Acupuncture improves learning and memory ability of posttraumatic stress disorder model rats through epigenetic regulation of microglial phosphatidylinositol 3-kinase pathway
Abstract
BACKGROUND:
Microglia express phosphatidylinositol 3-kinase (PI3K) has been implicated in the induction and maintenance of long-term potentiation (LTP) and in hippocampal synaptic plasticity. However, there are few studies on the interference of PI3K signal pathway in microglia.
OBJECTIVE:
The study goal is to gain a better understanding of the mechanism by which EA affects synapses provides insights into how electroacupuncture (EA) modulates synaptic plasticity in learning and memory.
METHODS:
Rat models of posttraumatic stress disorder (PTSD) were used to explore the effects of EA on microglial PI3K pathway, brain-derived neurotrophic factor (BDNF) and LTP, and the target and mechanism underlying the effects of EA on PI3K from the perspective of protein ubiquitination.
RESULTS:
EA induced microglial BDNF expression by activating the PI3K-AKT pathway, thereby facilitating LTP and synaptic plasticity. EA inhibited lincRNA 02023 to rescue the binding of WWP2 to PTEN, thereby promoting PTEN ubiquitination and degradation.
CONCLUSION:
The mechanism of EA improving the learning and memory ability of PTSD rats may be that it can promote the competitive combination of WWP2 and PTEN by inhibiting Linc RNA02023, and then lead to microglial PI3K and its pathway activation, BDNF up-regulation, and finally induce LTP and repair damaged synaptic plasticity.
1.Introduction
Glial cells, also referred to as gliocytes, are abundant in the central nervous system (CNS) and peripheral nervous system (PNS) alongside neurons. In mammals, the ratio of glial cells to neurons is approximately 10:1. Glial cells in the CNS mainly include astrocytes and oligodendrocytes (collectively known as macroglia) as well as microglia. These cells provide support and protection for the nervous system, regulating synapse formation and pruning during development; although their synapse regulation in adults is less deeply studied, glial cells may exert these effects throughout the lifespan.
Microglia, accounting for 5–12% of total glial cells [1], have long been considered immune sentinels that mediate inflammatory responses by secreting cytokines and chemokines. In addition, they are involved in many central activities, such as synaptic organization, transmission, and plasticity as well as the regulation of neuronal excitability (and thus memory formation) [2, 3]. Some reports have indicated that microglia are closely related to synaptic transmission and even in synaptic reconstruction [4]. Moreover, Microglia produce a wide range of signal molecules including cytokines, neurotransmitters, and extracellular matrix proteins, which regulate neuronal activity, synaptic activity and functional plasticity [5, 6]. These findings suggest that microglia play an active role in synaptic plasticity, which is the molecular biological basis of learning and memory. Indeed, the close interaction between microglia and synapses has led to the synaptic detachment hypothesis [7], under which microglia are theorized to selectively eliminate dysfunctional synapses. This microglia-mediated synaptic removal is generally believed to originate from activity-dependent refinement in the process of neural development but may occur in aging or neurodegenerative diseases [8]. Impaired microglia-mediated synaptic elimination leads to an imbalance between excitatory and inhibitory transmission, leading to various disturbances in brain functions: memory, cognition, sleep, movement, and social behaviours [9]. Microglia have been recorded in the thalamus, cerebral cortex and hippocampus and play a crucial role in synaptic plasticity and behavioural adaptation to diverse environments, most notably in response to chronic stress [10]. Therefore, therapeutic interventions aimed at bolstering microglial resilience to stress could be crucial for promoting anti-stress ability [11]. In addition, impaired microglia-mediated remodelling of neuronal circuits could severely impair learning and memory. For example, deletion or depletion of brain-derived neurotrophic factor (BDNF) in microglia results in cognitive impairment and fear conditioning [12, 13]. In addition, in the process of motor learning, inhibiting the release of BDNF microglia can reduce the formation of cortical dendritic spines in vivo [13].
Phosphatidylinositol-3-kinase (PI3K) contains of a regulatory subunit (p85) and a catalytic subunit (p110); this protein plays an active role in synaptic plasticity and the activating and maintenance of long-term potentiation (LTP) in the hippocampal CA1 region [14]. The downstream effector molecules of PI3K include protein kinase B (AKT), cAMP response element-binding protein (CREB) and BDNF. Phosphorylated AKT is responsible for effects of many genes, including BDNF, by stimulating the phosphorylation of the nuclear factor CREB. Studies have shown that there is a causal relationship between the increase of CREB phosphorylation and the promotion of BDNF expression [15], a key factor in synaptic plasticity. PI3K may regulate the development of BDNF, thereby affecting synaptic transmission as well as synaptic plasticity [16]. PI3K is also present in microglia. A recent study by Sreedharan Sajikumar [17] confirmed that inhibition of PI3K significantly reduced the expression of BDNF and further concluded that PI3K regulates BDNF expression through the PI3K-AKT pathway. This finding prompted us to investigate the effects of electroacupuncture (EA) on PI3K and to verify whether microglial PI3K underlies EA regulation of synaptic plasticity. In addition, we further explored the target and mechanism underlying the effect of EA on PI3K from the perspective of protein ubiquitination. Acupuncture inhibits lincRNA 02023, which is a long intergenic noncoding RNA that competitively binds to the target protein phosphatase and tensin homolog (PTEN) to promote its binding with the ubiquitin ligase WWP2. Binding of WWP2 to PTEN increases the ubiquitination degradation of PTEN, thereby upregulating the expression of downstream targets such as PI3K and AKT.
