Exploring active ingredients and mechanisms of Coptidis Rhizoma-ginger against colon cancer using network pharmacology and molecular docking
Abstract
BACKGROUND:
Colon cancer is the most prevalent and rapidly increasing malignancy globally. It has been suggested that some of the ingredients in the herb pair of Coptidis Rhizoma and ginger (Zingiber officinale), a traditional Chinese medicine, have potential anti-colon cancer properties.
OBJECTIVE:
This study aimed to investigate the molecular mechanisms underlying the effects of the Coptidis Rhizoma-ginger herb pair in treating colon cancer, using an integrated approach combining network pharmacology and molecular docking.
METHODS:
The ingredients of the herb pair Coptidis Rhizoma-ginger, along with their corresponding protein targets, were obtained from the Traditional Chinese Medicine System Pharmacology and Swiss Target Prediction databases. Target genes associated with colon cancer were retrieved from the GeneCards and OMIM databases. Then, the protein targets of the active ingredients in the herb pair were identified, and the disease-related overlapping targets were determined using the Venn online tool. The protein-protein interaction (PPI) network was constructed using STRING database and analyzed using Cytoscape 3.9.1 to identify key targets. Then, a compound-target-disease-pathway network map was constructed. The intersecting target genes were subjected to Gene Ontology (GO) enrichment and Kyoto Encyclopedia of Genes and Genomes (KEGG) pathway analyses for colon cancer treatment. Molecular docking was performed using the Molecular Operating Environment (MOE) software to predict the binding affinity between the key targets and active compounds.
RESULTS:
Besides 1922 disease-related targets, 630 targets associated with 20 potential active compounds of the herb pair Coptidis Rhizoma-ginger were collected. Of these, 229 intersection targets were obtained. Forty key targets, including STAT3, Akt1, SRC, and HSP90AA1, were further analyzed using the ClueGO plugin in Cytoscape. These targets are involved in biological processes such as miRNA-mediated gene silencing, phosphatidylinositol 3-kinase (PI3K) signaling, and telomerase activity. KEGG enrichment analysis showed that PI3K-Akt and hypoxia-inducible factor 1 (HIF-1) signaling pathways were closely related to colon cancer prevention by the herb pair Coptidis Rhizoma-ginger. Ten genes (Akt1, TP53, STAT3, SRC, HSP90AA1, JAK2, CASP3, PTGS2, BCl2, and ESR1) were identified as key genes for validation through molecular docking simulation.
CONCLUSIONS:
This study demonstrated that the herb pair Coptidis Rhizoma-ginger exerted preventive effects against colon cancer by targeting multiple genes, utilizing various active compounds, and modulating multiple pathways. These findings might provide the basis for further investigations into the molecular mechanisms underlying the therapeutic effects of Coptidis Rhizoma-ginger in colon cancer treatment, potentially leading to the development of novel drugs for combating this disease.
1.Introduction
Colon cancer is the third most common malignant tumor globally. The incidence and mortality of colon cancer continue to rise each year due to factors such as poor environmental conditions and unhealthy lifestyles [1, 2]. According to the American Cancer Society, approximately 149,500 newly cases of colon cancer were diagnosed and about 52,980 deaths were attributed to the disease in 2021. In recent years, it has been recognized that tumor prevention is one of the most promising approach to combating cancer. It involves using natural or synthetic compounds to inhibit or reverse tumorigenesis. Therefore, the search for low-toxicity and high-efficiency anticancer drugs has a broad application prospect and great practical significance. In recent decades, the compounds extracted from traditional Chinese medicine (TCM) for modern drug development have gained widespread attention. TCM has a long history of clinical application in tumor prevention and treatment, characterized by low toxicity, minimal side effects, and cost-effectiveness [3]. In addition, previous studies have reported herbal formulas as important therapeutic strategies and a new avenue in cancer chemotherapy [4]. Herb pairs are the simplest and most fundamental form of prescription therapy in TCM.
In TCM, Coptidis Rhizoma belongs to the buttercup family and consists of berberine, coptisine, palmidin A, quercetin, and other compounds. Its main functions include clearing away heat, drying up dampness, treating diarrhea, and detoxifying. It was also often prescribed to patients as one of the significant ingredients in TCM composite formulas. Several clinical studies have shown that berberine is safe and may reduce the risk of colorectal adenoma recurrence, making it a potential option for chemoprevention after polypectomy [5, 6]. Ginger (Zingiber officinale) is the rhizome of a perennial herb from the ginger family, long used as both a spice and medicine in Asian countries [7]. The health-promoting effects of ginger include gastrointestinal protection, immunomodulation, antilipidemic effects, antimicrobial activity, and other beneficial biological effects. The ginger extract has been demonstrated to be effective in preventing and treating cancer, attributed to compounds like 6-gingerol and 6-shogaol. These compounds exhibit immunomodulatory, antioxidant, and anticancer activities. In vitro experiments have demonstrated the anticancer activities of these compounds against gastrointestinal cancer. These activities attributed to the ability of these compounds to modulate the functions of several signaling molecules, such as STAT3, Akt, cyclooxygenase-2 (COX-2), Bcl-2, caspases, and other cell growth regulatory proteins [8, 9]. TCM is usually used in the form of compounded or paired medicines. It often follows the principle of “sovereign-minister-assistant-envoy” (Jun-Chen-Zuo-Shi in Chinese) to achieve comprehensive therapeutic effects via the combination of various herbs. The synergistic effects of TCM can improve therapeutic efficacy or reduce toxicity [10, 11]. However, a large number of compounds in the herb pair Coptidis Rhizoma-ginger have multiple targets and mechanisms in treating diseases. Hence, the combinatorial rules and roles of herb pairs in treating diseases remain to be elucidated. TCM and related formulas treat diseases synergistically via multi-target, multi-ingredient, and multi-pathway mechanisms.
Therefore, combining biological networks with drug action networks is essential to make new discoveries. Network pharmacology is a new paradigm that integrates the existing massive database of bioinformatics and pharmacological analysis to elucidate the multi-compound and multi-target relationships between various TCM herbs and reveal formulas for treating complicated diseases [12]. The Chinese medicine Pulsatilla decoction has some therapeutic effects on colon cancer. “Monotherapy” refers to the use of a single medicine to treat a disease. This study aimed to establish a drug-compound-disease-target-pathway network to investigate the potential mechanisms of the herb pair Coptidis Rhizoma-ginger in preventing colon cancer [13]. We used molecular docking to screen potential target genes, providing a theoretical foundation for further investigations and reasonable clinical applications of the compounds against colon cancer. The detailed workflow of the study design is shown in Fig. 1.
Figure 1.
Detailed workflow of the study design.
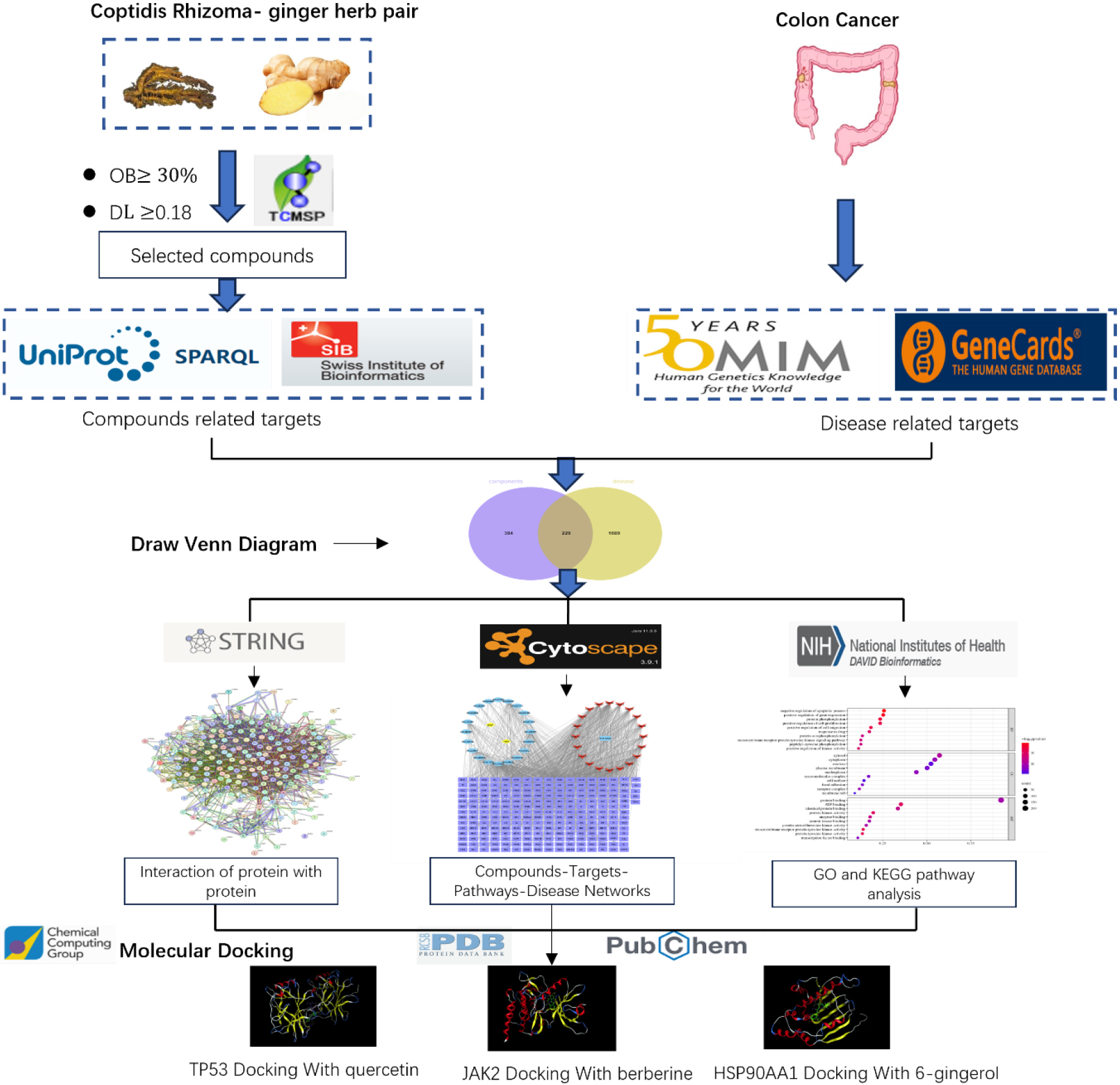
2.Materials and methods
2.1Collection of the active compounds
The Traditional Chinese Medicine System Pharmacology (TCMSP, http://tcmspw.com/tcmsp.php) database includes 499 Chinese medicines registered in Chinese Pharmacopoeia, containing 29,384 ingredients, 3311 targets, 837 related diseases, and pharmacokinetics. TCMSP provides information on the identification of the active ingredients, chemicals, and drug-target network, network of associated diseases, and pharmacokinetic properties of natural compounds, including oral bioavailability (OB), drug-likeness (DL), blood-brain barrier (BBB), drug similarity, and water solubility [14]. The TCMSP platform was used to retrieve the active ingredients of Coptidis Rhizoma and ginger based on the criteria of OB
A threshold of OB
OB is defined as the rate and extent of absorption of the active moiety from the drug product. High OB is often a key indicator in determining whether a bioactive molecule has pharmaceutical properties as a therapeutic. DL is the relative amount and rate of absorption of an orally administered drug into the body’s circulation. It is a qualitative property of a chemical widely used in drug discovery in an early stage.
