A review on the role of CAFs and CAF-derived exosomes in progression and metastasis of digestive system cancers
Abstract
Cancers evolve as a result of the accelerated proliferation of cancer cells in a complicated, enriched, and active microenvironment. Tumor microenvironment (TME) components are the master regulators of any step of cancer development. The tumor microenvironment is composed of many cellular and noncellular components that contribute to the evolution of cancer cells. Cancer-associated fibroblasts (CAFs) are activated fibroblasts in the TME that implicate in tumor progression and metastasis dissemination through secretion of oncogenic factors which are carried to the secondary metastatic sites through exosomes. In this review, we aimed to assess the role of CAF-derived exosomes in TME construction and pre-metastatic niche formation in different cancers of the digestive system in order to better understand some important mechanisms of metastasis and provide possible targets for clinical intervention. This review article is divided into two thematic parts explaining the general mechanisms of pre-metastatic niche formation and metastasis and the role of CAF-derived exosomes in different digestive system cancers including colorectal, gastric, esophageal, pancreatic, and liver cancers.
Abbreviations
ANXA6/LRP1/TSP1 | Annexin A6/LDL receptor-related protein 1/thrombospondin 1 |
BMDCs | Bone marrow-derived cells |
CAB-39 | Calcium-binding protein 39 |
CAFs | Cancer-associated fibroblasts |
CCA | Cholangiocarcinoma |
CM | Conditioned medium |
CRC | Colorectal cancer |
CSCs | Cancer stem cells |
ECM | Extracellular matrix |
EVs | Extracellular vesicles |
EMT | Epithelial-to-mesenchymal transition |
ESCC | Esophageal squamous cell carcinoma |
FGF | Fibroblast growth factor |
GC | Gastric cancer |
GEM | Gemcitabine |
GP130 | Glycoprotein 130 |
HCC | Hepatocellular carcinoma |
HGF | Hepatocyte growth factor |
IGF-2 | Insulin-like growth factor 2 |
lncRNA | Long non-coding RNA |
LncRNA CCAL | Colorectal cancer-associated lncRNA |
MDSCs | Myeloid-derived suppressor cells |
miRNAs | Micro RNAs |
MMPs | Matrix metalloproteinases |
MSCs | Mesenchymal stem cells |
ncRNA | Non-coding RNA |
NFs | Normal fibroblasts |
RAB14 | Ras-related protein 14 |
ROS | Reactive oxygen species |
SDF1 | Stromal derived factor |
SHH | Sonic hedgehog |
STAT3 | Signal transducer and activator of transcription 3 |
TDSFs | Tumor-derived secreted factors |
TNF-α | Tumor necrosis factor alpha |
TGFβ | Transforming growth factor-β |
TME | Tumor microenvironment |
VEGF-A | Vascular endothelial growth factor |
αSMA | Alpha smooth muscle actin |
5-Fu | 5-fluorouracil |
1Background
The main cause of about 90%of all cancer deaths is metastasis rather than primary tumors. The cancers of the digestive system are among the most significant healthcare concerns worldwide [1, 2].
More than 70%of gastrointestinal (GI) cancers have been reported with noticeable metastatic state, and appropriate treatment strategies of metastases are significant clinical concern as compared to primary tumor therapy [3]. Metastasis is managed by multiple factors and the role of tumor microenvironment (TME) is of vital importance. An active tumor microenvironment is a crucial hallmark of cancer progression and has numerous components that release enormous substantial signaling factors to facilitate tumor growth and metastasis [4].
By activating multiple signaling pathways and providing metastatic niche, TME components participate in various steps of cancer development including initiation, progression and metastasis of many cancers [1, 5]. Cancer associated fibroblasts (CAFs) comprise the majority of the tumor microenvironment components and mostly support the progression of tumor through secretion of different tumor-promoting factors and regulation of the interactions between tumor cells and microenvironment components. Since CAFs support the progression of gastrointestinal cancers by activating several tumor promoting mechanisms such as remodeling of matrices and drug resistance, these stromal cells are valuable targets to limit tumor growth [6]. Both tumor cells and stromal cells produce signal transmitting exosomes to help tumor expansion. The courage of metastatic distribution is provoked by exosome-managed alterations in TME and oncogenic signal transductions [7].
The exosomes produced by GI cancer cells foster cancer development and can be used as biomarkers for assessment of disease stage and the response to therapy [8]. Exosomes from tumor cells dictate activation of fibroblasts that release many cytokines to reinforce the progression of the disease [6]. While, exosomes released from CAFs transfer vital signals to increase their survival and invasiveness.
By means of exsomes, CAFs are implicated in the transduction of signals to the recipient cells to change their functions. In fact, CAFs generate exosomes to reciprocally interact with tumor cells and other TME components in order to create an enriched TME for tumor progression. The release of exosomes into the TME and blood circulation induces distant metastasis [9]. The present review aimed to assess the role of CAF-derived exosomes in TME construction and pre-metastatic niche formation. This review represents a summary of recent studies focusing on the function of CAFs, the most prominent component of TME, in progression of different digestive system cancer types, since it is becoming clear that exosome secretion from tumor fibroblasts may promote cancer progression and metastasis providing novel targets for clinical intervention.
2Factors involved in cancer metastasis
2.1Tumor microenvironment
TME, a master mediator of tumorigenesis, is a complex combination of extracellular matrix (ECM) components and several types of cells, such as tumor cells, immune cells, and cancer associated fibroblasts (CAFs), contributing to tumor initiation, progression and metastatic spread [10, 11]. The tumor growth is deeply affected by interactions between cancer cells and their surrounded environment, which eventually determines primary tumor eradication and metastasis. The disease progression is regulated either negatively or positively by TME-tumor cells interactions and it significantly depends on the stage of the tumor [12]. At the early stage, TME reflects anti-tumor functions that inhibits tumor progression but cancer cells adjust to the limitations, as disease progresses and TME switches to a pro-tumor state [13]. During cancer progression, residential immune cells in the TME do not produce a normal immune response, and these functionally defective components often inhibit immune response against tumor. Recruitment of inhibitory immune cells such as regulatory T cells and myeloid-derived suppressor cells (MDSCs) and alteration of immune cell phenotypes are the major strategies that tumors use to escape the immune response and support tumor progression [13]. One possible mechanism for immune evasion is regulated by CAFs. In fact, in order to boost tumor expansion, CAFs inhibit immune responses toward tumor cells [13].
CAFs contribute to ECM remodeling and chemotherapy resistance through different matrix metalloproteinases or matrixins secretion [14].
In order to elucidate this goal, CAFs secret several cytokines and growth factors. Production and release of hepatocyte growth factor, interlukin-6, and many other active molecules by CAFs facilitates the migration and invasion of cancer cells through altering matrix features. Exosomes are tiny equipment that mediate bidirectional connection between tumor cells and TME components through carrying these molecules around tumor cells environment to support tumor progression [7].
Exosome-mediated cell-cell communication is an important mechanism used by TME components to convey information participating in CAF activation, chemotherapy response alteration, and organ specific distant metastasis [4, 15].
Comprising diverse cell types in a range of functional niches, the TME modulates a vast array of cell interactions which organize TME remodeling to promote tumor initiation, progression, and metastasis [16].
2.2The characteristics of the pre-metastatic niche
Prior to cancer cells arrival to secondary distant sites, formation of an active environment is a fundamental mechanism to ensure their successful arrival and implementation. Primary tumor interacts with the metastatic organ environment and secrets numerous important factors that are implicated in creating the enriched microenvironment called pre-metastatic niche. The seeding of metastatic cancer cells requires substantial molecular and cellular alterations in the tissue microenvironment to produce fertile soil for secondary tumor formation in distant organs [17]. The primary tumor secretes some essential soluble molecules such as TDSFs including tumor necrosis factor alpha (TNF-α), transforming growth factor-β (TGF-β), and vascular endothelial growth factor (VEGF-A) along with extracellular vesicles (EVs) that are required for the preparation of distant receptive sites for de novo pre-metastatic niche formation and organ-specific metastasis or organotropism [18]. In fact, formation of a permissive microenvironment containing nutrients, ECM proteins, tumor-derived secreted factors (TDSFs), tumor-derived exosomes, suppressive immune cells, host stromal cells, and homing factors supports tumor cells survival and seeding in a specific distant organ. These components guarantee tumor growth by controlling attaching, self-renewal capacity, and the de-differentiation of CSCs [17, 19].