2.Methods
2.1Subjects and ethics statement
The experimental operation follows the National Institutes of Health Guide for the Care and Use of Laboratory Animals (National Academies Press, Washington, DC, USA). The experiment is permitted by the Biomedical Ethics Committee of Hainan Medical University(HYLL-2022-379). Adult male Sprague Dawley rats (2 months old, 190–220 g,
A total of two experiments were conducted, with various groups of rats. In the first experiment the rats were randomly assigned to four groups (ten rats each): the control, PTSD model induced by single prolonged stimulation (SPS), SPS with EA treatment (SPS
2.2Construction of the rat model of PTSD
The PTSD rat model was constructed by SPS, and the specific operation was based on the procedure of Souza et al. [18], which was suggested by the International Conference on the Progress of Basic and Clinical Research held by the Ministry of Education of Japan in 2005. The model induced psychological and physiological stress to simulate the PTSD-like symptoms of rats. In the current experimental protocol, each rat underwent various stressors including restraint (inside a plastic tube) for 2 h, swimming (in a rectangular sink filled to 30 cm with water maintained at 24
2.3EA treatment
The SPS
2.4Isolation of hippocampal microglia
Pentobarbital sodium and normal saline were combined at a ratio of 3 g:100 mL. This preparation was injected intraperitoneally into rats at 1 mL/kg body weight, and the rats were then perfused with precooled phosphate-buffered saline (PBS) to flush out the blood. The left and right hippocampus were carefully and quickly removed, and then the tissue was immediately cut as much as possible and digested for 10 min. Then, 5 ml of Dulbecco’s modified Eagle medium (DMEM)/F12 complete medium was added to terminate the digestion, and the cells were centrifuged at 1,000 rpm for 5 min to separate them from the digestion solution. Then, 500 times the volume of Alexa Fluor 647 Tmem119 (ab225494, Abcam) was infused into the cells, and they were incubated for 15 min at room temperature in the dark. Next, the samples were centrifuged at 1,000 rpm for 5 min to remove the supernatant, and then all the cells were sorted on a flow cytometer. The Alexa Fluor 647-Tmem119
2.5Western blot analysis
Microglial cell protein from hippocampus was obtained by flow cytometry. Cytoplasmic protein and nuclear protein were extracted using the kit of target protein (Beyotime Biotechnology, China). The protein was quantitatively extracted using the BCA protein kit (Thermo Fisher Scientific, USA). 50
2.6Preparation of hippocampal slices for electrophysiological recording
After the rats were anesthetized to death with pentobarbital sodium, the brain was rapidly transferred to artificial cerebrospinal fluid (ACSF) (2–4
With the aid of microscope, the stimulation electrode and recording electrode were placed in the radiation layer of hippocampal CA1 area and the apical dendritic area of CA1, respectively. The former stimulated the Schaffer collateral fibers, and the latter recorded the field excitatory postsynaptic potentials (fEPSPs). A direct test stimulation current with a duration of 100
2.7The catRAPID method
CatRAPID is a tool to calculate the binding characteristics of protein and RNA. According to the procedure described by Li et al., we chose the module catRAPID omics v2.0 (custom protein vs custom transcript set) and fill in the PTEN sequence, the type of RNA binding (long intergenic noncoding RNA) and the RNA sequence. After entering this information, we analysed the interaction tendency (which reflects the probability of interaction between a protein (or region) and an RNA (or region)) and generated an interaction matrix (displaying the binding region of interacting protein (y axis) and RNA (x axis) in the form of a heat map). The red shade of the heat map indicates the interaction score of a single amino acid and nucleotide pair).
2.8Statistical analyses
The data were statistically analysed using post hoc tests. Statistical analysis was performed using GraphPad Prism 9 software (GraphPad Software, San Diego, CA, USA). The significance level was set to
3.Results
Our previous studies confirmed that PTSD model rats showed significant emotional disturbances, such as anxiety, depression, and fear, as well as obvious declines in learning and memory. The EA treatment described in this paper effectively improved the above symptoms; its underlying mechanism may be related to alterations of the PI3K-AKT signalling pathway and synaptic plasticity in the hippocampus [19, 21]. As discussed above microglia express genes involved in neuronal synaptic plasticity, and PI3K and its associated PI3K-AKT-CREB signalling pathway play an important role in LTP. Therefore, this study aims to study the target role of PI3K in EA’s promotion of LTP.
