The effects of ALK5 inhibition and simultaneous inhibition or activation of HIF-1α in melanoma tumor growth and angiogenesis
Abstract
BACKGROUND:
Hypoxia is the most common signature of the tumor microenvironment that drives tumorigenesis through the complex crosstalk of a family of transcription factors called hypoxia-inducible factors (HIFs), with other intercellular signaling networks. Hypoxia increases transforming growth factor-beta (TGF-β) expression. TGF-β and HIF-1α play critical roles in several malignancies and their interactions in melanoma progression remain unknown. Therefore, the aim of this study was to assess the impact of inhibiting activin receptor-like kinase-5 (ALK5), a TGF-β receptor, on the response to HIF-1α activation or inhibition in melanoma tumor progression.
MATERIALS AND METHODS:
Tumors were induced in C57BL/6J mice by subcutaneous inoculation with B16F10 melanoma cells. Mice were divided into HIF-1α inhibitor, ALK5 inhibitor (1 mg/kg) and HIF-1α inhibitor (100 mg/kg), ALK5 inhibitor, HIF-1α activator (1000 mg/kg), HIF-1α activator and ALK5 inhibitor, and control groups to receive inhibitors and activators through intraperitoneal injection. The expression of E-cadherin was evaluated by RT-qPCR. Vessel density and platelet-derived growth factor receptor beta (PDGFR)-β+ cells around the vessels were investigated using immunohistochemistry.
RESULTS:
The groups receiving HIF-1α inhibitor and activator showed lower and higher tumor growth compared to the control group, respectively. E-cadherin expression decreased in all groups compared to the control group, illustrating the dual function of E-cadherin in the tumor microenvironment. Vascular density was reduced in the groups given HIF-1α inhibitor, ALK5 inhibitor, and ALK5 and HIF-1α inhibitor simultaneously. The percentage of PDGFR-β+ cells was reduced in the presence of HIF-1α inhibitor, ALK5 inhibitor, HIF-1α and ALK5 inhibitors, and upon simultaneous treatment with HIF-1α activator and ALK5 inhibitor.
CONCLUSION:
Despite increased expression and interaction between TGF-β and HIF-1α pathways in some cancers, in melanoma, inhibition of either pathway alone may have a stronger effect on tumor inhibition than simultaneous inhibition of both pathways. The synergistic effects may be context-dependent and should be further evaluated in different cancer types.
1Introduction
Malignant melanoma is to blame for 60–80% of skin cancer-related mortalities [1]. The induction of angiogenesis is an essential process for tumor progression. Anti-angiogenic therapies could therefore be promising options to limit tumor progression and metastasis [2, 3]. Melanoma angiogenesis is an erratic process showing heterogenic behavior in blood vessel formation. Melanoma cells motivate the expansion of existing blood vessels and attract bone marrow progenitor cells to hypoxic regions within the tumor microenvironment to stimulate vascular formation. In a process known as vascular mimicry, melanoma cells can adopt an endothelial-like phenotype and contribute to vascular construction [4]. Angiogenesis inhibition has been employed for several tumors such as renal, colorectal, and ovarian neoplasms, however there is a dearth of knowledge about clinical melanoma research [4]. Since melanoma is intensely vascularized, anti-angiogenic therapies have been shown to be beneficial for effective treatment [5].
TGF-β is a multifunctional cytokine that has been linked to the development of melanoma by activating the epithelial-mesenchymal transition (EMT) pathway, angiogenesis, and fostering an environment favorable to tumor growth [6, 7]. ALK1 and ALK5, type I TGF-β receptors, play crucial roles in development and maturation of blood vessels, by promoting proliferation of endothelial cells and recruiting pericytes around blood vessels, respectively [8]. ALK5 inhibitors antagonize TGF-β signaling through blockade of TGF-β receptor-activated Smad phosphorylation [9].
TGF-β members are assumed to be prognostic biomarkers in advanced tumors and also beneficial targets for restricting the progression of cancer [10]. Data from pre-clinical research focusing on TGF-β signaling suggest that this pathway is a potential target for cancer treatment [11]. However, TGF-β plays paradoxical dual roles, as both promoter and suppressor, in the progression of metastatic and non-metastatic pancreatic ductal adenocarcinoma (PDAC) [12]. The tumor suppressor activity is widely described as anti-proliferative and pro-apoptotic [13]. During cancer development, tumors escape the tumor-suppressive activities of TGF-β and utilize it as a growth promoter to induce tumor cell proliferation, migration, invasion, metastasis, and EMT [14]. For example, melanoma cells secrete elevated concentrations of TGF-β acting on tumor cells and their microenvironment through autocrine and paracrine processes and promote melanoma progression [15]. Furthermore, TGF-β receptors are involved in E-cadherin mediated induction of EMT by triggering the Smad2/3, Ras, and PI3K (Phosphatidylinositol-3-kinase) pathways, activating transcription factors which promote the expression of genes mediating the EMT process [16, 17]. The expressions of E-cadherin and other EMT-associated markers are also altered in hypoxic circumstances, as its low expression has been observed in cells which received hypoxia treatment [18].
TGF-β signaling interacts with other signaling pathways such as the HIF-1α pathway [19]. HIF-1 regulates adaptability to O2 depletion and plays critical oncogenic roles in the promotion of cancer cell proliferation and tumor angiogenesis, which have been studied in melanoma and other malignancies [20]. HIF-1α promotes tumor invasion and metastasis through the induction of pro-angiogenic factors such as platelet-derived growth factor (PDGF), which stimulate extravasation [21]. Functioning as an upstream regulator of TGF-β, HIF-1α contributes to cancer progression and malignancy [22]. The therapeutic benefit of the HIF-1 inhibitors has been studied in several cancer types [23]. Through its kinase activity, ALK5 stimulates the expression of HIF-1 and HIF-2. HIF-proteins are linked with activation of the TGF-β signaling pathway in a hypoxic environment. ALK5 is essential for the synergistic connection between TGF-β signaling and the hypoxia pathway which promotes the growth of tumors [24].
In line with other studies demonstrating TGF-β’s functional involvement in promoting angiogenesis, TGF-β receptor I (TGF-βRI) is also part of a complex with HIF-1/HIF-2-containing proteins in clear cell renal cell carcinomas (ccRCCs) in vitro, indicating a role for TGF-βRI in hypoxia. Since HIF-1 and TGF-β elicit synergistic effects to promote tumor growth in part by activating related target genes, the potential utility of a drug combination that specifically inhibits HIF-1 and TGF-βRI should be further investigated as a possible step forward in the development of more effective treatment methods in personalized medicine [25].
Treatment with anti-angiogenic factors is a promising approach in the treatment of cancer since most tumors must be supported by a functioning vascular network in order to grow beyond their usual boundaries and metastasize. With positive outcomes, the major challenge is the short period of successful therapeutic response before tumors begin to develop further. Researchers are therefore searching for alternative targets for multi-targeting of the parallel pro-angiogenic signaling circuits [26]. Although anti-angiogenic medicines can slow down tumor growth by directing less oxygen and nutrients to the tumor mass, this also obliterates the route for drug delivery [27]. This study intends to attenuate vessel maturation by decreasing pericyte recruitment surrounding blood vessels through ALK5 suppression and keeping ALK1 intact rather than completely destroying tumor vasculature.
HIF family members and HIF-driven transcriptional responses are critical regulators of the hypoxic tumor microenvironment [28]. The activity of the TGF-β1 promoter is regulated by HIF-1 binding to the hypoxia response element (HRE) [29]. Overall, the findings show that a hypoxic microenvironment can promote the proliferation of cancer cells via activating the TGF-β1 gene expression in a paracrine manner [29]. Although targeting HIFs can have synergistic benefits with targeting TGF-β, it also alters the intrinsic hypoxic nature of the tumor microenvironment, which presents a conflict when determining the precise function of TGF-β inhibition in the reduction of tumor progression. Furthermore, since TGF-β targeting therapeutics would be prescribed after HIF-related changes in the tumor microenvironment, we preferred to partially block the HIF pathway not to change the hypoxic nature of the tumor microenvironment. Accordingly, in this study we hypothesized that simultaneous inhibition of ALK5 and HIF-1α may be a promising therapeutic strategy to control tumor growth in the B16F10 melanoma animal model.
2Materials and methods
This study was designed to investigate the role of TGF-β and HIF-1α signaling pathway interactions in tumor progression.
All the experiments were approved by the Ethics Committee of Isfahan University of Medical Sciences, Isfahan, Iran (approval ID: IR.MUI.REC.1394.3.311).
2.1Cell culture
B16F10 melanoma cells were obtained from the National Cell Bank of Iran (NCBI, Pasteur Institute of Iran) and grown in DMEM complemented by glutamine, glucose, and 10% fetal bovine serum (FBS) with 5% CO2 at 37°C in a humidified atmosphere until the third passage before performing the experiments. At 70–80% confluence, sub-culturing was done with an aseptic technique in sterile circumstances to achieve optimal proliferation.
2.2Tumor-bearing animal model
Sixty healthy female C57BL/6J mice (4-5 weeks old, weighing 18–22 g) were purchased from the Pasteur Institute of Iran. The animals were maintained for two weeks in accordance with the The National Institutes of Health guide for the care and use of laboratory animals (NIH Publications No. 8023, revised 1978). Cells were implanted into recipient mice by subcutaneous injection of 1×106 B16F10 melanoma cells in 200μl phosphate-buffered saline (PBS) in the lateral flank. The day of inoculation was defined as day 0. Mice were checked daily for tumor incidence and development. Primary palpable tumors developed on days 8-9 and were measured in two dimensions three times per week using calipers. On day 10, the tumor-bearing mice were randomly assigned into six groups (n = 10 per group), receiving interventions as follows: HIF-1α inhibitor (HIF-ihb), ALK5 inhibitor and HIF-1α inhibitor (ALK5-ihb/HIF-ihb), ALK5 inhibitor (ALK5-ihb), HIF-1α activator (HIF-act), HIF-1α activator and ALK5 inhibitor (ALK5-ihb/HIF-act), and control groups.