CAFs and tumor exosomes play important roles in regulating ECM remodeling for the preparation of the pre-metastatic niche [20, 21]. CAFs which are induced by hypoxic condition produce TGFβ2 that can preserve the stemness of the niche. Furthermore, they may activate pathways which reduce chemotherapy response and facilitate tumor expansion.
In addition, hypoxia and inflammation promote the production of TDSFs from tumor cells which alter the functions and the migratory activity of myeloid cells facilitating pre-metastatic niche formation. For example, increased signal transducer and activator of transcription 3 (STAT3) activity in CD11b+myeloid cells, motivates the local invasion of primary tumor cells into the blood or lymphatic vessels to move towards the metastatic sites and create pre-metastatic niche [17].
Metastatic cells are able to provide the “soil” required to home cancer cells in the secondary sites. By secreting IL11, CAFs support distant metastasis through activating Glycoprotein 130 (GP130)/STAT3 signaling in CRC cells [22].
Since tumor microenvironment create a great and accommodating circumstance for the growth of tumor cells, it would be a helpful therapeutic target to limit colonization of circulatory tumor cells in distant organs.
2.3The diversity of CAFs and their functions
CAFs, the most abundant component of TME, are activated fibroblasts with pro-tumorigenic functions. However, a study by Ozdemir and colleagues showed that CAFs may have anti-tumoric functions, because the expression of CAFs in genetically engineered mouse models of pancreatic cancer limited tumor growth [23]. Basically CAFs are originated from enormous different precursors including normal fibroblasts, epithelial cells, endothelial cells, pericytes, and bone marrow-derived cells [24]. The differentiation and trans-differentiation of CAFs from their progenitors are managed by disparate secreted factors from cancer cells and processes of epithelial-mesenchymal and endothelial-mesenchymal transitions [25]. The diversity of CAFs origins contributes to the formation of heterogeneous CAFs that express different markers and play various and controversial functions [26]. There are diverse subtypes of CAFs including matrix secreting CAFs such as myofibroblasts, iCAFs (inflammatory CAFs), antigen presenting CAFs (apCAF), NF-κB-driven CAFs, and cancer-restraining CAFs which are differently expressed in various types of cancers and different stages [27]. NF-κB-driven CAFs increase cancer cell stemness and facilitate chemotherapy resistance. Cancer-restraining CAFs restrict the progression of the disease and cancer invasion, so that targeting these type of CAFs promotes tumor growth [26]. For instance, CAFs with high meflin expression and low α-SMA in pancreatic cancer are cancer-restraining and loss of these CAFs facilitates cancer progression. The therapeutic strategies and outcomes of targeting CAFs therefore depend on the CAFs subtypes, cancer types, and even cancer stage [27].
Many transcription factors with either pro-tumor or anti-tumor functions contribute to the mechanisms engaged in the reprogramming and changing the profile of CAFs. For instance, based on cancer type, CAFs play paradoxical anti-tumor or pro-tumor functions in an NF-κB dependent manner [27]. In fact, NF-κB signaling is implicated in carcinogenesis through induction of inflammatory processes and it also may restrict the tumor growth through arresting cell cycle and facilitating apoptosis [28]. Pallangyo and colleagues demonstrated an unexpected opposite process in a model of colitis-associated cancer in which IKKβ/NF-κB in CAFs represents tumor inhibitory functions through reduction of TGF-β and HGF expressions [29]. According to their experiments, IKKβ-deficient fibroblasts promote tumor angiogenesis and tumor progression and also by accumulating regulatory T cells support tumor cells scape from anti-tumor immunity. Since IKKβ is a negative regulator of TGF-β, it reduces the expression of TGF-β gene and HGF secretion contributing to tumor suppression [29]. However, activation of NF-κB pathway supports the proliferation of cancer cells and it also promotes tumor metastasis by providing an appropriate pre-metastatic niche [30].
The paradoxical dual functions of CAFs to promote or suppress tumor growth, emphasize understanding and clear clarification of the functions of CAFs in the TME [31].
CAFs play a critical role in constructing a supporting microenvironment to facilitate tumor growth, invasion, metastasis, and chemo-resistance [32]. CAFs secrete enormous factors including VEGF, HGF, stromal cell-derived factor 1 (SDF1), insulin-like growth factor 2 (IGF2), and TGF-β1 to promote tumor angiogenesis, neoplastic epithelial cell transformation, cancer cell proliferation, and invasion of tumors [33]. TGF-β participates in CAFs differentiation and metabolic reprogramming. CAFs express various fibroblastic and mesenchymal markers distinct from normal fibroblasts, and their most prominent marker is alpha-smooth muscle actin (α-SMA) [34].
As a result of inappropriate vascularization and decreased blood supply, cancer cells and stromal cells may have limited access to nutrients and oxygen during tumor growth and progression [35]. Metabolic reprogramming is an evolving mechanism induced by CAFs to improve bioenergetics and biosynthesis to adjust to the hypoxic conditions and hypo-nutrients [36]. CAFs provide energetic nutrients such as lactate, pyruvate, and ketone bodies to support tumor against deprivation and facilitate proliferation of tumor cells. CAFs can change CSCs profile toward an increased migratory behavior and can enhance drug resistance through modulation of ECM molecules and reservioring oncogenic signals [18, 37].
However, it should be point out that besides exciting and critical aspects of studying CAFs, it may face many challenges due to short culture times and manipulation difficulties. In addition, due to various origins and different protumoric and anti-tumoric functions, CAFs modulations for therapeutic interventions may encounter important challenges [38].
2.4Function of exosomes in cancer progression
Both normal cells and cancer cells release small membrane-bound vesicles called exosomes which are found in different body fluids like blood, urine, and saliva. These nano-vesicles contribute to enormous physiological processes. For example, they promote stem cell proliferation and differentiation, help tissue regeneration, improve neuronal function, and regulate cell signaling [39].
As exosomes play an essential role in intercellular communication which is substantial for many physiological processes, they also mediate communication of tumor cells with their surrounding stroma for the exchange of genetic information which results in tumor growth and invasion [39].
The function of the exosomes varies with their origins. Tumor derived exosomes are involved in the creation of premetastatic niches and the promotion of vasculugenesis, ensuring growth and metastasis of tumors [19]. Furthermore, the release of exosomes coated with hyaluronic acid (HA) by normal fibroblasts after radiation therapy can promote premetastatic niche and tumor growth [40].
Exosomes released from primary tumor may behave like endocrine system and enter into the lymphatic system and bloodstream to achieve distant organs to activate recipient stromal cells for creation of metastatic niche before the metastatic cells reach the secondary tumor sites. Circulating tumor-derived exosomes contain different miRNAs, non-coding RNAs, proteins, tumor related mutant substrates transporting information to the cells in metastatic sites [41]. Exosomes may contribute to tumor progression through inhibiting immune response against the tumor. Upregulation of exosomes and proinflammatory factors induces recruitment of tumor -associated macrophages, tumor-associated neutrophils, regulatory T cells, and MDSCs into distant metastatic organs to suppress anti-tumor immune response [42].
2.5Techniques of exosomes isolation
Carrying different biomarkers and their therapeutic potential make exosomes as critical targets for diagnostic applications and exosome-based therapy attracts many researchers all around the world [43].
Numerous insulation methods are available, each with unique properties and different isolation efficacies. Exosome separation approaches are mainly based on their size, density, surface characteristics, and receptors. For instanse, ultracentrifugation (UC) technique separates exosomes according to their size and density variations [44]. Exosomes can be captured by immunomagnetic beads that are coated with specific monoclonal antibodies and bind to their surface receptors [45]. Size-based methods such as UC and size-exclusion chromatography lack enough specificity in comparison to affinity-based isolation techniques. Different commercial kits for extracellular vesicle isolation use diverse methods including precipitation (miRCURY Exosome Isolation Kit), membrane affinity (Qiagen), size-exclusion chromatography such as Exo-spin Midi Columns (Cell Guidance Systems) and qEV Columns (Izon Science), and Sedimentation (Beckman Coulter Optima LE-80K) [46].
One of the high-performance approaches for exosome isolation is microfluidic-based technique which uses immunoaffinity, size, and mass principles. ExoChip is a common microfluidic device for exosome isolation which utilizes antibodies specified for exosomes markers [47]. The optimal purity, yield quantity, and quality of exosome isolation must be evaluated. The yield’s purity, lack of proteins co-isolation with exosomes, is evaluated by nanoparticle tracing analysis (NTA) and bicinchoninic acid assay [48]. Furthermore, western blot analysis is an effective test for accessing the exosomes markers intensity and then the purity of the yield [48]. Combination of multiple isolation methods improves the exosomes purification [49].