2.2Screening for colon cancer-related genes
Using “colon tumor” or “colon cancer” as keywords, we searched the OMIM database (https://www. omim.org/) and GeneCards database (https://www.genecards.org/). The GeneCards database was used to screen targets with a relevance score
2.3Clustering of Coptidis Rhizoma-ginger and colon cancer-related target genes
Subsequently, the protein targets of potential active ingredients of Coptidis Rhizoma and ginger were collected through TCMSP. The UniProt (https://www.uniprot.org/) database was used to standardize the names of protein targets to their official names, restricting the species to “Homo sapiens” Eventually, the protein targets of the active ingredients were obtained and converted into gene symbols. The SMILES representations of the active ingredients in Coptidis Rhizoma and ginger were molecularly analyzed using Swiss Target Prediction (http://www.swisstargetprediction.ch/), and potential targets with a probability
The target genes were intersected with colon cancerrelated genes using the Venn online tool (https://jvenn.toulouse.inrae.fr/app/example.html). The resulting intersected genes were identified as potential target genes for drugs that act on diseases [15].
2.4Protein-protein interaction network
Protein-protein interaction (PPI) network is a network of proteins interacting with each other to participate in vital processes. It is constructed for processes such as biological signaling, regulation of gene expression, energy and material metabolism, cell cycle regulation, and co-localization of potential target genes interacting with predicted genes [16]. The potential genes were imported to the STRING database platform (https://string-db.org) to obtain PPI relationship. Cytoscape software, version 3.9.1, was used for visualizing the PPI network [17]. The CytoNCA plugin was used to further analyze the topology of this network based on the conditions of betweenness, closeness, and degree, which were calculated to identify the key genes. The “degree” parameter represented the degree of the calculated node, that is, the number of edges directly connected to the node. “Betweenness” indicated the mediating role of a node in the shortest path of a network, whereas “closeness” indicated the average distance of a node to other nodes.
2.5Construction of drug-compound-disease-target-pathway network
A visual network of drug-compound-disease-target-pathway was established through Cytoscape software, version 3.9.1, to characterize the mechanism of action of the herb pair Coptidis Rhizoma-ginger for preventing and treating colon cancer. In this network, each node of different colors and shapes represented drugs, compounds, diseases, targets, or disease-related pathways, whereas edges represented the associations between nodes.
2.6Analysis of GO and KEGG pathway enrichment
The potential target genes were imported into the DAVID database (https://david.ncifcrf.gov/) for analysis of biological function enrichment using Gene Ontology (GO) and pathway analysis using the Kyoto Encyclopedia of Genes and Genomes (KEGG). GO functionally annotated the key genes into three main categories: cellular component (CC), molecular function (MF), and biological process (BP). Besides, the enrichment of BPs for key genes was further analyzed using Cytoscape’s ClueGO plugin. Moreover, bubble charts for GO and KEGG enrichment analysis were generated using the bioinformatics platform (http://www.bioinformatics.com.cn/) with a filtering condition set at
2.7Evaluation of binding capacity of active ingredients to key genes using molecular docking
Ten key genes were selected as the core receptor proteins. The names of proteins were entered into the PDB database (http://www.rcsb.org/pdb/), with the species set to “Homo sapiens” to obtain highly selective 3D target protein structures. The receptor proteins were imported to MOE software, and then the water and original ligand molecules were removed. Before molecular docking, the “QuickPrep” function was used to pre-process the receptor protein structure. The QuickPrep panel provided information such as structural problem corrections, protonation, deletion of unbound water molecules, and energy optimization. The screened active compounds were prepared using “Prepare Ligands” for molecular docking, which were downloaded from the PubChem database. It is generally accepted that a binding energy of <-5.0 kcal/mol (1 cal
3.Results
3.1Active ingredient and potential genes associated with colon cancer
A total of 313 active ingredients of the herb pair Coptidis Rhizoma-ginger were searched and collected in the TCMSP database, and 20 potential active compounds were identified to establish the database using OB
Table 1
Characterization of the active ingredients of the herb pair Coptidis Rhizoma-ginger
ID | Ingredient | Weight | OB | DL |
---|---|---|---|---|
MOL001454 | Berberine | 336.39 | 36.86 | 0.78 |
MOL013352 | Obacunone | 454.56 | 43.29 | 0.77 |
MOL002894 | Berberrubine | 322.36 | 35.74 | 0.73 |
MOL002897 | Epiberberine | 336.39 | 43.09 | 0.78 |
MOL002903 | (R)-Canadine | 339.42 | 55.37 | 0.77 |
MOL002904 | Berlambine | 351.38 | 36.68 | 0.82 |
MOL002907 | Corchoroside A_qt | 404.55 | 104.95 | 0.78 |
MOL000622 | Magnograndiolide | 266.37 | 63.71 | 0.19 |
MOL000762 | Palmidin A | 510.52 | 35.36 | 0.65 |
MOL000785 | Palmatine | 352.44 | 64.60 | 0.65 |
