Evaluation of scattered radiation dose received by medical staff during uterine artery embolization in the operating room
Abstract
BACKGROUND:
The air kerma radiation doses have gained much attention since the operating room interventional radiology is a place where medical staff are exposed to a fluoroscopy environment and gain a cumulative dose during the uterine artery embolization procedure.
OBJECTIVE:
We aimed to evaluate the radiation dose received by medical staff by applying a flat X-ray machine in the surgical room during uterine artery embolization.
METHODS:
An ATOM humanoid model was laid on the operating table and simulated a patient. The scattered radiation dose received by the radiologist, anesthetist and radiologic technologist was evaluated. The scintillation detector was adopted. The measurement points were 50 cm, 100 cm and 150 cm above the floor, representing the limbs, abdomen and thyroid level, respectively. We compared the X-rays under different tube voltages of 70, 80, and 90, respectively and frames per second (FPS) of 30, 15, and 7.5, respectively. We configured the dose level per pulse of 40 nGy with a fixed detector.
RESULTS:
In Section 1, when the tube voltage was 70 kVp and 7.5 FPS, the average radiation doses of limbs, abdomen and thyroid level was 0.48, 1.3 and 1.9
CONCLUSIONS:
Lead garment can effectively reduce medical staff from occupational doses with an average attenuation rate of 90%. 80 kVp was most commonly used. Fluoroscopy 7.5 FPS was used 100 cm above the floor in A section and the lowest radiation dose was 1.33
1.Introduction
Medical radiation is widely used in medical imaging diagnosis and treatment. With the development of the mobile X-ray imaging system, digital imaging can help doctors to make an instant diagnosis of discomfort in cardiopulmonary and chest, emphysema, hemothorax, cardiopulmonary resuscitation and follow-up care of intraoperative and postoperative patients [1]. Mobile X-ray equipment is commonly used in the normal ward, intensive ward and operating room, especially for patients who cannot leave the room [2, 3, 4, 5, 6, 7]. However, the mobile X-ray can only produce a single image. The C-arm mobile X-ray can produce a dynamic image, and provide angiography in interventional operation. While using the C-arm mobile X-ray, medical staff needs to examine the X-ray and wear lead garments to protect themselves from radiation. In interventional therapy, medical staff cannot avoid scattered radiation. Though the amount of radiation is low, they can get a radiation injury during long-time exposure [8]. According to the International Commission on Radiological Protection (ICRP) Report No. 160 in 2006, medical radiation increased from 15% in 1980 to 45% [9], with 5% from diagnostic X-ray radiation and 50% from natural radiation. In 2008 in Taiwan, 551 out of every 1,000 people received X-ray radiation [10]. According to the Radiation Safety Standard, the annual radiation dose per person is exclusive of natural radiation and medical radiation. Annual natural radiation in Taiwan is 2mSv. According to the United National Scientific Committee on the Effects of Atomic Radiation (UNSCEAR), medical radiation accounts for 98.2% of artificial radiation. In 2007, ICRP set up three principles for radiation protection. First, the X-ray should be used legally and the use of retrospective image reduces fluoroscopy time. Second, radiation protection should be optimized, remind radiologists of the continuous fluoroscopy time, and radiation dose between patients and staff must be reduced. Third, radiation doses should be regulated, check whether staff members are wearing lead protective clothing and affix personal doses to the inside of the leading garment [11]. ICRP principles are on quantitative standard and optimized precondition. Since the risk is different in different radiation conditions, radiation protection changes accordingly. We must follow as low as reasonably achievable (ALARA) principle, and adopt the lowest X-ray dose to satisfy the required medical imaging examination [12, 13]. Radiation technologists provide the best image parameters in the operating room operating X-ray machines. Thus, the radiation dose received by medical staff and patients can be reduced.