3The role of CAF-derived exosomes in different gastrointestinal cancer types
Globally, based on the latest news from the Global Cancer Statistics 2018, intestinal cancer types are among the most frequent causes of cancer mortality worldwide [50]. Gastrointestinal cancers (GI) cancers are classified as aggressive malignancies with a high possibility of resistance to therapy [51]. Different GI cancers include cancers of the colorectal, stomach, esophagus, pancreatic, liver and bile ducts among which colorectal cancer, stomach cancer, liver cancer and esophageal cancer are most common. The prevalence and mortality of GI cancers varies by country. High-income countries, for instance, have the highest prevalence of colorectal cancer, while liver, gastric, and esophageal cancers are more common in low-income countries. CAFs and their exosomes play critical roles in different GI cancers progression [Fig. 1]. A summary of the studies evaluating these entities is illustrated in Table 1.
Fig. 1
The role of CAF-derived exosomes contents in GI cancers progression. The tumor microenvironment contains many different cellular and non-cellular components. CAFs, the most abundant component of the tumor microenvironment, produce and release exosomes which are differently featured in different cancers. For instance, enhanced expression of miR-92a-3p by CAF-derived exosomes in CRC is associated to increased CSCs production and drug resistance, while decreased exosomal expression of miR-214 in GC is correlated to tumorigenesis and metastasis. In addition, miR320a and miR-195 down-regulations promote liver cancer development and up-regulations of snail and miR-106b are responsible for chemoresistance and progression of pancreatic cancer. CRC: colorectal cancer, GC: gastric cancer, CAFs: cancer associated fibroblasts, CSCs: cancer stem cells.
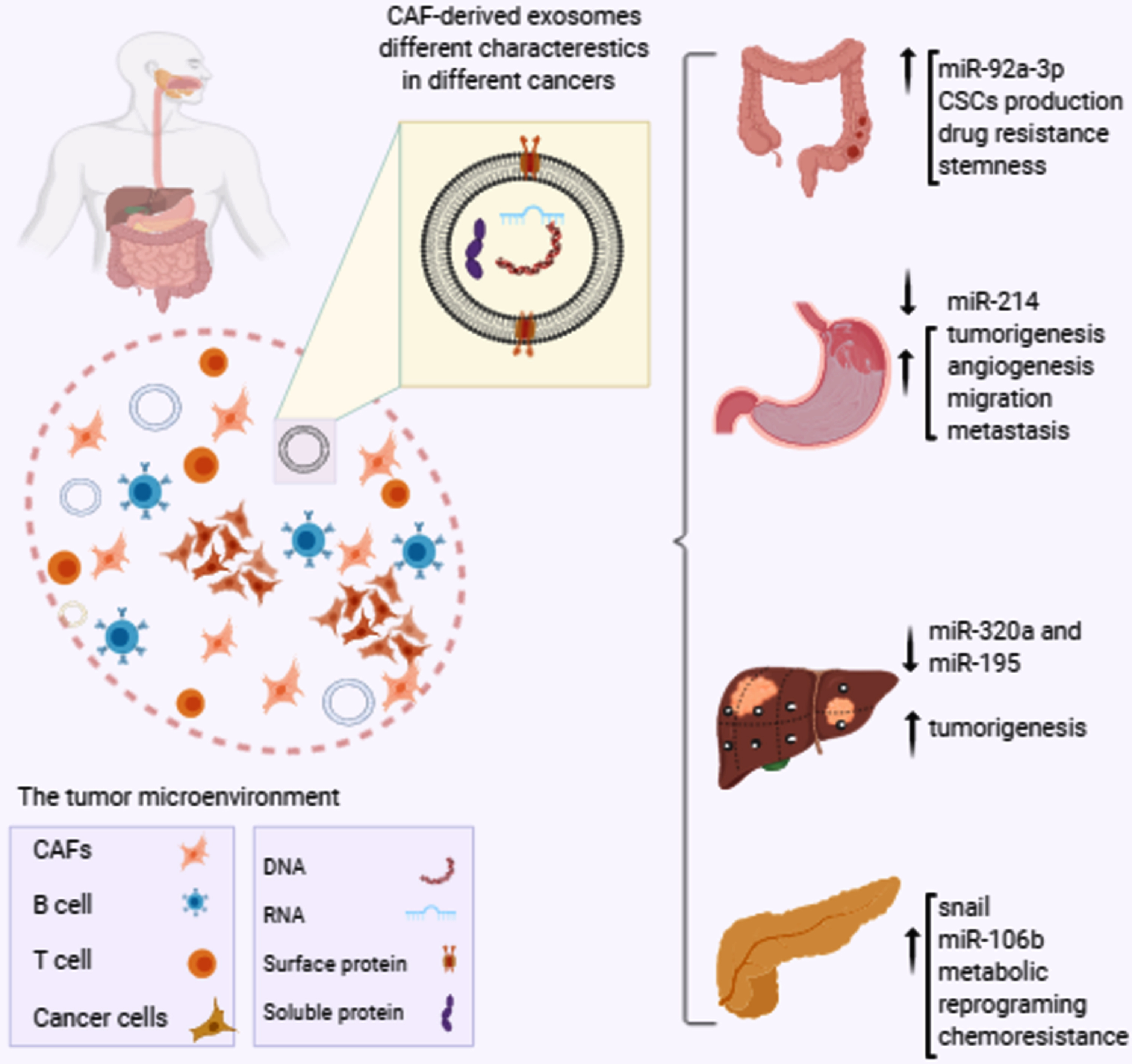
Table 1
Summary of CAF-exosomes functions in various GI cancers progression
Cancer Type | Samples | Alterations | Outcome | Ref. |
ESCCa | Stromal fibroblasts from primary cancer and normal tissues | Activation of sonic Hedgehog signaling pathway | Promotion of proliferation and migration of ESCC | G. Zhao 2020 |
Fresh-frozen tissues and serum samples | miR-451 provides a suitable niche for cancer cells | Aggressive behavior. Increases tumor progression and metastasis | S. Khazaei 2016 | |
The ESCC cell line (KYSE-30), human normal fibroblasts cell line (HFSF-PI3) | Secretion of miR-33a and miR-326 | Providing therapeutic targets for esophageal cancer | N. Nouraee 2016 | |
CRCb | Colorectal adenocarcinoma tissue samples | Increased CSCs production | Chemoresistance and tumor progression | Y. Hu 2015 |
CAFsf and NFsg from CRC and adjacent normal tissues | Elevated miR-92a-3p: enhance EMT-B catenin pathway | Resistance to chemotherapy, increased metastasis, decreased apoptosis | J. L. Hu 2019 | |
Tumor tissues of CRC patients | LncRNA H19 overexpression, Enhanced stemness | Chemoresistance and CRC progression | J. Ren 2018 | |
Samples from patients with biopsy proven CRC | Deregulation of microRNAs 329, 181a, 199b, 382, 215 and 21 Overexpression of miR-21 | Invasiveness, proliferation, chemotherapy resistance in surrounding tumor cells, Exosomes rely stromal gene expression to cancer cells | R. Bhome 2017 | |
Primary human colorectal tumor specimens | Wnt ligands secretion | Increase chemoresistance and self-renewal ability of CRC cells tumor growth | Y.-B. Hu 2018 | |
GCc | The primary CAFs and corresponding normal fibroblasts were isolated | Down regulation of miRNA-214 and overexpression of FGF9 in CAFs, Increased MMP2 activity | Increase malignancy, motility, and node invasion and metastasis poor prognosis | Y. Miki 2018 |
Human plasma and tumor samples of gastric cancer patients | Enhanced exosomal MMP11 | Increased growth and migratory behavior of cancer cells and metastasis | G. Xu 2019 | |
Plasma and tumor samples of gastric cancer patients | Enhanced exosomal miR-522 | Ferroptosis inhibition in cancer cells by targeting ALOX15 and blocking lipid-ROS accumulation: increase chemoresistance in GC | H. Zhang 2020 | |
PCd | CAF cell line created from patient-derived tumor samples | Increased exosome release containing Snail | Promotion of proliferation, drug resistance Exosome inhibitors: treatment options | K E Richards 2016 |
CAFs were isolated from primary fibroblasts of pancreatic cancer patients | Increase exosome release, transferring miRNAs, transferring miR-106b | Increased drug resistance | Yuan Fang 2019 | |
Patient derived fibroblast cells | Reprogram cancer cells Disabling mitochondrial oxidative and increase glycolysis | Increase cancer cells proliferation | H. Zhao 2016 | |
HCCe | CAFs and para-cancer fibroblasts (PAFs) of HCC patients. | miR-320a down-regulation in CAF-derived exosomes | Decrease anti-tumor functions of miR-320a Cancer progression and metastasis | Zhuochao Zhang 2017 |
aesophageal squamous cell carcinoma, bcolorectal cancer, cgastric cancer, dpancreatic cancer, ehepatocellular carcinoma, fcancer associated fibroblasts, gnormal fibroblasts.
