Skin anti-aging and wound healing effects of a passion fruit seed extract rich in piceatannol
Abstract
BACKGROUND:
UVB radiation triggers skin photoaging by inducing excess cellular oxidants, contributing to senescence, and overproducing matrix metalloproteinase-1 (MMP-1), causing skin wrinkles. Senescence also impedes skin wound closure. Passion fruit seeds (PFS) boast abundant piceatannol (PCT), recognized for its antioxidant and anti-aging properties.
OBJECTIVE:
To study potential of PFS extract in diminishing photoaging, accelerating wound healing, and enhancing SIRT1 production in human keratinocytes (HaCaT cells).
METHODS:
The PFS extract was assessed for PCT using HPLC analysis. The antioxidant effects, reduced senescence-associated β-galactosidase (SA-β-gal) activity, and MMP-1 production were measured in HaCaT cells exposed to UVB using a DCFA-DA assay, SA-β-gal activity, and flow cytometry/immunofluorescent including MMP-1 mRNA expression, respectively. The effect of wound healing acceleration was evaluated, including EGFR mRNA expression. Additionally, SIRT1 enhancement was investigated using flow cytometry and immunofluorescent techniques, which also included the assessment of SIRT1 mRNA expression.
RESULTS:
The PFS extract, which is rich in PCT, effectively diminished cellular oxidants and aging. It decreased UVB-induced cellular oxidants, senescence, and MMP-1 production at both protein and mRNA levels. Moreover, the extract enhanced wound closure by boosting cell migration and increasing EGFR mRNA expression. Additionally, SIRT1 production and expression, associated with cellular rejuvenation, increased in extract-treated cells.
CONCLUSIONS:
PFS extract exhibits potential for skincare and wound healing applications, offering a natural strategy to combat skin aging effects and support tissue repair.
1Introduction
UVB radiation causes skin photoaging by penetrating the outer layers of the skin and generating reactive oxygen species (ROS) in the keratinocytes. These ROS then trigger cellular senescence and the production of collagen degradation enzymes through cellular pathways, resulting in the loss of skin structure and increased vulnerability to wrinkles, sagging, and other signs of aging [1]. Cellular senescence refers to the gradual decline of physiological function and proliferative capacity of cells. It can be affected by external stressors such as exposure to UVB radiation or chemicals, which activate cellular pathways including p53 and p16 pathways in response to DNA damage and oxidative stress. These pathways can lead to cell cycle arrest and cellular senescence [2, 3]. Excessive ROS production stimulates the production of matrix metalloproteinase-1 (MMP-1), an enzyme responsible for the degradation of collagen fibers. ROS activates transcription and translation of MMP-1 protein through the mitogen-activated protein kinase (MAPK) pathway. The overproduction of MMP-1 results in the degradation of collagen fibers, which manifests as skin wrinkles [4]. The process of wound healing consists of four phases: hemostasis, inflammation, proliferation, and remodeling [5]. During the proliferation phase, re-epithelialization relies on the migration and proliferation of a large number of cells. One of the factors that influences this process is the epidermal growth factor (EGF) that interacts with the epidermal growth factor receptor (EGFR) and plays a pivotal role in the proliferation phase by activating the migration and proliferation of keratinocytes and fibroblasts, leading to the healing and closure of the wound [6]. However, the senescence of skin cells can lower the expression of EGFR, which can result in slower wound healing [7].
To combat senescence and collagen degradation caused by UVB irradiation, affected cells undergo a process of spontaneous elimination of ROS [8]. One common way to reduce ROS levels is through the production of enzymes that neutralize oxidants, such as superoxide dismutase (SOD) and glutathione peroxidase (GSH-PX), which are regulated by SIRT1 [9]. SIRT1 is a member of the family of mammalian sirtuins and a histone deacetylase of nicotinamide adenine dinucleotide (NAD+). It is reported to enhance cellular lifespan and is considered as a longevity enzyme. Furthermore, SIRT1 promotes cell proliferation by inhibiting p53 activity through deacetylation [10]. However, natural cellular responses to reduce ROS levels are often insufficient and thus, the use of antioxidant substances is crucial for maintaining cellular health [11]. Previous studies have reported that passion fruit seed (PFS) contains a phytochemical called piceatannol (PCT), which belongs to the phenolic group and has potential health benefits, including antioxidant, anti-cancer, anti-hyperpigmentation, and anti-aging properties [12–14]. While some previous studies have shown that PCT can increase the production of SIRT1 in human liver cells and monocytic cell lines [15, 16], the effects of PFS extract on the reduction of UVB-induced cellular senescence, MMP-1 production, enhancement of SIRT1 production, and wound healing in human keratinocytes are still limited. Therefore, this study aims to investigate the effects of PFS extract riches in PCT on the reduction of cellular senescence and MMP-1 production induced by UVB irradiation, as well as the enhancement of SIRT1 expression and wound healing in human keratinocytes.