3.1EA regulation of microglial expression of PI3K, AKT, CREB and BDNF
The expression of PI3K, AKT, CREB and BDNF in microglia was confirmed by Western blotting. The expression of all proteins was largely detected in the cytoplasm of microglia.
Figure 1.
EA activates the PI3K-AKT pathway. (A) Western blotting revealed the upregulation of PI3K, AKT and CREB expression and phosphorylation of AKT and CREB in microglial cells in the SPS
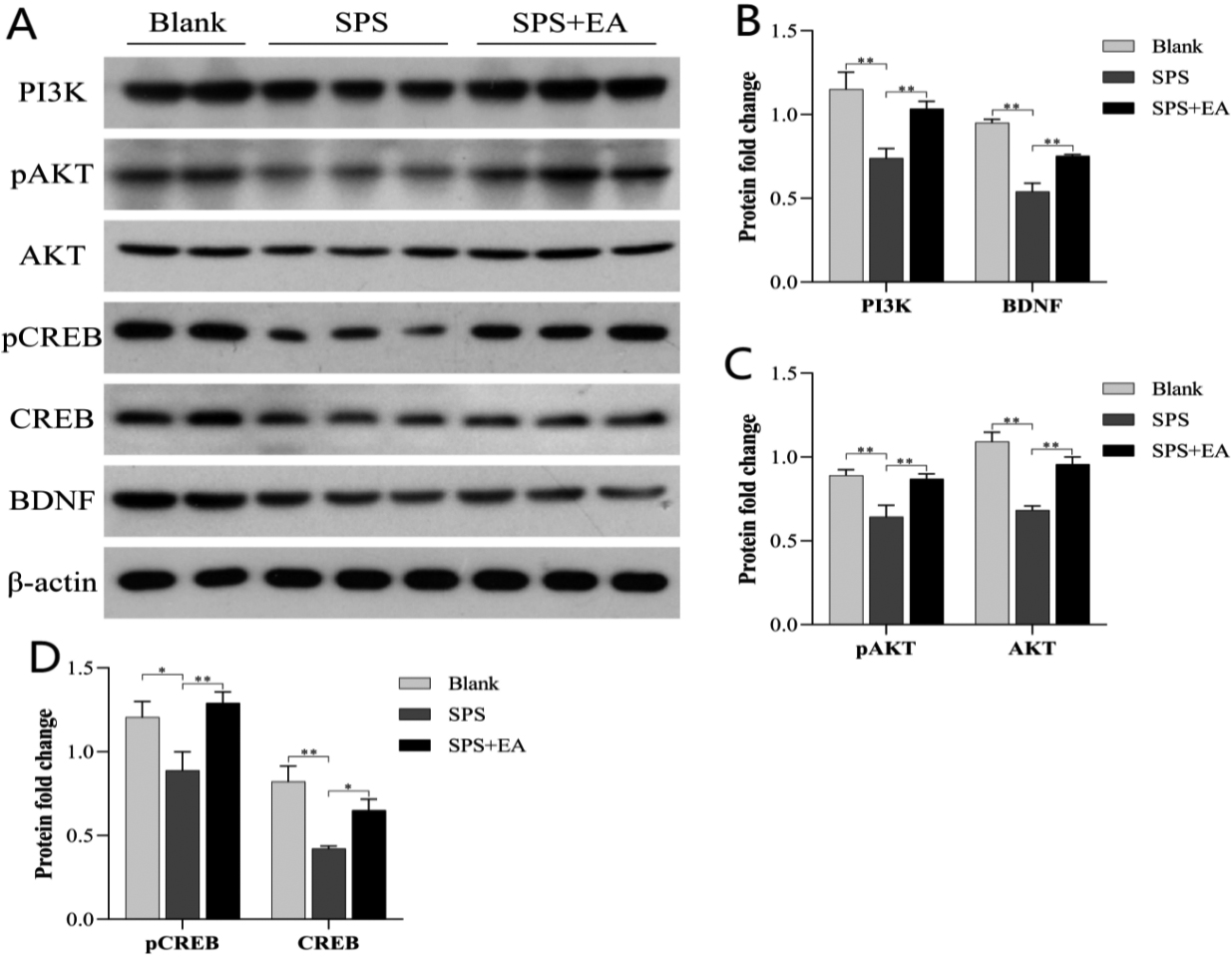
Figure 2.
