ml) were obtained from 57 healthy volunteers (mean age 28±5 yrs; ±SD) and, additionally, from 7 patients with systemic lupus erythematosus (SLE; mean age 39±11 yrs) as some RBC receptor abnormalities are intrinsic to this disease [17]. The study was approved by the local committee of Faculty of Fundamental Medicine of Lomonosov Moscow State University, and informed consent was obtained from all the participants.
In the whole blood samples and RBC suspensions hematocrit (Hct) was adjusted up to 40%. To prepare RBC suspensions, RBCs were separated using centrifugation at 1,400 g for 20 min and washed 3 times in 10 mM phosphate buffered saline (pH = 7.4). Then, they were resuspended in native plasma (NP), as well as in platelet-free plasma (PFP) and platelet-rich plasma (PRP) which were obtained by centrifugation at 3000 g for 25 min and at 150 g for 5 min, resp. In order to use optical tweezers, some of the PFP-based samples were diluted till the final concentration of ∼5.103 RBCs/μl.
2.2Study design
In each of the experiments, a sample was divided into two equal aliquots: one served as untreated control, and the other was incubated with monafram (which is a F(ab’)2 fragment of humanized form of the murine monoclonal antibody, CRC64, directed against GP IIb-IIIa) using previously validated protocol (20μg/ml for 30 min [18]). All the samples were tested in random order not later than 1.5–2.0 h after venipuncture. An effect of suppression of the specific fibrinogen binding on “natural” RBC aggregation was tested using whole blood samples, and the similar testing of RBC suspensions was regarded as a control one. To determine whether the observed reactions were dictated by commonly known action of GP IIb/IIIa inhibitor on platelets or by its action on RBC receptors, we estimated an influence of monafram on RBC aggregation in RBC suspensions with different amount of platelets. To test the strength of RBC aggregates in the absence of external influence of platelets, additional experiments with individual pairs of RBCs were carried out using optical tweezers, with 12–18 pairs of RBCs being tested in each sample.
The reagents were purchased from Sigma, except monafram, which was obtained from Russian Cardiology Research and Production Complex.
2.3Hemorheological analysis
RBC aggregation was monitored in the light scattering aggregometer LADE (rheological gap 0.9 mm, λ= 650 nm, laser power 1 mW; ReoMedLab, Moscow) at 25°C using the method of estimation of the output parameters, which was developed by N. Firsov et al. [12]. Briefly, after a short period of high shear rate motion, the flow was stopped and a time (t) - dependent decrease in backscattering intensity (I) was recorded within 2 min, reflecting in-progress RBC aggregation. Then, a hydrodynamic stability of RBC aggregates was tested in the range of apparent shear rates (γ′) from ∼2.5 s- 1 to ∼130 s- 1. The characteristic times of early and later RBC aggregation, T1,2, were calculated according to the formula T1,2 = t/ ln (I). The percentage of aggregates that dissociated at γ′ = ∼2.5 s-1, I2.5, characterized a strength of large RBC aggregates (I2 . 5 was taken with the opposite sign to transform it from the index of dissociation of aggregates into the index of their strength). The index of strength of RBC aggregates in bulk, β, was determined according to the formula β = γ′ / ln (I) [12]. In case of I2 . 5 > –21.6% and β >31.2 s- 1, a sample was considered to belong to a subject with “hyperaggregation” because 95% confidence intervals for their normal mean values had been found to range from –25.1% to –21.6% and from 2.3 s- 1 to 31.2 s- 1, resp. [19].