2Materials and methods
2.1Passion fruit seed (PFS) extract
PFS extract was obtained as the method described by Chalortham et al. [17]. Dried and milled PFS (50 g) was extracted in 60% ethanol (500 mL) at room temperature for 5 days with agitation. The resulting mixture was filtered and passed through the SP825 column. The flow-through was then partitioned with ethyl acetate, and the ethyl acetate fraction was evaporated and dried in a hot air oven (60°C, overnight). Finally, the PFS extract was stored at –20°C until use.
2.2Identification of PCT
PFS extracts was investigated to identify the presence of PCT by using the HPLC system (Waters 2695 equipped with Waters 2487 Dual λ Absorbance Detector, Waters, Milford, MA, USA) with the result compared against the standard PCT (TCI, Tokyo, Japan) concentration at 100 μg/mL. The dried powder of PFS extracts (125 mg) was dissolved in 1 mL of 60% ethanol followed by filtration through a 0.22 μm PES filter. The mobile phase of HPLC system comprised of deionized water (A) and HPLC grade acetonitrile (B) at a ratio of (A):(B) = 90:10% in the initiation period. Separation was carried out by isocratic elution at a ratio of (A):(B) at 75:25% at a temperature of 45°C. The flow rate was set to 0.8 mL/min with the injection volume at 5 μL. The HPLC C18 column (250×4.6 mm, i.d. 5 μm, ACE, Scottland) was employed as a stationary phase. The UV spectra was detected at 320 nm [17].
2.3Cell culture and PFS extract preparation
Human keratinocytes (HaCaT) (CLS ® 300493) were cultured in high glucose DMEM (Sigma, USA) supplemented with 10% FBS, 1% penicillin/streptomycin (Gibco, USA), and 3.7 mg/ml of sodium carbonate (Sigma, USA). The cells were incubated in 5% CO2 at 37°C humidified incubator as manufacturers’ recommendations. The dried powder of PFS extracts (125 mg) was dissolved in 1 mL of 60% ethanol and then filtered through a 0.22 μm PES filter. The resulting stock solution was subsequently diluted with culture media to achieve the desired final concentration before treatment with the cells.
2.4Cell viability
HaCaT cells were seeded in 96-well plates at a density of 1.5×104 cells/well and incubated in culture media. The cells were then treated with different concentrations of PFS extract or kojic acid (Sigma, USA) for 24 h. After incubation, the cells were washed with phosphate buffer saline (PBS) and 0.5 mg/mL of tetrazolium dye methylthiotetrazole (MTT) solution was added (100 μL/well), followed by a 2 h incubation period at 37°C. The solution was removed, and 100 μL of pure dimethyl sulfoxide (DMSO) was added to each well. The cells were agitated, and the absorbance was measured using an automatic microplate reader (Emax Plus, Molecular Devices, USA) at a wavelength of 570 nm. The absorbance values of the treated cells were compared to those of the control cells to determine the percentage of viable cells. Additionally, the non-lethal intensity of UVB radiation was determined by exposing the cells to various intensities of UVB radiation and evaluating them 24 h after irradiation using a UVB lamp (Phillips, The Netherland) [18].
