Comparison of gait patterns in elderly fallers and non-fallers
Abstract
Gait is associated with an important risk factor of falls in the elderly. It is important to find differences of quantitative gait variables between fallers and non-fallers. The aim of this study was to investigate gait patterns in elderly fallers and non-fallers. Thirty-eight fallers and 38 non-fallers of similar age and height participated in this study. Subjects walked across the GaitRite walkway at self-selected comfortable speeds. Spatio-temporal gait variables were measured to characterize gait patterns. Kinetic variables were derived from normalized vertical ground reaction force (GRF). Independent t-tests were performed to compare the fallers with the non-fallers. The fallers walked more slowly with shorter steps and more variable step times than the non-fallers (
1.Introduction
One-third of the elderly living in communities experience falls at least once a year [1, 2, 3]. The falls are serious problems that lead to injuries, such as hip fractures, and even death [4, 5, 6]. The quality of life for most elderly fallers has deteriorated because the activities of daily living have become restricted [7, 8]. Moreover, falls are the major cause of between 0.85% and 1.5% of national health care expenditure [9]. Therefore, the high cost of interventions necessitates identifying and targeting potential fallers [4].
An age-related decline in the neuromuscular skeletal system can affect gait control in the elderly [10]. Many studies have found age-related changes in gait patterns in the elderly. Some studies have demonstrated that the elderly showed slower gait velocity and cadence than the young [11, 12]. Also, the elderly have decreased stride length and single-leg support time than the young [11, 12]. Moreover, Prince et al. have suggested that the elderly have a gait strategy to maintain their dynamic balance by increased time spent in double-limb support while decreasing gait speed and taking shorter steps [13].
Gait dysfunction is an important risk factor of falls [14, 15]. Up to 70% of the falls by the elderly occur during walking [16]. Gait training is important for prevention of falls in the elderly [17]. Gait assessment is recommended in current fall guidelines [17]; it can also help rehabilitation and fall interventions. It is especially important to find quantitative gait variables that distinguish the elderly who are at risk for falls during walking from those who are not. Although some attempts have been made to characterize gait patterns of the elderly faller, previous studies were limited by having no age- and height-matched control groups [18]; so it is still unclear if quantitative gait variables can help to identify potential fallers.
Ground reaction force (GRF), which can measure braking and propulsive forces during gait, is a summation of forces produced by all body segments [19]. The GRF has been used to diagnose gait abnormality. Increases in magnitude and variability of the peaks of GRF during the weight-acceptance and push-off phases are assumed to be found in people with unstable locomotion [19, 20]. Recently, altered gait patterns during advancing phases of pregnancy were demonstrated by a significant decrease in vertical GRF of maximal weight acceptance [21]. However, the GRF of elderly fallers has not been explored to date. GRF may be relevant and informative for the investigation of fall-related differences in gait strategy.
Many quantitative variables have been measured to evaluate fall risk. Spatio-temporal gait variables and GRF variables were usually obtained from walkway mats embedded with pressure sensors [22]. Also, a force plate can measure GRF and body center of pressure (COP) [21, 23, 24]. Recently, maximum Lyapunov exponent, velocity of COP, sum vector, and signal magnitude area (SMA) were derived from acceleration signals using an inertia measurement unit (IMU) sensor for the evaluation of fall risk factors [25, 26, 27]. Although many quantitative variables have been validated, we still need an investigation of gait patterns in elderly fallers.
This study measured spatio-temporal gait variables and GRF variables in order to identify differences of gait patterns between the elderly fallers and non-fallers. Therefore, the aim of this study is to investigate differences between spatio-temporal and kinetic gait patterns among elderly fallers and non-fallers.
2.Methods
2.1Subjects
Seventy-eight elderly subjects (38 fallers and 38 non-fallers) participated in this study with written informed consent. The faller group included 10 men and 28 women who had a history of at least one fall in the previous year. Of the subjects, 25 had fallen once, 8 had fallen twice, and 5 had fallen three times or more.
Table 1
Subject characteristics
Characteristics | Faller ( | Nonfaller ( | Statistical significance |
---|---|---|---|
Mean (SD) | Mean (SD) | P-value | |
Age [years] | 74.8 (5.7) | 74.5 (5.0) | 0.682 |
Height [cm] | 154.3 (8.0) | 154.8 (8.9) | 0.749 |
Weight [kg] | 59.7 (7.9) | 57.7 (11.2) | 0.339 |
BMI [kg/m | 25.1 (2.5) | 24.3 (3.1) | 0.188 |
BFR [%] | 33.0 (7.4) | 31.0 (7.8) | 0.196 |
All subjects were recruited from elderly communities in Seoul city, Korea. Subject characteristics of the two groups are summarized in Table 1. Subjects who could not walk independently without assistance devices and those with any diseases that affected their physical activity (e.g., musculoskeletal disease, neurological disease, and cardiovascular disorders) were excluded. Approval was obtained for this study from the ethics committee of an institutional review board.
Height, weight, and body mass index (BMI) were measured using an electronic height and weight measurement system (SH-9600A, Sewoo system, Korea). Additionally, body fat ratio (BFR) was measured using a body fat measurement device (Inbody 4.0, Biospace, Korea). All subjects were asked to restrict alcohol and caffeine intake for two hours before the measurement.