MOL000098 | Quercetin | 302.25 | 46.43 | 0.28 |
MOL001458 | Coptisine | 320.34 | 30.67 | 0.86 |
MOL002668 | Worenine | 334.37 | 45.83 | 0.87 |
MOL008647 | Moupinamide | 313.38 | 86.71 | 0.26 |
MOL000358 | Beta-sitosterol | 414.79 | 36.91 | 0.75 |
MOL006129 | 6-Methylgingediacetate2 | 394.56 | 48.73 | 0.32 |
MOL000449 | Stigmasterol | 412.77 | 43.83 | 0.76 |
MOL001771 | Poriferast-5-en-3beta-ol | 414.79 | 36.91 | 0.75 |
MOL008698 | Dihydrocapsaicin | 307.48 | 47.07 | 0.19 |
MOL002467 | 6-gingerol | 294.43 | 35.64 | 0.16 |
DL, Drug-likeness; OB, oral bioavailability.
A total of 196 protein targets for the active ingredients of 2 herbs, denoted by a set
The sets
Figure 2.
Potential target genes and PPI network map of the herb pair Coptidis Rhizoma-ginger for treating colon cancer.
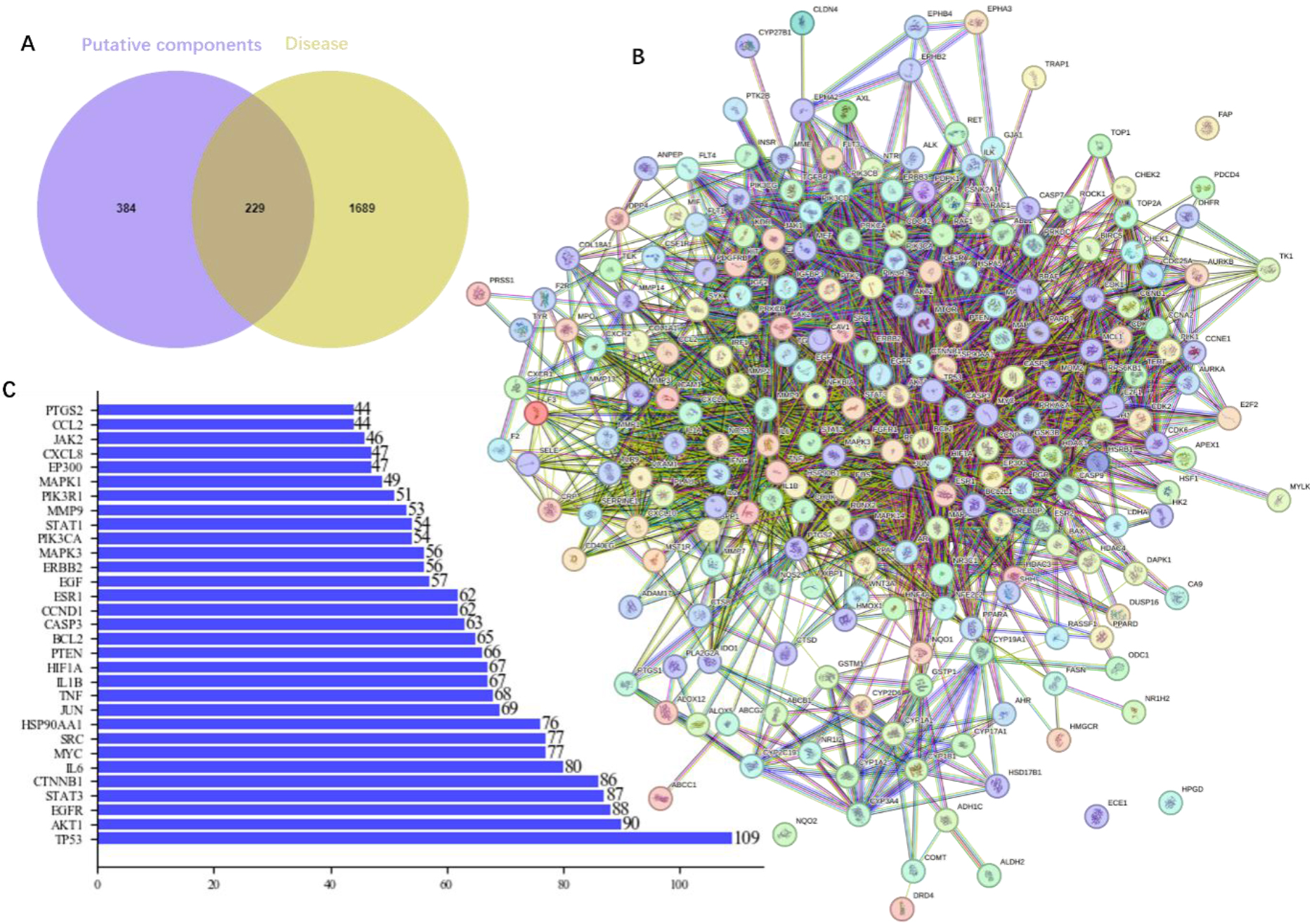
3.2PPI network analysis
Subsequently, 229 potential genes were imported into the STRING database to obtain the PPI network (Fig. 2B), and the species was restricted to “Homo sapiens” with interaction score
Table 2
Key targets of the herb pair Coptidis Rhizoma-ginger for treating colon cancer and the topological parameters
Uniprot ID | Gene symbol | Target name | Degree | Betweenness | Closeness |
---|---|---|---|---|---|
P40763 | STAT3 | Signal transducer and activator of transcription 3 | 87 | 0.038358384 | 0.587926509 |
P04626 | ERBB2 | Receptor protein-tyrosine kinase erbB-2 | 56 | 0.034872241 | 0.549019608 |
P00519 | ABL1 | Tyrosine-protein kinase ABL | 24 | 0.016354688 | 0.468619247 |
P28482 | MAPK1 | MAP kinase ERK2 | 49 | 0.011382439 | 0.527058824 |
P24385 | CCND1 | G1/S-specific cyclin-D1 | 62 | 0.020038187 | 0.55308642 |
P42336 | PIK3CA | PI3-kinase p110-alpha subunit | 54 | 0.010057476 | 0.520930233 |
P04637 | TP53 | Cellular tumor antigen p53 | 109 | 0.088584691 | 0.629213483 |
P00533 | EGFR | Epidermal growth factor receptor erbB1 | 88 | 0.058201474 | 0.602150538 |
P07900 | HSP90AA1 | Heat-shock protein HSP 90-alpha | 76 | 0.035989073 | 0.574358974 |
P05412 | JUN | Transcription factor AP-1 | 69 | 0.021902088 | 0.572890026 |
Q13950 | RUNX2 | Runt-related transcription factor 2 | 31 | 0.006606961 | 0.496674058 |
P01106 | MYC | Myc proto-oncogene protein | 77 | 0.033856568 | 0.571428571 |
P27986 | PIK3R1 | PI3-kinase p85-alpha subunit | 51 | 0.012591612 | 0.516129032 |
P35222 | CTNNB1 | Axin1/beta-catenin | 86 | 0.048210833 | 0.591029024 |
P01133 | EGF | Pro-epidermal growth factor | 57 | 0.020019528 | 0.538461538 |
P01375 | TNF | TNF-alpha | 68 | 0.029664049 | 0.554455446 |
P03372 | ESR1 | Estrogen receptor alpha | 62 | 0.050590907 | 0.56 |
P31749 | Akt1 | Serine/threonine-protein kinase Akt | 90 | 0.043940933 | 0.595744681 |
P13500 | CCL2 | C-C motif chemokine 2 | 44 | 0.010335114 | 0.496674058 |
P27361 | MAPK3 | MAP kinase ERK1 | 56 | 0.018673085 | 0.535885167 |
Q09472 | EP300 | Histone acetyltransferase p300 | 47 | 0.007116727 | 0.518518519 |
P01584 | IL1B | Interleukin-1 beta | 67 | 0.027188709 | 0.554455446 |
P37231 | PPARG | Peroxisome proliferator-activated receptor gamma | 37 | 0.01284196 | 0.511415525 |
P10721 | KIT | Stem cell growth factor receptor | 24 | 0.008596561 | 0.476595745 |
P10145 | CXCL8 | Interleukin-8 | 47 | 0.010735048 | 0.513761468 |
P42574 | CASP3 | Caspase-3 | 63 | 0.016300855 | 0.537170264 |
Q03135 | CAV1 | Caveolin-1 | 35 | 0.011551564 | 0.507936508 |
Q05397 | PTK2 | Focal adhesion kinase 1 | 35 | 0.007114828 | 0.494481236 |
P42224 | STAT1 | Signal transducer and activator of transcription 1-alpha/beta | 54 | 0.007898369 | 0.533333333 |
P35354 | PTGS2 | Cyclooxygenase-2 | 44 | 0.030673592 | 0.525821596 |
P60484 | PTEN | Phosphatidylinositol-3,4,5-trisphosphate 3-phosphatase and dual-specificity protein phosphatase PTEN | 66 | 0.022175915 | 0.549019608 |
P14780 | MMP9 | Matrix metalloproteinase 9 | 53 | 0.015857026 | 0.533333333 |
P12931 | SRC | Tyrosine-protein kinase SRC | 77 | 0.045362234 | 0.574358974 |
P10275 | AR | Androgen receptor | 32 | 0.010946326 | 0.497777778 |
O60674 | JAK2 | Tyrosine-protein kinase JAK2 | 46 | 0.006304914 | 0.512585812 |
P45983 | MAPK8 | c-Jun N-terminal kinase 1 | 35 | 0.006357242 | 0.50678733 |
P10415 | BCL2 | Apoptosis regulator Bcl-2 | 65 | 0.019562525 | 0.55308642 |
P05231 | IL6 | Interleukin-6 | 80 | 0.046656831 | 0.57881137 |
Q16665 | HIF1A | Hypoxia-inducible factor 1-alpha | 67 | 0.023429357 | 0.55721393 |
P17612 | PRKACA | cAMP-dependent protein kinase alpha-catalytic subunit | 31 | 0.011280685 | 0.474576271 |
Figure 3.
Representative map of protein-protein cluster network. The darker the red color, the stronger the correlation. (A) One protein-protein cluster with 31 nodes and 337 edges. (B) One protein-protein cluster with 31 nodes and 149 edges. (C) One protein-protein cluster with 26 nodes and 93 edges.
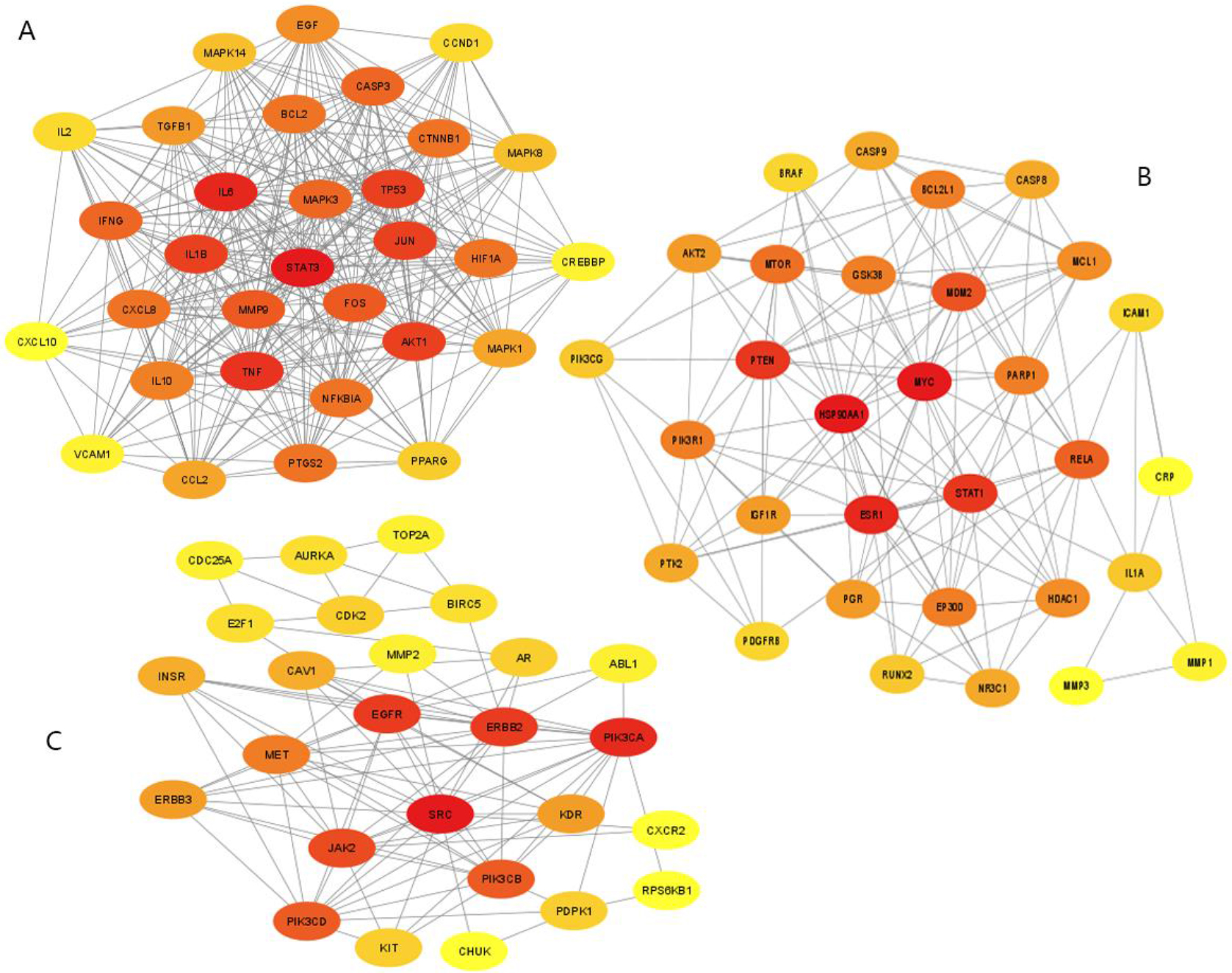
Cytoscape’s plugin MCDE was used to further analyze the PPI network (Fig. 2B), which consisted of 225 nodes and 2555 interaction edges, with 4 unconnected nodes deleted. In addition, the top three PPI cluster networks were established (Fig. 3A–C). Genes STAT3, IL6, Akt1, TNF, TP53, JUN, ILB1, and Akt1 were as the core genes of the first cluster network (31 nodes and 337 edges). MYC and HSP90AA1 were the core genes of the second cluster network (31 nodes and 149 edges), whereas the third cluster network (26 nodes and 93 edges) had SRC and PIK3CA as the core genes.