Aggregation forces in individual pairs of RBCs were studied by optical tweezers technique [1] using the method of RBC aggregation force measurement, which is described in details in Ref. [10]. Briefly, two independent optical traps were formed by two orthogonally polarized Nd-YAG laser beams (1064 nm, ∼27 pN). The system of detection and visual control of trapped RBCs was realized in transmission configuration using a CCD camera. Two RBCs were trapped by two optical traps and brought together to form a paired aggregate. Time of aggregate formation was set to 5 s. After that, the aggregate was pulled apart by increasing a distance between the traps with the fixed speed of 0.3μm/s. As the final result, RBCs dissociated either completely or till a finite contact area between the cells depending on the cells aggregation force. In the latter case, the endpoint distance between the centres of two RBCs, L, was determined and normalized relative to the averaged RBC diameter, D (D=(D1 + D2)/2, where D1 and D2 are the diameters of the first and the second RBCs in a pair aggregate, resp.) L/D was used to characterize RBC aggregation force.
2.4Miscellaneous techniques
The whole blood and RBC suspension Hct was determined by microhematocrit centrifugation.Microscopic examination of blood smears was also realized.
2.5Statistical analysis
Unless otherwise noted, values are presented as mean and standard deviation (SD). Paired Student’s t-test, as well as Wilcoxon test was used to estimate an effect of monafram. The data obtained in different types of RBC suspensions were compared using one way ANOVA. To analyze the data in different groups of persons, Mann-Whitney or independent-sample t-test was used. For all statistical comparisons, a p-value < 0.05 was considered statistically significant.
3Results
According to the RBC aggregation testing, the healthy subjects had been divided into 2 groups, with “hyperaggregation” (Group 1, n = 22) and with normal RBC aggregation (Group 2, n = 35). Standard blood tests, including immunological and coagulation ones, revealed no significant differences between these groups, however, in Group 1, hemorheological parameters were beyond the scope of 95% confidence intervals for their normal mean values.
3.1Effect of monafram on RBC aggregation in blood
It was found that monafram slowed down the early RBC aggregation in each of the blood samples and also on the average (by 19%), although the later aggregation remained practically unaffected (Table 1, T1, T2). In addition, monafram weakened RBCs interaction, but only in the “hyperaggregation” case (Table 1, Group 1, I2.5, β).
3.2Effect of monafram on RBC aggregation in plasma with different platelet content
In RBC suspensions based on platelet-free plasma, native plasma and platelet-rich plasma, monafram did not modify significantly the time of the later aggregate formation, T2 (the data is not shown), but it increased T1 by ∼20% in all the types of suspension both in Group 1 and Group 2 (Fig. 1). As for RBC disaggregation, monafram decreased the strength of RBC aggregates only in Group 1, mainly in the “NP” subgroup (Fig. 2, “NP”). Furthermore, the initial strength of RBC aggregates decreased in platelet-free plasma in Group 1, while it increased in rich platelet plasma in Group 2 (Fig. 2, “intact”).
3.3Effect of monafram on strength of paired RBC aggregates in healthy subjects and in SLE patients
Analysis of initial blood samples showed an increase in red blood cell aggregation and a decrease of its disaggregation in SLE patients compared with healthy individuals as well as in subgroups with “hyperaggregation” compared to those with normal RBC aggregation both in healthy individuals and SLE patients (Table 2).
In platelet-free suspensions, an estimation of the aggregation strength of individual pairs of RBCs using optical tweezers did not reveal any differences between the subgroups of healthy subjects (Fig. 3, “intact”, Group 1 vs. Group 2), and these results coincide well with the decrease of initially enhanced hydrodynamic stability of RBC aggregates in platelet-free plasma in Group 1 (Fig. 2). At the same time, the paired RBC aggregates were strengthened in SLE-Group 1 as compared to Group 1 of healthy subjects (p < 0.001) and against SLE-Group 2 the tendency was analogous (p < 0.053; Fig. 3, “intact”).
It was found that in all the healthy subjects and in SLE patients with normal parameters of RBC aggregation in the blood, the intact samples of RBC suspensions and the monafram treated ones did not differ in number of RBC pairs, that underwent the complete dissociation due to optical traps (28% and 20%, 25% and 33%, 52% and 50% in Groups 1, 2 and SLE-Group 2, resp.). In SLE-Group 1, the equivalent percentage values were 10% and 64%, i.e., the strength of RBC interaction was considerably reduced in the monafram treated samples (p < 0.001). The rest of the pairs showed a certain endpoint distance between the RBC centers (Fig. 3). This distance was smaller in SLE-Group 1 as compared with Group 1 of healthy subjects and with SLE–Group 2. Additionally, it increased under the action of monafram in SLE–Group 1.