Uterine artery embolization (UAE) is an effective way to control postpartum hemorrhage [14, 15]. The neonate will not be exposed to radiation exposure during uterine artery embolization examination. This experience led to the broader application of UAE as an alternative to surgery. Medical staff uses the C-arm mobile X-ray machine to conduct interventional therapy. Surgeon and medical staff occupation doses are strongly dependent on a number of factors, including the clinical condition of the patient, X-ray quality, fluoroscopy time and number of digital subtraction angiography (DSA) radiographs, physician skill and others. The measurement medical staff occupation doses usually use thermoluminescence dosimeters (TLD) and optically stimulated luminescence dosimeters (OSLD) but single examination of cumulative scattered radiation below in the protective clothing prevents dosimeter measurement [16]. However, there is no research on the radiation dose received by medical staff using the C-arm mobile X-ray machine during UAE, or scattered radiation data in different frames per second. Therefore, this research aims to evaluate radiation exposure of medical staff in UAE. We used a scintillation detector to simulate experiments with different X-ray sequences and tube voltages. The medical staff wore protective clothing, i.e. 0.5 mm-Pb-equivalent apron, thyroid collar and lead loaded glasses, which reduced the scatter radiation doses. This shows that radiation exposure levels can be reduced through the use of personal protective equipment.
2.Materials and methods
2.1X-ray machines
The flat mobile X-ray machine was used in this study. The size of the screen is 30
2.2ATOM phantom
In the present work, the CIRS ATOM female phantom (Model number 702-D, Computed Imaging Reference Systems Inc., Norfolk, USA) is an anthropomorphic. The selected prosthesis was female, with a height and weight of 160 cm and 55 kg, respectively. The prosthesis had no hands or feet and consisted of a human skeleton and soft tissues with densities similar to the human body, and was placed on an operating table for exposure to radiation.
2.3Radiation dosimeters
We employed a radiation dosimeter AT1121 scintillation detector to test the scattered radiation received by the medical staff. The tube voltages were 70, 80, 90 kVp, respectively. The average power ranged between 35 to 45 kVp. The X-ray spectrum of 70 to 90 kVp spread from 0–80 keV, with most X-rays between 25 to 70 keV. Hence, the power in such conditions is about 1.0 to 1.2. In this work, 1.1 was adopted. The scintillation detector was checked by Taiwan Atomic Energy Committee Nuclear Energy Institute, so the accuracy of the data is guaranteed. On 24 July 2017, the adjusted value of this machine was 0.88, which means that the reading value should multiplied by 0.88 to get the real value. The minimum detectable dose of AT1121 scintillation detector is 50 nSv and the minimum dose rate is 50 nSv/h. The adopted dose we used in this work is 82 nSv for 65 minutes, so the background dose rate is 0.0013
Table 1
Phantom study estimation of the scattered radiation dose received by medical staff X-ray parameter set
Parameter | Set protocols |
---|---|
Detector pulse (nGy) | 40 |
Tube voltage (keV) | 70; 80; 90 |
Tube current | Auto control |
Frames per second | 30; 15; 7.5 |
Above the floor (cm) | 50; 100; 150 |
Fluoroscopy time (s) | 60 |
Background dose ( | 0.0013 |
Table 2
Estimated upper abdominal dose from 100 cm away from the ground, obtained dose of each point from the scintillation detector dose rate (DR), and predicted exposure dose to human tissue Personnel dose (PD) at Sections 1–3
kVp | 70 | 80 | 90 | ||||
---|---|---|---|---|---|---|---|
Location | FPS | DR | PD | DR | PD | DR | PD |
A | 30 | 1.83 | 2.00 | 3.19 | 3.49 | 3.12 | 3.41 |
15 | 0.37 | 0.40 | 1.55 | 1.69 | 2.95 | 3.22 | |
7.5 | 1.3 | 1.42 | 1.35 | 1.48 | 2.54 | 2.78 | |
B | 30 | 0.01 | 0.01 | 0.02 | 0.02 | 0.05 | 0.05 |
15 | 0.01 | 0.01 | 0.03 | 0.03 | 0.05 | 0.05 | |
7.5 | 0.01 | 0.01 | 0.02 | 0.02 | 0.05 | 0.05 | |
C | 30 | 0.01 | 0.01 | 0.01 | 0.01 | 0.02 | 0.02 |
15 | 0.01 | 0.01 | 0.02 | 0.02 | 0.02 | 0.02 | |
7.5 | 0.01 | 0.01 | 0.01 | 0.01 | 0.02 | 0.02 |
Figure 1.
The distribution in the operating room. Sections 1 and 2 represent the scattered radiation received by the radiologist and anesthetist, respectively. Section 3 represents the scattered radiation received by radiologic technologist.
