An automatic recognition method of nematode survival rate based on bright field and dark field experimental images
Abstract
BACKGROUND:
The survival rate of experimental animals is a very important index in chemical toxicity evaluation experiments. The calculation of nematode survival rate is used in many experiments.
OBJECTIVE:
Traditional survival rate quantification methods require manual counting. This is a time-consuming and laborious work when using 384-well plate for high-throughput chemical toxicity assessment experiments. At present, there is a great need for an automatic method to identify the survival rate of nematodes in the experiment of chemical toxicity evaluation.
METHODS:
We designed an automatic nematode survival rate recognition method by combining the bright field experimental image of nematodes and the dark field image of nematodes which is captured after adding Propidium Iodide dye, and used it to calculate the nematode survival rate in different chemical environments. Experiment results show that the survival rate obtained by our automatic counting method is very similar to the survival rate obtained by manual counting.
RESULTS:
Through several different chemical experiments, we can see that chemicals with different toxicity have different effects on the survival rate of nematodes. And the survival rate of nematodes under different chemical concentrations has an obvious gradient trend from high concentration to low concentration. In addition, our method can quantify the motility of nematodes. There are also significant differences in the motility of nematodes cultured in different chemical environments. Moreover, the nematode motility under different chemical concentrations showed an obvious gradient change trend from high concentration to low concentration.
CONCLUSION:
Our study provides an accurate and efficient nematode survival rate recognition method for chemical toxicology research.
1.Introduction
With the development of technology, various environmental factors are recognized as hazarders to health. The traditional method of chemical toxicity assessment is rodent model animals-based. Current in vivo rodent models of toxicity can no longer meet the increasing requirements of toxicological evaluation of so many new chemicals. The conventional animal testing is labor intensive and time consuming, resulting in a large number of toxicants not being tested at all. In addition, the new model and assessment approach are urgently required due to the European Union’s Cosmetics Regulation, which has forbidden the use of ingredients tested in animals since March 2013, and the growing discrepancy between the number of chemicals being introduced into commerce and the ability to thoroughly evaluate their toxicity in animals. Therefore, there is a critical need to develop more efficient and accurate techniques of toxicity predictive screening based on the new technology and methods.
The nematode Caenorhabditis elegans is a classic model animal in the research of life science. It has great potential value in the chemical rapid toxicity screening and complying with principles of “3R” for short life cycle, easy operation and low cost. Undoubtedly, C. elegans has emerged as an important model animal in various fields including neurobiology, developmental biology, aging, and genetics [1, 2, 3]. C. elegans represents an excellent complement to in vitro cell culture-based systems and in vivo vertebrate models. In the recent decades, C. elegans has been proven to be a valuable model animal for both toxicity assessment and toxicological mechanism study of complex chemicals, such as heavy metal, fine particulate matter (PM2.5), and so on [4, 5, 6, 7, 8]. Compared with other model organisms, such as mice, nematodes have the advantages of low cost and easy operation. The use of nematodes in chemical toxicity assessment can increase the chemical toxicity evaluation efficiency, reduce cost, as well as refine, reduce, or replace vertebrate animal testing. Considering the welfare of experimental animals and the principle of substitution of experimental animals, it is very meaningful to use nematodes for chemical toxicity screening and evaluation experiments, particularly, by a way of high-throughput screening. The objective of high-throughput chemical screening is to shortlist chemicals showing high toxicity, thereby setting priority for regulations as well as further toxicity testing in mammalian models.
The survival rate of experimental animal is the most important index to measure the toxicity of chemicals, whether using rodents or nematodes. Previously, the computation of survival rate of nematodes requires manual counting, which is time-consuming and laborious. At present, there is a great need for an automatic method to identify the survival rate of nematodes in the experiment of chemical toxicity evaluation using nematodes.
Experiments using nematodes for chemical toxicity assessment generally measure the survival rate of nematodes after 12 or 24 hours of cultivation in the environment with chemicals. We have previously demonstrated that C. elegans as a multicellular model organism may have greater advantages in predicting the rodent acute toxicity than in vitro cytotoxicity model, and the more toxic the chemicals, the higher the mortality of nematodes. Compared with less toxic chemicals, nematodes cultured in more toxic chemicals die more after a certain period of time [9].