2.3Treatments
ALK5-ihb group: The TGF-βRI inhibitor “LY-364947” (an ATP-competitive inhibitor of TGF-βRI, Selleck Chemicals, TX, USA) was dissolved in 5 mg/mL DMSO and diluted with PBS for intraperitoneal administration at a dose of 1 mg/kg once daily for 6 days [30]. Due to high selectivity, the LY-364947 small molecule is used to block the ALK5 pathway and has been documented to specifically inhibit activin and no other signaling pathways [31].
HIF-ihb group: Methoxyestradiol (2-MeOE2), an inhibitor of HIF-1α (Selleck Chemicals, TX, USA) was dissolved in DMSO and diluted with PBS and administered intraperitoneally at 100 mg/kg to the HIF-ihb group once daily for 3 days [32].
ALK-ihb/HIF-ihb group: 1 mg/kg LY-364947 and 100 mg/kg 2-MeOE2 were administrated to the ALK-ihb/HIF-ihb group once daily for 3 days.
HIF-act group: IOX2, a potent inhibitor of HIF-1α prolyl hydroxylase-2 (PHD2) (SelleckChemicals, TX, USA), was dissolved in DMSO and diluted with PBS and then administered intraperitoneally at 1000 mg/kg once daily for 6 days [33].
ALK-ihb/HIF-act group: 1 mg/kg LY-364947 and 1000 mg/kg IOX2 were administrated once daily for 6 days.
Control group: Untreated mice received 100μL PBS with 0.1% DMSO once daily for 6 days.
After treatment, the animals were sacrificed following anesthesia through IP injection of ketamine (80–100 mg/kg) and xylazine (10–12.5 mg/kg) and underwent necropsies to be checked for the presence of macroscopic metastases and their tumors were removed for immunohistochemical staining and morphological measurements (weight, length (L), width (W), and depth (D)). The rate of tumor growth was investigated by measuring tumor volume at different time points using a Vernier caliper. Then, tumor volume (V) was determined using the formula V = L×W×D×3.14/6.
2.4Immunohistochemistry
Dissected tumors were fixed in 10% formalin, embedded in paraffin, and cut into 5-μm thick sections. Tumor sections were deparaffinized in xylene, rehydrated in graded alcohol, and transferred to PBS. After antigen retrieval, the endogenous peroxidase was blocked with 3% hydrogen peroxide in methanol for 20 minutes. Sections were next incubated with bovine serum albumin (BSA) for 1 hour at room temperature. The slides were then exposed with a primary antibody overnight at 4°C (anti-mouse CD31; 1:200 Ebioscience Co. and anti-mouse PDGFR-β; 1:50 Ebioscience Co.) and then incubated with a biotinylated secondary antibody for 1 hour at 37°C (HRP-Streptavidin; 1:500 Biolegend Co.). The reaction was developed with a DAB substrate, and the sections were counterstained with hematoxylin. The angiogenic response was measured by the number of CD31-positive cells and PDGFR-β+ cells, which were counted in five fields of three different slides using an Olympus light microscope with high power field (×40 magnification) by two independent observers.
2.5Reverse transcriptase–polymerase chain reaction (RT-PCR)
RNA was extracted from tumor tissues using YTzol solution (Yekta Tajhiz Azma, Iran) according to the manufacturer’s instructions and cDNA was synthesized by utilizing Revert AidTM Reverse Transcriptase (Fermentas, Vilnius, Lithuania) with oligo-dT primers. Quantitative real-time RT-PCR was performed using specific primers for E-cadherin, with glyceraldehyde-3-phosphate dehydrogenase (GAPDH) serving as the internal housekeeping control, using SYBR Green qPCR Master Mix (Fermentas, Vilnius, Lithuania) and run in the Rotor-gene 6000 cycler (Corbett Life Science, Sydney Australia). The primers were designed by beacon designer version 8.0: E-cadherin forward primer: 5-TCCTCGCCCTGCTGATTCTG-3, E-cadherin reverse primer: 5-CTGGTCTTCTTCTCCACCTCCTTC-3, and GAPDH forward primer: 5′-AGTCCA CTGGCGTCTTCAC-3′; GAPDH reverse primer: 5′-AGGCATTGCTGAT GATCTTGAG-3′ [34]. The PCR reaction was run using the following conditions: 10 minutes at 95°C for the initial denaturation, 45 amplification cycles consisting of denaturation at 95°C for 15 s, annealing at 60°C for 30 s, and an extension at 72°C for 30 s. The amplification efficiencies were checked for both E-cadherin and housekeeping genes. The q-PCR data were analyzed using the comparative CT method. The difference in cycle threshold, ΔCT, was determined as the difference between the tested gene and GAPDH. For calculations of fold-changes, we then obtained Δ ΔCT, where ΔCt = Ct (Target Gene) –Ct (GAPDH) for each treatment, as reported previously [35].
2.6Statistical analysis
Statistical analysis was performed using SPSS version 20 (SPSS Inc, Chicago, IL). One-way ANOVA was used to determine if there were any significant differences between the groups and post-hoc Tukey test was employed to distinguish where those significant differences lay. Results were considered statistically significant if the P-value was <0.05.
3Results
Tumor weight was significantly reduced in the groups that received HIF inhibitor (p < 0.01) and ALK5 inhibitor (p < 0.05). The typical photos of the isolated tumor from each group showing the different sizes of tumors after drug treatments are shown in Fig. 1. Despite our expectations, the decrease in the tumor weight in the group given both inhibitors was not significant. There was no significant difference in the tumor weight of HIF-act and HIF-act/ALK5-ihb groups when compared to the control group (Fig. 2).
Fig. 1
Typical photos of isolated tumors from each group.
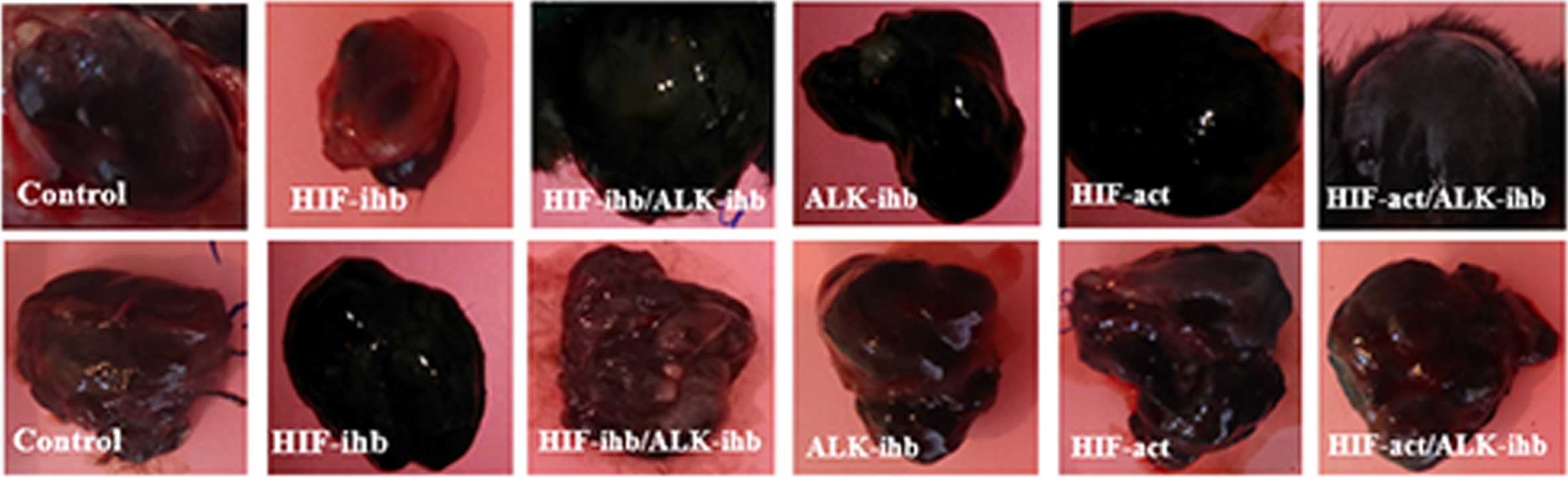
Fig. 2
Tumor weights on the last day of the experiments. Tumor weight significantly decreased in the HIF-ihb (**p < 0.01) and ALK5-ihb (*p < 0.05) groups. Each bar represents mean±SEM. Alk5-ihb: ALK5 inhibitor, HIF-ihb: HIF-1α inhibitor, HIF-act: HIF-1α activator.