2.2Experiments and outcome measures
Gait was measured using a GaitRite (CIR Systems Inc. Clifton, NJ, USA), a 427 cm long portable walkway mat embedded with an active pressure sensor area that was 366 cm long and 61 cm wide. The GaitRite system has been validated against a motion analysis system [28] and has been assessed as having excellent test-retest reliability for the elderly [29]. All subjects walked on the GaitRite after warming up with some stretches for about three minutes. Each subject was then instructed to walk straight for 8 m at his or her self-selected walking speed. Subjects started walking from a point 2 m before the start of the mat and stopped at a point 2 m past the mat for exclusion of the first and last few steps of each trial. Three trials were recorded for each subject. The average of the three was used for subsequent analysis.
Figure 1.
Definition of temporal gait variables during gait cycle.
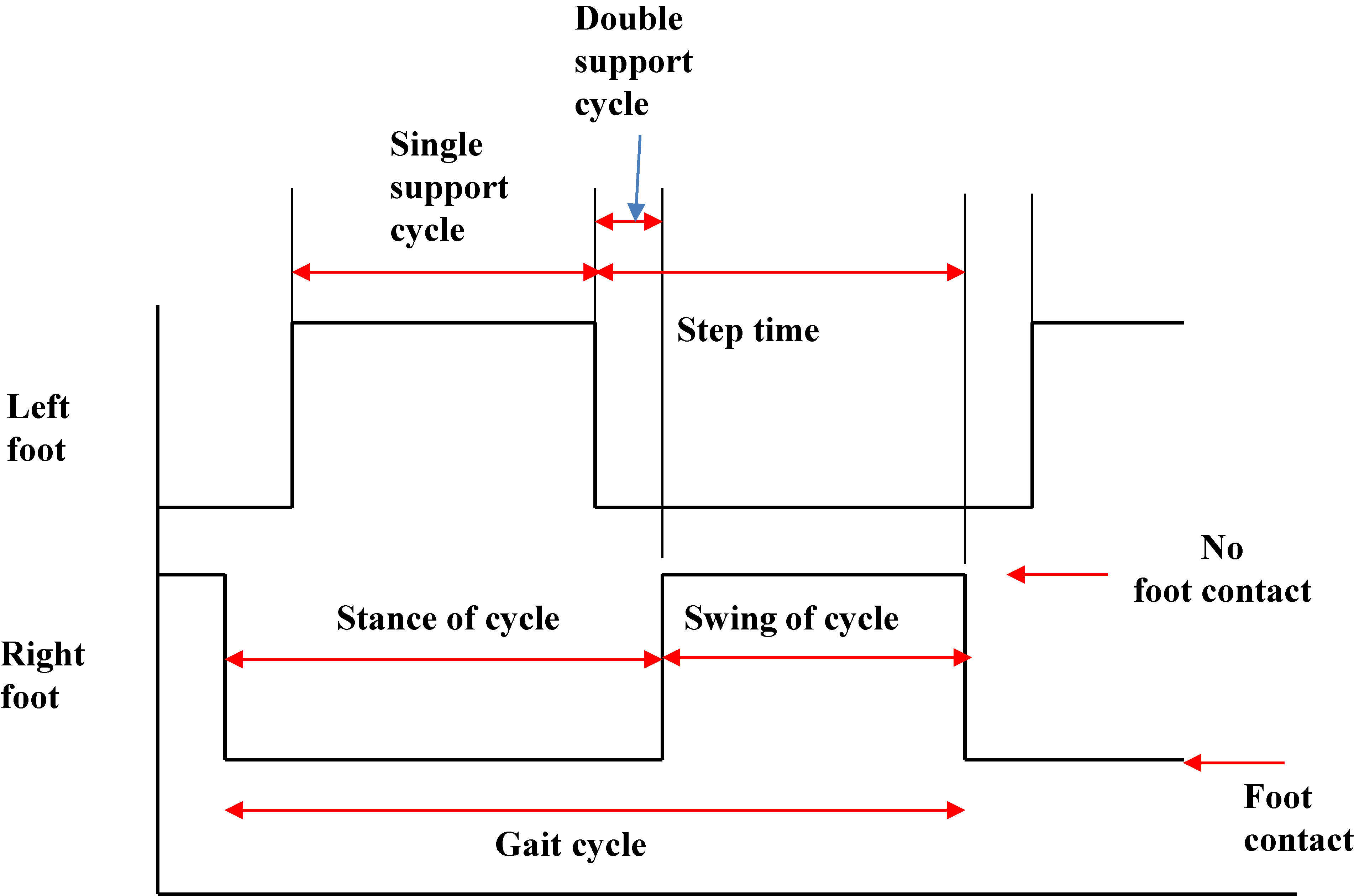
As temporal gait variables, gait velocity, swing of cycle, stance of cycle, single-limb support cycle, double-limb support cycle, step time, and variability of step time were derived from commercial GaitRite software as shown in Fig. 1. Also, spatial variables, such as step length, variability of step length, and toe in/out angle, were selected. Step length was defined as length from the previous heel center to the current center on the opposite foot. Toe in/out angle was defined as the angle between the line of progression and the midline of the footprint. Additionally, kinetic variables were derived from vertical GRF, as shown in Fig. 2. Peak force value in maximal weight acceptance (MWA), mid-stance (MS) and push-off phase (PO) were