Treatment with PI3K rescued AKT and BDNF expression. (A) Active PI3K was injected into the culture medium to restore the defect of PI3K level. This addition significantly increased levels of phosphorylated AKT and total AKT and rescued BDNF expression. (B) Western blot data were quantified and normalized to the
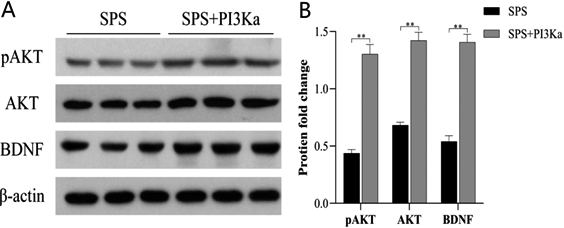
As shown in Fig. 1, compared with the blank control, the expression of PI3K (
3.2PI3K regulation of AKT and BDNF levels in microglia
As shown in Fig. 2, the addition of active PI3K directly led to a significant increase in the levels of phosphorylated AKT (
3.3PI3K activation due to lincRNA 02023 interference of WWP2 binding to PTEN
The domain of the PTEN protein (amino acids 101–179) is considered the main RNA binding region; this region is also the binding site for the E3 ubiquitin ligase WWP2 (amino acids 100–187); that is, the binding sites of PTEN for lincRNA 02023 and WWP2 overlap. We used catRAPID to predict the region of lincRNA 02023 that binds to the PTEN protein as well as the site on PTEN that binds lincRNA 02023, as shown Fig. 3 below. The colour of the heatmap represents the interaction score of an amino acid-nucleotide pair; interactions scores ranged from
Figure 3.
Heatmap of predicted binding sites for lincRNA 02023 and PTEN. The x- and y-axes represent indices of RNA nucleotides and protein sequences respectively. The colour of the heatmap refers to the interaction score (
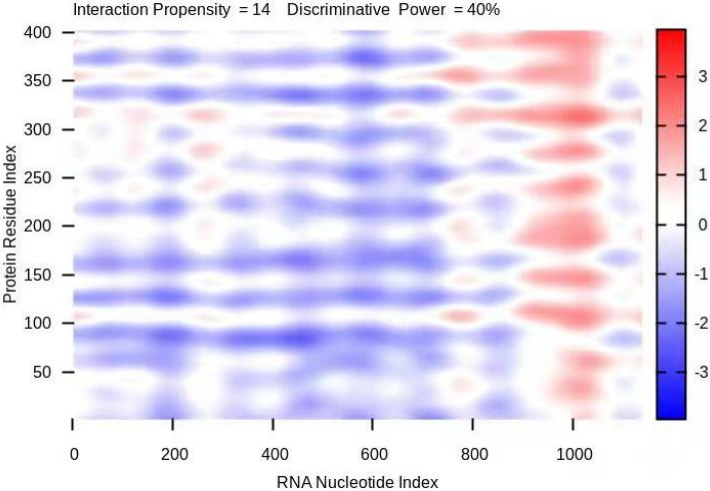
Figure 4.
Activation of PI3K is achieved by inhibiting the expression of lincRNA 02023, which increases the binding affinity of PTEN for WWP2. (A) The relative expression of lincRNA 02023 in the hippocampus of rats in the control, SPS and SPS