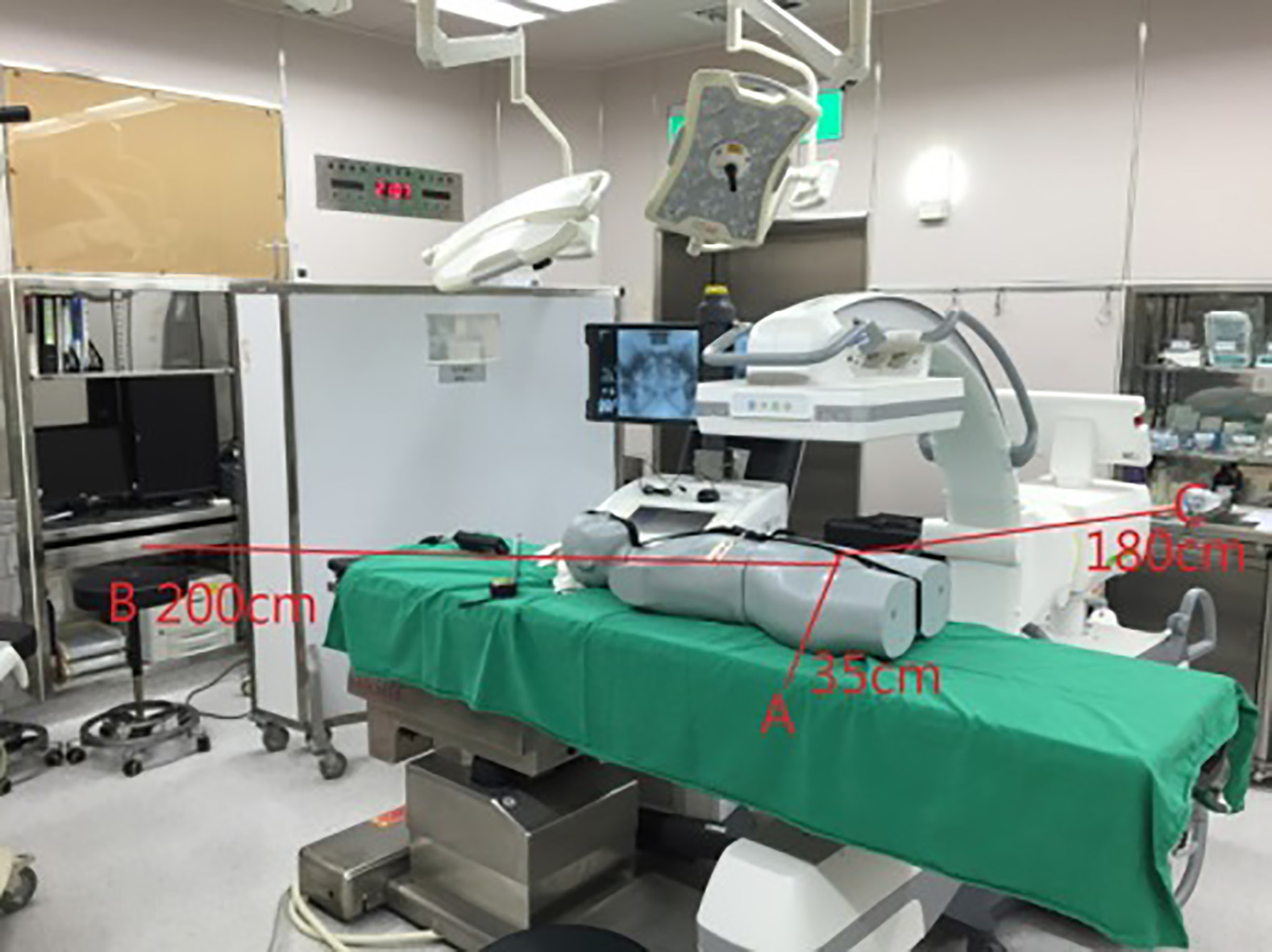
2.4Experimental data for clinical exposure
This study was approved by the Chang Gung Memorial Hospital, Taiwan and approval was granted by the Chang Gung Hospital Institutional Review Board under number 201800590B0. All the data was collected in one surgery room at Kaohsiung Chang Gung Hospital, Taiwan. The ATOM female phantom laid on its back on the operating table, which was 100 cm above the floor, which was according to the clinical practice, radiologists’ height and the minimum operating height of the C-arm X-ray machine. Theoretically, the operating bed height will affect the scattered radiation received at different heights of the medical staff. The density structure of the model’s body is highly similar to the human bone and soft tissue, so it is commonly used for scattered radiation experiments [17, 18]. Radiologist in Section 1, anesthetist in Section 2 and radiologic technologist in Section 3 received different doses of radiation so that the differences could be compared. They were 35 cm, 200 cm and 180 cm away from the central ray. As shown in Fig. 1, using the CIRS ATOM female phantom can represent the scattered radiation that is produced from the patient’s X-ray. The mobile C-arm X-ray machine uses the automatic control parameter. The clinical examination tube voltage varied from 70 to 90 kVp, depending on the patient’s figure. The tube voltage and current was automatically controlled. Fluoroscopy was set at 30 FPS. The tube voltage of the mobile C-arm X-ray machine was clinically set to 70, 80 and 90 kVp. Phantom study estimation of the scattered radiation dose received by medical staff X-ray parameter is listed in Table 1. The fluoroscopies are 30, 15 and 7.5 FPS and were configured with a fixed detector dose level per pulse of 40 nGy. The exposure doses were tested in three different body heights, 50 cm above the floor representing the legs, 100 cm above the floor representing the abdomen and 150 cm above the floor representing the thyroid. Simulative medical staff wear lead garments including protective collar, waistcoat and apron 0.5 mm of Pb equivalent. The area outside lead (OL) garment represents medical staff without protection, and inside lead (IL) garment represents medical staff with protection. We collected the scattered radiation dose of both garments.
Table 3
Scattered radiation received by radiologist in different X-ray parameters
Measure- ment location | FPS | kVp | cm | OL | IL | Attenua- tion rate | kVp | OL | IL | Attenua- tion rate | kVp | OL | IL | Attenua- tion rate |