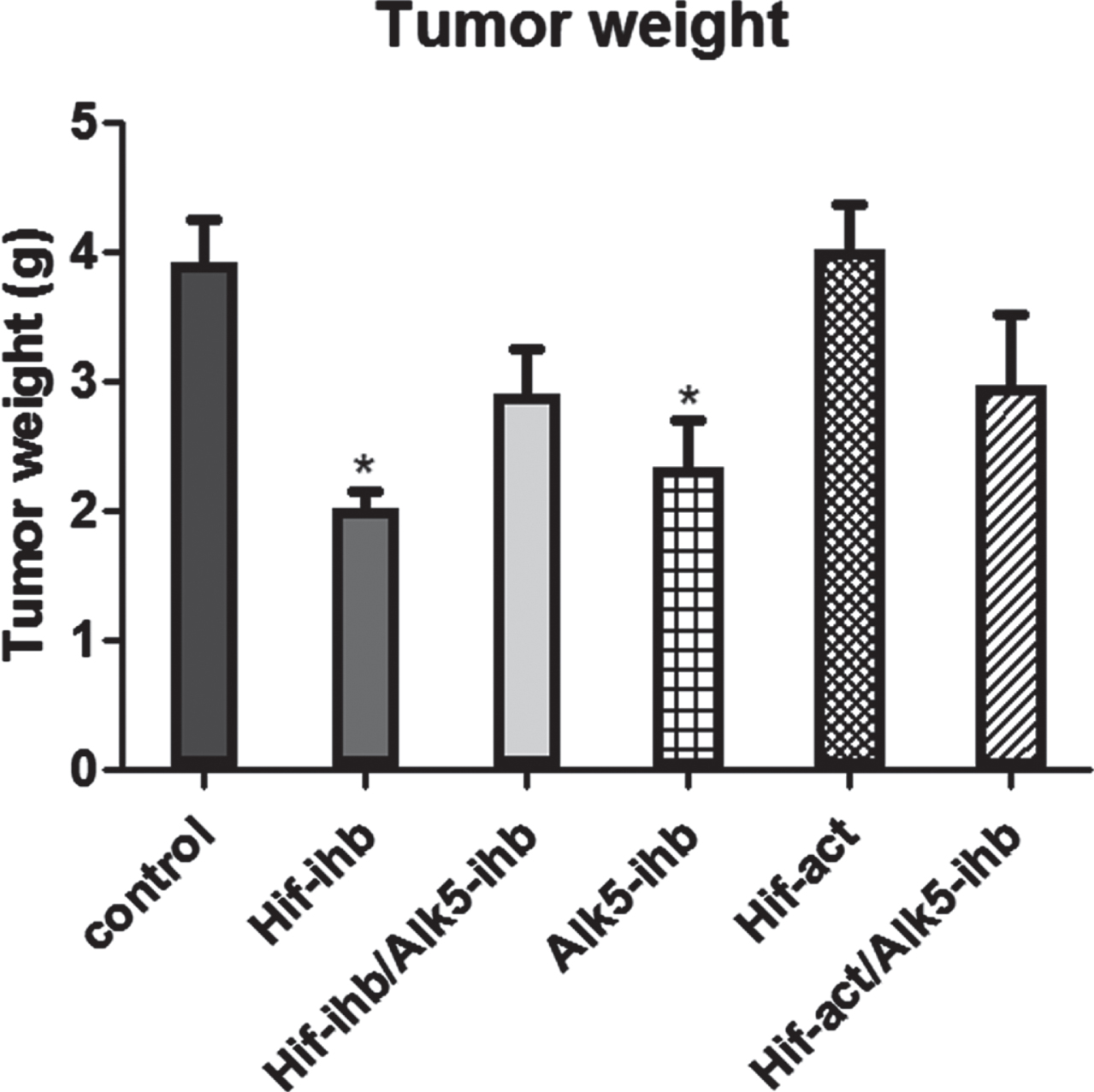
The rate of tumor growth showed a significantly lower speed in the HIF-ihb group (p < 0.005) in comparison to the control group. The tumor growth rate reduction in HIF-ihb/ALK5-ihb and ALK5-ihb was not significant. In addition, the tumor growth rate in the HIF-act group showed a higher speed than the HIF-ihb group (p < 0.05) (Fig. 3).
Fig. 3
The rate of tumor growth in the melanoma mouse model. The rate of tumor growth showed a significantly lighter slope in the HIF-ihb group (***p < 0.005) compared to the control group and the HIF-act group, indicating the role of HIF inhibition in tumor growth prevention. The tumor volumes measured on the 3rd, 6th, and 9th day after intervention commencement. Alk5-ihb: ALK5 inhibitor, HIF-ihb: HIF-1α inhibitor, HIF-act: HIF-1α activator.
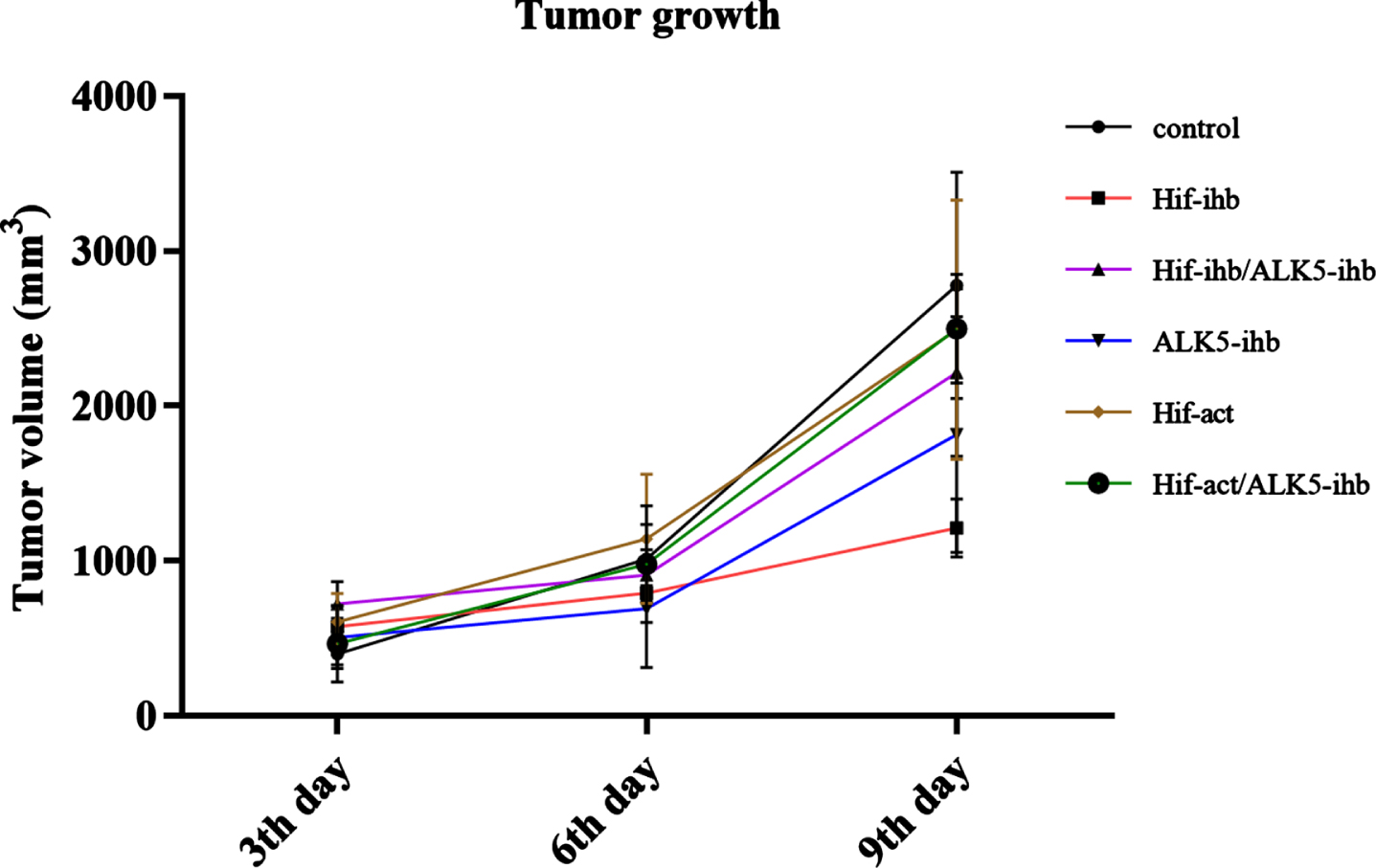
There were significant reductions in the E-cadherin expression of tumor tissues in all treated groups compared to the control group (***p < 0.001), as shown in Fig. 4. The expression of E-cadherin in the HIF-ihb group was greater than for the HIF-act group (*p < 0.05). There were no significant differences in E-cadherin expression between HIF-ihb/ALK5-ihb and HIF-act/ALK5-ihb groups.
Fig. 4
The effect of HIF-1α and ALK5 signaling pathways on E-cadherin expression in a mouse melanoma model. E-cadherin expression was significantly reduced in all treated groups compared with the control group (***p < 0.001). E-cadherin expression in the HIF-ihb group was higher than in the HIF-act group (*p < 0.05). Each bar represents mean±SEM. Alk5-ihb: ALK5 inhibitor, HIF-ihb: HIF-1α inhibitor, HIF-act: HIF-1α activator.
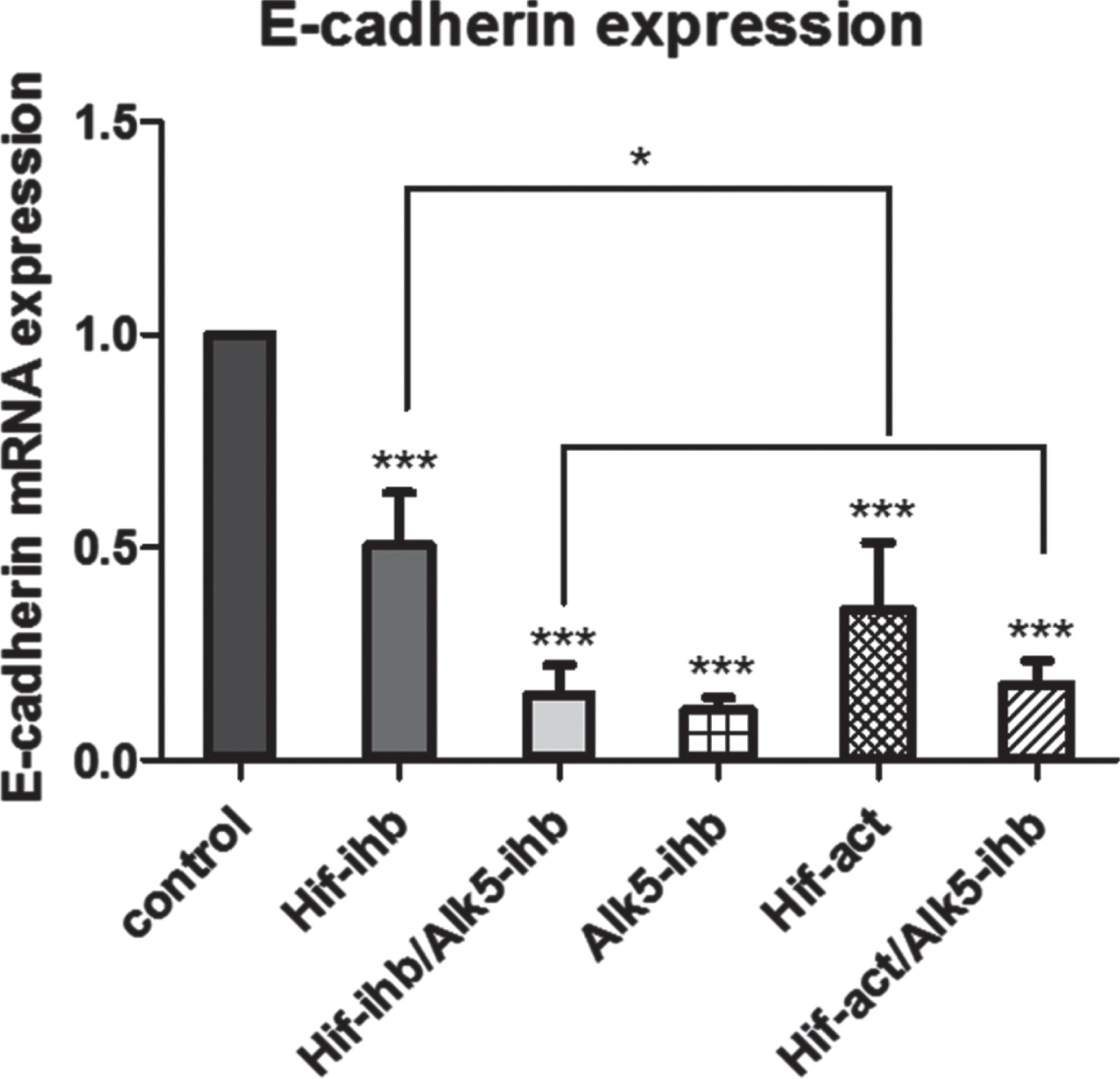
Vascular density was reduced in the HIF-ihb (***p < 0.001), HIF-ihb/ ALK5-ihb (***p < 0.001), and ALK5-ihb (***p < 0.001) groups. Capillary density was higher in the HIF-act group than the HIF-ihb group. Significantly the capillary density in HIF-act/ALK5-ihb group was more than the HIF-ihb/ALK5-ihb group (Fig. 5). The number of PDGFR-β+ cells around microvessels was reduced in mice receiving the TGF-βRI inhibitor. PDGFR-β+ cells were reduced in the HIF-ihb (***p < 0.001), HIF-ihb/ALK5-ihb (***p < 0.001), ALK5-ihb (***p < 0.001), and HIF-act/ALK5-ihb (***p < 0.001) groups. PDGFR-β+ cells recruitment significantly increased in HIF-act group in comparison to HIF-ihb. There was no significant difference in the recruitment of PDGFR-β+ cells between the HIF-ihb/ALK5-ihb and HIF-act/ALK5-ihb groups (Fig. 6). The immunohistochemical image depicting blood vessels is shown in Figs. 7 and 8.