3.3Drug-compound-disease-target-pathway
The network of drug-compound-disease-target-pathway is shown in Fig. 4, including 272 nodes (20 compounds, 229 target genes, 20 pathways, 2 drugs, and 1 disease) and 311 edges. The yellow prismatic nodes indicated the Chinese herbs Coptidis Rhizoma and ginger. The blue rectangles indicated colon cancer, and the V nodes indicated the 20 significant signaling pathways (the top of 20 pathways in Fig. 4). The purple rectangular nodes indicated the 229 candidate targets, the blue elliptical nodes represented the 20 potential active compounds, and the connecting lines represented interactions. According to the network analysis, compounds from the herb pair Coptidis Rhizoma-ginger acted on at least one target gene, with quercetin (MOL000098) and 6-gingerol (MOL002467) considered as the most important and effective compounds interacting with 133 target genes. Furthermore, most of the target genes were regulated by at least two active compounds and four genes potentially involved in each pathway associated with colon cancer. The network analysis showed that the herb pair Coptidis Rhizoma-ginger had multi-compound and multi-target therapeutic characteristics against colon cancer.
Figure 4.
Potential target gene network of the herb pair Coptidis Rhizoma-ginger.
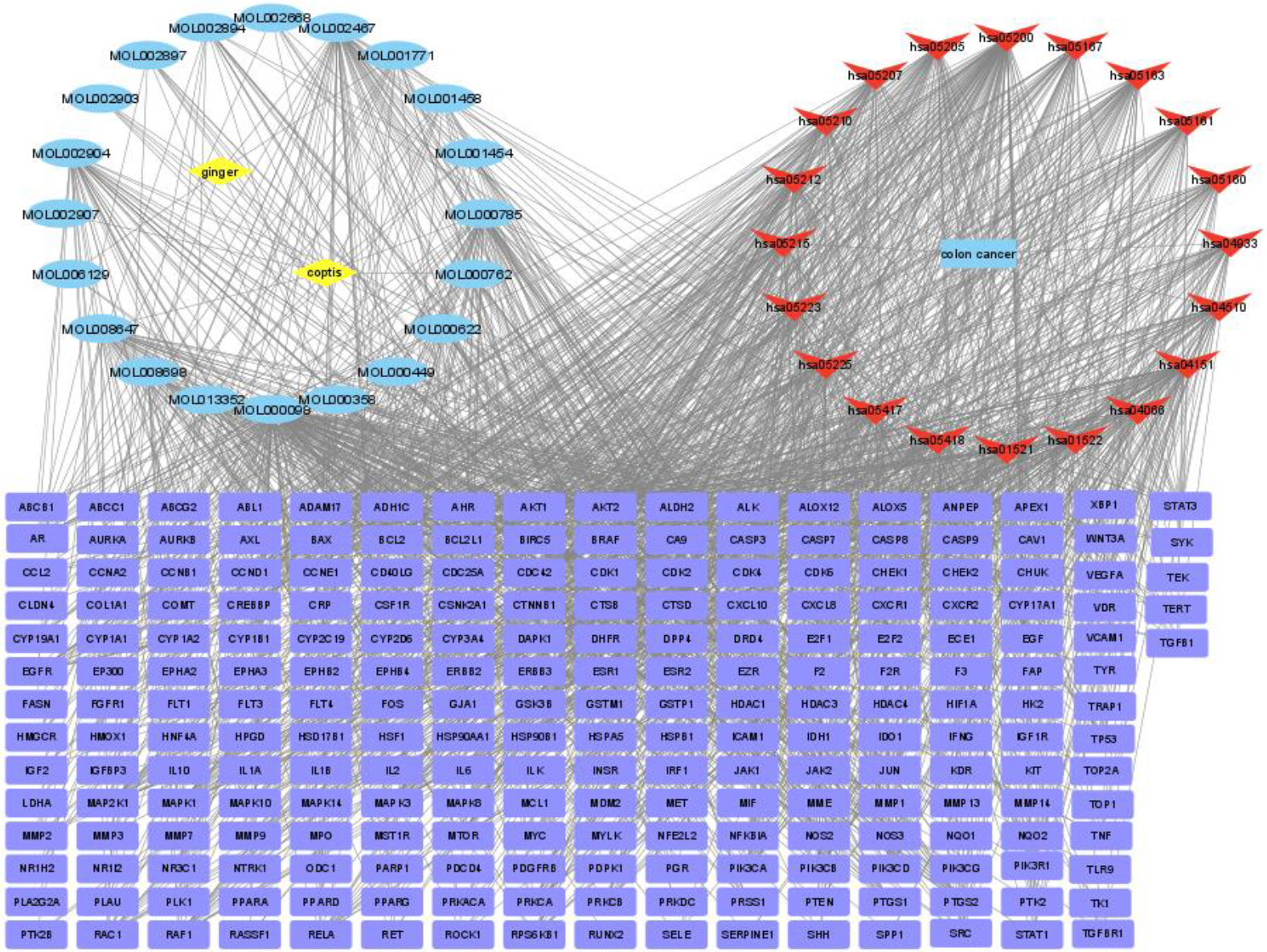
3.4GO enrichment analysis
A total of 229 potential genes were obtained from GO and KEGG enrichment analysis (
Figure 5.
Enrichment analysis of BPs, CCs, and MFs of the top 10 key targets. (A) Bubble chart illustrating GO enrichment analysis. (B) Visualization analysis of the 40 key genes.
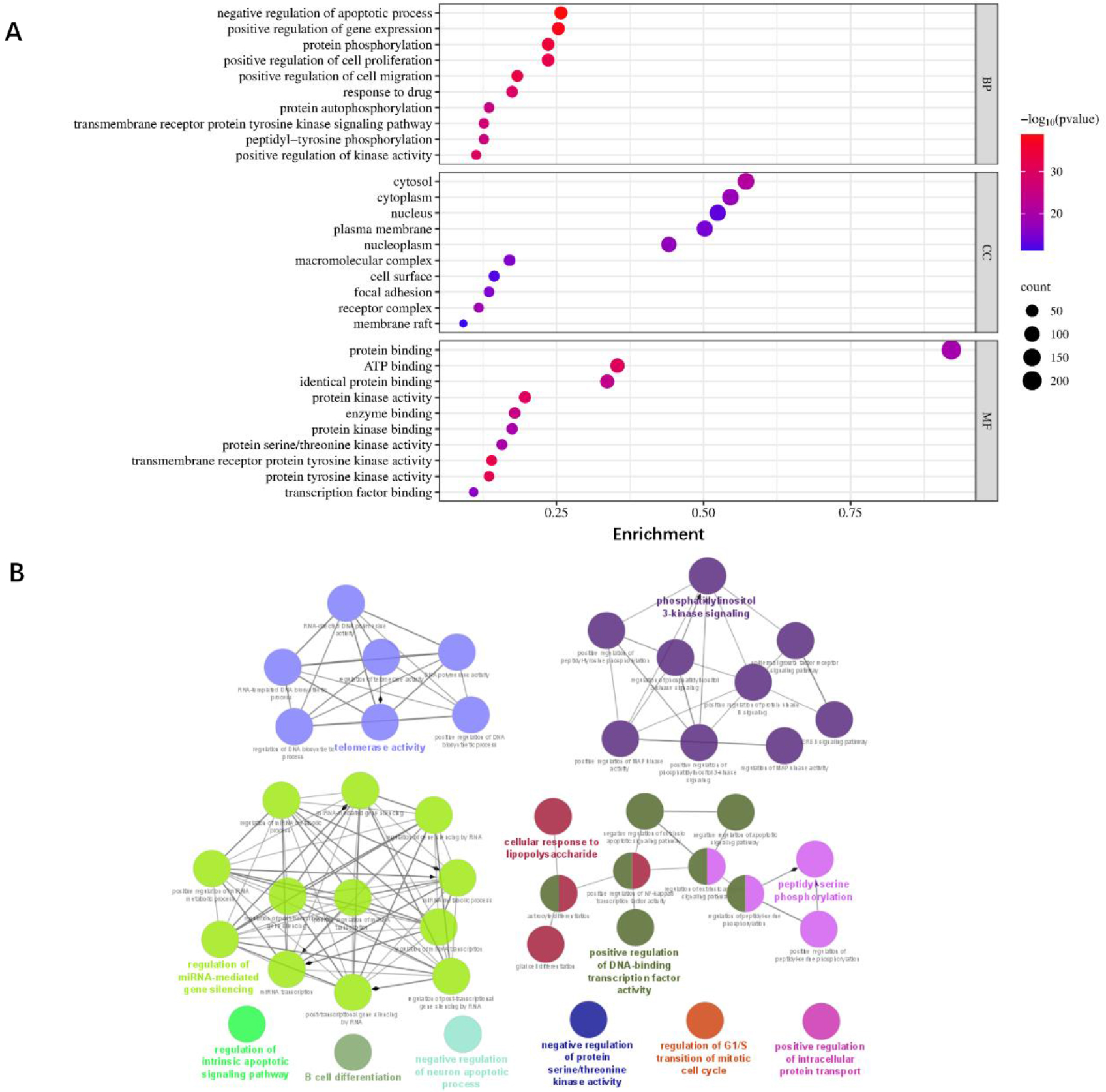
3.5KEGG pathway enrichment analysis
The KEGG pathway enrichment analysis was used to investigate how the herb pair Coptidis Rhizoma-ginger acted on the signaling pathway. The top 20 significant signaling pathways with
Figure 6.
Top 20 remarkably enriched KEGG signaling pathway analyses of the herb pair in colon cancer.