---|---|---|---|---|---|---|---|---|---|---|---|---|---|---|
50 | 15.09 | 0.75 | 95% | 90.72 | 1.61 | 98% | 127.54 | 3.39 | 97% | |||||
30 | 100 | 38.33 | 1.83 | 95% | 53.78 | 3.19 | 94% | 106.29 | 3.12 | 97% | ||||
150 | 3.90 | 1.40 | 64% | 9.41 | 2.66 | 72% | 17.50 | 4.24 | 76% | |||||
50 | 10.52 | 0.61 | 94% | 86.07 | 1.66 | 98% | 104.54 | 3.52 | 97% | |||||
A | 15 | 70 | 100 | 13.02 | 0.37 | 97% | 80 | 11.66 | 1.55 | 87% | 90 | 85.26 | 2.95 | 97% |
150 | 4.10 | 1.70 | 59% | 8.70 | 4.06 | 53% | 13.89 | 4.13 | 70% | |||||
50 | 9.38 | 0.48 | 95% | 12.43 | 1.36 | 89% | 12.73 | 3.37 | 74% | |||||
7.5 | 100 | 10.78 | 1.30 | 88% | 11.16 | 1.35 | 88% | 12.53 | 2.54 | 80% | ||||
150 | 11.78 | 1.90 | 84% | 6.52 | 2.66 | 59% | 10.80 | 3.51 | 68% | |||||
50 | 0.47 | 0.06 | 87% | 0.65 | 0.08 | 88% | 0.93 | 0.12 | 87% | |||||
30 | 100 | 0.85 | 0.01 | 99% | 1.24 | 0.02 | 98% | 1.74 | 0.05 | 97% | ||||
150 | 0.53 | 0.02 | 96% | 0.76 | 0.04 | 95% | 1.17 | 0.07 | 94% | |||||
50 | 0.39 | 0.02 | 95% | 0.56 | 0.05 | 91% | 0.84 | 0.09 | 89% | |||||
B | 15 | 70 | 100 | 0.78 | 0.01 | 99% | 80 | 1.27 | 0.03 | 98% | 90 | 1.70 | 0.05 | 97% |
150 | 0.51 | 0.02 | 96% | 0.82 | 0.03 | 96% | 1.13 | 0.07 | 94% | |||||
50 | 0.31 | 0.02 | 94% | 0.55 | 0.05 | 91% | 0.74 | 0.06 | 92% | |||||
7.5 | 100 | 0.59 | 0.01 | 98% | 1.08 | 0.02 | 98% | 1.52 | 0.05 | 97% | ||||
150 | 0.37 | 0.01 | 97% | 0.71 | 0.03 | 96% | 0.94 | 0.05 | 95% | |||||
50 | 0.21 | 0.03 | 86% | 0.30 | 0.07 | 77% | 0.43 | 0.08 | 81% | |||||
30 | 100 | 0.18 | 0.01 | 94% | 0.28 | 0.01 | 96% | 0.40 | 0.02 | 95% | ||||
150 | 0.25 | 0.01 | 96% | 0.39 | 0.02 | 95% | 0.62 | 0.05 | 92% | |||||
50 | 0.15 | 0.03 | 80% | 0.25 | 0.05 | 80% | 0.33 | 0.06 | 82% | |||||
C | 15 | 70 | 100 | 0.17 | 0.01 | 94% | 80 | 0.26 | 0.02 | 92% | 90 | 0.35 | 0.02 | 94% |
150 | 0.23 | 0.01 | 96% | 0.40 | 0.02 | 95% | 0.54 | 0.03 | 94% | |||||
50 | 0.11 | 0.04 | 64% | 0.22 | 0.05 | 77% | 0.29 | 0.07 | 76% | |||||
7.5 | 100 | 0.13 | 0.01 | 92% | 0.21 | 0.01 | 95% | 0.27 | 0.02 | 93% | ||||
150 | 0.18 | 0.01 | 94% | 0.33 | 0.02 | 94% | 0.46 | 0.02 | 96% |
Dose unit
3.Results