calculated by a self-developed analysis algorithm. The following variables were calculated from the peak force values: time to reach MWA (TMWA), time to reach MS (TMS), and time to reach PO (TPO). All GRF variables were calculated after normalization by body mass 24. Independent t-tests were performed to compare fallers and non-fallers. Statistical analysis was performed using SPSS ver.16 for Windows (SPSS Inc., Chicago, IL, USA). Differences between the groups were considered statistically significance at
Table 2
Descriptive statistics of spatio-temporal gait variables in fallers and non-fallers groups
Gait variables | Faller | Non-faller | Statistical significance | ||
---|---|---|---|---|---|
Mean (SD) | Mean (SD) | P-value | |||
Temporal variables | |||||
Gait velocity [cm/s] | 100.5 | (15.7) | 108.5 | (16.6) | 0.035 |
Swing of cycle [%] | 36.6 | (1.9) | 37.7 | (1.6) | 0.013 |
Stance of cycle [%] | 63.4 | (1.9) | 62.4 | (1.6) | 0.011 |
Single support cycle [%] | 36.9 | (2.0) | 37.5 | (1.7) | 0.186 |
Double support cycle [%] | 26.6 | (3.2) | 24.5 | (2.9) | 0.003 |
Step time [s] | 0.55 | (0.06) | 0.53 | (0.06) | 0.325 |
Variability of step time [s] | 0.04 | (0.04) | 0.02 | (0.02) | 0.015 |
Spatial variables | |||||
Step length [cm] | 54.2 | (7.2) | 57.3 | (7.3) | 0.040 |
Variability of step length [cm] | 2.6 | (1.6) | 2.7 | (1.6) | 0.918 |
Toe in/out angle | 8.0 | (6.3) | 6.5 | (6.9) | 0.051 |
Figure 2.
Representative gait variables from the vertical GRF. FMWA, peak force in maximal weight acceptance; FMS, peak force in mid stance; FPO, peak force in push-off; TMWA, time to reach FMWA; TMS, time to reach MS; TPO, time to reach push-off.
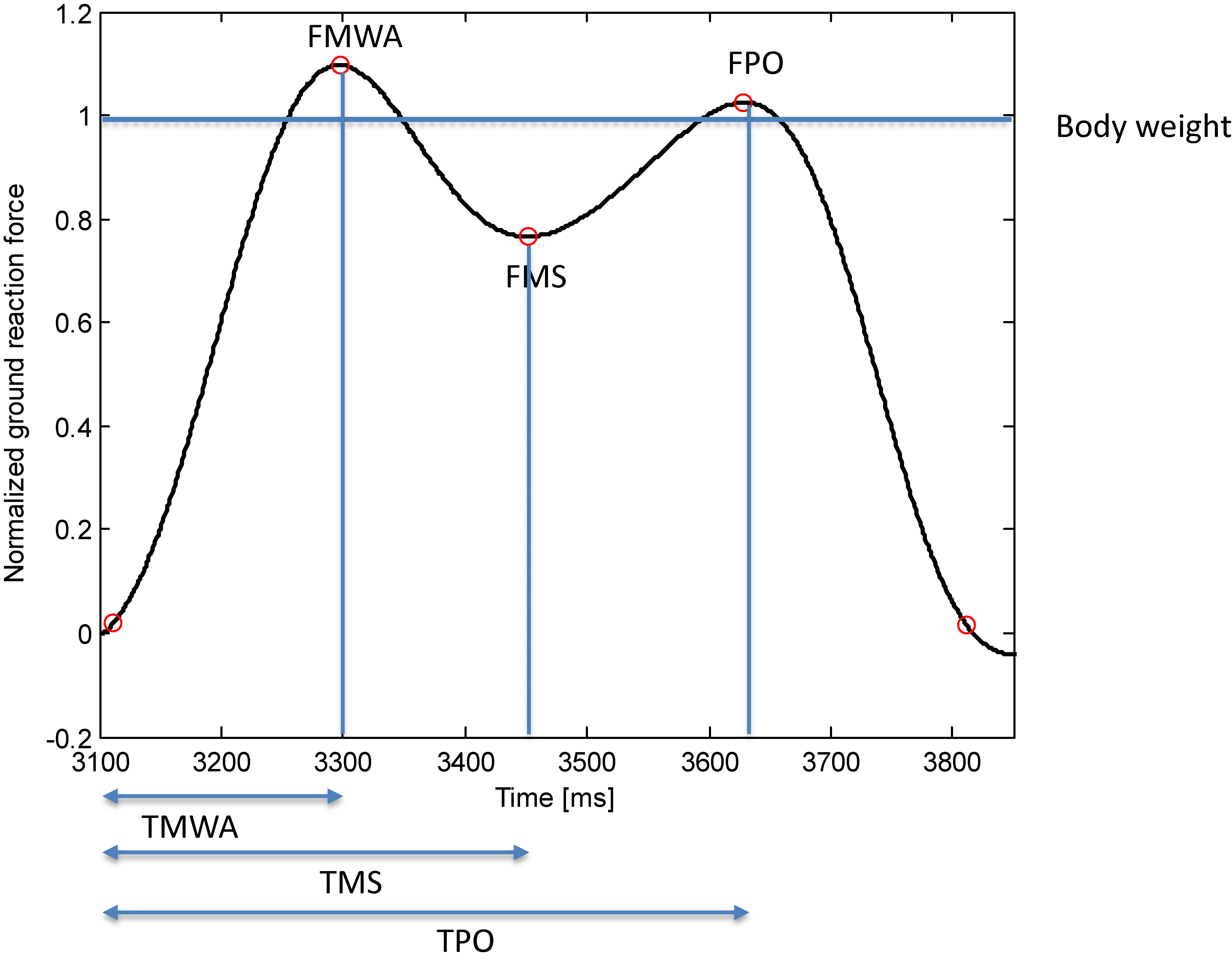
Figure 3.