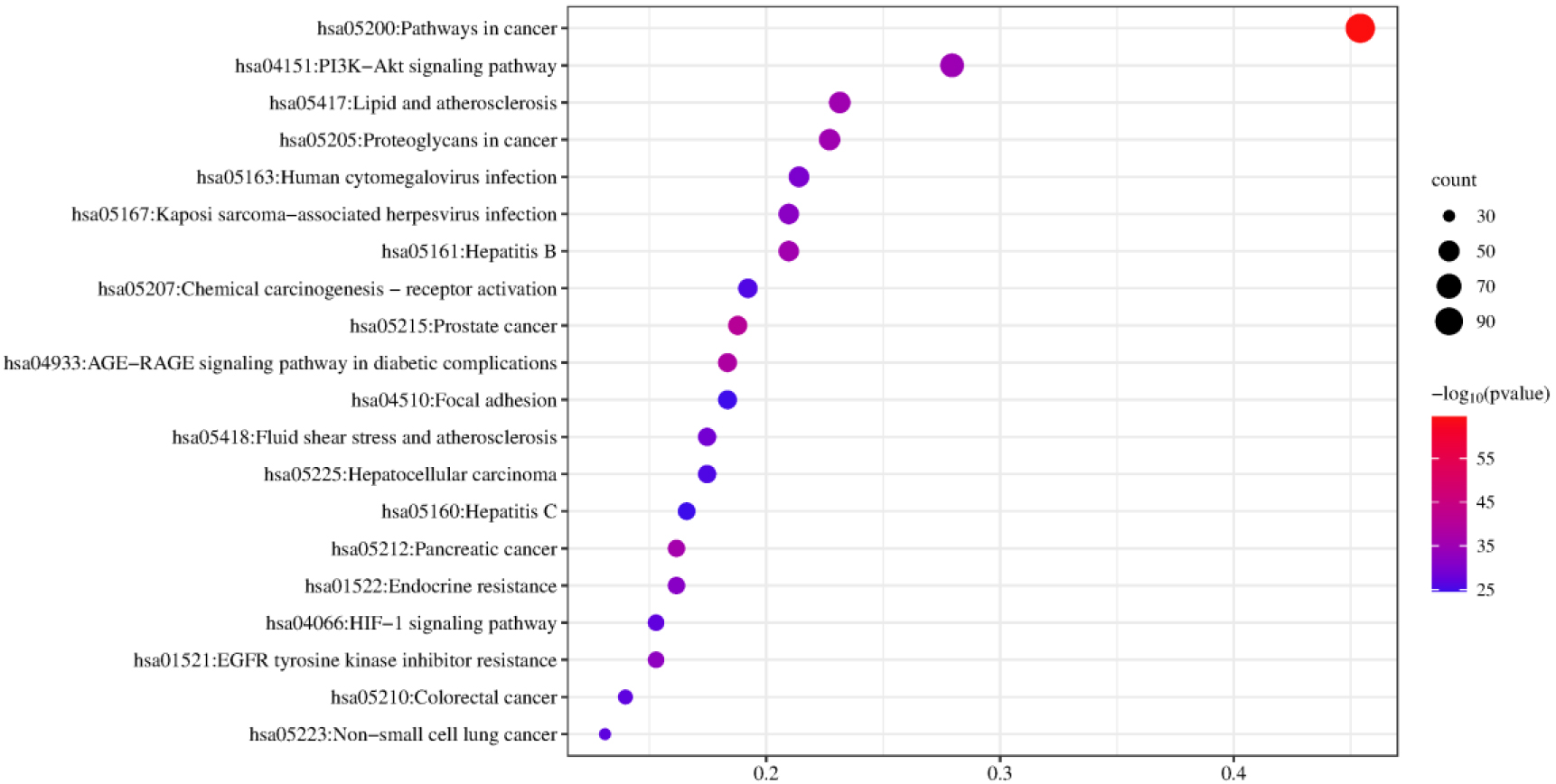
3.6Molecular docking results and analysis
A total of 10 key genes (Akt1, TP53, STAT3, SRC, HSP90AA1, JAK2, CASP3, PTGS2, Bcl2, and ESR1) displayed strong interactions with potential compounds, pathways, and other genes. Therefore, the 10 key genes were selected as the targets for molecular docking. Molecular docking technology, as an emerging technology for drug molecular screening, uses the “lock and key principle” of one-to-one correspondence between ligands and receptors, which helps in the computer system-assisted high-throughput screening of drug molecules.
After the docking was completed using MOE software, the docking results were viewed in the newly opened Database Viewer window. In the S column, a smaller negative value indicated that the ligand molecule bound more tightly to the receptor. Different scoring functions provided different results. In this case, we chose London dG Scoring to evaluate the free energy of binding of a ligand from a given pose with the following function.
Where
The results of binding energies and scores of the compounds docking with the proteins are presented in Table 3. The results of low free energy of binding for target-protein docking were visualized using MOE software (Fig. 8).
4.Discussion
The incidence of colon cancer is high due to the high intake of red meat and a low intake of dietary fiber. Additionally, factors such as obesity, lack of physical exercise, smoking, and alcoholism have been shown to further increase the risk of colon cancer [23]. Therefore, many Chinese herbs not only serve as medicines but also are used in the daily diet and as cancer prophylactic in patients who prefer healthy treatment after tumor surgery. Network pharmacology has become a promising tool to analyze the network of interactions among drug, targets, and diseases in recent years. In this study, the molecular mechanisms underlying the multi-targeted action of the herb pair Coptis Rhizoma-ginger in colon cancer was investigated using network pharmacology and molecular docking. The study showed that the herb pair could prevent colon cancer through multi-compound, multi-target, and multi-pathway modulation.
Table 3
Binding energies and scores of 10 core targets and their interacting compounds
Target gene | PDB ID | Components | Molecule name | London dG score | |
---|---|---|---|---|---|
Akt1 | 2uzs | MOL000098 | Quercetin | | |
CASP3 | 3H0E | MOL002467 | 6-Gingerol | | |
TP53 | 5O1F | MOL000098 | Quercetin | | |
STAT3 | 6gfa | MOL001454 MOL002467 MOL000098 | Berberine Gingerol Quercetin | | |
SRC | 2bdf | MOL000098 | Quercetin | | |
HSP90AA1 | 1BYQ | MOL002467 | 6-Gingerol | | |
JAK2 | 5aep | MOL001454 MOL001458 | Berberine Coptisine | | |
BCl2 | 5uuk | MOL000762 | Palmidin A | | |
ESR1 | 6v8t | MOL001458 | Coptisine | | |
PTGS2 | 5f19 | MOL000098 MOL001454 | Quercetin Berberine | | |
Figure 7.
Main pathways were colored using a KEGG mapper. The red color denotes the targets of the herb pair C. Rhizoma-ginger regulation in colon cancer. (A) PI3K-Akt signaling pathway. (B) HIF-1 signaling pathway.
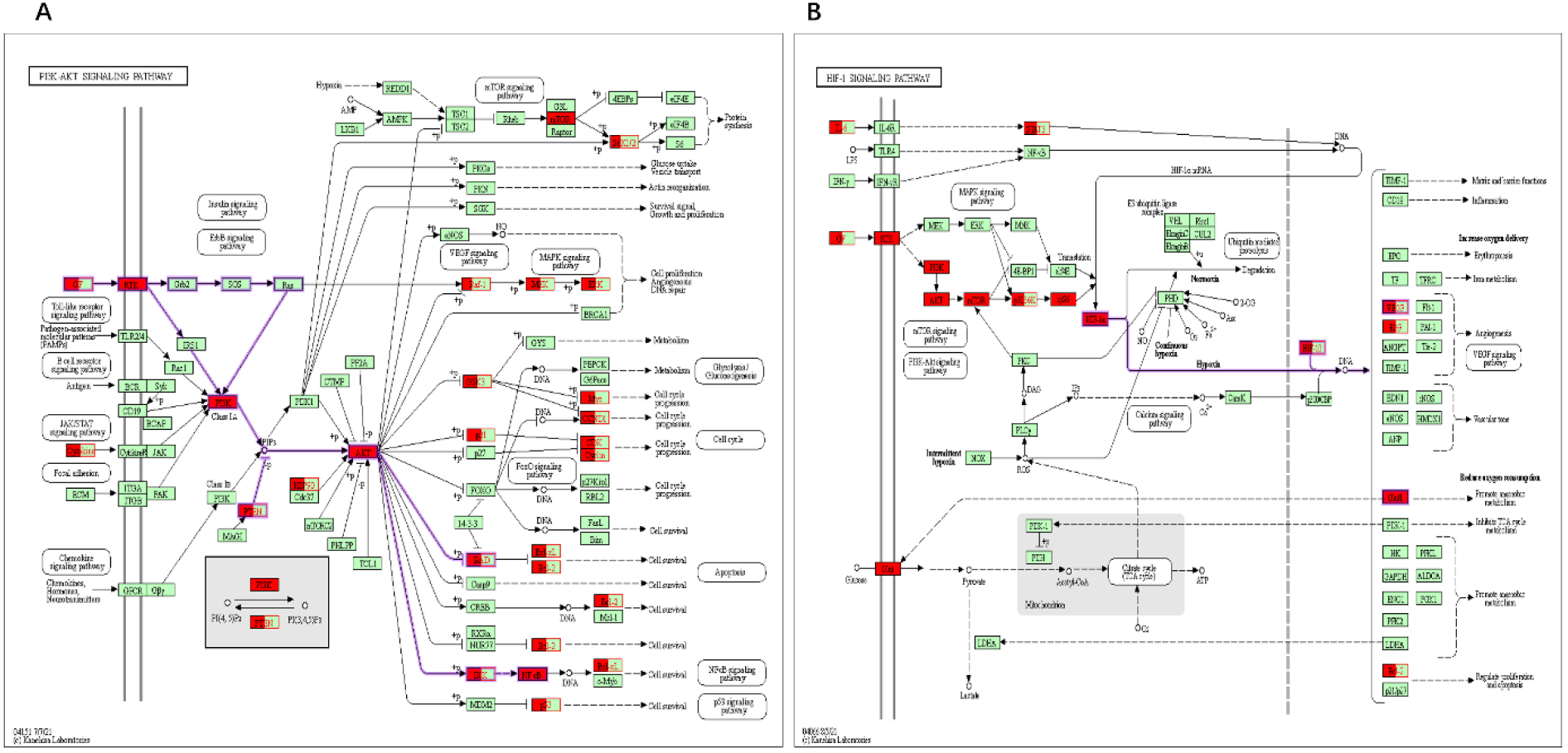
Figure 8.
Results of analysis of the mode of action of active compounds with five protein targets using molecular docking. (A) Action mode of palmidin A (MOL000762) with target BCl2 (PDB:5uuk). (B–F) Action mode of 6-gingerol (MOL002467) with targets HSP90AA1 (PDB:1BYQ) and STAT3 (PDB:6gfa). (C–F) Action mode of berberine (MOL001454) with targets JAK2 (PDB:5aep) and STAT3 (PDB:6gfa). (D and E) Action mode of coptisine (MOL001454) with targets JAK2 (PDB:5aep) and ESR1 (PDB:6v8t).