The experiment was designed to analyze scattered dose received during UAE in different sections. The humanoid model received X-ray. Different heights of the medical staff model (50, 100 and 150 cm) simulate different parts of the body. Fluoroscopy was set at 30, 15, and 7.5 FPS, and tube voltages were set at 70, 80 and 90 kVp. Every tested point continuously received one-minute X-ray. The background radiation is 1.26 nSv/min specifically. Table 2 shows the scattered radiation exposure received by the radiologist in Section 1, on the right side of the patient, 35 cm from the central ray. When the wave sequence is 15 FPS, 100 cm above the floor, in 70 kVp, the exposure rate is lowest at 0.37
Table 3 shows the scattered radiation received by the radiologist in Section 1, by the anesthetist in Section 2 and by the radiologic technologist in Section 3. The occupational exposure dose was accepted at different regions and x-ray tube voltage parameters.
4.Discussion
The clinical data of four patients from June 2015 to May 2016 was collected. The average duration of the X-ray in UAE is 45.9
In Section 1 with 80 kVp 15 fs, the points 50 cm, 100 cm and 150 cm above the floor represent leg, abdomen and thyroid respectively. The scattered radiations OL garment on these points are 86.07, 11.66, and 8.7
There are limitations to this work. First, we manually set the kVp but could not change mA, so our fixed detector dose level per pulse was 40 nGy. Also, the thickness of the model was fixed. We could not evaluate how different thicknesses of the body could affect scattered radiation. However, the machine can adjust mA with kVp to make a proper X-ray. We adopted kVp from 70 to 90, and mA changes from 42 to 68. In clinical condition, we still used automatic tube current. Second, values of different points could not be collected in one exposure, because the scattered radiation IL garment in one minute is too little, TLD is designed to test discontinuous radiation dose in one month. The sensitivity of TLD-100H (LiF: Mg, Cu, P) is 1
5.Conclusions
The research results show the possible radiation dose that medical staff will receive in UAE. By comparing different X-ray scattered radiations in different sections, we learned that higher kVp has more force of penetration. Using 15 FPS in Section 1 was 70, 80, 90 kVp respectively, and protective clothing inside radiation dose was 0.37, 1.55, 2.95 uSv/min respectively. When the 0.5 mm-Pb-equivalent lead garment was set at 90 kvp, the X-ray attenuation capability was 88%. When the tube voltage was 70 kVp, the wave sequence was 15 FPS, and the exposure dose rate was 0.89
The average exposure time for UAE for a procedure was 45.9
Acknowledgments
This study was supported by the Chang Gung Memorial Hospital Taiwan (grant numbers: CMRPG8G1561 and CMRPG8J0081).
Conflict of interest
None to report.