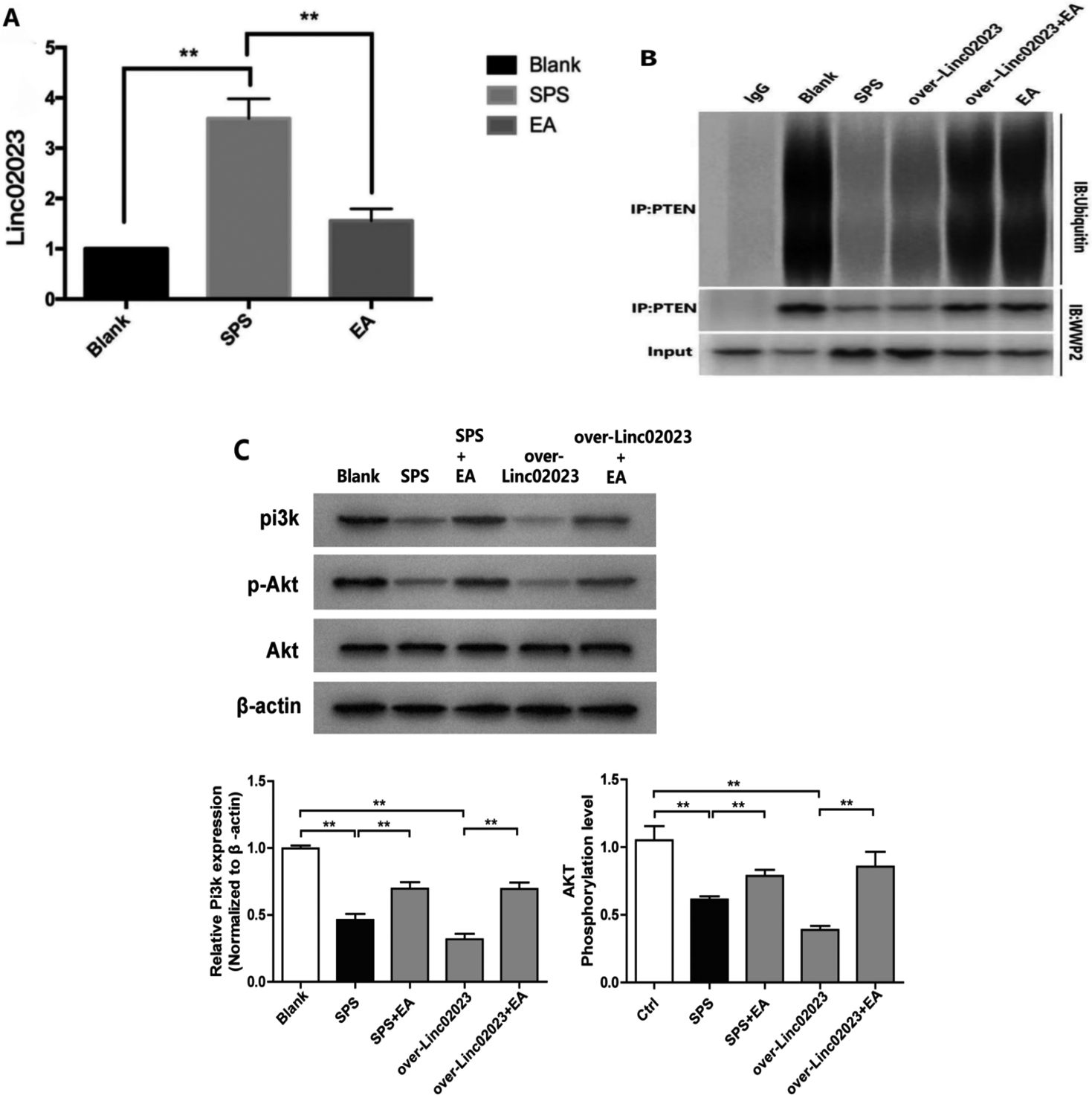
To further confirm that lincRNA 02023 mediates the effects of EA, we compared the expression of lincRNA 02023 in the SPS group with that in SPS
3.4EA restores synaptic plasticity of the hippocampal CA1 region by activating the PI3K-AKT-CREB signalling pathway in microglia
Recent studies have shown that the PI3K-AKT-CREB pathway influences synaptic plasticity by regulating BDNF; therefore, we tested the effect of EA on LTP in PTSD rat models, in which the PI3K-AKT-CREB signalling pathway was compromised. In addition to the control, SPS, and SPS
Figure 5.
Positions of electrodes during recordings. The red arrow points to the location of the stimulation electrode in the hippocampal section, and the blue arrow points to the location of the recording electrode.
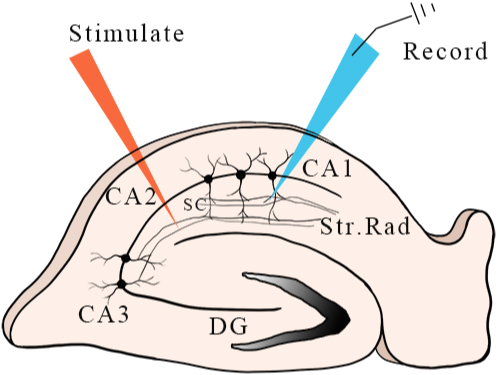
Figure 6.
The effect of EA on LTP. We set the mean value of the basal fEPSP before high frequency stimulation (HFS) as the baseline value (100%) and observed the fEPSP at each time point before and after HFS as well as changes in hippocampal LTP (and the effect of EA on LTP). These values in the control, SPS, and SPS
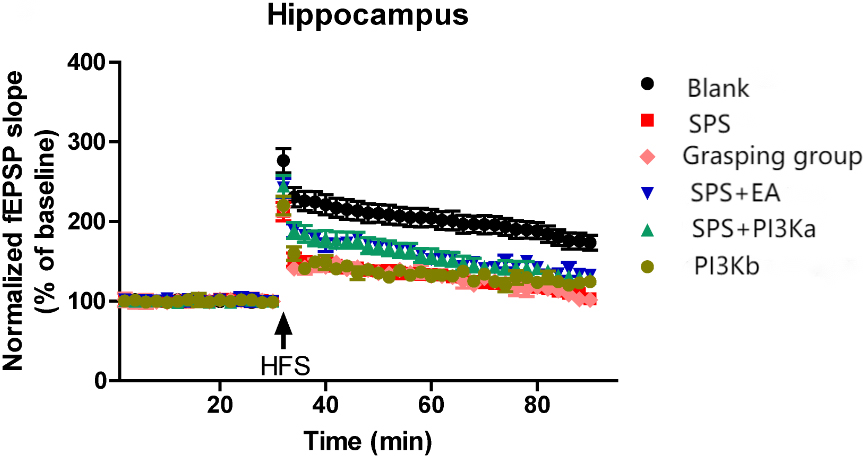
In the present study, we found that the expression of lincRNA 02023 was abnormally upregulated, weakening the ability of WWP2 to bind to PTEN. Further investigation indicated that EA inhibited lincRNA 02023 and thus rescued the binding of WWP2 to PTEN, promoting PTEN ubiquitination and degradation to activate the PI3K-AKT pathway, which is a key factor in regulating LTP and restoring synaptic plasticity.