3.1Colorectal cancer
Colorectal cancer (CRC) is the world’s third most lethal and fourth most frequently diagnosed cancer. The prevalence of CRCs worldwide is increasing, especially among populations with western lifestyles [52].
Yibing Hu et al in 2015 have shown that exosome secretion by CAFs promotes chemo-resistance and tumor progression via tremendous production of CSCs. They found that CAF-derived exosomes increased the proliferation and growth of CSCs that are the target for 5-fluorouracil (5-Fu) or oxaliplatin (OXA). Therefore, inhibition of exosome production prohibits CSC production and improves drug sensitivity [53].
In 2019 L.J Hu et al, designed in vitro experiments that showed human CRC cells treated with CAFs conditioned medium (CM) gained a higher migration capacity and chemotherapy resistance compared to normal fibroblasts. They assumed that these tumor-promoting effects were the result of the release of exosomes to CM and MicroRNA microarray analysis showed altered miRNA expression in CAF-derived exosomes. They concluded that patients may have poor prognosis due to increased drug resistance through mechanisms mediated by CAF-derived exosomes. In colon cancer, CAFs produce exosomes with elevated miR-92a-3p which enhances epithelial-mesenchymal transition (EMT) and resistance to chemotherapy. In fact, miR-92a-3p overexpression is associated with increased metastasis and enhanced EMT through activation of Wnt/β-catenin pathway and decreased mitochondrial apoptosis by inhibiting apoptosis modulators such as MOAP1 and FBXW7 [54]. CAFs and cancer cells communication is vital for the progression of the disease. Long non-coding RNA (lncRNA) H19 overexpression is responsible for increased stemness profile, chemo-resistance, and disease progression in colorectal cancer patients. A study by Jing Ren and colleagues showed that H19 was overexpressed in tumor tissues of colitis-associated cancer mice and CRC patient samples. In order to discriminate the effect of normal fibroblasts exosome and CAF-exosomes, they separately isolated these exosomes and found that CAF-secreted exosomes transfer H19 to CRC cell lines SW480 or HCT116 cells [55]. CAFs enhance stemness and chemo-resistance by transferring exosomal H19 to the CRC cells which competes with miR-141. Since miR-141 restricts the stemness of CRC cells, exosomal uptake of H19, an endogenous competitive RNA for miR-141, stimulates β-catenin pathway and increased stemness and chemo-resistance.
LncRNA CCAL (colorectal cancer-associated lncRNA) is responsible for drug resistance in oxaliplatin-treated CRC cells. In situ hybridization (ISH) assay detected higher TME expression of CCAL than cancer nests of CRC tissues. In fact, CAFs express and transfer CCAL via exosomal interactions to CRC cells. Transferred CCAL affords chemo-resistance, inhibits apoptosis, and stimulates β-catenin pathway in vitro and in vivo. Hence, CCAL targeting can re-sensitize cancer cells for oxaliplatin treatment program.
In 2013, a valuable study by Bullock and colleagues demonstrated that mir-21 deregulation in TME plays a crucial role in metastatic progression. They reported overexpression of miR-21 in CRC stromal fibroblasts. Ectopic miR-21 expression in fibroblasts co-cultured with CRC cells protected cancer cells against oxaliplatin apoptotic effects and increased their proliferation. Fibroblastic mir-21 overexpression promotes myofibroblasts trans-differentiation from fibroblasts and cancer invasion, progression, and chemo-resistance through a TGF-β-dependent pathway. Matrix metalloproteinase 2 (MMP2) over-activation by miR-21 as a result of RECK downregulation which is an important regulator of MMP2, supports tumor invasion [57].
CAFs produce exosomes with various miRNAs cargo which phenotypically alter CRC recipient cells leading to enhanced proliferation and chemo-resistance. Rahul Bhome’s study represented a different hallmark of miRNA expression by CAF-derived exosomes that are rich in 329, 181a, 199b, 382, 215 miRNAs with a greater amount of miR-21. This study revealed that miR-21 overexpression by CAFs is correlated with proliferative and invasive capacities and increased chemo-resistance of surrounding tumor cells [58]. Exosomes therefore are important communication tools between stromal fibroblasts and CRC cells conveying numerous stromal genes and onocomirs to the recipient cells. Higher expression of miR-21 in CAFs than in normal fibroblasts confirmed its stromal origin transferring oncogenic signals to CRC cells through exosomal communication.
In addition, there was an increased rate of liver metastasis of CRC cells with over-expressed miR-21 in orthotopic xenografts models. In vivo evaluation of the functions of miRNAs in CRC metastasis was first investigated in this study [58].
Another suggested method through which CAF exosomes mediate alteration of CRC cells toward an invasive profile and induce chemotherapy resistance is Wnt ligands production.
Exosomes containing Wnt ligands increase CRC cells self-renewal. In vivo experiments confirmed the importance of Wnts function in chemotherapy resistance [59].
3.2Esophageal squamous cell carcinoma (ESCC)
The prevalence of esophageal cancer varies worldwide. Esophageal cancer is the sixth leading cause of cancer-related deaths worldwide. The incidence esophageal cancer, a life-threatening malignant tumor, is rising worldwide especially in western countries [60, 61].
One of the important molecular events that underlie the tumorigenesis of esophageal cancer is SHH signaling activation contributing in multiple critical aspects of the disease progression. Zhao et al’s study showed that exosomes derived from CAF-CM and CAFs significantly enhance the in vitro and in vivo proliferation and migration capabilities of ESCC cell lines. Exosomal SHH produced by CAFs is one of the reasons for tumor promoting functions of exosomes. Transfer of SHH by CAF-exosomes into the ESCC cells and activation of Hedgehog signaling facilitates cancer progression and metastasis through direct impacts on proliferative and migratory capacities. According to the transwell assay results exosomes from CAFs induce a dose-dependent increase in migration of ESCC cells. Restricting exosomes functions and inhibition of SHH signaling by cyclopamine may prevent progression of the disease offering novel options to cure ESCC patients [62].
CAF-exosomes contain various oncomirs influencing cancer cell metastatic features. Downregulation of anti-tumor miRNAs and upregulation of the pro-tumor ones stimulate the tumor growth, aggressiveness, and metastasis. In 2017, a study emphasized on the function of miR-451 in TME that is a diagnostic molecule in esophageal cancer and revealed that CAFs produce exosomes filled by miR-451 to transmit signals for suitable metastatic niche preparation. As a result of exosomal packaging the amount of mir-451 differs in serum sample and tumor tissue of the same patient. Unlike the other studies that introduced miR-451 as a tumor suppressor factor, they explained a different function of miR-451 in the TME. Calcium-binding protein 39 (CAB39) and Ras-related protein 14 (RAB14) are important factors that are engaged by miR-451 in favor of promoting proliferation and migration of cancer cells. Then they suggested new molecular targets for restricting cancer progression and improving survival of patients with this aggressive type of cancer [63].
A co-culture system consisting of fibroblasts and esophageal carcinoma cell line (KYSE-30 cells) was prepared to mimic TME. The two the co-cultured cell lines were physically separated sharing the same CM. CAFs contribute to tumor growth and metastasis through mediating the production of growth factors and remodeling of tumor stroma. CAFs produce miRNAs to transfer metastatic information to cancer cells and exosomes are responsible for the packaging and carrying miRNAs within the tumor stroma. The aim of this study was to assess the functions of CAFs miRNA secretion in TME suggesting new therapeutic targets. By mediating fibroblast-CAF transition, elevated exosomal miR-33a and miR-326 in CM may alter surrounding tumor cells towards metastatic profile and enhance angiogenesis [64].