In some studies, the phenotype of nematodes was calculated by processing the experimental images of nematodes [10, 11]. However, these methods are difficult to accurately calculate the survival rate of nematodes in a short time because of the influence of some factors such as the activity frequency of nematodes, the morphology of nematodes and the length of experimental video shooting time.
Designing an automatic calculation method for nematode survival rate is very helpful for the toxicity assessment of chemicals using nematodes. Here, we designed an automatic nematode survival rate recognition method and used it to calculate the nematode survival rate in different chemical environments.
2.Methods
2.1C. elegans culture conditions
Wild-type (N2) strain of C. elegans was maintained on NGM agar plates (3 g/L NaCl, 17 g/L agar, 2.5 g/L peptone, 1 ml/L cholesterol (5 mg/methyl alcohol), 1 ml/L 1M MgSO
2.2Growth synchronization
N2 animals were grown to gravid adult on 90 mm NGM agar plates supplemented with E. coli OP50. The plates were rinsed with M9 buffer (3 g/L KH
2.3The used chemicals and concentrations
The Cadmium chloride was obtained from Sigma-Aldrich. The Saffron was obtained from Huamiao Co. The solutions were prepared in K-Miedium (3.04 g NaCl and 2.39 g KCl in 1L ultrapure water) [7]. According to the C. elegans 24 h median lethal concentration (LC
Table 1
List of chemicals and concentrations
Group name | Chemical name | Concentration (mg/ml) |
---|---|---|
X1 | Saffron | 0.5 |
X2 | Saffron | 0.25 |
X3 | Saffron | 0.125 |
X4 | Saffron | 0.0625 |
Cd1 | Cadmium chloride | 8 |
Cd2 | Cadmium chloride | 4 |
Cd3 | Cadmium chloride | 2 |
Cd4 | Cadmium chloride | 1 |
K | Control (k-medium) | 0 |
2.4Chemical treatment
Worms were exposed to chemicals for 384-well plate acute toxicity experiment tests. Eight parallel tests were then carried out for the specific points of the concentration gradients for each chemical. Worms were then observed with each specific chemical condition in K-medium for 24 h. In every single well, about 10
2.5Experimental images and videos capture
Videos of each well were taken with the programmed acquisition procedure under the bright field (10 frames per well) and dark field (3 frames per well). 1 uL PI (1mg/mL) dye was added in each well before take the dark field videos. All videos are collected using the digital camera (ZEISS, Observer.Z1) attached to an inverted microscope [7].
2.6Bright field image processing
The bright field experimental image processing pipeline is shown in the first row of Fig. 4. First, the color image is converted to gray image; and then the uneven illumination in bright field image is solved [6, 11]. Next, we segment the gray image and get the segmented result image. We can calculate the number of nematodes in the segmented result image.
2.7Dark field image processing
The dark field experimental image processing pipeline is shown in the second row of Fig. 4. First, the color image is converted to gray image. Next, we segment the gray image and get the segmented result image. We can calculate the number of nematodes in the segmented result image. In dark field images, only dead nematodes show fluorescence. The number of live nematodes is obtained by subtracting the number of nematodes in the dark field image from the number of nematodes in the bright field image.
2.8Bright field video processing
The motility of nematodes can be obtained by processing the experimental video of nematodes. The motility reflects the average motility of nematodes in each chemical environment. Each video consists of a fixed number of frames. Each frame is an image, it is processed in the same way as above. Motility is quantified by the change of nematode body position in two adjacent frames.
2.9Survival rate quantification automatically
The number of nematodes identified from the bright field experiment image is the original number of nematodes (set it as x). The nematodes identified from the dark field experimental images are the number of nematodes that died under different experimental conditions (set it as y). Then, the survival rate of nematodes under different experimental conditions is (x-y)/x.
2.10Quantification of worm motility
We designed the method to quantify the motility of nematodes cultured in different chemical environments for 24 hours. Nematode motility is a phenotype that is difficult to quantify manually. By shooting and analyzing the nematode experiment video, we can quantitatively give the nematode motility in different environments. The motility of nematodes can be obtained by processing the experimental video of nematodes. The motility reflects the average motility of nematodes in each chemical environment. Each video consists of a fixed number of frames. Each frame is an image, it is processed in the same way as above. Motility is quantified by the change of nematode body position in two adjacent frames.