Significant difference between fallers and non-fallers in spatio-temporal variables (
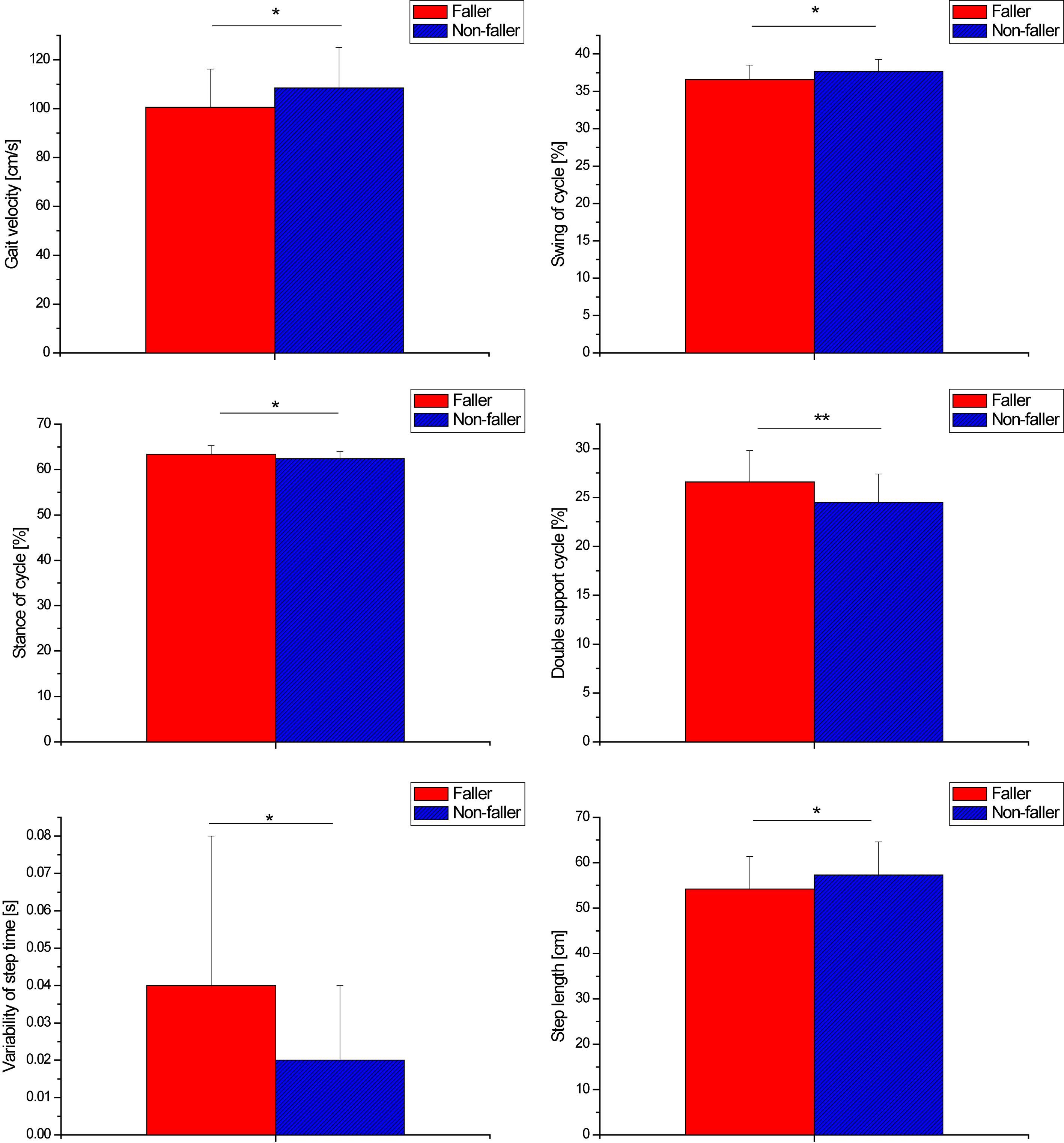
3.Results
Descriptive statics of subject characteristics are summarized in Table 1. There were no significant differences between the fallers and the non-fallers in all the variables. That is, those in the control (non-faller) group with comparable ages, heights, and other characteristics were well recruited in order to exclude age and anthropometric effects.
Table 3
Results of GRF gait variables in fallers and non-fallers group
GRF gait variables | Faller | Non-faller | Statistical significance |
---|---|---|---|
Mean (SD) | Mean (SD) | P-value | |
FMWA | 1.07 (0.10) | 1.07 (0.10) | 0.831 |
FMS | 0.82 (0.07) | 0.81 (0.09) | 0.363 |
FPO | 1.02 (0.08) | 1.01 (0.06) | 0.655 |
TMWA | 0.22 (0.07) | 0.19 (0.04) | 0.035 |
TMS | 0.37 (0.07) | 0.34 (0.05) | 0.021 |
TPO | 0.56 (0.07) | 0.55 (0.07) | 0.724 |
TD | 0.76 (0.09) | 0.75 (0.08) | 0.606 |
Figure 4.