4.Discussion
Existing studies have shown that the expression of PI3K in microglia is regulated by epigenetics. PI3K may play an important targeted role in the maintenance of LTP through post-translational modification. We verified the effect of EA treatment on PI3K levels and its downstream effectors as well as on LTP.
One of the core PTSD symptom is the persistence of memories of the traumatic event that triggered PTSD (i.e., traumatic fear memory) [22]. Recent studies have confirmed that acupuncture can suppress or eliminate fear memory [23, 24] as well as improve sleep architecture, relieve depression, and alleviate spatial learning and memory deficits [25]; moreover, acupuncture has relatively long-term effects and few side effects. Therefore, exploring the neurobiological mechanism underlying the effects of acupuncture may enhance its beneficial effects. Animal models of PTSD have a guiding role in identifying the neurobiological mechanisms by which exposure to traumatic stress leads to specific PTSD symptoms [26]. The PI3K signaling pathway is sensitive to traumatic stress in various animal models of PTSD; inhibiting PI3K-AKT signalling has been shown to enhance persistent fear memory [27]. Synaptic plasticity is the neurobiological basis of learning and memory, and the PI3K-AKT pathway regulates BDNF to participate in the induction and maintenance of LTP in hippocampal CA1 region, thus contributing to synaptic plasticity. However, the level of PI3K in microglia is mainly related to its role in microglia-mediated inflammation [14]. This study explored the mechanism of EA-induced extinction of fear memory from the perspective of the relationship between the PI3K signalling pathway in microglia and its effects on synapses. The PI3K-AKT signalling pathway was inhibited in hippocampal glial cells of PTSD model rats, and PI3K played a key mediating role in restoring this pathway; thus, activation of PI3K increased the expression of downstream effectors (AKT, CREB and BDNF). Our research suggests that electroacupuncture promotes the expression of downstream pAKT, pCREB and other proteins in microglia by activating the level of PI3K in microglia, thus activating BDNF to increase synaptic transmission and plasticity. Microglia promote synaptic formation through BDNF signal transduction to play the role of learning and memory [13]. In the present study, BDNF expression was not completely eliminated as BDNF expression is also regulated by other pathways. However, we clearly showed that microglial PI3K levels affect BDNF expression and that EA treatment significantly alters microglial PI3K expression. To further explore whether microglial PI3K levels are directly involved in modulating neuronal LTP, field recordings were conducted in hippocampal brain slices. The results showed that LTP in hippocampal slices (inhibited by SPS) was rescued by EA treatment in a similar manner to the effect of PI3K addition, suggesting that microglial BDNF levels by inhibiting the PI3K-AKT pathway, contributes to neuronal LTP and synaptic plasticity. However, the level of PI3K/BDNF may also be mediated by neurons and other microglial pathways to reduce the inhibition of this pathway in brain slices.
Protein ubiquitination is an important posttranslational modification that regulates many biological processes including signalling pathways. WWP2 ubiquitinates and regulates various biological processes by degrading different substrate proteins as well as activating or inhibiting the downstream signalling pathways of different substrate proteins. For example, WWP2 regulates PTEN degradation, affecting the PI3K-AKT signalling pathway [28]. PTEN is an important substrate of WWP2, a major ubiquitin ligase that regulates PTEN protein levels. Studies have found that PTEN is a potent negative regulator of the PI3K-AKT pathway; that is, WWP2 promotes the ubiquitination and degradation of PTEN, thereby exciting the PI3K-AKT signalling pathway [29]. In recent years, studies have reported that PTEN levels can be regulated by acupuncture, such as at “Bai hui” and “Tai chong” acupuncture [30] as well as EA [31]; acupuncture increases the expression of the downstream targets PI3K, AKT and mTOR by suppressing the expression of PTEN, thus activating the PI3K-AKT signalling pathway. PTEN-mediated dysregulation of the PI3K-AKT signalling pathway is an important mechanism underlying the decline in learning and memory.