3.3Gastric cancer
Globally gastric cancer (GC) appears to be the fifth most prevalent cancer and the third main cause of cancer-related mortality [65]. Local recurrence, peritoneal spread, and distant organ metastases are the most important reasons of deaths from gastric cancer [66].
By secreting numerous promoting factors in the TME CAFs facilitate gastric cancer progression. Gastric cancer exosomes support tumor progression and metastasis by facilitating angiogenesis, tumorigenesis, and immune escape of tumor cells [67].
Exosomes of gastric cancer facilitate adhesion of cancer cells to the mesothelium supporting metastasis. Exosomes from gastric cancer cells guarantee the disease progression by stimulating production of pro-tumor immune cells and silencing anti-tumor response through differentiation of T cells to regulatory T cells. Gastric cancer exosomes induce mesenchymal stem cell (MSCs) transition to CAFs. GC cells exosomes are able to promote angiogenesis and metastasis via myofibroblasts generation from different origins and endothelial cells activation.
MSCs produce exosomes which increase tumorigenicity of gastric cancer cells and their metastatic potential. Exosomes from CAFs promote metastasis in various cancers.
As miR-214 directly targets FGF-9, the downregulation of miR-214 occurring in GC CAFs increases the expression of FGF-9 and, subsequently, the migration of GC cells by inducing EMT. MiR-214 transfection of GC CAFs suppresses EMT and invasion of GC cells with no effect on proliferation. Therefore, miR-214 prohibits the role of CAFs in supporting cancer progression [68].
CAFs support the proliferation, growth and progression of surrounding scirrhous gastric cancer cells. Scirrhous gastric cancer cells (OCUM-12 and NUGC-3 cells) are unique in taking up the exosomes released from CAFs compared to other types of gastric cancer cells. The CAF-derived exosomes specifically express CD9 on their surfaces, which is significantly associated with cancer cell invasion and motility behavior. A positive correlation exists between CD9 positive exosomes release and malignancy of Scirrhous gastric cancer or Borrmann type IV gastric carcinoma which is a diffused type of gastric cancer. Motility-promoting activity of CAF-derived exosomes diminished with the use of CD9 inhibitors. In fact, CD9-positive exosomes increase MMP2 expression which is correlated with lymph node metastasis, increased migratory behavior, and venous invasion of scirrhous-type gastric cancer cells [69]. According to their results, CD9-positive patients showed a lower 5-year survival rate compared to CD9-negative cases.
When the cancer is at an advanced stage, probably can be cured by chemotherapy. While cisplatin and paclitaxel are the first set of drugs for the treatment of gastric cancer, these anticancer drugs may activate chemoresistance-involved pathways. Production of exosomal miR-522 by CAFs may inhibit ALOX15 which is a potent producer of lipid-ROS [70]. Lipid-ROS stimulates ferroptosis which is an iron dependent non-apoptotic cell death. In fact, cisplatin and paclitaxel increase miR-522 production of CAFs and increase chemo-resistance by ferroptosis inhibition [70]. By de-ubiquitination USP7 performs its role in hnRNPA1 stabilization that is a master mediator of miR-522 packaging to exosomes. Thus, inactivation of USP7/hnRNPA1 axis inhibits miR-522-mediated suppression of ALOX15 leading to increased cell death and chemo-sensitivity [70].
MMPs are the main ECM enzymes that participate in matrix degradation [71]. Guifang Xu’ study revealed that exosomal matrix metalloproteinase 11 (MMP11) from CAFs is associated with the growth and migratory behavior of GC cells and metastasis. Overexpression of exosomal MMP11 in serum and tumor tissue of patients is clinically important and affects patients’ overall survival. MiR-139 derived from CAF-secreted exosomes of gastric cancer prevents growth of the tumor and metastasis. MiR-139 in exosomes decreases MMP11 so that it limits tumor growth and improves the overall survival of patients with gastric cancer [72]. They showed that there is down-regulated expression of miR-139 in gastric cancer CAFs leading to enhanced pro-tumor activity of CAF-MMP11 expression and metastasis.
Circular RNAs (circRNAs), a novel family of endogenous noncoding RNAs, play crucial roles in gene expression regulation [73]. circRNAs contribute in cancer progression and metastasis. In gastric cancer patients, ciRS-133 (an exosomal circRNA) is involved in cancer-related cachexia, a metabolic syndrome which is associated with body weight loss and systemic inflammation [74].
3.4Pancreatic cancer
Pancreatic cancer is the seventh most common cause of cancer deaths in both sexes worldwide with higher prevalence in advanced nations. Because of Poor diagnosis and treatment pancreatic cancer mortality rate is extremely high with a 5-year overall survival rate of about 5 percent [75, 76].
Gemcitabine (GEM) is the most frequently used chemotherapeutic agent for pancreatic cancer, but recurrence of cancer is the major challenge patients face after treatment. Gemcitabine-treated patients with pancreatic cancer can have good chances of disease-free survival if chemo-resistance and reappearance of the disease are inhibited.
Chemo-resistance of CAFs is one their natural ability to support cancer progression relapse. Richards et al’s study showed that snail and is up-regulated in chemo-resistant cancer cells. Snail is a chemo-resistance promoting factor that is transferred by exosomes to the recipient cancer cells which increase tumor growth and cancer cells survival. In fact, the increase of exosome release by CAFs after receiving GEM is responsible for drug resistance [77]. They observed increased snail expression in CAF-released exosomes which causes cells to proliferate and act against anti-cancer GEM effects. Suppression of exosome release prevented tumor cells survival and chemo-resistance suggesting a new avenue for drug response enhancement [77].
Resistance to GEM is accomplished by activation of the cancer pathway Akt-GSK3β-Snail. Consequently, agents suppressing this pathway’s activation improve response to GEM therapy.
In 2019, Yuan Fang and colleagues described another mechanism of CAFs functioning in promoting drug resistant after GEM therapy [78]. They found that conditioned medium and CAF-exosomes play a critical role in drug resistance induction and GEM treatment increased the ability of CAFs in triggering cancer cells proliferation. GEM exposure elevates miR-106b expression in CAFs and CAF-exosomes [78].
According to their observations miR-106b transmission by CAF-exosomes to the pancreatic cancer cells induce drug resistant through targeting TP53INP1. Hence, either suppressing exosome secretion or inhibiting miR-106b expression may be beneficial in the avoidance of chemo-resistance, suggesting therapeutic targets [78].
The presence of annexin A6/LDL receptor-related protein 1/thrombospondin 1 (ANXA6/LRP1/TSP1) complex in the TME provide optimal conditions to support tumor cell survival. Due to their restrictive presence in the serum samples of PDA patients, ANXA6 + EVs can be considered as a potential biomarker for defining PDA grade. It seems CAFs are the only producers of ANXA6/LRP1/TSP1 and, in order to enhance PDA invasiveness, they transfer this complex to the neighboring cancer cells. Unlike ANXA6 injection which promotes invasiveness of PDA and distant metastasis, loss of ANXA6 in CAFs resulted in reduced tumorigenecis and prevention of metastasis [79].
Metabolic reprogramming by CAFs is a vital phenomenon to produce energetic metabolites to support cancer cells survival and proliferation. Transmission of miR-22 and miR-125b by pancreatic CAF-derived exosomes prevents regular mitochondrial oxidative phosphorylation and stimulate glycolysis and glutamine’s reductive carboxylation [80]. CAF-derived exosomes are involved in activating the Warburg effect in cancer cells, which is an altered metabolism based on aerobic fermentation in which the rate of glucose uptake and fermentation increases tremendously, producing lactate [80].
By transporting lactate, acetate, amino acids, TCA cycle intermediates, and lipids to the cancer cells, Exosomes ensure the survival of cancer cells [80]. Targeting exosomal communication to prevent metabolite cargo delivery can be a beneficial therapeutic option. Consequently, a beneficial therapeutic option would be to target exosomal communication to suppress metabolite cargo delivery.
3.5Liver cancer
Hepatocellular carcinoma (HCC) is the most common type of primary liver cancer and is really probable to be developed in people with liver diseases. Intrahepatic cholangiocarcinoma is the second most common type of liver cancer developing in intrahepatic bile ducts [81].
Both miR-320a and miR-195 are anti-tumor factors for which growth of liver associated cancers is restricted by their overexpression. In 2017 a study by Zhuochao Zhang and colleagues using Xenograft models demonstrated that miR-320a up-regulation is correlated with less cancer colonization. MiRNA sequencing elucidated that miR-320a is down-regulated in CAF-derived exosomes. Based on the results of in vitro and in vivo studies, by targeting PBX3, miR-320a act as anti-tumor factor suppressing cancer progression and metastasis. MAPK signaling activity is associated with metastasis through upregulation of MMP2 and EMT induction. In fact, miR-320a performs its anti-tumor function by inhibiting MAPK pathway [82].