Representative GRF in fallers and non-fallers groups.
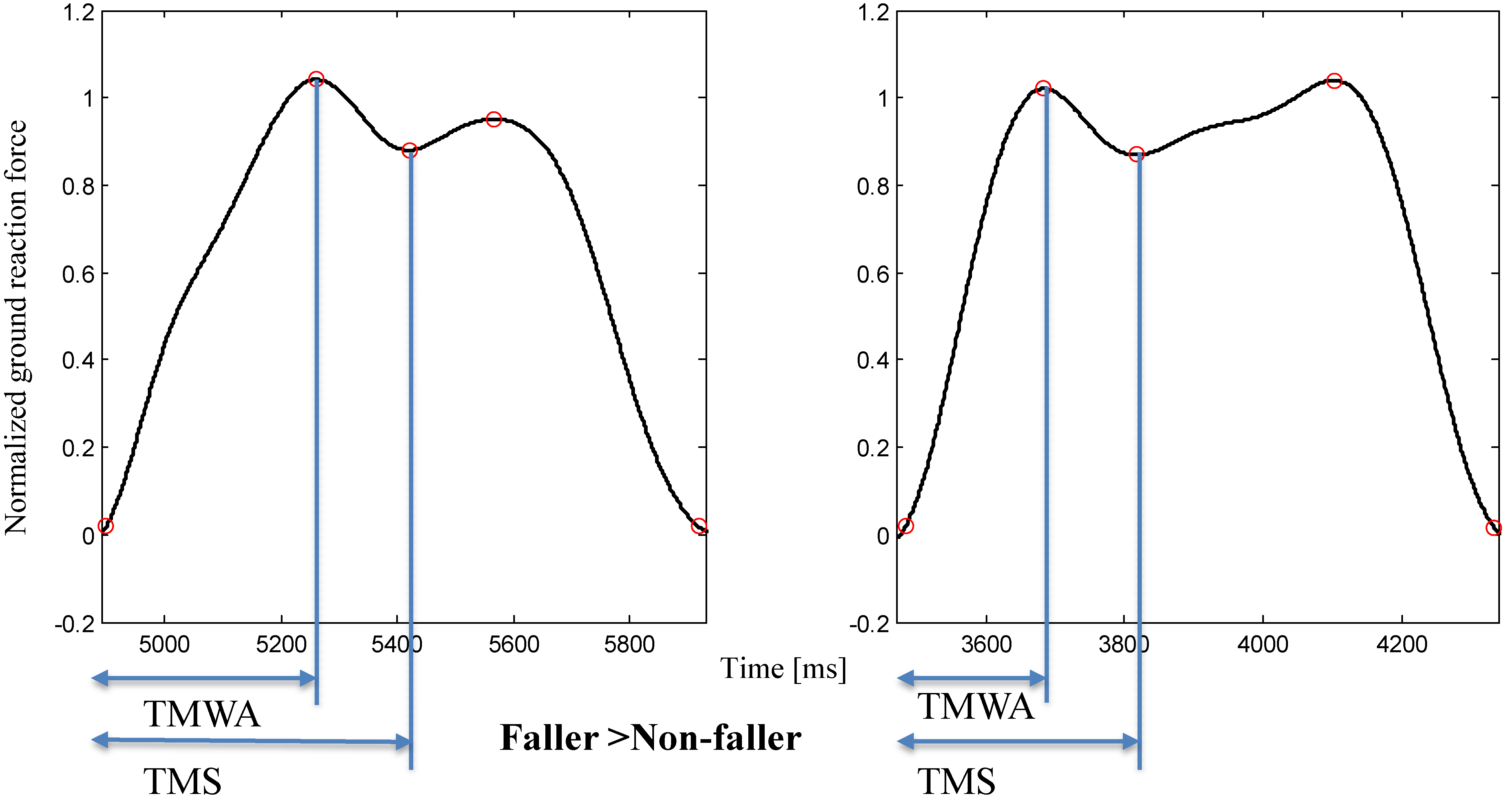
Figure 5.