References
[1] | Ganapathy A, Adhikari NK, Spiegelman J, Scales DC. Routine chest x-rays in intensive care units: A systematic review and meta-analysis. Crit Care. (2012) ; 16: (2): R68. |
[2] | McCormick JT, O’mara MS, Papasavas PK, Caushaj PF. The use of routine chest X-ray films after chest tube removal in postoperative cardiac patients. The Annals of Thoracic Surgery. (2002) ; 74: (6): 2161-4. |
[3] | Chen CL. The right posterior sector graft in living donor liver transplantation revisited. Liver Transplantation. (2014) ; 20: (9): 1019-20. |
[4] | Shi Z, Hao H, Zhao M, Feng Y, He L, Wang Y, et al. A deep CNN based transfer learning method for false positive reduction. Multimedia Tools and Applications. (2019) ; 78: (1): 1017-33. |
[5] | Chiang H-W, Chen T-R, Peng S-L, Chiang H-J, Chao S-Y. Application of a computer-assisted system to calculate liver volumetry in living donor liver transplantation. International Journal of Advancements in Computing Technology. (2012) ; 4: (23). |
[6] | Asrani A, Kaewlai R, Digumarthy S, Gilman M, Shepard J-AO. Urgent findings on portable chest radiography: What the radiologist should know. American Journal of Roentgenology. (2011) ; 196: (6_supplement): S45-S61. |
[7] | Zheng X, Chiang H-W, Li J-H, Chiang H-J, Lin L-H. Personal exposure prescription method reduces dose in radiography. Radiologic Technology. (2018) ; 89: (5): 435-40. |
[8] | Sherer MAS, Visconti PJ, Ritenour ER, Haynes K. Radiation Protection in Medical Radiography-E-Book: Elsevier Health Sciences; (2014) . |
[9] | Schauer DA, Linton OW. National Council on Radiation Protection and Measurements report shows substantial medical exposure increase. Radiological Society of North America, Inc.; (2009) . |
[10] | Tung C, Yang C, Yeh C, Chen T. Population dose from medical diagnostic exposure in Taiwan. Radiation Protection Dosimetry. (2011) ; 146: (1-3): 248-51. |
[11] | Asano T, Sato K, Onodera J-i. United nations scientific committee on the effects of atomic radiation 2000 report. Japanese Journal of Health Physics. (2001) ; 36: (2): 149-58. |
[12] | Colang JE, Killion JB, Vano E. Patient dose from CT: A literature review. Radiologic Technology. (2007) ; 79: (1): 17-26. |
[13] | Brenner DJ, Hall EJ. Computed tomography – an increasing source of radiation exposure. New England Journal of Medicine. (2007) ; 357: (22): 2277-84. |
[14] | Yu P-C, Ou H-Y, Tsang LL-C, Kung F-T, Hsu T-Y, Cheng Y-F. Prophylactic intraoperative uterine artery embolization to control hemorrhage in abnormal placentation during late gestation. Fertility and Sterility. (2009) ; 91: (5): 1951-5. |
[15] | Vedantham S, Goodwin SC, McLucas B, Mohr G. Uterine artery embolization: An underused method of controlling pelvic hemorrhage. American Journal of Obstetrics and Gynecology. (1997) ; 176: (4): 938-48. |
[16] | Nishizawa K, Masuda Y, Morinaga K, Suzuki S, Kikuyama S, Yoshida T, et al. Surface dose measurement in patients and physicians and effective dose estimation in patients during uterine artery embolisation. Radiation Protection Dosimetry. (2008) ; 128: (3): 343-50. |
[17] | Chiang H-W, Liu Y-L, Chen T-R, Chen C-L, Chiang H-J, Chao S-Y. Scattered radiation doses absorbed by technicians at different distances from X-ray exposure: Experiments on prosthesis. Bio-medical Materials and Engineering. (2015) ; 26: (s1): S1641-S50. |
[18] | Pauwels R, Beinsberger J, Collaert B, Theodorakou C, Rogers J, Walker A, et al. Effective dose range for dental cone beam computed tomography scanners. European Journal of Radiology. (2012) ; 81: (2): 267-71. |
[19] | Petoussi-Henss N, Bolch W, Eckerman K, Endo A, Hertel N, Hunt J, et al. Conversion coefficients for radiological protection quantities for external radiation exposures. Annals of the ICRP. (2010) ; 40: (2-5): 1-257. |
[20] | Scuderi GJ, Brusovanik GV, Campbell DR, Henry RP, Kwon B, Vaccaro AR. Evaluation of non-lead-based protective radiological material in spinal surgery. The Spine Journal. (2006) ; 6: (5): 577-82. |