Noncoding RNAs are critical for the regulation of gene and protein expression and are implicated in neurodevelopmental disorders. The largest subclass of long noncoding RNAs (lncRNAs) are lincRNAs. A study found 43 differentially expressed lincRNAs and 190 differentially expressed mRNAs associated with fear extinction. Microarray analysis of rats exposed to stress, fear and anxiety showed that LincRNA was differentially expressed in brain regions represented by hippocampus [32], and subsequent enrichment analysis [33] indicated that lincRNAs were involved with many biological functions including neuroactive ligand receptors, interactions, calcium signalling, and the PI3K-AKT pathway. However, the role of lincRNAs in the functional regulation and posttranslational modification of proteins involved in signal transduction pathway remains unclear. Using catRAPID, we predicted that the binding region of the PTEN protein for lincRNA 02023 highly overlaps with that of WWP2; that is, lincRNA 02023 competes with WWP2 for binding to PTEN, influencing the ubiquitination of PTEN protein (Fig. 3). A recent study showed that lincRNA 02023 directly binds to a special site on the PTEN protein, inhibits WWP2-mediated ubiquitination and degradation of the PTEN protein, and inhibits phosphorylation of the AKT protein, thereby inhibiting downstream signalling pathways [28].
5.Conclusion
In this study, we found that the expression of lincRNA 02023 was abnormally upregulated in the hippocampus of PTSD model rats and that the binding affinity of PTEN for WWP2 was weakened. Further investigation indicated that EA inhibited lincRNA 02023 expression, rescuing the binding of WWP2 to PTEN, and thereby promoting the ubiquitination and degradation of PTEN, activating the PI3K-AKT pathway (Fig. 4). Our future studies may provide insight into the mechanisms underlying interactions between synaptic tags and plasticity-related products (PRPs) that stabilize cellular associative plasticity.
Funding
This work was supported by the National Natural Science Foundation of China (Nos 82260974, 81873384, 81574086) and the Hainan Provincial Natural Science Foundation (Nos 819QN223, 822RC688).
Data availability statement
The data that support the findings of this study are available from the corresponding author (Mi Li) upon reasonable request.
Author contributions
Mi Li, Lijie Yang, Kai Li and Yiqiang Xie contributed to the conception of the study; Mi Li and Xian Wang performed the experiment; Yiqiang Xie and Kai Li contributed to the oversight and leadership of the research planning and execution; Mi Li, Yong Jiang and Kai Li contributed significantly to the analysis and manuscript preparation; Mi Li and Xian Wang performed the data analyses and wrote the manuscript; Kai Li, Yiqiang Xie and Yong Jiang helped perform the analysis and provided constructive discussion.
Ethics statement
All experiments were carried out in accordance with the National Institutes of Health Guide for the Care and Use of Laboratory Animals (National Academies Press, Washington, DC, USA). The study protocol was approved by the Biomedical Ethics Committee of Hainan Medical University (HYLL-2022-379).
Acknowledgments
The authors sincerely thank all collaborators, in particular Professor Hong Zhang, who provided key preliminary research results for this paper.
Conflict of interest
The authors have no conflicts of interest to report.
References
[1] | Norden DM, Godbout JP. Review: Microglia of the aged brain: primed to be activated and resistant to regulation. Neuropathol Appl Neurobiol. (2013) ; 39: (1): 19-34. |
[2] | Reemst K, Noctor SC, Lucassen PJ, et al. The indispensable roles of microglia and astrocytes during brain development. Front Hum Neurosci. (2016) ; 10: : 566. |
[3] | Hertz L, Chen Y. Editorial: All 3 types of glial cells are important for memory formation. Front Integr Neurosci. (2016) ; 10: : 31. |
[4] | Wu Y, Dissing-Olesen L, MacVicar BA, et al. Microglia: Dynamic mediators of synapse development and plasticity. Trends Immunol. (2015) ; 36: (10): 605-613. |
[5] | Bechade C, Cantaut-Belarif Y, Bessis A. Microglial control of neuronal activity. Front Cell Neurosci. (2013) ; 7: : 32. |
[6] | Ji K, Miyauchi J, Tsirka SE. Microglia: An active player in the regulation of synaptic activity. Neural Plast. (2013) ; 2013: : 627325. |
[7] | Blinzinger K, Kreutzberg G. Displacement of synaptic terminals from regenerating motoneurons by microglial cells. Z Zellforsch Mikrosk Anat. (1968) ; 85: (2): 145-157. |
[8] | Rajendran L, Paolicelli RC. Microglia-mediated synapse loss in alzheimer’s disease. J Neurosci. (2018) ; 38: (12): 2911-2919. |
[9] | Miyanishi K, Sato A, Kihara N, et al. Synaptic elimination by microglia and disturbed higher brain functions. Neurochem Int. (2021) ; 142: : 104901. |