Significant difference between fallers and non-fallers in GRF variables (
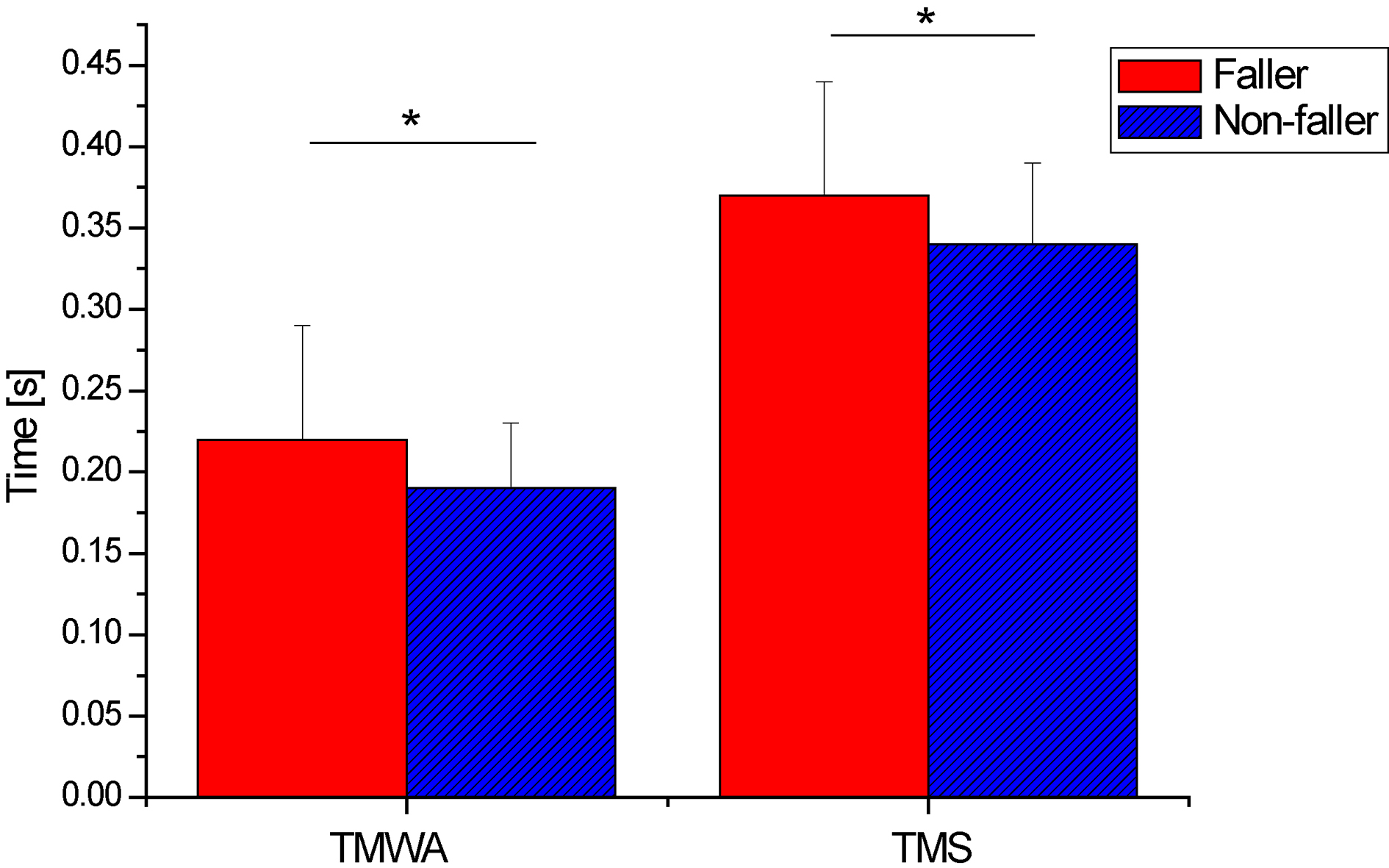
Table 2 shows the group differences in spatio-temporal gait variables. Also, Fig. 3 shows the results of significant group differences. Compared to the non-fallers, the fallers walked more slowly, with shorter steps (
Figure 4 shows representative comparison of GRF variables between fallers and non-fallers. The results for the GRF variables are shown in Table 3. Figure 5 shows the results of significant group differences among GRF variables. The TMWA and TMS of the fallers were significantly longer than those of the non-fallers (
4.Discussion
In this study, spatio-temporal gait variables and GRF gait variables were investigated in the elderly fallers as compared to non-fallers in control groups matched in age, gender, and anthropometry. Although they had similar characteristics, group differences were observed in specific gait variables. The results for each gait variable are discussed below.
Although the height of the fallers was similar to that of the non-fallers (Table 1), the fallers walked with shorter steps than the non-fallers (Table 2 and Fig. 3). The shorter steps may have resulted in reduced gait velocity. In fact, gait speed was strongly correlated with step length in this study (
By comparison with the non-fallers, the fallers exhibited a longer stance phase and a shorter swing phase (Table 2 and Fig. 3). That is, the fallers may maintain gait stability by increasing the stance phase. Increased total stance phase would be affected by the double-limb support cycle rather than the single limb-support cycle; i.e., the correlation coefficient between the stance cycle and the double-support cycle was
Although there was no significant difference in the variability of step length, temporal variability (variability of step time) for the fallers was significantly greater than for the non-fallers (Table 2 and Fig. 3). The fallers walked with a more irregular gait rhythm than the non-fallers. The temporal variability has been regarded as a reliable gait variable in the rhythmic stepping mechanism, depending on the highest levels of gait control [31]. Moreover, it has been reported that increased temporal gait variability reflects inefficient gait control and unstable gait in the elderly [32]. Blin et al. reported that variable gait rhythm may be related to inability to generate muscle force at a constant level [33]. In this study, the more variable gait rhythm of the fallers may be associated with lack of the ability to generate identical muscle force in repetitive gait cycles. In a previous study, age-related change was not observed in the gait variability, even though the elderly walk slower than the young [34]. This study demonstrated that a fall-related difference exists in the temporal gait variability. The variability of step time could be an important indicator for distinguishing the prospective fallers from the non-fallers.