2.11Statistical analysis
Results are presented as means
3.Results
3.1The survival rate of different chemicals
The calculation of nematode survival rate is used in many experiments. Traditional methods require manual counting. This is a time-consuming and laborious work. We designed an automatic nematode survival rate recognition method and used it to calculate the nematode survival rate in different chemical environments. We can automatically identify the survival rate of nematodes by combining the bright field experimental image of nematodes and the dark field image after adding Propidium Iodide (PI) dye. In the dark field experiment image after adding the PI dye, the dead nematode will have strong fluorescence. Thus, the dead nematodes can be identified from the dark field experiment images [9].
We designed experiments of nematodes in several different chemical environments. The used chemicals and concentrations are shown in Table 1. These chemicals have varying degrees of toxicity. Each chemical also has different toxicity at different concentrations. Figure 1 shows the survival rate of nematodes in different experimental environments obtained by our automatic recognition method. The 24-hour survival rate after the addition of chemicals is an important indicator of toxicity. In Fig. 1, we can see that there is significant difference among different chemicals and different concentrations in 24-hour survival rate. The survival rate obtained by our automatic calculation method shows that the survival rate of nematodes decreases with the increase of saffron concentration. Compared with the Control (k-medium) group, different concentrations of Cadmium chloride will affect the survival rate of nematodes. Cadmium chloride has great toxicity to nematodes. Compared with saffron, Cadmium chloride had a greater effect on the survival rate of nematodes. The survival rate obtained by our automatic recognition method shows that the survival rate of nematodes decreased with the increase of Cadmium chloride concentration.
Figure 1.
The program automatic counting worm survival rate results under different chemicals with different concentrations.
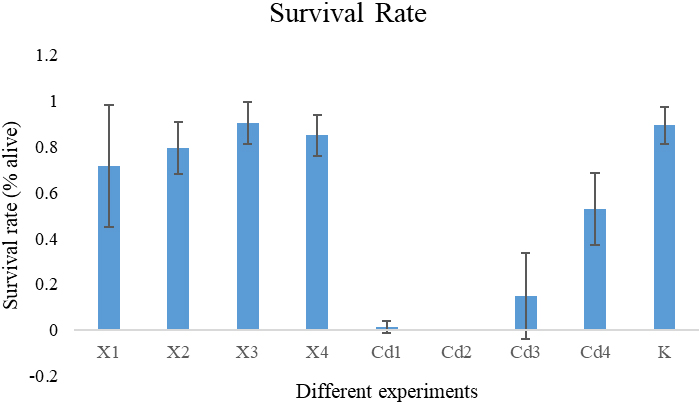
Figure 2.
The manual counting survival rate results and the program automatic counting survival rate results.
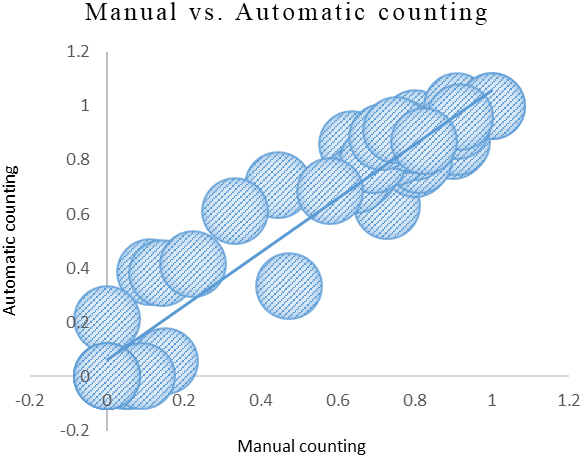
Figure 3.
The program automatic quantified worm motility under different chemicals with different concentrations.