Fig. 5
The effect of HIF-1α and ALK5 signaling pathways on the tumor vessel density in a mouse melanoma model. There was significantly lower vessel density in the HIF-ihb, HIF-ihb/alk5-ihb, and Alk-ihb groups than in the control group. Capillary density increased in the HIF-act group compared with the HIF-ihb. The capillary density in the HIF-act/Alk5-ihb group was higher than in the HIF-ihb/ Alk5-ihb group. (***p < 0.001) and (*p < 0.05). Each bar represents mean±SEM. Alk5-ihb: ALK5 inhibitor, HIF-ihb: HIF-1α inhibitor, HIF-act: HIF-1α activator.
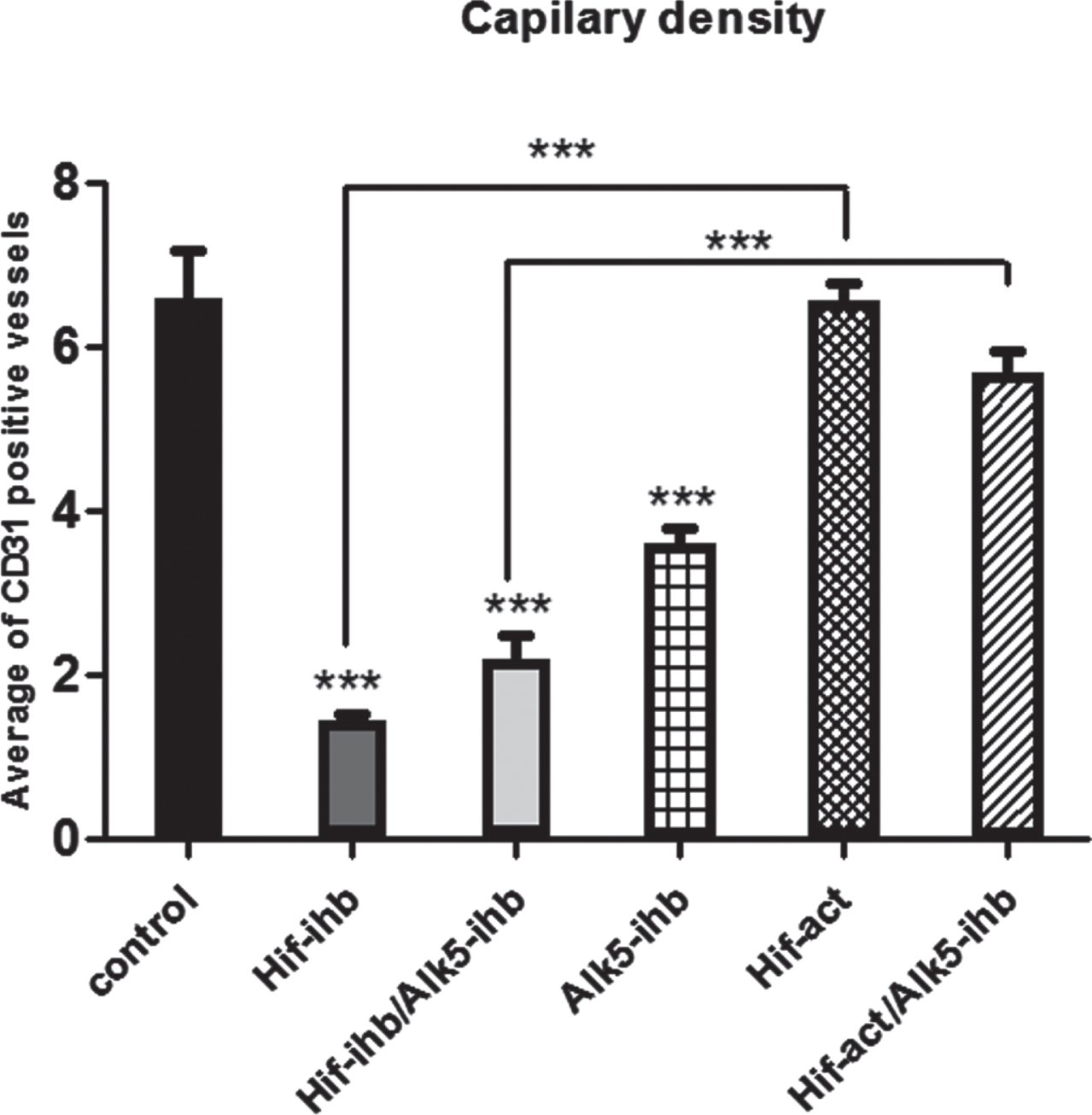
Fig. 6
Effect of HIF-1α and ALK5 signaling pathways on PDGFR-β+ cells around microvessels in a mouse melanoma model. The number of PDGFR-β+ cells around the vessels was significantly reduced in the groups that received HIF-1α inhibitor, Alk-5 inhibitor, both the HIF-1α and ALK5 inhibitors, and simultaneous HIF-1α activator and ALK5 inhibitor, compared with the control group. There was a significant increase in PDGFR-β+ cells surrounding the vessels in the HIF-act group in comparison to HIF-ihb (***p < 0.001). Each bar represents mean±SEM. Alk5-ihb: ALK5 inhibitor, HIF-ihb: HIF-1α inhibitor, HIF-act: HIF-1α activator.
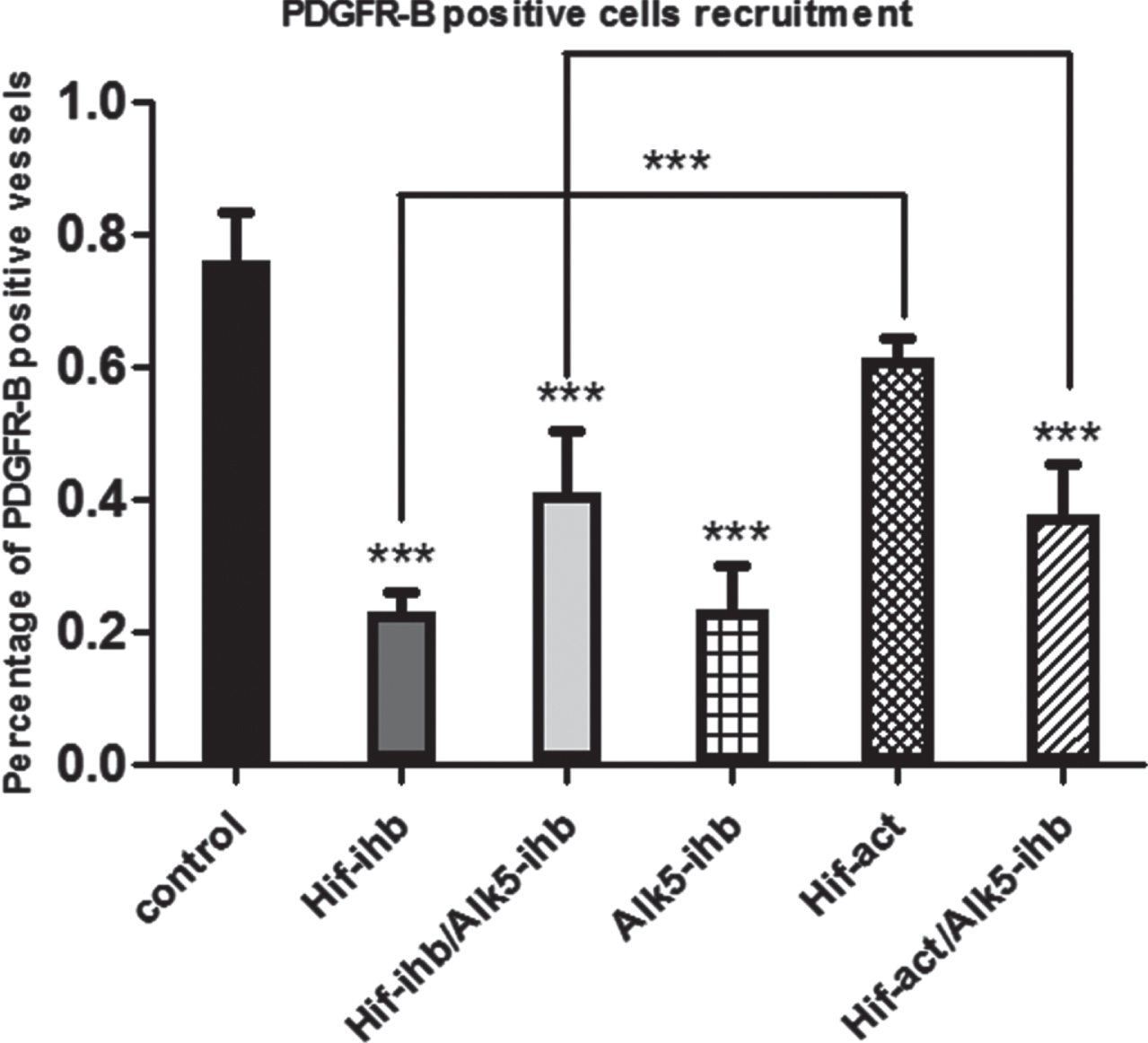
Fig. 7
Hematoxylin and eosin with anti-CD31 immunohistochemical staining of melanoma tumor tissue. Effect of HIF-1α and ALK5 signaling pathways on tumor vessel density in a mouse melanoma model between the control and treatment groups (40x magnification). Picture A represents CD31 staining in the control group. Pictures B, C, D, E, and F represent CD31 staining in HIF-ihb, HIF-ihb/Alk5-ihb, Alk5-ihb, HIF-act, and HIF-act/Alk5-ihb groups respectively. Alk5-ihb: ALK5 inhibitor, HIF-ihb: HIF-1α inhibitor, HIF-act: HIF-1α activator. Scale bar is 500 um.