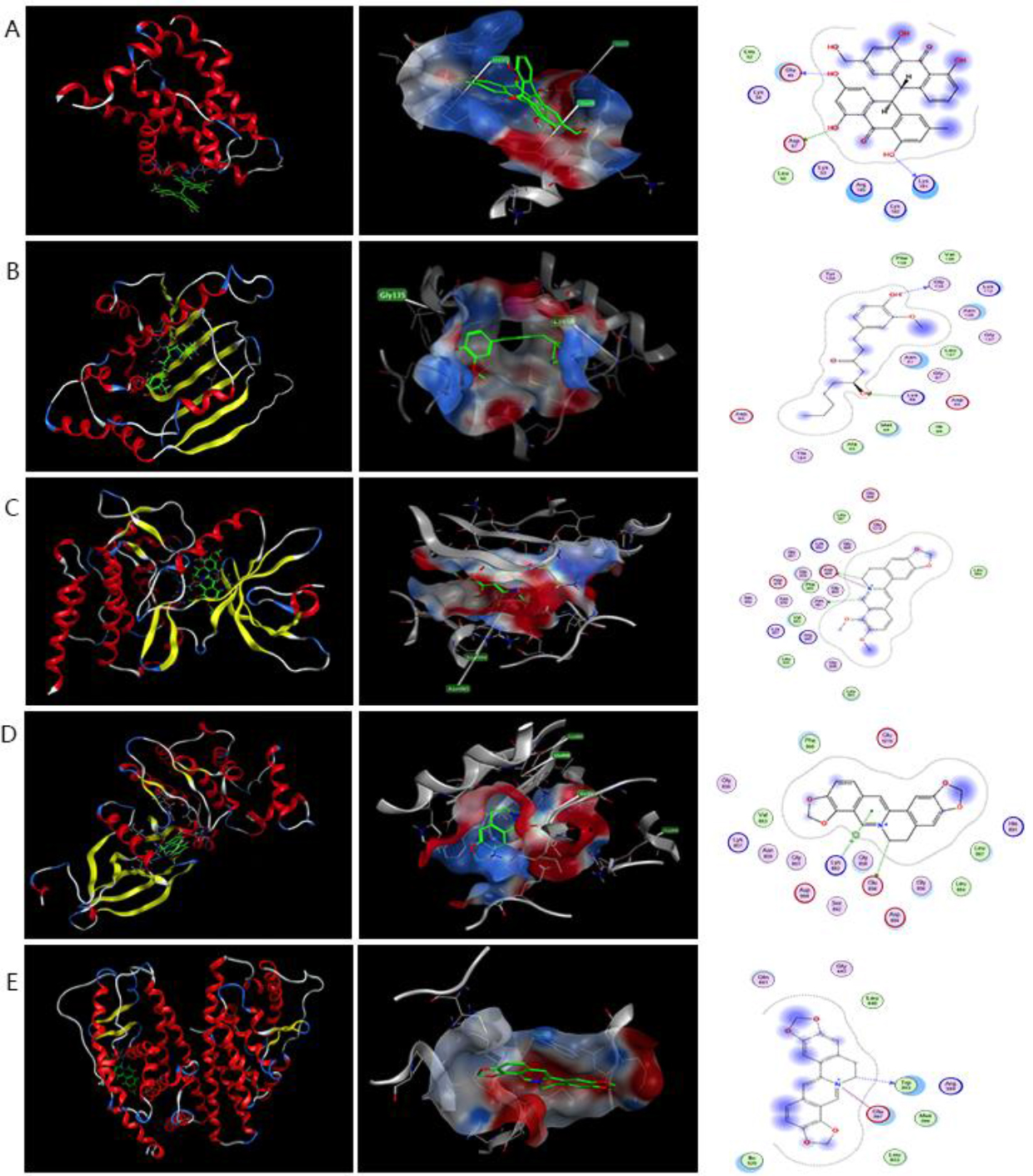
Figure 8.
(G and H) Action mode of quercetin (MOL001454) with targets STAT3 (PDB:6gfa) and SRC (PDB:2bdf). (I and J) Action mode with targets TP53 (PDB:5O1F) and Akt1 (PDB:2uzs).
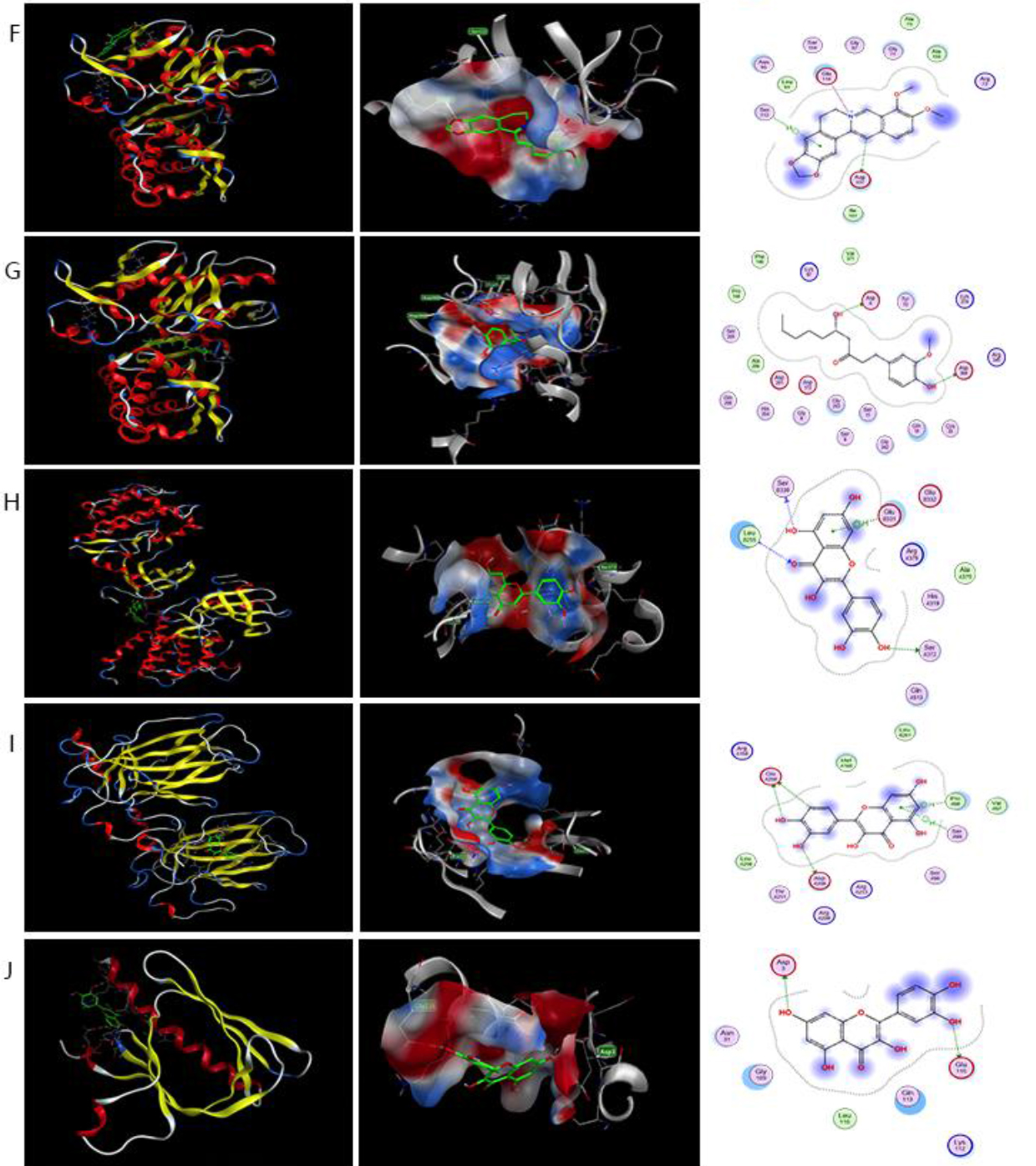
A total of 20 potential active ingredients from the herb pair Coptis Rhizoma-ginger were identified from the TCMSP database, with 14 originating from Coptis Rhizoma and the remaining from ginger. Quercetin, 6-gingerol, berberine, coptisine, palmidin A, and beta-sitosterol play an anti-colon cancer role. Quercetin, the active ingredient of Coptis Rhizoma, also a natural flavonoid found in many vegetables, has been shown to induce the apoptosis of human colon cancer cells, reduce proliferation, and inhibit mitotic processes [24, 25]. Quercetin treatment enhances cytotoxicity and apoptosis in colon cancer stem cells and HT-29 cells, blocking tumor cells in the G2/M phase [26]. The ginger extract inhibits cell proliferation in HCT 116 and HT 29 colon cancer cells by inducing apoptosis in the G0/G1 phase [27]. Further, 6-gingerol, as a naturally occurring plant phenol, is the most abundant ingredient in fresh (dried) ginger, which has displayed a dose- and time-dependent increase in the level of p27Kip1 in LoVo colon cancer cells, exerting a G2/M-arresting effect [28]. Berberine is an alkaloid isolated and extracted from Coptis Rhizoma, barberry, and other plants. It can downregulate COX-2 and PGE2 expression levels and JAK2/STAT3 signaling in SW620 and LoVo colorectal cancer cells [29]. Beta-sitosterol in ginger, a major dietary phytosterol, has been shown to prevent colon carcinogenesis in rodent models by inhibiting dysregulated cell cycle progression and inducing apoptosis; it also exerted antiproliferative effects on HT116 and HT-29 human colon cancer cells [30, 31, 32]. Coptisine is a natural alkaloid from Coptis Rhizoma shown to exhibit significant cytotoxic activity against HCT-116 cells during colon cancer tumorigenesis by inducing G1-phase cell cycle arrest and increasing apoptosis with preferential inhibition of survival pathway and activation of the caspase protease family in HCT-116 cells [33, 34]. Some studies have explored the anticancer effect of berberine on HT-29, SW-480, and HCT-116 human colorectal cancer cell lines [35]. Beta-sitosterol is a plant sterol that can interfere with different cell signaling pathways, including cell cycle, apoptosis, proliferation, survival, invasion, angiogenesis, and inflammation. It can also inhibit the proliferation of colon cancer cells by downregulating the expression of LEF-1 [36]. However, its poor aqueous solubility and bioavailability, coupled with low targeting efficacy, limit its therapeutic efficacy and clinical application [37]. Therefore, it is hypothesized that the treatment efficacy of the herb pair Coptis Rhizoma-ginger is mediated by quercetin, 6-gingerol, coptisine, and berberine.