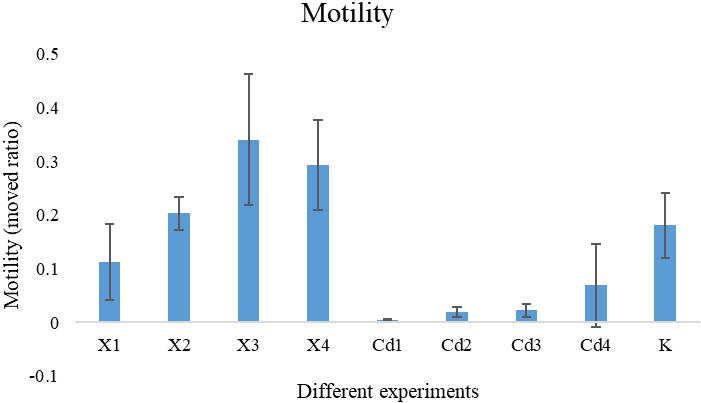
Figure 4.
The pipeline of the process of bright field and dark field experimental images (2.5X, Inverted ZEISS Observer.Z1). The first row is the processing flow of bright field experimental image. The second row is the processing flow of dark field experiment image.
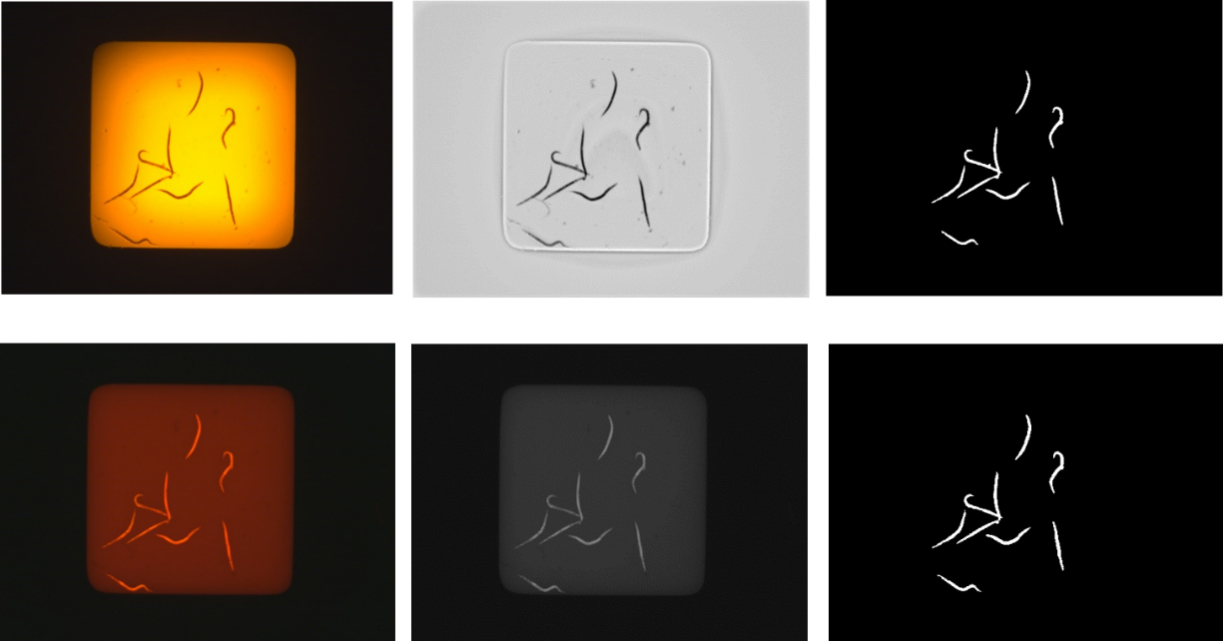
We also use the traditional method [9] to compute the nematode survival rate manually. Figure 2 shows the comparison between the nematode survival rate obtained by manual counting and the nematode survival rate obtained by our automatic counting method. The x-axis in the figure is the survival rate of nematodes obtained by manual counting, and the y-axis is the survival rate of nematodes obtained by our automatic counting method. In Fig. 2, we can see that the manual counting survival rate results and the program automatic counting survival rate results are similar. The Pearson correlation coefficient (PCC) between the manual counting survival rate results and the program automatic counting survival rate results is 0.9606431. This proves that the survival rate obtained by our automatic counting method is very similar to the survival rate obtained by manual counting.