Furthermore, MiR-195 is an anti-tumor factor as many studies show and its reduction in TME and cholangiocarcinoma (CCA) cells increases their proliferative and aggressive capacity. In 2017 Ling Li and colleagues first reported downregulation of miR-195 in CCA [83]. They indicated that administration of extracellular vesicles containing miR-195 leads to reduced tumor growth and enhanced overall survival of rats with CCA. MiR-195 suppress multiple downstream pro-tumor genes to restrict tumor progression. Either targeting extracellular vesicles or utilizing as anti-cancer drugs delivery are valuable therapeutic options.
4Conclusions
Recently scientists have come to believe that targeting the tumor stroma components can be as important as tumor cells for cancer treatment. CAFs are critical components participating in cancer progression through enhancing the survival of cancer cells, drug resistance, and distant metastasis. By restricting metastatic niche formation and reducing drug resistance, CAF-directed anti-cancer strategies may be novel therapeutic treatments to reduce the risk of cancer progression. In different types of GI cancers, CAFs and their secreted exosomes with various contents mediate numerous signaling pathways to facilitate metastases to distant organs. Inhibition of either the functions of CAFs or exosome release can be a promising direction for the treatment of cancers of the digestive system.
Acknowledgment
The authors have no acknowledgments. This research received no specific grant from any funding agency in the public, commercial, or not-for-profit sectors.
Author contributions
CONCEPTION: SHJ
DATA CURATION AND ANALYSIS: BZ
PREPARATION OF THE MANUSCRIPT: BZ
REVISION FOR IMPORTANT INTELLECTUAL CONTENT: LR and PD
SUPERVISION: All authors
Conflict of interest
The authors declare that there is no conflict of interests.
References
[1] | Quante M , Varga J , Wang TC , Greten FR . The gastrointestinal tumor microenvironment. Gastroenterology. W.B. Saunders; (2013) ;145: (1):63–78. doi: 10.1053/j.gastro.2013.03.052 |
[2] | Hassanzade J , Molavi e vardanjani H , Farahmand M , Rajaiifard AR . Incidence and mortality rate of common gastrointestinal cancers in South of Iran, a population based study. Iran. J. Cancer Prev. (2011) ;4: (4):163–9. |
[3] | Zhao Y , Li J , Li D , Wang Z , Zhao J , Wu X , et al. Tumor biology and multidisciplinary strategies of oligometastasis in gastrointestinal cancers. Semin. Cancer Biol. (2020) ;60: :334–43. doi: 10.1016/j.semcancer.2019.08.026 |
[4] | Bae S , Brumbaugh J , Bonavida B . Exosomes derived from cancerous and non-cancerous cells regulate the anti-tumor response in the tumor microenvironment. Genes and Cancer. (2018) ;9: (3-4):87–100. doi: 10.18632/genesandcancer.172 |
[5] | Östman A , Augsten M . Cancer-associated fibroblasts and tumor growth - bystanders turning into key players. Curr. Opin. Genet. Dev. (2009) ;19: (1):67–73. doi: 10.1016/j.gde.2009.01.003 |
[6] | Kobayashi H , Enomoto A , Woods SL , Burt AD , Takahashi M , Worthley DL . Cancer-associated fibroblasts in gastrointestinal cancer. Nat. Rev. Gastroenterol. Hepatol. (2019) ;16: :282–95. doi: 10.1038/s41575-019-0115-0 |
[7] | Wan Z , Gao X , Dong Y , Zhao Y , Chen X , Yang G , et al. Exosome-mediated cell-cell communication in tumor progression. Am J Cancer Res. (2018) ;8: :1661–73. |
[8] | Scavo MP , Depalo N , Tutino V , De Nunzio V , Ingrosso C , Rizzi F , et al. Exosomes for diagnosis and therapy in gastrointestinal cancers. Int. J. Mol. Sci. (2020) ;21: (1):367. doi: 10.3390/ijms21010367 |
[9] | Herrera M , Llorens C , Rodríguez M , Herrera A , Ramos R , Gil B , et al. Differential distribution and enrichment of non-coding RNAs in exosomes from normal and Cancer-associated fibroblasts in colorectal cancer. Mol. Cancer. (2018) ;17: :114. doi: 10.1186/s12943-018-0863-4 |
[10] | Chen F , Zhuang X , Lin L , Yu P , Wang Y , Shi Y , et al. New horizons in tumor microenvironment biology: Challenges and opportunities. BMC Med. (2015) ;13. doi: 10.1186/s12916-015-0278-7 |
[11] | Heidary Z , Ghaisari J , Moein S , Haghjooy Javanmard S . The double-edged sword role of fibroblasts in the interaction with cancer cells; an agent-based modeling approach. ChammasR, editor. PLoS One. (2020) ;15: :e0232965. doi: 10.1371/journal.pone.0232965 |
[12] | Liu T , Han C , Wang S , Fang P , Ma Z , Xu L , et al. Cancer-associated fibroblasts: an emerging target of anti-cancer immunotherapy. J Hematol Oncol. (2019) ;12: :86. doi: 10.1186/s13045-019-0770-1 |
[13] | Zahavi DJ , Weiner LM . Tumor mechanisms of resistance to immune attack. Prog Mol Biol Transl Sci. (2019) ;164: :61–100. doi: 10.1016/bs.pmbts.2019.03.009 |
[14] | Burgos-Panadero R , Lucantoni F , Gamero-Sandemetrio E , Cruz-Merino L de la , Álvaro T , Noguera R . The tumour microenvironment as an integrated framework to understand cancer biology. Cancer Lett. (2019) ;146: :112–22. doi: 10.1016/j.canlet.2019.07.010 |
[15] | Tian W , Liu S , Li B . Potential Role of Exosomes in Cancer Metastasis. Biomed Res. Int. (2019) . doi: 10.1155/2019/4649705 |
[16] | Li I , Nabet BY . Exosomes in the tumor microenvironment as mediators of cancer therapy resistance. Mol. Cancer. (2019) ;18: :32. doi: 10.1186/s12943-019-0975-5 |
[17] | Liu Y , Cao X . Characteristics and Significance of the Pre-metastatic Niche. Cancer Cell. (2016) ;30: (5):668–81. doi: 10.1016/j.ccell.2016.09.011 |
[18] | Valcz G , Buzás EI , Szállási Z , Kalmár A , Krenács T , Tulassay Z , et al. Perspective: bidirectional exosomal transport between cancer stem cells and their fibroblast-rich microenvironment during metastasis formation. npj Breast Cancer. (2018) ;4: :18. doi: 10.1038/s41523-018-0071-9 |
[19] | Guo Y , Ji X , Liu J , Fan D , Zhou Q , Chen C , et al. Effects of exosomes on pre-metastatic niche formation in tumors. Mol. Cancer. (2019) ;18: :39. doi: 10.1186/s12943-019-0995-1 |
[20] | dos Anjos Pultz B , Andrés Cordero da Luz F , Socorro Faria S , Peixoto Ferreira de Souza L , Cristina Brígido Tavares P , Alonso Goulart V , et al. The multifaceted role of extracellular vesicles in metastasis: Priming the soil for seeding. Int J Cancer. (2017) ;140: :2397–407. doi: 10.1002/ijc.30595 |
[21] | Psaila B , Lyden D . The metastatic niche: Adapting the foreign soil. Nat. Rev. Cancer. (2009) ;9: :285–93. doi: 10.1038/nrc2621 |
[22] | Batista S , Gregório AC , Otake AH , Couto N , Costa-Silva B . The Gastrointestinal Tumor Microenvironment: An Updated Biological and Clinical Perspective. J Oncol. (2019) . doi: 10.1155/2019/6240505 |
[23] | Özdemir BC , Pentcheva-Hoang T , Carstens JL , Zheng X , Wu CC , Simpson TR , et al. Depletion of carcinoma-associated fibroblasts and fibrosis induces immunosuppression and accelerates pancreas cancer with reduced survival. Cancer Cell. (2014) ;25: (6):719–34. doi: 10.1016/j.ccr.2014.04.005 |