The active compounds of the herb pair Coptis Rhizoma-ginger can inhibit the expression of pro-inflammatory cytokines and enzymes, such as COX-2 and nuclear factor-kappa B. They can also scavenge reactive oxygen species and reduce oxidative stress-induced damage to DNA, proteins, and lipids, thus inhibiting tumor initiation and progression. These compounds play a crucial role in the inflammatory process associated with the potential mechanism of treating colon cancer. Xu et al. [38] reported novel berberine analogues that could target RXR
Based on “compound-target-pathway-disease” network (Fig. 6) analysis, it was predicted that SRC, HSP90AA1, Akt1, TP53, STAT3, and JAK2 were the key targets for treating colon cancer, which were directly or indirectly related to compounds such as quercetin, berberine, palmidin A, 6-gingerol, and coptisine. Quercetin compounds showed strong associations with 33 key target genes whereas 6-gingerol compounds showed strong associations with 16 key target genes. This suggested that both quercetin and 6-gingerol compounds could be potential active ingredients for colon cancer prevention. However, the E (kcal/mol) score of 6-gingerol was higher than that of quercetin, which might be due to poorer DL properties (DL
Through GO enrichment analysis, we learned that the herb pair was involved in the negative regulation of the apoptotic process, negative regulation of the apoptotic process, and other BPs. Also, we found that the regulation of miRNA-mediated gene silencing, phosphatidylinositol 3-kinase (PI3K) signaling, and telomerase activity played essential roles in regulating cancer pathways. Some studies have shown that the excessive activation of the PI3K/Akt/mTOR pathway played a significant role in tumorigenesis and progression. As an essential kinase in this pathway, PI3K regulated the expression of downstream signaling proteins and mediated the activation of the PI3K/Akt/mTOR pathway. In particular, PI3K-related factors were overexpressed in many cancers, including breast cancer, non-small-cell lung cancer, and colorectal cancer [39, 40]. In addition, telomerase activation is a crucial stage in tumor development and is widely recognized as both a tumor marker and a target for antitumor therapy. In recent years, the inhibition of telomerase activity by TCM has become one of the hotspots in tumor therapy. Some BPs, such as negative regulation of the apoptotic process, have been shown to regulate signaling pathways in human colon cancer [41].
The enrichment analysis of KEGG pathways revealed the therapeutic effects of the herb pair on colon cancer through pathways such as the cancer signaling pathway, PI3K-Akt signaling, lipid and atherosclerosis, proteoglycans in the cancer signaling pathway, and so forth. The PI3K/Akt pathway is a key signaling pathway focusing on serine/threonine kinase Akt and involving genes such as Akt1, JAK2, HSP90AA1, TP53, BCl2, and CASAS. The PI3K/Akt pathway plays a crucial role in various downstream pathways, including metabolism, apoptosis, cell growth, survival, and angiogenesis, and is also one of the most frequently activated pathways in cancer [42].
PI3K signaling pathway plays an oncogenic role in the development and progression of colon cancer; the phosphorylation of Akt has been associated with the inhibition of cell proliferation and apoptosis in human colon cancer [43, 44]. The inhibition of the PI3K/Akt pathway has been used in cancer therapy. Another signaling pathway, that is, hypoxia-inducible factor 1 (HIF-1) signaling, has been effectively used as a promising target for therapeutics because it has a significant impact on tumor proliferation, angiogenesis, and metastasis [45, 46]. Moreover, in previous studies, some genes were identified to be associated with both apoptosis and hypoxia in cancer, along with the “PI3K-AKT signaling pathway” and the “HIF-1 signaling pathway” [47]. Recent studies suggest that a combination of HIF-1 and Akt inhibitors may be a reasonable treatment approach for patients with colon cancer. Therefore, the PI3K-Akt in combination with HIF-1 signaling can be considered as an important signaling pathway in treating colon cancer.
As seen in the drug-compound-disease-target-pathway (Fig. 4), one target gene can be regulated by not only one compound but also multiple compounds and multiple pathways. These genes include but are not limited to Akt1, TP53, STAT3, SRC, HSP90AA1, JAK2, CASP3, PTGS2, BCl2, and ESR1. TP53 is one of the most significant mutated genes in human colon cancer associated with 15 pathways, including the PI3K-Akt signaling pathway. Two genes Akt1 and PIK3CA are associated with all important pathways. In addition, the core target genes Akt1, TP53, HSP90AA1, and STAT3 have been shown to be potential targets for treating colon cancer through molecular docking. HSP90AA1 is closely related to tumor cell cycle, apoptosis, invasion, and differentiation, and is a key protein in treating colon cancer [48, 49]. These findings revealed that the active ingredients of the herb pair were characterized by the modulation of multiple target genes in theory.
5.Conclusions
A total of 20 active compounds, 630 target genes, and 1922 disease-associated targets were identified from the herb pair Coptis Rhizoma-ginger as potentially relevant to the treatment of colon cancer. Forty key genes were generated in the PPI network. The GO biological functions and KEGG pathway enrichment found that PI3K-Akt and HIF-1 signaling pathways played an essential role in colon cancer prevention. Also, these pathways were a potential target for therapeutic intervention and the development of novel anti-colon cancer drugs. Moreover, BCl2, HSP90AA1, STAT3, JAK2, ESR1, SRC, TP53, and Akt1 are hypothesized to be the most important key targets. Meanwhile, palmidin A, 6-gingerol, berberine, coptisine, and quercetin were obtained by molecular docking and used for structural modification and development of new drugs to treat colon cancer. Antitumor Chinese medicines are highly suitable for developing new drugs for the clinical treatment of colon cancer due to their multi-target, multi-pathway, and multi-effect characteristics, as well as their low toxicity and side effects.
Acknowledgments
This study was partially supported by the Macau Science and Technology Development Funds (Grant No. 0056/2020/AFJ) from the Macau Special Administrative Region of the People’s Republic of China, and the Key Project from the University of Educational Commission of Guangdong Province of China Funds (Natural, Grant No. 2019GZDXM005).
Conflict of interest
None to report.
References
[1] | Vasaikar S, et al. Proteogenomic analysis of human colon cancer reveals new therapeutic opportunities. Cell. (2019) ; 177: : 1035-1049e1019. |
[2] | Boman B, Huang E. Human colon cancer stem cells: a new paradigm in gastrointestinal oncology. J Clin Oncol. (2008) ; 26: : 2828-38. |
[3] | Luo T, Lu Y, Yan Sk et al. Network Pharmacology in Research of Chinese Medicine Formula: Methodology, Application and Prospective. Chin J Integr Med. (2020) ; 26: : 72-80. doi: 10.1007/s11655-019-3064-0. |
[4] | Fan Y, Ma Z, Zhao L, Wang W, Gao M, Jia X, Ouyang H. Anti-tumor activities and mechanisms of traditional Chinese medicines formulas: a review Biomed. Pharmacother. (2020) ; 132: . Article 110820, doi: 10.1016/j.biopha.2020.110820. |
[5] | Chen YX, Gao QY, Zou TH, et al. Berberine versus placebo for the prevention of recurrence of colorectal adenoma: a multicentre, double-blinded, randomised controlled study. Lancet Gastroenterol Hepatol. (2020) Mar; 5: (3): 267-275. doi: 10.1016/S2468-1253(19)30409-1. Epub 2020 Jan 8. PMID: 31926918. |
[6] | Couder-García BDC, Jacobo-Herrera NJ, Zentella-Dehesa A, Rocha-Zavaleta L, Tavarez-Santamaría Z, Martínez-Vázquez M. The Phytosterol Peniocerol Inhibits Cell Proliferation and Tumor Growth in a Colon Cancer Xenograft Model. Front Oncol. (2019) Dec 3; 9: : 1341. doi: 10.3389/fonc.2019.01341. PMID: 31850224; PMCID: PMC6901603. |
[7] | Govindarajan VS. Ginger: chemistry, technology, and quality evaluation: part 1. Crit Rev Food Sci. (1982) ; 17: : 196. |
[8] | Prasad S, Tyagi AK. Ginger and its constituents: role in prevention and treatment of gastrointestinal cancer. Gastroenterol Res Pract. (2015) ; 2015: : 142979. doi: 10.1155/2015/142979. Epub 2015 Mar 8. PMID: 25838819; PMCID: PMC4369959. |
[9] | Nachvak SM, Soleimani D, Rahimi M, Azizi A, Moradinazar M, Rouhani MH, Halashi B, Abbasi A, Miryan M. Ginger as an anticolorectal cancer spice: A systematic review of in vitro to clinical evidence. Food Science & Nutrition. (2023) ; 11: : 651-660. doi: 10.1002/fsn3.3153. |
[10] | Zhou M, Hong Y, Lin X, et al. Recent pharmaceutical evidence on the compatibility rationality of traditional Chinese medicine. J Ethnopharmacol. (2017) ; 206: : 363-375. |
[11] | Lee J, Park J, Park H, et al. Synergistic effect of Bupleuri Radix and Scutellariae Radix on adipogenesis and AMP-activated protein kinase: a network pharmacological approach. Evid Based Complement Alternat Med. (2018) ; 2018: : 5269731. |
[12] | Li S, Zhang B. Traditional Chinese medicine network pharmacology: theory, methodology and application. Chin J Nat Med. (2013) ; 11: (2): 11020. Epub 2013/06/22. doi: 10.1016/S1875-5364(13)60037-0 PMID: 23787177 |