The results show that the designed method can automatically and accurately calculate the survival rate of nematodes in different chemical environments and it will save a lot of manpower and avoid the operation deviation of different experimenters.
3.2Quantification of worm motility
In addition to the survival rate of nematodes, the activity ability of nematodes is another important index to observe the impact of different chemicals on nematodes.
Figure 3 shows the quantitative results of nematode motility in different chemical environments. From the figure, we can see that saffron and Cadmium chloride have an effect on the motility of nematodes. Because of the high toxicity of Cadmium chloride, most nematodes have died after being cultured in the environment with Cadmium chloride for 24 hours. Even in the environment with low concentration of Cadmium chloride, the motility of nematodes after 24 hours of culture is relatively weak. Saffron is less toxic than Cadmium chloride. Saffron has little effect on the survival rate of nematodes. Saffron will not reduce the motility of nematodes, and low concentration of saffron can promote the motility of nematodes. Compared with the control group, the nematodes cultured in the environment with low concentration of saffron have higher motility.
4.Discussion
The toxicity assessment and risk management of chemicals is highly associated with human health. At present, the conventional model of which rodent tests can no longer meet the increasing requirements of chemical toxicological evaluation. Studies on the non-rodent in vivo model organisms are becoming a potential perspective and frontiers in the research field of chemicals toxicity risk assessment. Meanwhile, there is an urgent need to develop more efficient and accurate screening techniques for toxicity prediction in Tox21 (Toxicology in the 21st Century) program, which is a multiagency collaborative effort among the NIH’s NIEHS/NTP, the EPA, and the FDA [12]. Its Phase III proposed that incorporate into the testing strategy more physiologically-relevant cell types and lower organisms (e.g., zebrafish, C. elegans), coupled to high content screening and high throughput transcriptomics platforms to assess chemical toxicity [13].
Nematode is a very important model organism [14]. Work in C. elegans has led to seminal discoveries in neuroscience, development, signal transduction, cell death, aging, and RNA interference [15]. The unique features of C. elegans make it an excellent model to complement mammalian models in toxicology research. Characteristics of this model animal that have contributed to its success include its well-characterized genome and genetic manipulability, invariant and fully described developmental program, ease of maintenance, short life cycle, simple construction and small body size. This model organism has been used in the area of toxicology [10, 16]. Many researches demonstrated that C. elegans is an efficient and sensitive model for assessing chemical toxicity.
C. elegans is a valuable model for predicting chemicals’ acute toxicity in rodents [9, 17]. The unique features of C. elegans indicate that the nematode is an excellent model to complement mammalian models in toxicology research. Experiments with C. elegans do not have the experimental complexity as in vertebrate models, yet still permit the testing of hypotheses in vivo. Evidences show that there is a significant correlation of chemical acute toxicity between the nematode and the rodent [9]. High-throughput chemical screening in 384 well plates or microfluidic chips with nematode has been proposed as a quicker and less expensive method for toxicity testing [6, 18]. This makes nematodes to be used in many chemical toxicity assessment experiments [16, 19, 20, 21, 22]. Thus C. elegans may provide a rapid, efficient and cost-effective method for predicting the acute toxicity of a large number of chemicals in a relatively short time.
However, until now, there is no automatic calculation method to accurately calculate the survival rate of nematodes in a short time because of the influence of diverse factors such as the activity frequency of nematodes, the morphology of nematodes and the length of experimental video shooting time. Experiments using nematodes for chemical toxicity assessment generally measure the survival rate of nematodes after 12 or 24 hours of cultivation in the environment with chemicals [9, 23]. The more toxic the chemicals, the higher the mortality of nematodes. Compared with less toxic chemicals, nematodes cultured in more toxic chemicals die more after a certain period of time.
Chemical toxicity assessment experiment is different from experiments such as aging. Most aging related experiments need to maintain the observation of nematodes throughout their life cycle. In the toxicity evaluation experiment, we only need to observe the survival rate in a fixed period of time. Therefore, PI dye can be used to identify whether nematodes survive, so as to improve the accuracy of nematode survival rate identification. In addition, our method can quantify the motility of nematodes. Thus, the effects of different chemicals on nematodes can be studied from more indicators.