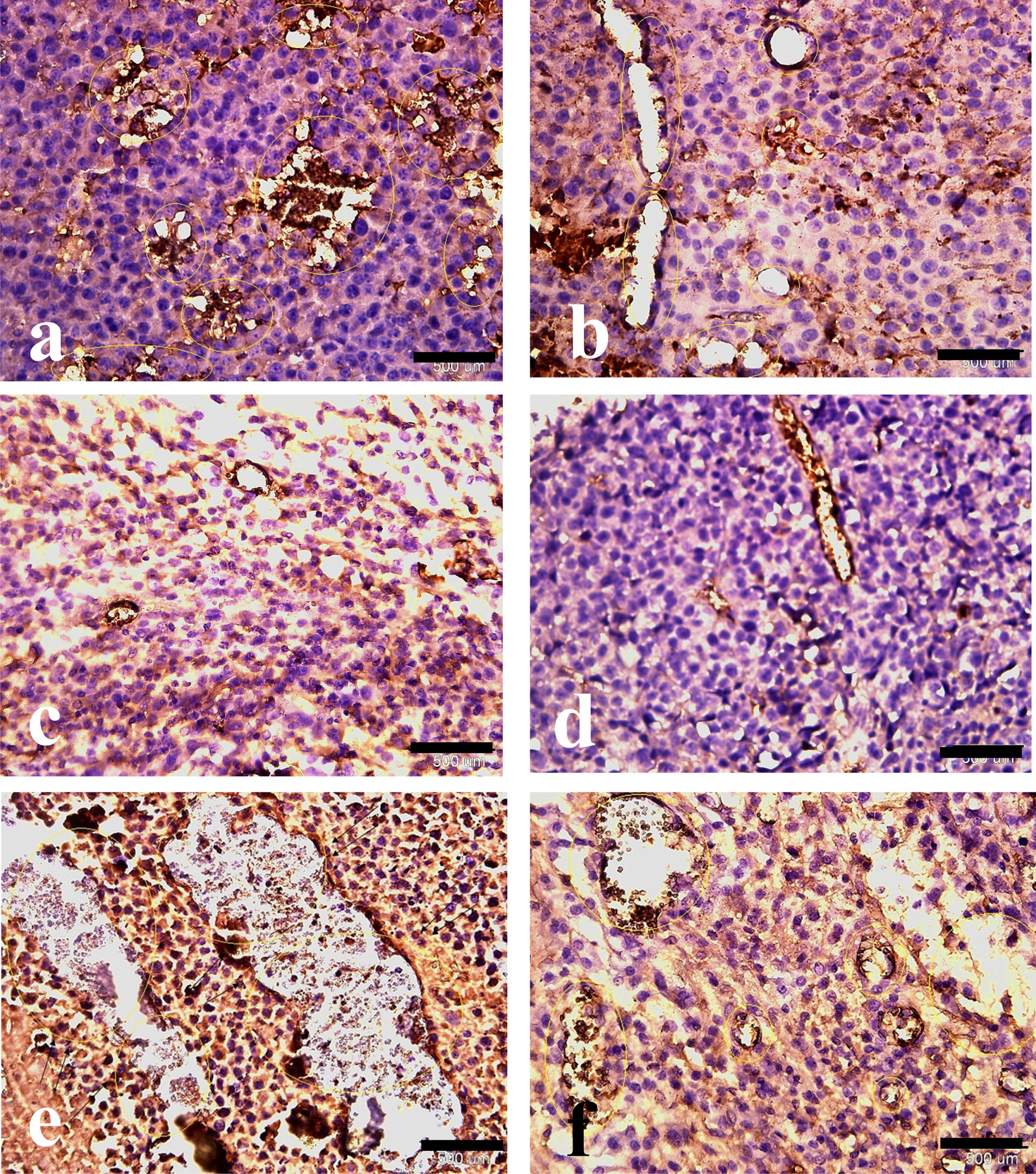
Fig. 8
Hematoxylin and eosin with anti-PDGFR immunohistochemical staining of melanoma tumor tissue. Effect of HIF-1α and ALK5 signaling pathways on pericyte recruitment in a mouse melanoma model between the control and treatment groups (40x magnification). Picture A represents PDGFR staining in the control group. Pictures B, C, D, E, and F represent PDGFR staining in HIF-ihb, HIF-ihb/Alk5-ihb, Alk5-ihb, HIF-act, and HIF-act/ Alk5-ihb groups, respectively. Alk5-ihb: ALK5 inhibitor, HIF-ihb: HIF-1α inhibitor, HIF-act: HIF-1α activator. Scale bar is 500 µm.
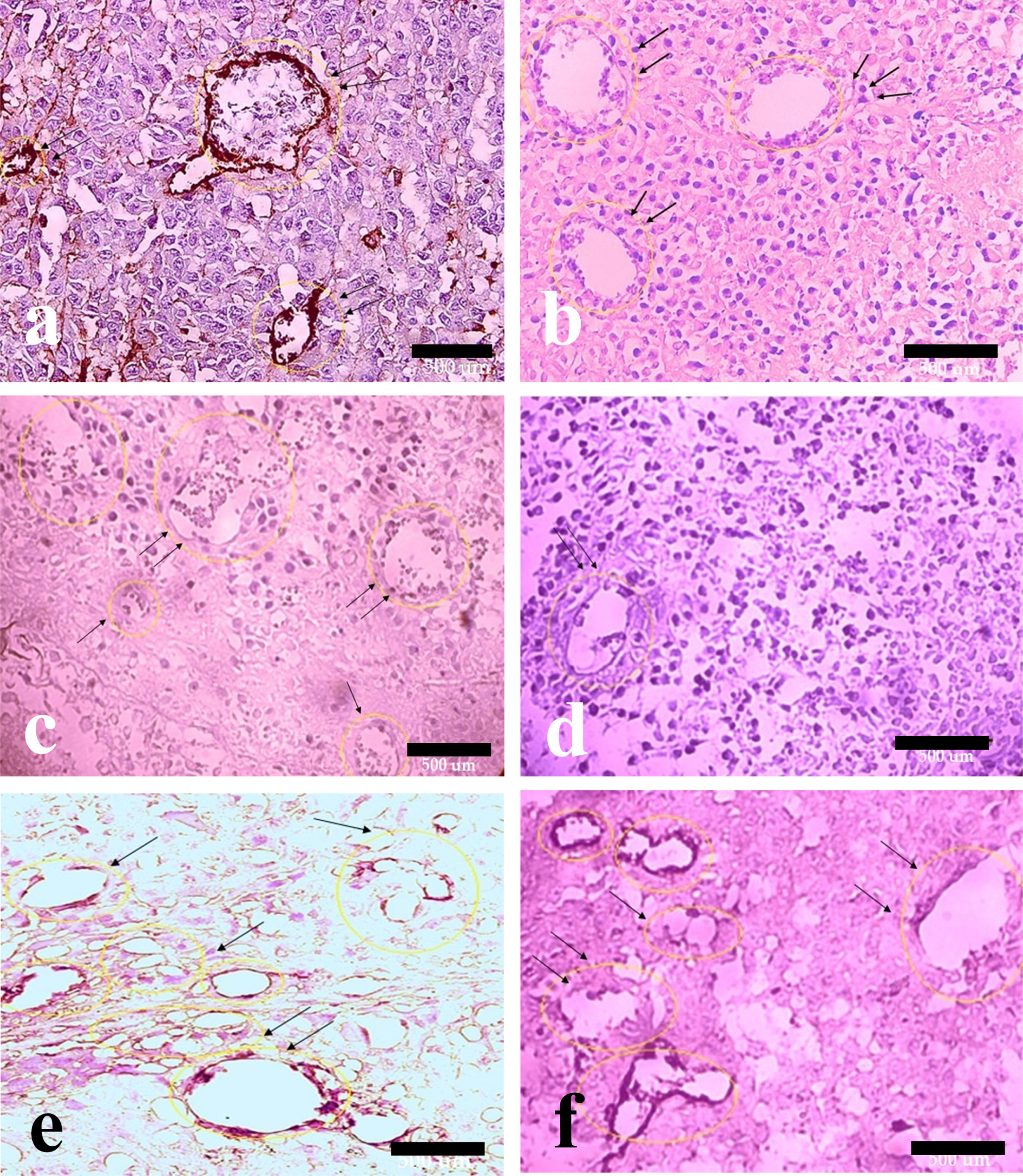
4Discussion
Malignant melanocyte-derived tumors, such as melanoma, typically affect the skin. In addition, melanoma can also develop in the meninges, on numerous mucosal surfaces, and in the eyes [36]. Being one of the most invasive tumors, malignant melanoma can metastasize to the lung, bone, brain, liver, and lymph nodes from the original tumor site [37].