The vertical GRF has been regarded as a representative measure for gait analysis [35]. The GRF variables were associated with many gait variables and functional performance [36]. Many studies have used the vertical GRF in gait analysis. McCrory et al. have examined differences between pregnant fallers and non-fallers in GRF variables [24]. A decrease in GRF of maximal weight acceptance has been demonstrated in advanced phases of pregnancy [21]. Also, an increase in time variables, e.g., TMWA and TMS, may be a protective mechanism against overloading the contact area of the foot despite the increase in body mass [21]. In addition, the vertical GRF is affected by aging and provides correlative information about the ability of the elderly to walk. Specifically, the elderly exhibited lower GRF at the maximal weight acceptance and push-off phases, and a higher minimum value at mid-stance, than the young [37]. Although some studies have analyzed gait GRF variables, the GRF pattern of the elderly fallers has not been explored to date. This study demonstrated that the fallers showed longer TMWA and TMS than the non-fallers. That is, the fallers have a long contact time at the loading response and mid-stance phases. The fallers may have a gait strategy with longer initial stance time, which may be associated with increased double-limb stance. Indeed, double-limb support time was significantly correlated with TMWA (
Recently, IMU sensors have been used for the evaluation of fall risk, such as gait and postural stability in construction or iron workers. Jebelli et al. demonstrated the usefulness of nonlinear dynamics, such as the maximum Lyapunov exponent, which was derived from acceleration signals, for the evaluation of gait stability in construction workers [25]. Moreover, the usefulness of velocity of center of pressure and magnitude of acceleration were validated for fall risk [26]. Yang et al. used sum vector magnitude (SVM) and signal magnitude area (SMA) of acceleration signals to detect near-miss falls of iron workers [27]. These variables may also be useful for distinguishing fallers from non-fallers in the elderly. Our study focused on only spatio-temporal and GRF variables. As further study, consideration of the IMU variables together with our variables would yield more information on gait analysis of elderly fallers.
In summary, this study demonstrated that (1) the elderly fallers walked slower with shorter steps, and more irregular steps than non-fallers; (2) the elderly fallers had a longer stance phase because of increased double-limb stance; (3) the elderly fallers walked longer in the loading response and mid-stance phases. These results will contribute to distinguishing prospective fallers from non-fallers, and the understanding of gait strategies of the elderly fallers may lead to effective interventions to prevent falls in the elderly.
Acknowledgments
This research was supported by Basic Science Research Program through the National Research Foundation of Korea (NRF) funded by the Ministry of Education and Ministry of Science (2015R1D1A1A01060411, 2016R1A6A3A11930880).
Conflict of interest
None to report.
References
[1] | Campbell AJ, Reinken J, Allan BC, Martinez GS. Falls in old age: a study of frequency and related clinical factors. Age Ageing. (1981) Nov; 10: (4): 264-70. |
[2] | Kojima S, Furuna T, Ikeda N, Nakamura M, Sawada Y. Falls among community-dwelling elderly people of Hokkaido, Japan. Geriatr Gerontol Int. (2008) Dec; 8: (4): 272-7. |
[3] | Prudham D, Evans JG. Factors associated with falls in the elderly: a community study. Age Ageing. (1981) Aug; 10: (3): 141-6. |
[4] | Hill K, Schwarz J, Flicker L, Carroll S. Falls among healthy, community-dwelling, older women: a prospective study of frequency, circumstances, consequences and prediction accuracy. Aust N Z J Public Health. (1999) Feb; 23: (1): 41-8. |
[5] | Kannus P, Parkkari J, Koskinen S, Niemi S, Palvanen M, Jarvinen M, et al. Fall-induced injuries and deaths among older adults. JAMA. (1999) May 26; 281: (20): 1895-9. |
[6] | Parkkari J, Kannus P, Palvanen M, Natri A, Vainio J, Aho H, et al. Majority of hip fractures occur as a result of a fall and impact on the greater trochanter of the femur: a prospective controlled hip fracture study with 206 consecutive patients. Calcif Tissue Int. (1999) Sep; 65: (3): 183-7. |
[7] | Gibson M, Andres R, Kennedy T, Coppard L. The prevention of falls in later life. A report of the Kellogg International Work Group on the Prevention of Falls by the Elderly. Dan Med Bull. (1987) Apr; 34: (Suppl 4): 1-24. |
[8] | Masud T, Morris RO. Epidemiology of falls. Age Ageing. (2001) Nov; 30: (Suppl 4): 3-7. |
[9] | Heinrich S, Rapp K, Rissmann U, Becker C, Konig HH. Cost of falls in old age: a systematic review. Osteoporos Int. (2010) Jun; 21: (6): 891-902. |
[10] | Morley JE. Mobility performance: a high-tech test for geriatricians. J Gerontol A Biol Sci Med Sci. (2003) Aug; 58: (8): 712-4. |