[13] | Zhang MM, Wang D, Lu F. et al. Identification of the active substances and mechanisms of ginger for the treatment of colon cancer based on network pharmacology and molecular docking. BioData Mining. (2021) ; 14: : 1. doi: 10.1186/s13040-020-00232-9. |
[14] | Bai LL, Chen H, Zhou P, Yu J. Identification of Tumor Necrosis Factor-Alpha (TNF-α) Inhibitor in Rheumatoid Arthritis Using Network Pharmacology and Molecular Docking. Front Pharmacol. (2021) May 21; 12: : 690118. doi: 10.3389/fphar.2021.690118. PMID: 34093213; PMCID: PMC8175775. |
[15] | Huang D, Wen X, Lu C, Zhang B, Fu Z, Huang Y, Niu K, Yang F. Investigating the molecular mechanism of Compound Danshen Dropping Pills for the treatment of epilepsy by utilizing network pharmacology and molecular docking technology. Ann Transl Med. (2022) Feb; 10: (4): 216. doi: 10.21037/atm-22-195. PMID: 35280369; PMCID: PMC8908140. |
[16] | Qin T, Wu L, Hua Q, Song Z, Pan Y, Liu T. Prediction of the Mechanisms of Action of Shenkang in Chronic Kidney Disease: A Network Pharmacology Study and Experimental Validation. J Ethnopharmacol. (2020) ; 246: : 112128. doi: 10.1016/j.jep.2019.112128. |
[17] | Kohl M, Wiese S, Warscheid B. Cytoscape: software for visualization and analysis of biological networks (M)//data mining in proteomics. Methods Mol Biol. (2011) ; 96: : 291303. |
[18] | Jafri MA, Ansari SA, Alqahtani MH. et al. Roles of telomeres and telomerase in cancer, and advances in telomerase-targeted therapies. Genome Med. (2016) ; 8: : 69. doi: 10.1186/s13073-016-0324-x. |
[19] | Robinson NJ, Schiemann WP. Telomerase in Cancer: Function, Regulation, and Clinical Translation. Cancers (Basel). (2022) Feb 5; 14: (3): 808. doi: 10.3390/cancers14030808. PMID: 35159075; PMCID: PMC8834434. |
[20] | Narayanankutty A. PI3K/Akt/mTOR Pathway as a Therapeutic Target for Colorectal Cancer: A Review of Preclinical and Clinical Evidence. Curr Drug Targets. (2019) ; 20: (12): 1217-1226. doi: 10.2174/1389450120666190618123846. PMID: 31215384. |
[21] | Hernandez-Aya LF, Gonzalez-Angulo AM. Targeting the phosphatidylinositol 3-kinase signaling pathway in breast cancer. Oncologist. (2011) ; 16: (4): 404-14. doi: 10.1634/theoncologist.2010-0402. Epub 2011 Mar 15. PMID: 21406469; PMCID: PMC3228119. |
[22] | Wicks EE. Semenza GL. Hypoxia-inducible factors: cancer progression and clinicaltranslation. J Clin Invest. (2022) ; 132: (11): e159839. doi: 10.1172/JCI159839. |
[23] | Durko L, Malecka-Panas E. Lifestyle Modifications and Colorectal Cancer. Curr Colorectal Cancer Rep. (2014) ; 10: (1): 45-54. doi: 10.1007/s11888-013-0203-4. PMID: 24659930; PMCID: PMC3950624. |
[24] | Zhang XA, Zhang S, Yin Q, Zhang J. Quercetin induces human colon cancer cells apoptosis by inhibiting the nuclear factor-kappa B Pathway. Pharmacogn Mag. (2015) Apr-Jun; 11: (42): 404-9. doi: 10.4103/0973-1296.153096. PMID: 25829782; PMCID: PMC4378141. |
[25] | Reyes-Farias M, Carrasco-Pozo C. The Anti-Cancer Effect of Quercetin: Molecular Implications in Cancer Metabolism. Int J Mol Sci. (2019) ; 20: : 3177. doi: 10.3390/ijms20133177. |
[26] | Zhao L, Zhao H, Zhao Y, Sui M, Liu J, Li P, Liu N, Zhang K. Role of Ginseng, Quercetin, and Tea in Enhancing Chemotherapeutic Efficacy of Colorectal Cancer. Front Med (Lausanne). (2022) Jun 20; 9: : 939424. doi: 10.3389/fmed.2022.939424. PMID: 35795631; PMCID: PMC9252166. |
[27] | Abdullah S, Abidin SAZ, Murad NA, Makpol S, Ngah WZW, Yusof YAM. Ginger extract (Zingiber officinale) triggers apoptosis and G0/G1 cells arrest in HCT 116 and HT 29 colon cancer cell lines. Afr J Biochem Res (2010) ; 4: : 134-142. |
[28] | Lin CB, Lin CC, Tsay GJ. 6-Gingerol Inhibits Growth of Colon Cancer Cell LoVo via Induction of G2/M Arrest. Evid Based Complement Alternat Med. (2012) ; 2012: : 326096. doi: 10.1155/2012/326096. Epub 2012 Jun 7. PMID: 22719783; PMCID: PMC3375166. |
[29] | Liu X, Ji Q, Ye N, Sui H, Zhou L, Zhu H, Fan Z, Cai J, Li Q. Berberine Inhibits Invasion and Metastasis of Colorectal Cancer Cells via COX-2/PGE2 Mediated JAK2/STAT3 Signaling Pathway. PLoS One. (2015) May 8; 10: (5): e0123478. doi: 10.1371/journal.pone.0123478. PMID: 25954974; PMCID: PMC4425560. |
[30] | Choi YH, Kong KR, Kim YA, Jung KO, Kil JH, Rhee SH, Park KY. Induction of Bax and activation of caspases during beta-sitosterol-mediated apoptosis in human colon cancer cells. Int J Oncol. (2003) Dec; 23: (6): 1657-62. PMID: 14612938. |
[31] | Khan Z, Nath N, Rauf A, Emran TB, Mitra S, Islam F, Chandran D, Barua J, Khandaker MU, Idris AM, Wilairatana P, Thiruvengadam M. Multifunctional roles and pharmacological potential of β-sitosterol: Emerging evidence toward clinical applications. Chemico-Biological Interactions. (2022) ; 365: : 110117. ISSN 0009-2797, doi: 10.1016/j.cbi.2022.110117. |
[32] | Huang J, Xu M, Fang Y, Lu M, Pan Z, Huang W, |
[33] | Huang T, Xiao Y, Yi L. et al. Coptisine from Rhizoma Coptidis Suppresses HCT-116 Cells-related Tumor Growth in vitro and in vivo. Sci Rep. (2017) ; 7: : 38524. doi: 10.1038/srep38524. |
[34] | Inoue N, Terabayashi T, Takiguchi-Kawashima Y, Fujinami D, Matsuoka S, Kawano M, Tanaka K, Tsumura H, Ishizaki T, Narahara H, Kohda D, Nishida Y, Hanada K. The benzylisoquinoline alkaloids, berberine and coptisine, act against camptothecin-resistant topoisomerase I mutants. Sci Rep. (2021) Apr 8; 11: (1): 7718. doi: 10.1038/s41598-021-87344-2. PMID: 33833336; PMCID: PMC8032691. |
[35] | Tarawneh N, Hamadneh L, Abu-Irmaileh B, Shraideh Z, Bustanji Y, Abdalla S. Berberine Inhibited Growth and Migration of Human Colon Cancer Cell Lines by Increasing Phosphatase and Tensin and Inhibiting Aquaporins 1, 3 and 5 Expressions. Molecules. (2023) Apr 29; 28: (9): 3823. doi: 10.3390/molecules28093823. PMID: 37175233; PMCID: PMC10180100. |
[36] | Gu S, Liu F, Xie X, Ding M, Wang Z, Xing X, Xiao T, Sun X. β-Sitosterol blocks the LEF-1-mediated Wnt/β-catenin pathway to inhibit proliferation of human colon cancer cells. Cellular Signalling. (2023) . doi: 10.1016/j.cellsig.2022.110585. |
[37] | Wang H, Wang Z, Zhang Z, Liu J, Hong L. β-Sitosterol as a Promising Anticancer Agent for Chemoprevention and Chemotherapy: Mechanisms of Action and Future Prospects, Advances in Nutrition. (2023) . doi: 10.1016/j.advnut.2023.05.013. |
[38] | Xu B, Jiang X, Xiong J, Lan J, Tian Y, Zhong L, Wang X, Xu N, Cao H, Zhang W, Zhang H, Hong X, Zhan YY, Zhang Y, Hu T. Structure-Activity Relationship Study Enables the Discovery of a Novel Berberine Analogue as the RXRα Activator to Inhibit Colon Cancer. J Med Chem. (2020) Jun 11; 63: (11): 5841-5855. doi: 10.1021/acs.jmedchem.0c00088. Epub 2020 May 28. PMID: 32391701. |
[39] | Xu Z, Han X, Ou D, et al. Targeting PI3K/AKT/mTOR-Mediated Autophagy for Tumor Therapy. Applied Microbiology and Biotechnology. (2020) ; 104: : 575-587. doi: 10.1007/s00253-019-10257-8. |
[40] | Thorpe LM, Yuzugullu H, Zhao JJ. PI3K in Cancer: Diver-gent Roles of Isoforms, Modes of Activation and Therapeutic Targeting. Nature Reviews Cancer. (2015) ; 15: : 7-24. doi: 10.1038/nrc3860. |
[41] | Huang R, Zhou PK. HIF-1 signaling: a key orchestrator of cancer radioresistance. Radiat Med Prot. (2020) ; 1: : 7-14. doi: 10.1016/j.radmp.2020.01.006. |
[42] | Kumar A, Rajendran V, Sethumadhavan R, Purohit R. AKT kinase pathway: a leading target in cancer research. Scientific World Journal. (2013) Nov 13; 2013: : 756134. doi: 10.1155/2013/756134. PMID: 24327805; PMCID: PMC3845396. |
[43] | Danielsen SA, Eide PW, Nesbakken A, Guren T, Leithe E, Lothe RA. Portrait of the PI3K/AKT pathway in colorectal cancer. Biochim Biophys Acta. (2015) Jan; 1855: (1): 104-21. doi: 10.1016/j.bbcan.2014.09.008. Epub 2014 Nov 22. PMID: 25450577. |
[44] | Koveitypour Z, Panahi F, Vakilian M. et al. Signaling pathways involved in colorectal cancer progression. Cell Biosci. (2019) ; 9: : 97. doi: 10.1186/s13578-019-0361-4. |
[45] | Jin X, Dai L, Ma Y. et al. Implications of HIF-1α in the tumorigenesis and progression of pancreatic cancer. Cancer Cell Int. (2020) ; 20: : 273. doi: 10.1186/s12935-020-01370-0. |
[46] | Xiao B, Wang G, Huo H, Li W. Identification of HIF-1α/VEGFA signaling pathway and transcription factors in Kashin-Beck disease by integrated bioinformatics analysis. Exp Ther Med. (2021) Oct; 22: (4): 1115. doi: 10.3892/etm.2021.10549. Epub 2021 Aug 4. PMID: 34504569; PMCID: PMC8383754. |
[47] | Kawaguchi N, Tashiro K, Taniguchi K, Kawai M, Tanaka KJ, Okuda M, Hayashi K, Chiyama U. Nogo-B (Reticulon-4B) functions as a negative regulator of the apoptotic pathway through the interaction with c-FLIP in colorectal cancer cells. Biochim Biophys Acta Mol Basis Dis. (2018) ; 1864: . 2600-2609. doi: 10.1016/j.bbadis.2018.04.018. |
[48] | Zhang M, Peng Y, Yang Z. et al. DAB2IP down-regulates HSP90AA1 to inhibit the malignant biological behaviors of colorectal cancer. BMC Cancer. (2022) ; 22: : 561. doi: 10.1186/s12885-022-09596-z. |
[49] | Szczuka I, Wierzbicki J, Serek P, Szczȩśniak-Siȩga BM, Krzystek-Korpacka M. Heat Shock Proteins HSPA1 and HSP90AA1 Are Upregulated in Colorectal Polyps and Can Be Targeted in Cancer Cells by Anti-Inflammatory Oxicams with Arylpiperazine Pharmacophore and Benzoyl Moiety Substitutions at Thiazine Ring. Biomolecules. (2021) Oct 27; 11: (11): 1588. doi: 10.3390/biom11111588. PMID: 34827586; PMCID: PMC8615942. |