Melanoma is particularly aggressive and different from other cancer types. For instance, melanoma cells are different due to being quite good at resisting the host immune system, although they are highly antigenic. Furthermore, they are more “stem-like” than other solid tumor cells, due to their strong angiogenicity, mesenchymal origin, and shared cell surface markers with vascular cells, and a different gene expression profile [37].
Melanoma treatment and diagnosis may be affected by how melanogenesis and melanin pigment alter the behavior of normal and malignant melanocytes [38, 39]. Although melanin is helpful for preventing the development of skin malignancies such as cutaneous melanoma, it may also be required for malignant transformation of melanocytes [40]. When melanogenesis is triggered in melanoma cells, there are significant enhancements in nuclear HIF-1 expression along with overexpression of other HIF-1 dependent genes that control response to stress, glucose metabolism, and angiogenesis [41].
Angiogenesis is a crucial stage in the development of almost all human malignancies. This study investigated the effects of ALK5 inhibition on the response to HIF-1α activation or inhibition on melanoma tumor growth, angiogenesis, and EMT. Previous studies have indicated the beneficial effects of the inhibition of TGF-β in animal studies of various types of cancer, and also in early-stage clinical trials, through its direct influence on the tumor microenvironment [42].
The effects of the cytokine TGF-β on tumor development are complex. It can both suppress and promote tumor growth by inhibiting tumor growth and promoting apoptosis, or enhancing epithelial to mesenchymal transition leading to cell invasion and metastasis, respectively [43]. The complex role of fibrosis in cancer progression is controversial and may be context dependent. Fibrosis may either restrain or enhance cancer progression through reprogramming of cancer stroma [44]. Interactions between TGF-β1 and HIF-1α signaling pathways play a critical role in fibrogenesis [45]. The synergistic inhibitory functions of targeting TGF-β1 and HIF-1α pathways in the progression of some other cancer types, such as renal cell carcinoma, have been reported [24]. However, in our study simultaneous inhibition of TGF-β1 and HIF-1α pathways led to enhanced tumor growth, potentially through reduction of the inhibitory effect of fibrosis on tumor progression. TGF-β has an antimitotic effect at low concentrations, but at higher concentrations melanoma tumor cells escape this effect, and indirectly create a favorable tumor microenvironment in several ways, including the promotion of neovascularization [46]. Although the cues that lead to the interchange between tumor suppressor and tumor promoter roles of TGF-β are not fully understood, it is clear that ALK5 is necessary for the pro-metastatic activity of TGF-β [47]. TGF-β/ALK5 signaling phosphorylates Smad2 and Smad3, which form heteromeric complexes with the common partner (Co-Smad) Smad4, then translocate to the nucleus and participate in transcriptional regulation of target genes [48, 49].
Inhibitors of ALK5 antagonize TGF-β signaling by blocking TGF-β receptor-activated Smad (R-Smad) phosphorylation and inducing ubiquitin-mediated degradation of Smad4. For instance, one of the inhibitory effects of LY-364947 is reducing p-Smad2 and p-Smad3 which has been frequently tested and published in several papers [50–52].
Given that TGF-β plays a crucial role in the development of tumors, a number of drugs intended to block TGF-β signaling have been tested in both animal studies and human clinical trials; however, no success has yet been shown for clinical use. While ALK5 inhibition by YR-290 showed antitumor activity in breast cancer in animal models [53], a phase III trial of ALK5 inhibitor (LY-573636) in melanoma patients led to early closure of the study due to toxicity, with three deaths related to the drug (thrombocytopenia). The toxicity imbalance was attributed to an unexpectedly low tasisulam clearance in a subset of patients leading to drug accumulation, underscoring the importance of pharmacokinetic monitoring of compounds with complex dosing, even in late-phase studies leading to halting of the clinical trial [54]. While TGF-β signaling is mediated by the ALK5 receptor in most cell types, the effects of TGF-β in endothelial cells are mediated by both ALK5 and the endothelial cell restricted receptor ALK1. However, ALK1 (which stimulates EC migration and proliferation) and ALK5 (which inhibits both processes) signaling pathways appear to have opposite roles in vascular development, and studies in ALK5 deficient mice suggest that ALK5 is needed for TGF-β/ALK1 signaling [55]. One of the upstream regulators of TGF-β is HIF-1 which facilitates breast cancer progression [22]. Another study, however, claimed that HIF-1α might not be associated with TGF-β1 induction in gastric cancer cells under hypoxic conditions [56]. Therefore, in the current study, the effects of simultaneous ALK5 inhibition and HIF-1α activation or ALK5 inhibition and HIF-1α inhibition on tumor volume, PDGFR-β+ cells, and E-cadherin gene expression have been also investigated to better understand whether this interaction exists in melanoma or not. HIF-1α activation is implicated in tumor progression by inducing angiogenesis and promoting metastasis [57].
In our study, tumor volume significantly increased in all treated groups in which HIF-1α was activated, whether ALK5 was inhibited or not. Activated pericytes promote angiogenesis through the HIF pathway [58]. In our study, vessel density significantly increased in groups in which HIF-1α was activated. HIF-1α inhibition resulted in a decreased vessel density in comparison to the control group. Capillary density significantly decreased in the HIF-ihb group compared with the HIF-act group.
Our results showed that ALK5 inhibition led to significantly decreased numbers of PDGFR-β+ cells in the HIF-modified groups, whether activated or inhibited. Although HIF-1α inhibition significantly decreased the percentage of PDGFR-β+ cells around blood vessels, the potent effect of ALK5 inhibition on PDGFR-β+ cell recruitment, whether HIF is inhibited or activated, is controversial and noticeable. The proportion of PDGFR-β+ cells has been considerably reduced in the group that received HIF-1α activator compared to the control group. PDGFR-β+ cells surrounding the vessels significantly increased in the HIF-act group compared to HIF-ihb. PDGFR-β is a vital receptor on vessel-associated pericytes and fibroblasts, mediating fundamental processes in tumor progression [59]. PDGFR-β plays an essential role in the EMT of the epicardium [60]. Interestingly, in a study on EMT-derived breast epithelial cells, it has been shown that PDGFR-β, a marker for naive MSCs, is exclusively expressed in EMT-derived cells and not in their normal epithelial counterparts [61]. On the other hand, it has been shown that a subset of PDGFR-β+ progenitor perivascular cells is recruited from the bone marrow to perivascular sites in tumors to initiate tumor vasculogenesis [62].
In our study, E-cadherin gene expression was lower in all groups compared to the control group. Paradoxically, it seems to be an unreported outcome to date. According to our results, E-cadherin expression significantly decreased in the group receiving HIF activator. HIF-1α overexpression is correlated with enhanced expression of ZEB1 and decreased E-cadherin expression contributing to the EMT process [63]. Stabilization and overexpression of HIF decrease the expression of E-cadherin and may promote cancer metastasis, as we observed that the tumor growth increased after HIF-1α activation when compared with HIF-1α inhibition [57]. Even though the prevailing belief is that E-cadherin overexpression prevents motility, and invasion, and its reduction is a fundamental prerequisite for the development of metastatic cancer, our results show that its reduction is not always associated with cancer progression. For instance, decreased expression of E-cadherin after inhibition of ALK5 and HIF-1α was not correlated with increased tumor growth. In line with our results, in breast cancer, enhanced E-cadherin concentrations have been reported in proliferating cancer cells in extremely invasive, dedifferentiated, metastatic cancers, and tumors with epithelial-mesenchymal transition, and hence with poor prognosis [64]. This may suggest that E-cadherin does not just have a metastatic suppressor role.
Therefore, the E-cadherin paradoxical role could be mentioned as an impact of the tumor microenvironment, thus showing how context-dependent circumstances can drive molecular functions alongside alternative routes.
Although assessment of E-cadherin expression levels is one of the most important ways for the evaluation of EMT, measuring other EMT-related factors like Claudin-1 could help to achieve more precise results in the TGF- β/HIF-1α-EMT axis, and not measuring Claudin-1 and E-cadherin protein levels is one of the limitations of our study.
The beneficial impacts of HIF inhibition have been previously demonstrated in pre-clinical mouse models. HIF-1α is a crucial element in worsening the transformation of regular melanocytes into melanoma [65, 66]. HIF-1α overexpression in primary melanoma and its metastases has a strong positive association with the intensity of malignancy and poor prognosis. It has also a direct supporting effect on tumor angiogenesis through VEGF. HIF-1 prevents the expression of E-cadherin, as a key step of EMT, followed by a rise in mesenchymal markers and cell motility [67]. According to our results, E-cadherin expression was highly expressed in the HIF-ihb group compared with HIF-act which is consistent with the results of other experiments in which HIF activation down-regulates E-cadherin expression. ALK5 inhibition was significantly effective in reducing E-cadherin expression. Furthermore, ALK5 inhibition may weaken the effect of HIF-inhibition to increase E-cadherin expression. On the other hand, TGF-β has become one of the most essential elements of EMT promotion. As a consequence of their mutual transcription stimulation in numerous kinds of cancer cells, elevated levels of both HIF-1 and TGF-β enhance EMT caused by hypoxia [68]. A main synergistic interaction exists between the TGF-β signaling and hypoxia pathway, which may facilitate tumor progression through ALK5 and HIF-α interaction [24].
As we have not observed any synergistic effects for the inhibition of none of the two signaling pathways, it seems necessary to investigate this in different cancer cell lines, with different inhibitors. Indeed, the most important limitation of our study was not performing the experiments on cell lines other than melanoma cells. Lacking the ability to simulate the complex process of tumorigenesis may be a major limitation of almost all animal studies.
5Conclusion
The tumor promoting effect of HIF1-α activation, even when ALK5 was inhibited, was demonstrated in this study, raising significant concerns regarding not only the status of the TGF-β pathway as a tumor growth promoter, but also the crosstalk between TGF-β and other active pathways, such as HIF1-α in the tumor microenvironment. Our results suggest that modification of TGF-β signaling would be a better strategy for cancer treatment. In addition, simultaneous inhibition of TGF-β and HIF-1α signaling pathways did not show significant synergistic inhibitory effects on melanoma progression warranting further experiments on other cancer cell lines.
Acknowledgments
We would like to express our gratitude to the members of the regenerative medicine research center (RMRC), Applied physiology research center (APRC), Isfahan University of medical sciences, and Prof. Gordon A Ferns and Mrs. Maryam Vatani for reviewing and improving the text.