4Discussion
The results obtained in this study confirmed and extended our earlier observations [18] and indicated that, in healthy subjects with normal RBC aggregation, there was a deceleration of the first stages of the aggregation, most probably, due to inhibition of specific fibrinogen-RBC binding. Moreover, similar to Carvalho et al. [6, 7], who found a lack of stability of fibrinogen-RBC binding at molecular level, we estimated that the specific fibrinogen-RBC binding did not affect the strength of cells interaction in aggregates. The monafram-induced effects turned out to be nearly equal in platelet-free suspensions and blood samples (Fig. 1, Table 1). Thus, monafram modified fibrinogen-erythrocyte binding rather than fibrinogen-platelet one under non-activated platelet conditions. Our findings argue for the ability of specific fibrinogen-RBC binding to promote early accelerated formation of RBC aggregates of ordinary strength. Earlier, we detected that accelerated formation of RBC aggregates of near-normal strength may be regarded as a precursor of SLE improvement [18], and under physiological conditions it may also have beneficial physiological consequences, enhancing known effects of reversible RBC aggregation [3].
The exact RBC membrane sites of fibrinogen binding remain unclear. Initially, it was shown that it is possible to inhibit RBC aggregation by DIDS (4,4’-diisothiocyanatostilbene-2,2’-disulfonic acid) which binds to the band 3 of RBC membrane [9]. Later, RGD/KGD-related inhibition of RBC aggregation and fibrinogen-RBC specific binding was demonstrated, allowing an unknown integrin-like receptor to become the most probable fibrinogen-binding site on RBCs [6, 7, 13, 18]. CD47 was also proposed as a mediator for the fibrinogen-RBC binding [15] and it can associate both with Rh complex, forming a link to the band 3 complex by binding to the band 4.2 protein [5], and with some integrins, inducing their activation [20]. In any case, it is clear that the specific fibrinogen-RBC binding can influence on RBC aggregation which is mainly caused by non-specific processes, and our results show that this specific binding induces early accelerated formation of non-strengthened RBC aggregates under physiological conditions.
As opposed to the platelet-independent normal RBC intercellular interactions (Fig. 1, Group 2), it seems to be platelet-sensitive in healthy subjects with strengthened aggregates where monafram showed a tendency to decelerate RBC aggregation in platelet-rich plasma to a smaller degree than in platelet-free one (by 9% vs, 31%; Fig. 1, Group 1). In this case, the initially strengthened RBC aggregates lost an excess of their strength in platelet-free plasma (Fig. 2, Group 1). Moreover, even in the case of normal RBC aggregation, excessive platelets likely encourage strengthening of initially non-strengthened aggregates (Fig. 2, Group 2), and this effect is consistent with the previous studies [4], demonstrating an increase in RBC interactions in platelet-rich plasma. Thus, platelets (microparticles) could be responsible for the monafram-sensitive strengthening of RBC aggregates in healthy subjects; however, our previous study showed that this strengthening is often transient and it does not widely crop up under physiological conditions [18].
Using optical tweezers, we found some differences in mechanisms underlying for the strengthening of RBC aggregate in normal and SLE subjects. Indeed, the abnormal strengthening of RBC aggregates in the whole blood samples was observed both in Group 1 and SLE-Group 1 (Table 2, I2.5 and β), however, the platelet-free plasma was effective in its normalizing in Group 1, i.e., in healthy subjects, but not in SLE-Group 1, where RBC aggregate strength became normal due to monafram (Fig. 3). Thus, in SLE, strengthening of RBC aggregates could predominantly be a result of abnormal specific fibrinogen-RBC binding.