[24] | LeBleu VS , Kalluri R . A peek into cancer-associated fibroblasts: Origins, functions and translational impact. Dis Model Mech. (2018) ;11. doi: 10.1242/dmm.029447 |
[25] | Lee YT , Tan YJ , Falasca M , Oon CE . Cancer-associated fibroblasts: Epigenetic regulation and therapeutic intervention in breast cancer. Cancers. (2020) ;12: (10):1–23. doi: 10.3390/cancers12102949 |
[26] | Bu L , Baba H , Yasuda T , Uchihara T , Ishimoto T . Functional diversity of cancer-associated fibroblasts in modulating drug resistance. Cancer Sci. (2020) ;111: (10):3468–77. doi: 10.1111/cas.14578 |
[27] | Biffi G , Tuveson DA . Diversity and Biology of Cancer-Associated Fibroblasts. Physiol Rev. (2021) ;101: :147–76. doi: 10.1152/physrev.00048.2019 |
[28] | Perkins ND . NF-κB: Tumor promoter or suppressor? Trends Cell Biol. (2004) ;14: :64–9. doi: 10.1016/j.tcb.2003.12.004 |
[29] | Pallangyo CK , Ziegler PK , Greten FR . IKKβ acts as a tumor suppressor in cancer-associated fibroblasts during intestinal tumorigenesis. J Exp Med. (2015) ;212: :2253–66. doi: 10.1084/jem.20150576 |
[30] | Xia Y , Shen S , Verma IM . NF-κB, an active player in human cancers. Cancer Immunol. Res. (2014) ;2: (9):823–30. doi: 10.1158/2326-6066.CIR-14-0112 |
[31] | Nurmik M , Ullmann P , Rodriguez F , Haan S , Letellier E . In search of definitions: Cancer-associated fibroblasts and their markers. Int J Cancer. (2020) ;146: :895–905. doi: 10.1002/ijc.32193 |
[32] | Sun Y , Wang R , Qiao M , Xu Y , Guan W , Wang L . Cancer associated fibroblasts tailored tumor microenvironment of therapy resistance in gastrointestinal cancers. J. Cell. Physiol. (2018) ;233: (9):6359–69. doi: 10.1002/jc26433 |
[33] | Wang FT , Sun WEI , Zhang JT , Fan YZ . Cancer-associated fibroblast regulation of tumor neo-angiogenesis as a therapeutic target in cancer (Review). Oncol. Lett. (2019) ;17: (3):3055–65. doi: 10.3892/ol.2019.9973 |
[34] | Santi A , Kugeratski FG , Zanivan S . Cancer Associated Fibroblasts: The Architects of Stroma Remodeling. Proteomics. (2018) ;18: (5-6). doi: 10.1002/pmic.201700167 |
[35] | Pouysségur J , Dayan F , Mazure NM . Hypoxia signalling in cancer and approaches to enforce tumour regression. Nature. (2006) ;441: :437–43. doi: 10.1038/nature04871 |
[36] | Yoshida GJ . Metabolic reprogramming: The emerging concept and associated therapeutic strategies. J. ExClin. Cancer Res. (2015) ;34. doi: 10.1186/s13046-015-0221-y |
[37] | Prakash J . Cancer-Associated Fibroblasts: Perspectives in Cancer Therapy. Trends in Cancer. (2016) ;2: (6):277–9. doi: 10.1016/j.trecan.2016.04.005 |
[38] | Sahai E , Astsaturov I , Cukierman E , DeNardo DG , Egeblad M , Evans RM , et al. A framework for advancing our understanding of cancer-associated fibroblasts. Nat. Rev. Cancer. (2020) ;20: :174–86. doi: 10.1038/s41568-019-0238-1 |
[39] | Stoorvogel W , Kleijmeer MJ , Geuze HJ , Raposo G . The biogenesis and functions of exosomes. Traffic. (2002) ;3: (1):321–30. doi: 10.1034/j.1600-0854.2002.30502.x |
[40] | Zare N , Kefayat A , Javanmard S . Evaluation of radiation and ammonium lactate effects on hyaluronic acid expression as a pro-cancerous factor in supernatant and exosome isolated from supernatant of primary mouse fibroblast cell culture. Int J Prev Med. (2020) ;11: :125. doi: 10.4103/ijpvm.IJPVM_135_20 |
[41] | Meng X , Pan J , Sun S , Gong Z . Circulating exosomes and their cargos in blood as novel biomarkers for cancer. Transl Cancer Res. (2017) ;7: :S226–42. |
[42] | Kahlert C , Kalluri R . Exosomes in tumor microenvironment influence cancer progression and metastasis. J Mol Med. (2013) ;91: :431–437. doi: 10.1007/s00109-013-1020-6 |
[43] | Mentkowski KI , Snitzer JD , Rusnak S , Lang JK . Therapeutic Potential of Engineered Extracellular Vesicles. AAPS J. (2018) ;20: :1–17. doi: 10.1208/s12248-018-0211-z |
[44] | Popović M , Marco A de . Canonical and selective approaches in exosome purification and their implications for diagnostic accuracy. Transl Cancer Res. (2018) )7–. |
[45] | Oksvold MP , Neurauter A , Pedersen KW . Magnetic bead-based isolation of exosomes. In SioudM. (ed.) RNA Interference. Methods in Molecular Biology (Methods and Protocols), vol 1218: . New York: Humana Press. doi: 10.1007/978-1-4939-1538-5_27 |
[46] | Buschmann D , Kirchner B , Hermann S , Märte M , Wurmser C , Brandes F , et al. Evaluation of serum extracellular vesicle isolation methods for profiling miRNAs by next-generation sequencing. Journal of Extracellular Vesicles. (2018) ;7. doi: 10.1080/20013078.2018.1481321 |
[47] | Chen C , Skog J , Hsu CH , Lessard RT , Balaj L , Wurdinger T , et al. Microfluidic isolation and transcriptome analysis of serum microvesicles. Lab Chip. (2010) ;10: :505–11. doi: 10.1039/B916199F |
[48] | Kluszczyńska K , Czernek L , Cypryk W , Pęczek Ł , Düchler M . Methods for the Determination of the Purity of Exosomes. Current pharmaceutical design. (2019) ;25: (42):4464–85. doi: 10.2174/1381612825666191206162712 |
[49] | Zhang Y , Bi J , Huang J , Tang Y , Du S , Li P . Exosome: A review of its classification, isolation techniques, storage, diagnostic and targeted therapy applications. Int. J. Nanomedicine. (2020) ;15: :6917–34. doi: 10.2147/IJN.S264498 |
[50] | Bray F , Ferlay J , Soerjomataram I , Siegel RL , Torre LA , Jemal A . Global cancer statistics 2018: GLOBOCAN estimates of incidence and mortality worldwide for 36 cancers in 185 countries. CA Cancer J Clin. (2018) ;68: :394–424. doi: 10.3322/caac.21492 |
[51] | Sun Y , Wang R , Qiao M , Xu Y , Guan W , Wang L . Cancer associated fibroblasts tailored tumor microenvironment of therapy resistance in gastrointestinal cancers. J. Cell. Physiol. (2018) ;233: (9):6359–69. doi: 10.1002/jc26433 |
[52] | Rawla P , Sunkara T , Barsouk A . Epidemiology of colorectal cancer: Incidence, mortality, survival, and risk factors. Prz. Gastroenterol. (2019) ;14: (2):89–103. doi: 10.5114/pg.2018.81072 |
[53] | Hu Y , Yan C , Mu L , Huang K , Li X , Tao D , et al. Fibroblast-derived exosomes contribute to chemoresistance through priming cancer stem cells in colorectal cancer. PLoS One. (2015) ;10. doi: 10.1371/journal.pone.0125625 |
[54] | Hu JL , Wang W , Lan XL , Zeng ZC , Liang YS , Yan YR , et al. CAFs secreted exosomes promote metastasis and chemotherapy resistance by enhancing cell stemness and epithelial-mesenchymal transition in colorectal cancer. Mol Cancer. (2019) ;18. doi: 10.1186/s12943-019-1019-x |
[55] | Ren J , Ding L , Zhang D , Shi G , Xu Q , Theranostics SS- , et al. Carcinoma-associated fibroblasts promote the stemness and chemoresistance of colorectal cancer by transferring exosomal lncRNA H19. Theranostics. (2018) ;8: (14):3932–48. doi: 10.7150/thno.25541 |