[10] | Milior G, Lecours C, Samson L, et al. Fractalkine receptor deficiency impairs microglial and neuronal responsiveness to chronic stress. Brain Behav Immun. (2016) ; 55: : 114-125. |
[11] | Tay TL, Savage JC, Hui CW, et al. Microglia across the lifespan: From origin to function in brain development, plasticity and cognition. J Physiol. (2017) ; 595: (6): 1929-1945. |
[12] | Rogers JT, Morganti JM, Bachstetter AD, et al. CX3CR1 deficiency leads to impairment of hippocampal cognitive function and synaptic plasticity. J Neurosci. (2011) ; 31: (45): 16241-16250. |
[13] | Parkhurst CN, Yang G, Ninan I, et al. Microglia promote learning-dependent synapse formation through brain-derived neurotrophic factor. Cell. (2013) ; 155: (7): 1596-1609. |
[14] | Sui L, Wang J, Li BM. Role of the phosphoinositide 3-kinase-Akt-mammalian target of the rapamycin signaling pathway in long-term potentiation and trace fear conditioning memory in rat medial prefrontal cortex. Learn Mem. (2008) ; 15: (10): 762-776. |
[15] | Tao X, Finkbeiner S, Arnold DB, et al. Ca2+ influx regulates BDNF transcription by a CREB family transcription factor-dependent mechanism. Neuron. (1998) ; 20: (4): 709-726. |
[16] | Welberg L. Synaptic plasticity: A synaptic role for microglia. Nat Rev Neurosci. (2014) ; 15: (2): 69. |
[17] | Saw G, Krishna K, Gupta N, et al. Epigenetic regulation of microglial phosphatidylinositol 3-kinase pathway involved in long-term potentiation and synaptic plasticity in rats. Glia. (2020) ; 68: (3): 656-669. |
[18] | Souza RR, Noble LJ, McIntyre CK. Using the single prolonged stress model to examine the pathophysiology of PTSD. Front Pharmacol. (2017) ; 8: : 615. |
[19] | Li M, Xie Y, Niu K, et al. Electroacupuncture ameliorates post-traumatic stress disorder in rats via a mechanism involving the BDNF-TrkB signaling pathway. Cell Mol Biol (Noisy-le-grand). (2020) ; 66: (3): 165-170. |
[20] | Yi G. Experimental Acupuncture. 5. China Traditional Chinese Medicine Press. (2021) . |
[21] | Li M, Li K, Zhang H, et al. Study on the mechanism of TMRK electroacupuncture in repairing synaptic plasticity in amygdala and hippocampus to relieve fear memory in PTSD rats. Technol Health Care. (2019) ; 27: (S1): 425-443. |
[22] | Milad MR, Pitman RK, Ellis CB, et al. Neurobiological basis of failure to recall extinction memory in posttraumatic stress disorder. Biol Psychiatry. (2009) ; 66: (12): 1075-1082. |
[23] | Lee B. Neuroprotective effect of acupuncture against single prolonged stress-induced memory impairments and inflammation in rat brain via modulation of brain-derived neurotrophic factor expression. Evid Based Complement Alternat Med. (2022) ; 2022: : 4430484. |
[24] | Li M, Li K, Zhang H, et al. Study on the mechanism of TMRK electroacupuncture in repairing synaptic plasticity in amygdala and hippocampus to relieve fear memory in PTSD rats. Technol Health Care. (2019) ; 27: (S1): 425-443. |
[25] | Kwon CY, Lee B, Kim SH. Efficacy and underlying mechanism of acupuncture in the treatment of posttraumatic stress disorder: A systematic review of animal studies. J Clin Med. (2021) ; 10: (8). |
[26] | Deslauriers J, Toth M, Der-Avakian A, et al. Current status of animal models of posttraumatic stress disorder: Behavioral and biological phenotypes, and future challenges in improving translation. Biol Psychiatry. (2018) ; 83: (10): 895-907. |
[27] | Kritman M, Maroun M. Inhibition of the PI3 kinase cascade in corticolimbic circuit: Temporal and differential effects on contextual fear and extinction. Int J Neuropsychopharmacol. (2013) ; 16: (4): 825-833. |
[28] | Maddika S, Kavela S, Rani N, et al. WWP2 is an E3 ubiquitin ligase for PTEN. Nat Cell Biol. (2011) ; 13: (6): 728-733. |
[29] | Li H, Zhang P, Zhang Q, et al. WWP2 is a physiological ubiquitin ligase for phosphatase and tensin homolog (PTEN) in mice. J Biol Chem. (2018) ; 293: (23): 8886-8899. |
[30] | Zhi J. Mechanism of electroacupuncture on inhibiting myocardial hypertrophy in spontaneously hypertensive rats by regulating PI3K/AKT pathway. Beijing University of Traditional Chinese Medicine. (2019) . |
[31] | Zhe W, Zanhua Z. Effects of electroacupuncture on the expression of PTEN/mTOR signaling pathway in spinal cord injury. Liaoning Journal of Traditional Chinese Medicine. (2020) ; 47: (04): 198-200. |
[32] | Spadaro PA, Flavell CR, Widagdo J, et al. Long Noncoding RNA-Directed Epigenetic Regulation of Gene Expression Is Associated With Anxiety-like Behavior in Mice. Biol Psychiatry. (2015) ; 78: (12): 848-859. |
[33] | Daskalakis NP, Provost AC, Hunter RG, et al. Noncoding RNAs: Stress, glucocorticoids, and posttraumatic stress disorder. Biol Psychiatry. (2018) ; 83: (10): 849-865. |