Taken together the results of the study permit us to suppose that the interaction of fibrinogen with the unknown receptor on the erythrocyte membrane, that is sensitive to GP IIb-IIIa inhibitors, can accelerate the early phase of normal erythrocyte aggregation without pronounced influence on the strength of intercellular interaction. At the same time, in patients with SLE, the specific binding of fibrinogen to the erythrocyte membrane could change both their aggregation and disaggregation.
Acknowledgments
We would like to thank Professor A.V. Mazurov at Russian Cardiology Research and Production Complex for providing monofram and staff members at E.M. Tareev Clinic of the Nephrology, Internal and Professional Diseases for carrying out standard blood tests.
References
[1] | Ashkin A . Acceleration and trapping of particles by radiation pressure. Phys Rev Lett (1970) ;24: :156–9. |
[2] | Baskurt OK . In vivo correlates of altered blood rheology. Biorheology (2008) ;45: :629–38. |
[3] | Baskurt OK , Meiselman HJ . Erythrocyte aggregation: Basic aspects and clinical importance. Clin Hemorheol Microcirc (2013) ;53: :2–337. |
[4] | Bäumler H , Schüjrer B , Distler J . Rouleau formation of erythrocytes is influenced by thrombocytes. Folia Haematol Int Mag Klin Morphol Blutforsch (1987) ;114: :478–9. |
[5] | Bruce LJ , Beckmann R , Ribeiro ML , Peters LL , Chasis JA , Delaunay J , et al. A band 3-based macrocomplex of integral and peripheral proteins in the RBC membrane. Blood (2003) ;101: :4180–8. |
[6] | Carvalho FA , Connell S , Miltenberger-Miltenyi G , Pereira SV , Tavares A , Ariëns RA , et al. Atomic force microscopy-based molecular recognition of a fibrinogen receptor on human erythrocytes. ACS Nano (2010) ;4: :4609–20. |
[7] | Carvalho FA , Santos NC . Atomic force microscopy-based force spectroscopy - biological and biomedical applications. IUBMB Life (2012) ;64: :465–72. |
[8] | Chien S , Jan K . Ultrastructural basis of the mechanism of rouleaux formation. Microvasc Res (1973) ;5: :155–66. |
[9] | Fabry TL . Mechanism of erythrocyte aggregation and sedimentation. Blood (1987) ;70: :1572–6. |
[10] | Khokhlova MD , Lyubin EV , Zhdanov AG , Rykova SY , Sokolova IA , Fedyanin AA . Normal and system lupus erythematosus red blood cell interactions studied by double trap optical tweezers: Direct measurements of aggregation forces. J Biomed Opt (2012) ;17: (2):025001. |
[11] | Knisely MH , Bloch EH , Eliot TS , Warner L . Sludged blood. Science (1947) ;106: :43140. |