We choose the Saffron as the subject, because of the crocin, an active constituent of Crocus sativus L. (saffron) has the protective effect on neurotoxicity in Wistar rats. The Cadmium chloride was used as the positive control [24]. We found that the nematodes exhibit motility decline rather than death when exposed to high concentration of Saffron.
Propidium iodide cannot cross the membrane of live cells and has evidenced of having no toxic effects on neurons [25]. Flow cytometric method for measuring the percentage of apoptotic nuclei after propidium iodide staining in hypotonic buffer proves useful for assessing apoptosis of specific cell populations in heterogeneous tissues such as bone marrow, thymus and lymph nodes [26]. But propidium iodide was confirmed that has acute toxicity on mouse (LD
5.Conclusion
The proposed method can automatically calculate the survival rate of nematodes in different chemical environments. This will save a lot of manpower and avoid the operation deviation of different experimenters. Experiment results show that the survival rate obtained by our automatic counting method is very similar to the survival rate obtained by manual counting. Through several different chemical experiments, we can see that chemicals with different toxicity have different effects on the survival rate of nematodes. And the survival rate of nematodes under different chemical concentrations has an obvious gradient trend from high concentration to low concentration. In addition, our method can quantify the motility of nematodes. There are also significant differences in the motility of nematodes cultured in different chemical environments. Moreover, the nematode motility under different chemical concentrations showed an obvious gradient change trend from high concentration to low concentration.
Acknowledgments
This work was supported by the National Key Research and Development Program of China (No. 2019YFC1604901).
Conflict of interest
None to report.
References
[1] | Apfeld J, Alper S. What Can We Learn About Human Disease from the Nematode C. elegans? In: DiStefano JK, editor. Disease Gene Identification: Methods and Protocols. New York, NY: Springer New York; (2018) . pp. 53-75. |
[2] | Park H-EH, Jung Y, Lee S-JV. Survival assays using Caenorhabditis elegans. Molecules and Cells. (2017) ; 40: : 90-9. |
[3] | Yuan J, Chang S-Y, Yin S-G, Liu Z-Y, Cheng X, Liu X-J, et al. Two conserved epigenetic regulators prevent healthy ageing. Nature. (2020) ; 579: : 118-22. |
[4] | Boyd WA, McBride SJ, Rice JR, Snyder DW, Freedman JH. A high-throughput method for assessing chemical toxicity using a Caenorhabditis elegans reproduction assay. Toxicology and Applied Pharmacology. (2010) ; 245: : 153-9. |
[5] | Boyd WA, Williams PL. Comparison of the sensitivity of three nematode species to copper and their utility in aquatic and soil toxicity tests. Environmental Toxicology and Chemistry. (2003) ; 22: : 2768-74. |
[6] | Gao S, Chen W, Zeng Y, Jing H, Zhang N, Flavel M, et al. Classification and prediction of toxicity of chemicals using an automated phenotypic profiling of Caenorhabditis elegans. BMC Pharmacology and Toxicology. (2018) ; 19: : 18. |
[7] | Gao S, Chen W, Zhang N, Xu C, Jing H, Zhang W, et al. A High-throughput Assay for the Prediction of Chemical Toxicity by Automated Phenotypic Profiling of Caenorhabditis elegans. JoVE. (2019) ; e59082. |
[8] | Kim M, Jeong J, Kim H, Choi J. High-throughput COPAS assay for screening of developmental and reproductive toxicity of nanoparticles using the nematode Caenorhabditis elegans. Journal of Applied Toxicology. (2019) ; 39: : 1470-9. |
[9] | Li Y, Gao S, Jing H, Qi L, Ning J, Tan Z, et al. Correlation of chemical acute toxicity between the nematode and the rodent. Toxicology Research. (2013) ; 2: : 403-12. |