Author contributions
Conception: SHJ.
Data curation: BZ.
Analysis of data: SHJ.
Preparation of the manuscript: BZ.
Revision for important intellectual content: RN, IL, and MM.
Supervision: SHJ.
Conflict of interest
The authors declare no conflict of interest.
Ethical considerations
All procedures were performed in accordance with the ethical standards of the local ethics committee of Isfahan University of Medical Sciences based on the NIH guidelines in the design of all protocols.
Funding
This study was funded by Isfahan University of medical sciences.
References
[1] | Bandarchi B , Jabbari CA , Vedadi A , Navab R . Molecular biology of normal melanocytes and melanoma cells. J Clin Pathol. (2013) ;66: (8):644–8. doi: 10.1136/jclinpath-2013-201471. |
[2] | Bhatt RS , Atkins MB . Molecular pathways: Can activin-like kinase pathway inhibition enhance the limited efficacy of VEGF inhibitors? Clin Cancer Res. (2014) ;20: (11):2838–45. doi: 10.1158/1078-0432.CCR-13-2788. |
[3] | Vecchia L , Olivieri C , Scotti C . Activin receptor-like kinase A novel anti-angiogenesis target from TGF-β family. Mini-Reviews Med Chem. (2013) ;13: (10):1398–406. doi: 10.2174/13895575113139990065. |
[4] | Quaresmini D , Guida M . Neoangiogenesis in melanoma: An issue in biology and systemic treatment. Frontiers in Immunology. (2020) ;11: :584903. doi: 10.3389/fimmu.2020.584903. |
[5] | Corrie PG , Basu B , Ahmad Zaki K . Targeting angiogenesis in melanoma: Prospects for the future. Therapeutic Advances in Medical Oncology. (2010) ;2: (6):367–80. doi: 10.1177/1758834010380101. |
[6] | Kodama S , Podyma-Inoue KA , Uchihashi T , Kurioka K , Takahashi H , Sugauchi A , et al. Progression of melanoma is suppressed by targeting all transforming growth factor-β isoforms with an Fc chimeric receptor. Oncol Rep. (2021) ;46: (3):1–13. doi: 10.3892/or.2021.8148. |
[7] | Javelaud D , Alexaki VI , Mauviel A . Transforming growth factor-β in cutaneous melanoma. Pigment Cell Melanoma Res. (2008) ;21: (2):123–32. doi: 10.1111/j.1755-148X.2008.00450.x. |
[8] | Guerrero PA , McCarty JH . TGF-β activation and signaling in angiogenesis. Physiologic and Pathologic Angiogenesis-Signaling Mechanisms and Targeted Therapy. 2017. doi: 10.5772/66405. |
[9] | Orlova VV , Liu Z , Goumans MJ , ten Dijke P . Controlling angiogenesis by two unique TGF-β type I receptor signaling pathways. Histology and Histopathology. (2011) ;26: (9). doi: 10.14670/HH-26.1219. |
[10] | Jakowlew SB . Transforming growth factor-β in cancer and metastasis. Cancer and Metastasis Reviews. (2006) ;25: (3):435–57. doi: 10.1007/s10555-006-9006-2. |
[11] | Wakefield LM , Roberts AB . TGF-β signaling: Positive and negative effects on tumorigenesis. Current Opinion in Genetics and Development. Elsevier Ltd; Vol. 12, 2002, pp. 22-9. doi: 10.1016/s0959-437x(01)00259-3. |
[12] | Husain SM , Kansal R , Alvarez MA , Glazer ES . TGF-beta blockade paradoxically activates non-SMAD signaling. Cancer Res. (2019) ;79: (24 Supplement):C18–C18. doi: 10.1158/1538-7445.PANCA19-C18. |
[13] | Neel J-C , Humbert L , Lebrun J-J . The dual role of TGFβ in human cancer: From tumor suppression to cancer metastasis. ISRN Mol Biol. (2012) ;2012: :1–28. doi: 10.5402/2012/381428. |
[14] | Howley BV , Howe PH . TGF-beta signaling in cancer: Post-transcriptional regulation of EMT via hnRNP E1. Cytokine. Academic Press; 2019, Vol. 118, pp. 19-26. doi: 10.1016/j.cyto.2017.12.032. |
[15] | Perrot CY , Javelaud D , Mauviel A . Insights into the transforming growth factor-β signaling pathway in cutaneous melanoma. Annals of Dermatology. (2013) ;25: :135–44. doi: 10.5021/ad.2013.25.2.135. |
[16] | Cho ES , Kang HE , Kim NH , Yook JI . Therapeutic implications of cancer epithelial-mesenchymal transition (EMT). Archives of Pharmacal Research. (2019) ;42: (1):14–24. doi: 10.1007/s12272-018-01108-7. |
[17] | Wang B , Liu T , Wu JC , Luo SZ , Chen R , Lu LG , Xu MY . STAT3 aggravates TGF-β1-induced hepatic epithelial-to-mesenchymal transition and migration. Biomedicine & Pharmacotherapy. (2018) ;98: :214–21. doi: 10.1016/j.biopha.2017.12.035. |
[18] | Ou XW , Wang RX , Kang MF , Shi JQ . Hypoxia promotes migration and invasion of gastric cancer cells by activating HIF-1α and inhibiting NDRG2 associated signaling pathway. Eur Rev Med Pharmacol Sci. (2018) ;22: (23):8237–47. doi: 10.26355/eurrev_201812_16518. |
[19] | Dunn LK , Mohammad KS , Fournier PGJ , McKenna CR , Davis HW , Niewolna M , et al. Hypoxia and TGF-β drive breast cancer bone metastases through parallel signaling pathways in tumor cells and the bone microenvironment. PLoS One. (2009) ;4: (9). doi: 10.1371/journal.pone.0006896. |
[20] | Malekan M , Ebrahimzadeh MA , Sheida F . The role of Hypoxia-Inducible Factor-1alpha and its signaling in melanoma. Biomed Pharmacother. (2021) ;141: :111873. doi: 10.1016/j.biopha.2021.111873. |
[21] | Unwith S , Zhao H , Hennah L , Ma D . The potential role of HIF on tumour progression and dissemination. International Journal of Cancer. (2015) ;136: (11):2491–503. doi: 10.1002/ijc.28889. |
[22] | Peng J , Wang X , Ran L , Song J , Luo R , Wang Y . Hypoxia-inducible factor 1α regulates the transforming growth factor β1/ SMAD family member 3 pathway to promote breast cancer progression. J Breast Cancer. (2018) ;21: (3):259–66. doi: 10.4048/jbc.2018.21.e42. |
[23] | Fallah J , Rini BI . HIF inhibitors: Status of current clinical development. Current Oncology Reports. (2019) ;21: (1):1–0. doi: 10.1007/s11912-019-0752-z. |
[24] | Mallikarjuna P. T , Sitaram R , Aripaka K , Ljungberg B , Landström M . Interactions between TGF-β type I receptor and hypoxia-inducible factor-α mediates a synergistic crosstalk leading to poor prognosis for patients with clear cell renal cell carcinoma. Cell Cycle. (2019) ;18: (17):2141–56. doi: 10.1080/15384101.2019.1642069. |
[25] | Mallikarjuna P , Zhou Y , Landström M . The synergistic cooperation between TGF-β and hypoxia in cancer and fibrosis. Biomolecules. (2022) ;12: (5):635. doi: 10.3390/biom12050635. |
[26] | Fallah A , Sadeghinia A , Kahroba H , Samadi A , Heidari HR , Bradaran B , et al. Therapeutic targeting of angiogenesis molecular pathways in angiogenesis-dependent diseases. Biomedicine & Pharmacotherapy. (2019) ;110: :775–85. doi: 10.1016/j.biopha.2018.12.022. |
[27] | Ribatti D , Annese T , Ruggieri S , Tamma R , Crivellato E . Limitations of anti-angiogenic treatment of tumors. Translational Oncology. (2019) ;12: (7):981–6. doi: 10.1016/j.tranon.2019.04.022. |
[28] | Petrova V , Annicchiarico-Petruzzelli M , Melino G , Amelio I . The hypoxic tumour microenvironment. Oncogenesis. (2018) ;7: (1):1–3. doi: 10.1038/s41389-017-0011-9. |
[29] | Hung SP , Yang MH , Tseng KF , Lee OK . Hypoxia-induced secretion of TGF-β1 in mesenchymal stem cell promotes breast cancer cell progression. Cell transplantation. (2013) ;22: (10):1869–82. doi: 10.3727/096368912X657954. |
[30] | Oka M , Iwata C , Suzuki HI , Kiyono K , Morishita Y , Watabe T , et al. Inhibition of endogenous TGF-2 signaling enhances lymphangiogenesis. Blood. (2008) ;111: (9):4571–9. doi: 10.1182/blood-2007-10-120337. |
[31] | Peng SB , Yan L , Xia X , Watkins SA , Brooks HB , Beight D , et al. Kinetic characterization of novel pyrazole TGF-β receptor I kinase inhibitors and their blockade of the epithelial-mesenchymal transition. Biochemistry. (2005) ;44: (7):2293–304. doi: 10.1021/bi048851x. |
[32] | Kang SH , Cho HT , Devi S , Zhang Z , Escuin D , Liang Z , et al. Antitumor effect of 2-methoxyestradiol in a rat orthotopic brain tumor model. Cancer Res. (2006) ;66: (24):11991–7. doi: 10.1158/0008-5472.CAN-06-1320. |
[33] | Chan MC , Atasoylu O , Hodson E , Tumber A , Leung IK , Chowdhury R , et al. Potent and selective triazole-based inhibitors of the hypoxia-inducible factor prolyl-hydroxylases with activity in the murine brain. PLoS One. (2015) ;10: (7):e0132004. doi: 10.1371/journal.pone.0132004. eCollection 2015. |
[34] | Nedaeinia R , Sharifi M , Avan A , Kazemi M , Nabinejad A , Ferns GA , et al. Inhibition of microRNA-21 via locked nucleic acid-anti-miR suppressed metastatic features of colorectal cancer cells through modulation of programmed cell death 4. Tumor Biology. (2017) ;39: (3):1010428317692261. doi: 10.1177/1010428317692261. |