[12] | Korotaeva TV , Firsov NN , Bjelle A , Vishlova MA . Erythrocytes aggregation in healthy donors at native and standard hematocrit: The influence of sex, age, immunoglobulins and fibrinogen concentrations. Standardization of parameters. Clin Hemorheol Microcirc (2007) ;36: :335–43. |
[13] | Lominadze D , Dean W . Involvement of fibrinogen specific binding in erythrocyte aggregation. FEBS Lett (2002) ;517: :4–14. |
[14] | Meiselman HJ . Red blood cell aggregation: 45 years being curious. Biorheology (2009) ;46: :1–19. |
[15] | de Oliveira S , Vitorino de Almeida V , Calado A , Rosario HS , Saldanha C . Integrin-associated protein (CD47) is a putative mediator for soluble fibrinogen interaction with human red blood cells membrane. Biochimica et Biophysica Acta (2012) ;1818: :481–90. |
[16] | Saldanha C . Instrumental analysis applied to erythrocyte properties. Journal of Cellular Biotechnology (2015) ;1: :81–93. |
[17] | Singh V , Mahoney JA , Petri M . Erythrocyte C4d and complement receptor 1 in systemic lupus erythematosus. J Rheumatol (2008) ;35: :1989–93. |
[18] | Sokolova IA , Muravyov AV , Khokhlova MD , Rikova SYu , Lyubin EV , Gafarova MA , et al. An effect of glycoprotein IIb/IIIa inhibitors on the kinetics of red blood cells aggregation. Clin Hemorheol Microcirc (2014) ;57: :291–302. |
[19] | Sokolova IA , Rikova SYu , Shahnazarov AA , Gafarova MA , Krasnova TN , Khokhlova MD , et al. Erythrocyte aggregation: Some questions and hypothesis. Russian J Biomech (2011) ;15: :7–22. |
[20] | Soto-Pantoja DR , Stein EV , Rogers NM , Sharifi-Sanjani M , Isenberg JS , Roberts DD . Therapeutic opportunities for targeting the ubiquitous cell surface receptor CD47. Expert Opin Ther Targets (2013) ;17: :89–103. |
Figures and Tables
Fig.1
Influence of monafram on characteristic time of early RBC aggregation (T1) in platelet-free plasma (PFP), native plasma (NP), and platelet rich plasma (PRP) of healthy volunteers with “hyperaggregation” (Group 1, n = 6) and normal aggregation (Group 2, n = 12). Difference from intact samples, •p < 0.05 and difference from Group 2, ∧p < 0.05, ∧∧p < 0.01.
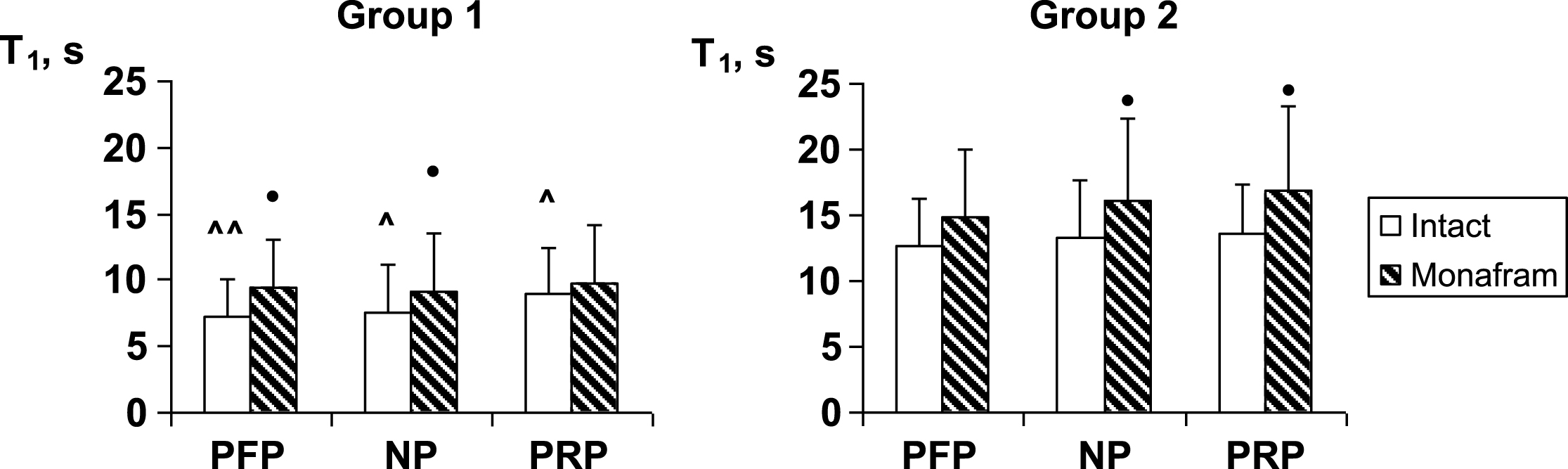
Fig.2
Influence of monafram on hydrodynamic strength of RBC aggregates (β) in platelet-free plasma (PFP), native plasma (NP), and platelet rich plasma (PRP) of healthy volunteers with “hyperaggregation” (Group 1, n = 6) and normal aggregation (Group 2, n = 12). A comparison of monafram-treated and intact samples, •p < 0.05. Difference from Group 2, ∧p < 0.001 and difference from “NP”, *p < 0.05.