[10] | Stroustrup N, Ulmschneider BE, Nash ZM, López-Moyado IF, Apfeld J, Fontana W. The Caenorhabditis elegans Lifespan Machine. Nature Methods. (2013) ; 10: : 665-70. |
[11] | Wählby C, Kamentsky L, Liu ZH, Riklin-Raviv T, Conery AL, O’Rourke EJ, et al. An image analysis toolbox for high-throughput C. elegans assays. Nature Methods. (2012) ; 9: : 714-6. |
[12] | Krewski D, Acosta D Jr, Andersen M, Anderson H, Bailar JC 3rd, Boekelheide K, et al. Toxicity testing in the 21st century: A vision and a strategy. J Toxicol Environ Health B Crit Rev. (2010) ; 13: : 51-138. |
[13] | Paules RS. The future of Tox21 – Improving on biological coverage and human relevance using high-throughput transcriptomic technologies. Toxicology Letters. (2016) ; 259: : S67. |
[14] | Brenner S. The genetics of Caenorhabditis elegans. Genetics. (1974) ; 77: : 71-94. |
[15] | Antoshechkin I, Sternberg PW. The versatile worm: Genetic and genomic resources for Caenorhabditis elegans research. Nature Reviews Genetics. (2007) ; 8: : 518-32. |
[16] | Leung MCK, Williams PL, Benedetto A, Au C, Helmcke KJ, Aschner M, et al. Caenorhabditis elegans: An emerging model in biomedical and environmental toxicology. Toxicological Sciences. (2008) ; 106: : 5-28. |
[17] | Boyd WA, Smith MV, Co CA, Pirone JR, Rice JR, Shockley KR, et al. Developmental Effects of the ToxCastTM Phase I and Phase II Chemicals in Caenorhabditis elegans and Corresponding Responses in Zebrafish, Rats, and Rabbits. Environ Health Perspect. (2016) ; 124: : 586-93. |
[18] | Xian B, Shen J, Chen W, Sun N, Qiao N, Jiang D, et al. WormFarm: A quantitative control and measurement device toward automated Caenorhabditis elegans aging analysis. Aging Cell. (2013) ; 12: : 398-409. |
[19] | Moyson S, Vissenberg K, Fransen E, Blust R, Husson SJ. Mixture effects of copper, cadmium, and zinc on mortality and behavior of Caenorhabditis elegans. Environmental Toxicology and Chemistry. (2018) ; 37: : 145-59. |
[20] | Dengg M, van Meel JCA. Caenorhabditis elegans as model system for rapid toxicity assessment of pharmaceutical compounds. Journal of Pharmacological and Toxicological Methods. (2004) ; 50: : 209-14. |
[21] | Schouest K, Zitova A, Spillane C, Papkovsky DB. Toxicological assessment of chemicals using Caenorhabditis elegans and optical oxygen respirometry. Environmental Toxicology and Chemistry. (2009) ; 28: : 791-9. |
[22] | Sprando RL, Olejnik N, Cinar HN, Ferguson M. A method to rank order water soluble compounds according to their toxicity using Caenorhabditis elegans, a Complex Object Parametric Analyzer and Sorter, and axenic liquid media. Food and Chemical Toxicology. (2009) ; 47: : 722-8. |
[23] | Mehri S, Abnous K, Khooei A, Mousavi SH, Shariaty VM, Hosseinzadeh H. Crocin reduced acrylamide-induced neurotoxicity in Wistar rat through inhibition of oxidative stress. Iran J Basic Med Sci. (2015) ; 18: : 902-8. |
[24] | Lawes M, Pinkas A, Frohlich BA, Iroegbu JD, Ijomone OM, Aschner M. Metal-induced neurotoxicity in a RAGE-expressing C. elegans model. Neurotoxicology. (2020) ; 80: : 71-5. |
[25] | Hezel M, Ebrahimi F, Koch M, Dehghani F. Propidium iodide staining: A new application in fluorescence microscopy for analysis of cytoarchitecture in adult and developing rodent brain. Micron. (2012) ; 43: : 1031-8. |
[26] | Nicoletti I, Migliorati G, Pagliacci MC, Grignani F, Riccardi C. A rapid and simple method for measuring thymocyte apoptosis by propidium iodide staining and flow cytometry. J Immunol Methods. (1991) ; 139: : 271-9. |
[27] | WOOLFE. Trypanocidal action of phenanthridine compounds: Further 2: 7-diamino phenanthridinium compounds. Br J Pharmacol Chemother. (1956) ; 11: : 334-8. |