[35] | Zarrin B , Javanmard SH , Samani F , Tajadini M , Jamady M , Esmaeli N , et al. The effect of duloxetine on tau protein and migration in breast cancer cell line. Int J Cancer Manag. (2017) ;10: (5). doi: 10.5812/ijcm.6422. |
[36] | Ali Z , Yousaf N , Larkin J . Melanoma epidemiology, biology and prognosis. EJC Suppl. (2013) ;11: (2):81–91. doi: 10.1016/j.ejcsup.2013.07.012. |
[37] | Braeuer RR , Watson IR , Wu CJ , Mobley AK , Kamiya T , Shoshan E , et al. Why is melanoma so metastatic? Pigment Cell Melanoma Res. (2014) ;27: (1):19–36. doi: 10.1111/pcmr.12172. |
[38] | Brozyna AA , Jóźwicki W , Roszkowski K , Filipiak J , Slominski AT , Brozyna AA . et al. Melanin content in melanomametastases affects the outcome of radiotherapy. Oncotarget. (2016) ;7: (14):17844–53. doi: 10.18632/oncotarget.7528. |
[39] | Liu J , Fukunaga-Kalabis M , Li L , Herlyn M . Developmental pathways activated in melanocytes and melanoma. Arch Biochem Biophys. (2014) ;0: :13. doi: 10.1016/j.abb.2014.07.023. |
[40] | Slominski RM , Sarna T , Płonka PM , Raman C , Brozyna AA , Slominski AT . Melanoma, melanin, and melanogenesis: The yin and yang relationship. Front Oncol. (2022) ;12: :377. doi: 10.3389/fonc.2022.842496. |
[41] | Slominski A , Kim TK , Brozyna AA , Janjetovic Z , Brooks DLP , Schwab LP , et al. The role of melanogenesis in regulation of melanoma behavior: Melanogenesis leads to stimulation of HIF-1α expression and HIF-dependent attendant pathways. Arch Biochem Biophys. (2014) ;563: :79. doi: 10.1016/j.abb.2014.06.030. |
[42] | Pickup M , Novitskiy S , Moses HL . The roles of TGFβ in the tumour microenvironment. Nature Reviews Cancer. (2013) ;13: :788–99. doi: 10.1038/nrc3603. |
[43] | Principe DR , Doll JA , Bauer J , Jung B , Munshi HG , Bartholin L , et al. TGF-β: Duality of function between tumor prevention and carcinogenesis. Journal of the National Cancer Institute. (2014) ;106: . doi: 10.1093/jnci/djt369. |
[44] | Chandler C , Liu T , Buckanovich R , Coffman LG . The double edge sword of fibrosis in cancer. Transl Res. (2019) ;209: :55–67. doi: 10.1016/j.trsl.2019.02.006. |
[45] | Basu RK , Hubchak S , Hayashida T , Runyan CE , Schumacker PT , Schnaper HW . Interdependence of HIF-1α and TGF-β3/Smad3 signaling in normoxic and hypoxic renal epithelial cell collagen expression. Am J Physiol - Ren Physiol. (2011) ;300: (4):898–905. doi: 10.1152/ajprenal.00335.2010. |
[46] | Lebrin F , Deckers M , Bertolino P , Ten Dijke P . TGF-β receptor function in the endothelium. Cardiovascular Research. (2005) ;65: :599–608. doi: 10.1016/j.cardiores.2004.10.036. |
[47] | Schniewind B , Groth S , Sebens Müerköster S , Sipos B , Schäfer H , Kalthoff H , et al. Dissecting the role of TGF-beta type I receptor/ALK5 in pancreatic ductal adenocarcinoma: Smad activation is crucial for both the tumor suppressive and prometastatic function. Oncogene. (2007) ;26: (33):4850–62. doi: 10.1038/sj.onc.1210272. |
[48] | Hata A , Chen YG . TGF-β signaling from receptors to Smads. Cold Spring Harbor Perspectives in Biology. (2016) ;8: (9):a022061. doi: 10.1101/cshperspect.a022061. |
[49] | Massagué J . TGF-β signal transduction. Annu Rev Biochem. (1998) ;67: (1):753–91. doi: 10.1146/annurev.biochem.67.1.753. |
[50] | Yoon JH , Jung SM , Park SH , Kato M , Yamashita T , Lee IK , et al. Activin receptor-like kinase5 inhibition suppresses mouse melanoma by ubiquitin degradation of Smad4, thereby derepressing eomesodermin in cytotoxic T lymphocytes. EMBO Mol Med. (2013) ;5: (11):1720–39. doi: 10.1002/emmm.201302524. |
[51] | Karkampouna S , Goumans MJ , ten Dijke P , Dooley S , Kruithof-de Julio M . Inhibition of TGFβ type I receptor activity facilitates liver regeneration upon acute CCl4 intoxication in mice. Arch Toxicol. (2016) ;90: (2):347–57. doi: 10.1007/s00204-014-1436-y. |
[52] | Wang P , Karakose E , Liu H , Swartz E , Ackeifi C , Zlatanic V , et al. Combined inhibition of DYRK1A, SMAD, and trithorax pathways synergizes to induce robust replication in adult human beta cells. Cell Metab. (2019) ;29: (3):638–52.e5. doi: 10.1016/j.cmet.2018.12.005. |
[53] | Fang Y , Chen Y , Yu L , Zheng C , Qi Y , Li Z , et al. Inhibition of breast cancer metastases by a novel inhibitor of TGFβ receptor 1. JNCI J Natl Cancer Inst. (2013) ;105: (1):47–58. doi: 10.1093/jnci/djs485. |
[54] | Hamid O , Ilaria R , Garbe C , Wolter P , Maio M , Hutson TE , et al. A randomized, open-label clinical trial of tasisulam sodium versus paclitaxel as second-line treatment in patients with metastatic melanoma. Cancer. (2014) ;120: (13):2016–24. doi: 10.1002/cncr.28635. |
[55] | Goumans MJ , Valdimarsdottir G , Itoh S , Lebrin F , Larsson J , Mummery C , et al. Activin receptor-like kinase (ALK)1 is an antagonistic mediator of lateral TGFβ/ALK5 signaling. Mol Cell. (2003) ;12: (4):817–28. doi: 10.1016/s1097-2765(03)00386-1. |
[56] | Deng B , Zhu JM , Wang Y , Liu TT , Ding YB , Xiao WM , et al. Intratumor hypoxia promotes immune tolerance by inducing regulatory T cells via TGF-β1 in gastric cancer. PLoS One. (2013) ;8: (5). doi: 10.1371/journal.pone.0063777. |
[57] | Emami Nejad A , Najafgholian S , Rostami A , Sistani A , Shojaeifar S , Esparvarinha M , Nedaeinia R , Haghjooy Javanmard S , Taherian M , Ahmadlou M , Salehi R . The role of hypoxia in the tumor microenvironment and development of cancer stem cell: A novel approach to developing treatment. Cancer Cell International. (2021) ;21: (1):1–26. doi: 10.1186/s12935-020-01719-5. |
[58] | Mayo JN , Bearden SE . Driving the hypoxia-inducible pathway in human pericytes promotes vascular density in an exosome-dependent manner. Microcirculation. (2015) ;22: (8):711–23. doi: 10.1111/micc.12227. |
[59] | Kinugasa Y , Matsui T , Takakura N . CD44 expressed on cancer-associated fibroblasts is a functional molecule supporting the stemness and drug resistance of malignant cancer cells in the tumor microenvironment. Stem Cells. (2014) ;32: (1):145–56. doi: 10.1002/stem.1556. |
[60] | Mellgren A , Huang G , Tallquist M . Platelet derived growth factorreceptor beta function in epithelial to mesenchymal transition ofthe epicardium. (2006) ;114: (suppl_18). doi: 10.1161/circ.114.suppl_18.II_183-a. |
[61] | Battula VL , Evans KW , Hollier BG , Shi Y , Marini FC , Ayyanan A , et al. Epithelial-mesenchymal transition-derived cells exhibit multilineage differentiation potential similar to mesenchymal stem cells. Stem Cells. (2010) ;28: (8):1435–45. doi: 10.1002/stem.467. |
[62] | Song S , Ewald AJ , Stallcup W , Werb Z , Bergers G . PDGFRβ+ perivascular progenitor cells in tumours regulate pericyte differentiation and vascular survival. Nat Cell Biol. (2005) ;7: (9):870–9. doi: 10.1038/ncb1288. |
[63] | Zhang W , Shi X , Peng Y , Wu M , Zhang P , Xie R , et al. HIF-1α promotes epithelial-mesenchymal transition and metastasis through direct regulation of ZEB1 in colorectal cancer. PLoS One. (2015) ;10: (6). doi: 10.1371/journal.pone.0129603. |
[64] | Chu K , Boley KM , Moraes R , Barsky SH , Robertson FM . The paradox of E-cadherin: Role in response to hypoxia in the tumor microenvironment and regulation of energy metabolism. Oncotarget. (2013) ;4: (3):446–62. |
[65] | Shay JES , Imtiyaz HZ , Sivanand S , Durham AC , Skuli N , Hsu S , et al. Inhibition of hypoxia-inducible factors limits tumor progression in a mouse model of colorectal cancer. Carcinogenesis. (2014) ;35: (5):1067–77. doi: 10.1093/carcin/bgu004. |
[66] | Nys K , Maes H , Dudek AM , Agostinis P . Uncovering the role of hypoxia inducible factor-1α in skin carcinogenesis. Biochimica et Biophysica Acta (BBA)-Reviews on Cancer. (2011) ;1816: (1):1–2. doi: 10.1016/j.bbcan.2011.02.001. |
[67] | Krishnamachary B , Zagzag D , Nagasawa H , Rainey K , Okuyama H , Baek JH , et al. Hypoxia-inducible factor-1-dependent repression of E-cadherin in von Hippel-Lindau tumor suppressor-null renal cell carcinoma mediated by TCF3, ZFHX1A, and ZFHX1B. Cancer Res. (2006) ;66: (5):2725–31. doi: 10.1158/0008-5472.CAN-05-3719. |
[68] | Mcmahon S , Charbonneau M , Grandmont S , Richard DE , Dubois CM . Transforming growth factor 1 induces hypoxia-inducible factor-1 stabilization through selective inhibition of PHD2 expression. Journal of Biological Chemistry. (2417) ;281: (34):1–81. doi: 10.1074/jbc.M604507200. |