[56] | Deng X , Ruan H , Zhang X , Xu X , Zhu Y , Peng H , et al. Long noncoding RNA CCAL transferred from fibroblasts by exosomes promotes chemoresistance of colorectal cancer cells. Int J Cancer. (2020) ;146: :1700–16. doi: 10.1002/ijc.32608 |
[57] | Bullock MD , Pickard KM , Nielsen BS , Sayan AE , Jenei V , Mellone M , et al. Pleiotropic actions of miR-21 highlight the critical role of deregulated stromal microRNAs during colorectal cancer progression. Cell Death Dis. (2013) ;4: :e684. doi: 10.1038/cddis.2013.213 |
[58] | Bhome R , Goh RW , Bullock MD , Pillar N , Thirdborough SM , Mellone M , et al. Exosomal microRNAs derived from colorectal cancer-associated fibroblasts: Role in driving cancer progression. Aging. (2017) ;9: :2666–94. doi: 10.18632/aging.101355 |
[59] | Hu YB , Yan C , Mu L , Mi YL , Zhao H , Hu H , et al. Exosomal Wnt-induced dedifferentiation of colorectal cancer cells contributes to chemotherapy resistance. Oncogene. (2019) ;38: :1951–65. doi: 10.1038/s41388-018-0557-9 |
[60] | Zhang Y . Epidemiology of esophageal cancer. World J. Gastroenterol. (2013) ;19: :5598–606. doi: 10.3748/wjg.v19.i34.5598 |
[61] | Di Pardo BJ , Bronson NW , Diggs BS , Thomas CR , Hunter JG , Dolan JP . The Global Burden of Esophageal Cancer: A Disability-Adjusted Life-Year Approach. World J Surg. (2016) ;40: :395–401. doi: 10.1007/s00268-015-3356-2 |
[62] | Zhao G , Li H , Guo Q , Zhou A , Wang X , Li P , et al. Exosomal Sonic Hedgehog derived from cancer-associated fibroblasts promotes proliferation and migration of esophageal squamous cell carcinoma. Cancer Med. (2020) ;9: :2500–13. doi: 10.1002/cam4.2873 |
[63] | Khazaei S , Nouraee N , Moradi A , Mowla SJ . A novel signaling role for miR-451 in esophageal tumor microenvironment and its contribution to tumor progression. Clin Transl Oncol. (2016) ;19: (5):633–640. doi: 10.1007/s12094-016-1575-0 |
[64] | Nouraee N , Khazaei S , Vasei M , Razavipour SF , Sadeghizadeh M , Javad Mowla S . MicroRNAs contribution in tumor microenvironment of esophageal cancer. Cancer Biomarkers. (2016) ;16: :367–76. doi: 10.3233/CBM-160575 |
[65] | Rawla P , Barsouk A . Epidemiology of gastric cancer: Global trends, risk factors and prevention. Prz. Gastroenterol. (2019) ;14: (1):26–38. doi: 10.5114/pg.2018.80001 |
[66] | Luo Z , Rong Z , Huang C . Surgery Strategies for Gastric Cancer With Liver Metastasis. Front. Oncol. (2019) ;9: :1353. doi: 10.3389/fonc.2019.01353 |
[67] | Fu M , Gu J , Jiang P , Qian H , Xu W , Zhang X . Exosomes in gastric cancer: Roles, mechanisms, and applications. Mol. Cancer. (2019) ;18: :41. doi: 10.1186/s12943-019-1001-7 |
[68] | Wang R , Sun Y , Yu W , Yan Y , Qiao M , Jiang R , et al. Downregulation of miRNA-214 in cancer-associated fibroblasts contributes to migration and invasion of gastric cancer cells through targeting FGF9 and inducing EMT. J Exp Clin Cancer Res. (2019) ;38: :20. doi: 10.1186/s13046-018-0995-9 |
[69] | Miki Y , Yashiro M , Okuno T , Kitayama K , Masuda G , Hirakawa K , et al. CD9-positive exosomes from cancerassociated fibroblasts stimulate the migration ability of scirrhous-type gastric cancer cells. Br J Cancer. (2018) ;118: :867–77. doi: 10.1038/bjc.2017.487 |
[70] | Zhang H , Deng T , Liu R , Ning T , Yang H , Liu D , et al. CAF secreted miR-522 suppresses ferroptosis and promotes acquired chemo-resistance in gastric cancer. Mol Cancer. (2020) ;19: :43. doi: 10.1186/s12943-020-01168-8 |
[71] | Jabłońska-Trypuć A , Matejczyk M , Rosochacki S , Jabłoń AJ , Trypuć J-T . Matrix metalloproteinases (MMPs), the main extracellular matrix (ECM) enzymes in collagen degradation, as a target for anticancer drugs Matrix metalloproteinases (MMPs), the main extracellular matrix (ECM) enzymes in collagen degradation, as a target for anticancer drugs. J Enzym Inhib Med Chem. (2016) ;31: :177–83. doi: 10.3109/14756366.2016.1161620 |
[72] | Xu G , Zhang B , Ye J , Cao S , Shi J , Zhao Y , et al. Exosomal miRNA-139 in cancer-associated fibroblasts inhibits gastric cancer progression by repressing mmp11 expression. Int J Biol Sci. (2019) ;15: :2320–9. doi: 10.7150/ijbs.33750 |
[73] | Dai J , Su Y , Zhong S , Cong L , Liu B , Yang J , et al. Exosomes: key players in cancer and potential therapeutic strategy. Signal Transduct. Target Ther. (2020) ;5: :1–10. doi: 10.1038/s41392-020-00261-0 |
[74] | Zhang H , Zhu L , Bai M , Liu Y , Zhan Y , Deng T , et al. Exosomal circRNA derived from gastric tumor promotes white adipose browning by targeting the miR-133/PRDM16 pathway. Int J Cancer. (2019) ;144: :2501–15. doi: 10.1002/ijc.31977 |
[75] | Rawla P , Sunkara T , Gaduputi V . Epidemiology of Pancreatic Cancer: Global Trends, Etiology and Risk Factors. World J Oncol. (2019) ;10: :10–27. doi: 10.14740/wjon1166 |
[76] | Pourshams A , Sepanlou SG , Ikuta KS , Bisignano C , Safiri S , Roshandel G , et al. The global, regional, and national burden of pancreatic cancer and its attributable risk factors in 195 countries and territories, 1990-2017: a systematic analysis for the Global Burden of Disease Study 2017. Lancet Gastroenterol Hepatol. (2019) ;4: :934–47. doi: 10.1016/S2468-1253(19)30347-4 |
[77] | Richards KE , Zeleniak AE , Fishel ML , Wu J , Littlepage LE , Hill R . Cancer-associated fibroblast exosomes regulate survival and proliferation of pancreatic cancer cells. Oncogene. (2017) ;36: :1770–8. doi: 10.1038/onc.2016.353 |
[78] | Fang Y , Zhou W , Rong Y , Kuang T , Xu X , Wu W , et al. Exosomal miRNA-106b from cancer-associated fibroblast promotes gemcitabine resistance in pancreatic cancer. Exp Cell Res. (2019) ;383: :1115–43. doi: 10.1016/j.yexcr.2019.111543 |
[79] | Leca J , Martinez S , Lac S , Nigri J , Secq V , Rubis M , et al. Cancer-associated fibroblast-derived annexin A6+extracellular vesicles support pancreatic cancer aggressiveness. J Clin Invest. (2016) ;126: :4140–56. doi: 10.1172/JCI87734 |
[80] | Zhao H , Yang L , Baddour J , Achreja A , Bernard V , Moss T , et al. Tumor microenvironment derived exosomes pleiotropically modulate cancer cell metabolism. Elife. (2016) ;5. doi: 10.7554/eLife.10250 |
[81] | Salehiniya H , Mohammadian M , Mahdavifar N , Mohammadian-Hafshejani A , Salehiniya H . Liver cancer in the world: epidemiology, incidence, mortality and risk factors. WCRJ. (2018) ;5: (2):e1082. |
[82] | Zhang Z , Li X , Sun W , Yue S , Yang J , Li J , et al. Loss of exosomal miR-320a from cancer-associated fibroblasts contributes to HCC proliferation and metastasis. Cancer Lett. (2017) ;397: :33–42. doi: 10.1016/j.canlet.2017.03.004 |
[83] | Li L , Piontek K , Ishida M , Fausther M , Dranoff JA , Fu R , et al. Extracellular vesicles carry microRNA-195 to intrahepatic cholangiocarcinoma and improve survival in a rat model. Hepatology. (2017) ;65: :501–14. doi: 10.1002/he28735 |