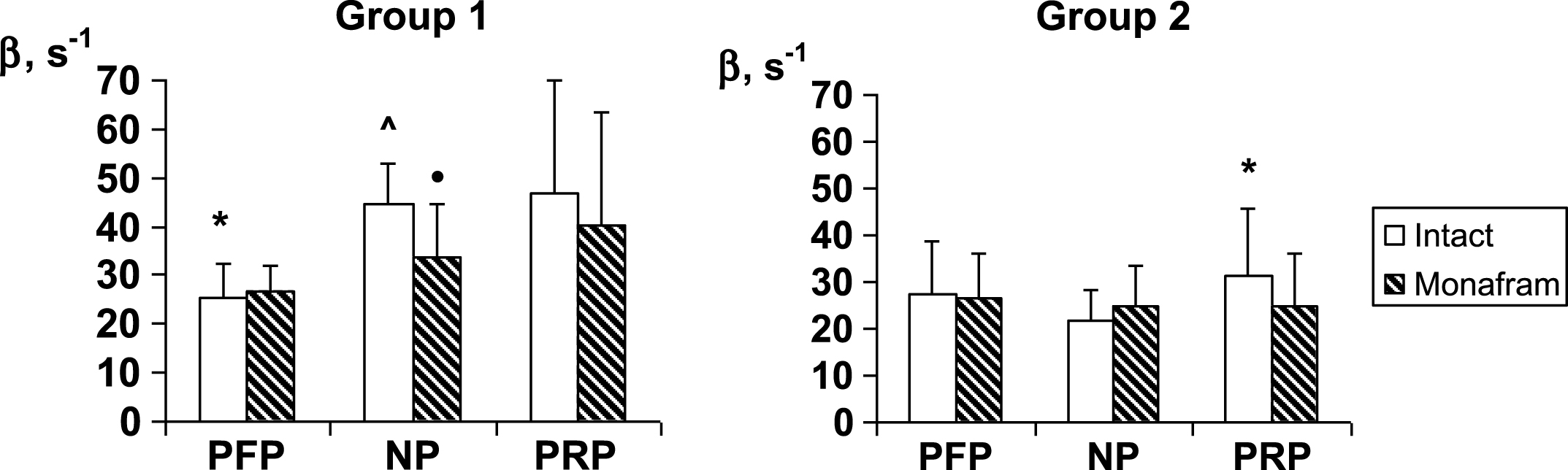
Fig.3
Influence of monafram on index of strength of paired RBC aggregates (L/D; median values along with 25 and 75 percentiles) in healthy and systemic lupus erythematosus (SLE) subjects with “hyperaggregation” (Group 1, n = 8 and SLE-Group 1, n = 5, resp.) and normal parameters of RBC aggregation (Group 2, n = 6 and SLE-Group 2, n = 2, resp.). A comparison of monafram-treated and intact samples, •p < 0.01. Difference from SLE-Group 2, ∨p < 0.01 and difference from Group 1, &p < 0.05.