[11] | Bohannon RW, Andrews AW, Thomas MW. Walking speed: reference values and correlates for older adults. J Orthop Sports Phys Ther. (1996) Aug; 24: (2): 86-90. |
[12] | Feltner ME, MacRae PG, McNitt-Gray JL. Quantitative gait assessment as a predictor of prospective and retrospective falls in community-dwelling older women. Arch Phys Med Rehabil. (1994) Apr; 75: (4): 447-53. |
[13] | Prince F, Corriveau H, Hebert R, Winter DA. Gait in the elderly. Gait and Posture. (1997) ; 5: : 128-35. |
[14] | Campbell AJ, Borrie MJ, Spears GF. Risk factors for falls in a community-based prospective study of people 70 years and older. J Gerontol. (1989) Jul; 44: (4): M112-7. |
[15] | Rubenstein LZ, Robbins AS, Schulman BL, Rosado J, Osterweil D, Josephson KR. Falls and instability in the elderly. J Am Geriatr Soc. (1988) Mar; 36: (3): 266-78. |
[16] | Norton R, Campbell AJ, Lee-Joe T, Robinson E, Butler M. Circumstances of falls resulting in hip fractures among older people. J Am Geriatr Soc. (1997) Sep; 45: (9): 1108-12. |
[17] | Ganz DA, Bao Y, Shekelle PG, Rubenstein LZ. Will my patient fall? JAMA. (2007) Jan 3; 297: (1): 77-86. |
[18] | Auvinet B, Berrut G, Touzard C, Moutel L, Collet N, Chaleil D, et al. Gait Abnormalities in Elderly Fallers. Journal of Aging and Physical Activity. (2003) ; 11: : 40-52. |
[19] | Hollman JH, Brey RH, Bang TJ, Kaufman KR. Does walking in a virtual environment induce unstable gait? An examination of vertical ground reaction forces. Gait Posture. (2007) Jul; 26: (2): 289-94. |
[20] | Giakas G, Baltzopoulos V. Time and frequency domain analysis of ground reaction forces during walking: An investigation of variability and symmetry. Gait & Posture. (1997) ; 5: : 189-97. |
[21] | Gimunová M, Kasović M, Zvonař M, Turřínek P, Matković B, Ventruba P, et al. Analysis of ground reaction force in gait during different phases of pregnancy. Kinesiology. (2015) ; 47: : 236-41. |
[22] | Verghese J, Holtzer R, Lipton RB, Wang C. Quantitative gait markers and incident fall risk in older adults. J Gerontol A Biol Sci Med Sci. (2009) Aug; 64: (8): 896-901. |
[23] | Kim JW, Eom GM, Kim CS, Kim DH, Lee JH, Park BK, et al. Sex differences in the postural sway characteristics of young and elderly subjects during quiet natural standing. Geriatr Gerontol Int. (2010) Apr; 10: (2): 191-8. |
[24] | McCrory JL, Chambers AJ, Daftary A, Redfern MS. Ground reaction forces during gait in pregnant fallers and non-fallers. Gait Posture. (2011) Oct; 34: (4): 524-8. |
[25] | Jebelli H, Ahn CR, Stentz TL. The validation of gait-stability metrics to assess construction workers’ fall risk. Computing in Civil and Building Engineering 2014. (2014) . |
[26] | Jebelli H, Ahn CR, Stentz TL. Fall risk analysis of construction workers using inertial measurement units: Validating the usefulness of the postural stability metrics in construction. Safety Science. (2016) ; 84: : 161-70. |
[27] | Yang K, Jebelli H, Ahn CR, Vuran MC. Threshold-based approach to detect near-miss falls of iron workers using inertial measurement units 2015. (2015) . |
[28] | Webster KE, Wittwer JE, Feller JA. Validity of the GAITRite walkway system for the measurement of averaged and individual step parameters of gait. Gait Posture. (2005) Dec; 22: (4): 317-21. |
[29] | Menz HB, Latt MD, Tiedemann A, Mun San Kwan M, Lord SR. Reliability of the GAITRite walkway system for the quantification of temporo-spatial parameters of gait in young and older people. Gait Posture. (2004) Aug; 20: (1): 20-5. |
[30] | Winter DA, Patla AE, Frank JS, Walt SE. Biomechanical walking pattern changes in the fit and healthy elderly. Phys Ther. (1990) Jun; 70: (6): 340-7. |
[31] | Beauchet O, Annweiler C, Lecordroch Y, Allali G, Dubost V, Herrmann FR, et al. Walking speed-related changes in stride time variability: effects of decreased speed. J Neuroeng Rehabil. (2009) Aug 5; 6: : 32. |
[32] | Herman T, Giladi N, Gurevich T, Hausdorff JM. Gait instability and fractal dynamics of older adults with a “cautious” gait: why do certain older adults walk fearfully? Gait Posture. (2005) Feb; 21: (2): 178-85. |
[33] | Blin O, Ferrandez AM, Pailhous J, Serratrice G. Dopa-sensitive and dopa-resistant gait parameters in Parkinson’s disease. J Neurol Sci. (1991) May; 103: (1): 51-4. |
[34] | Hausdorff JM, Mitchell SL, Firtion R, Peng CK, Cudkowicz ME, Wei JY, et al. Altered fractal dynamics of gait: reduced stride-interval correlations with aging and Huntington’s disease. J Appl Physiol (1985). (1997) Jan; 82: (1): 262-9. |
[35] | Jacobs NA, Skorecki J, Charnley J. Analysis of the vertical component of force in normal and pathological gait. J Biomech. (1972) Jan; 5: (1): 11-34. |
[36] | Nilsson J, Thorstensson A. Ground reaction forces at different speeds of human walking and running. Acta Physiol Scand. (1989) Jun; 136: (2): 217-27. |
[37] | Yamada T, Maie K. The characteristics of walking in old men analyzed from the ground reaction force. J Anthrop Soc. (1988) ; 96: : 7-15. |
[38] | Martin PE, Marsh AP. Step length and frequency effects on ground reaction forces during walking. J Biomech. (1992) Oct; 25: (10): 1237-9. |