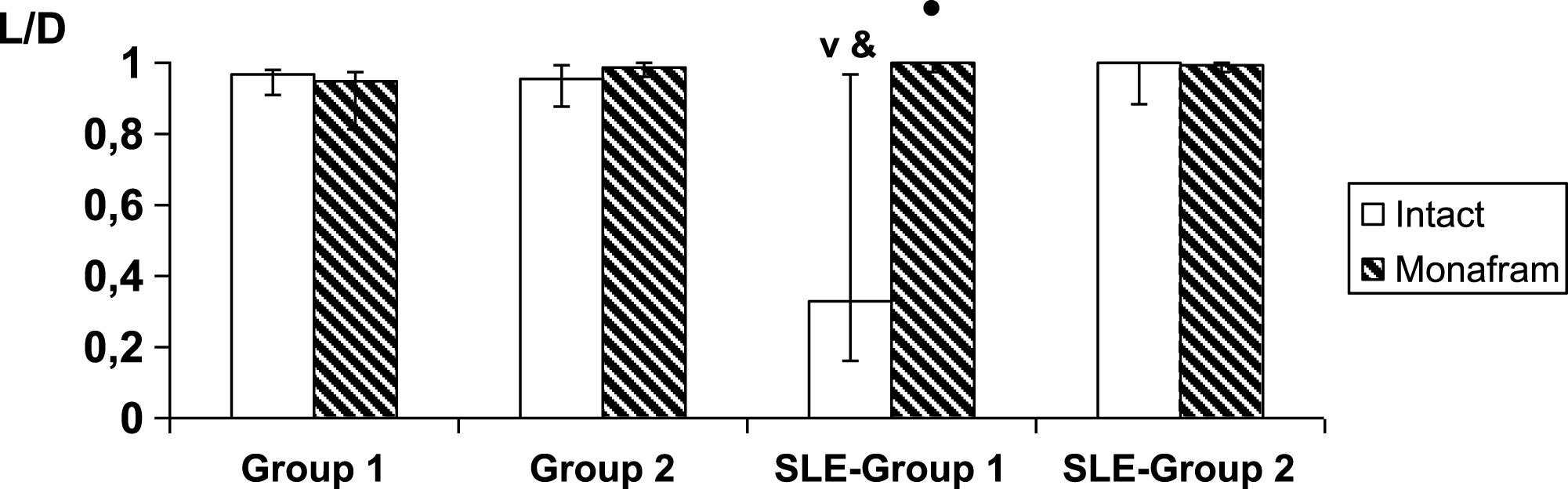
Table 1
Influence of monafram on characteristic times of early and later RBC aggregation (T1,2) and indexes of hydrodynamic strength for all (β) and large (I2.5) RBC aggregates in blood samples of healthy volunteers with “hyperaggregation” (Group 1, n = 8) and normal aggregation (Group 2, n = 17)*
Group 1 | Group 2 | |||
Intact | Monafram | Intact | Monafram | |
T1, s | 6.1±2.8∧ | 7.6±3.3• | 9.2±3.2 | 10.7±4.3•• |
T2, s | 33.9±2.2 | 34.5±2.0 | 35.3±2.5 | 35.2±2.0 |
I2.5, % . | –13.4±5.9∧∧ | –21.3±5.4• | –25.3±5.8 | –25.2±4.9 |
β, s- 1 | 37.2±7.8∧∧ | 32.7±8.5 | 24.0±7.3 | 23.7±8.0 |
*Difference from intact samples, •p < 0.01, ••p < 0.001 and difference from Group 2, ∧p < 0.05, ∧∧p < 0.001.
Table 2
Characteristic times of early RBC aggregation (T1) and indexes of hydrodynamic strength for all (β) and large (I2.5) RBC aggregates in blood samples of healthy and systemic lupus erythematosus (SLE) subjects with RBC “hyperaggregation” (Group 1, n = 8 and SLE-Group 1, n = 5, resp.) and normal parameters of RBC aggregation (Group 2, n = 6 and SLE-Group 2, n = 2, resp.)*
Group 1 | Group 2 | SLE-Group 1 | SLE-Group 2 | |
T1, s | 7.8±2.8 | 11.1±3.1 | 5.1±1.8 V | 6.4±0.8 & |
I2.5, % | –18.0±1.7 ∧ | –28.6±2.8 | –10.8±5.2 V & | –22.0±7.8 & |
β, s- 1 | 34.3±4.6∧ | 20.8±4.4 | 42.1±11.2 | 38.1±10.9 & |
*Difference from Group 2, ∧p < 0.001 and difference from SLE-Group 2, vp < 0.05. A comparison of SLE-Group 1 vs. Group 1 and SLE-Group 2 vs. Group 2, &p < 0.05.