2.5Cellular oxidants
To determine the effect of PFS extract on anti-cellular oxidants that are induced by UVB radiation, 2,7 dichlorohydrofluorescein diacetate (DCFH-DA) assay was performed as reported previously [19]. Briefly, 1.5×104 cells/well of HaCaT cells were seeded in black 96 well plates followed with 24 h incubation. Culture media was removed then, washed once with PBS, and the cells were exposed under UVB radiation at 100 mJ/cm2 followed with the addition of 200 μL of the test agents then, incubated for 24 h. The supernatant was removed, and PBS was used for washing followed with addition of 200 μL of 10 μM DCFH-DA (Sigma, USA) solution for 1 h. The fluorescence intensity of 2,7-dichlorofluorescein (DCF), which is a product of the cellular oxidation process of DCFH-DA, was measured using a fluorescent microplate reader (TECAN Spark, USA) with excitation/emission wavelengths of 485/535 nm, respectively. The results of cellular oxidants were presented as a percentage relative to the DCF level in unirradiated cells. Kojic acid (Sigma, USA) was used as a positive control at 1 mM.
2.6Senescence associated β-galactosidase
Senescence of HaCaT cell was assessed by modifying the SA-β-galactosidase protocol described previously [20]. 5×105 HaCaT cells were seeded in 6-well plates and maintained for 24 h. Culture medium was aspirated, and the cells were exposed to UVB radiation at an intensity of 100 mJ/cm2. The cells were treated with PFS extract for 48 h (daily treatment). After treatments, the cells were washed with PBS and fixed with a fixative solution (0.2% glutaraldehyde + 2% formaldehyde in PBS) at room temperature for 5 min. The fixed cells were then washed twice with PBS and incubated with 1 mg/mL X-gal staining solution (Sigma, USA) for 16 h in a dark 37°C incubator. After incubation, the cells were observed under an inverted microscope (Olympus, Japan) and the percentage of SA-β-gal-positive cells was calculated and compared to that of the untreated cells. Kojic acid (Sigma, USA) was used as a positive control.
2.7Flow cytometry
Flow cytometric analysis was performed to determine the percentage of MMP-1 and SIRT1 positive cells. To measure MMP-1 levels, HaCaT cells (1×106) were cultured in 6-well plates for 24 h. The cells were then exposed to UVB radiation (100 mJ/cm2) and treated with PFS extract for 24 h. To measure SIRT1 levels, HaCaT cells (1×106) were cultured in 6-well plates for 24 h and then treated with PFS extract for 6 h. After treatment, each cell group was trypsinized, fixed with 4% paraformaldehyde, and permeabilized with 0.25% Triton X-100. The cells were then incubated individually with primary antibodies, including MMP-1 monoclonal primary antibody (Santa Cruz, USA) at a concentration of 1:500 for 2 h, and SIRT1 monoclonal primary antibody (Santa Cruz, USA) at a concentration of 1:500 for 2 h. The secondary antibody, Alexa Fluro ® 488 (Abcam, USA), at a concentration of 1:1000, was then incubated for 15 min. The cells were washed with PBS and resuspended with 1% paraformaldehyde prior to analysis using a flow cytometer (FACScan, Becton Dickinson, USA) [21].
2.8Immunofluorescent staining
Immunofluorescent staining was used to evaluate the presence of MMP-1 and SIRT1. To relatively evaluate MMP-1 expression levels, HaCaT cells (5×103) were cultured on a cover slip for 24 h, exposed to UVB and treated with PFS extract for 2 h. For SIRT1 expression levels, HaCaT cells (5×103) were cultured on a cover slip for 24 h and treated with PFS extract for 6 h. After the treatment, the cells were washed with cold PBS and fixed with cold methanol for 45 min. The fixed cells were then washed and permeabilized with a 0.1% Triton X-100 solution for 15 min. The cells were blocked with 1% BSA for 1 h and washed with PBS. MMP-1 or SIRT1 monoclonal primary antibodies (Santa Cruz, USA) were separately incubated with the fixed cells at a concentration of 1:500 for 2 h, followed by the addition of secondary antibodies, including Alexa Fluor 594 ® for detecting MMP-1, and Alexa Fluor 488 ® (Abcam, USA) for detecting SIRT1. Nuclear staining was performed by adding Hoechst-33342 and washing thoroughly. The cells were then observed under a fluorescent microscope (Olympus, Japan) and recorded. The intensity of MMP-1 or SIRT1 within the cells was analyzed, and the percentages were determined using Image J software [22].
2.9Measurement of wound healing
To investigate the wound healing acceleration effect of PFS extract, the wound gap was measured after treatment. HaCaT cells (1×106 cells/well) were cultured in 6-well plates until reaching 90% confluence. The cells were washed with PBS and then treated with 10 μM of mitomycin C (Roche, Germany) for 1 h to inhibit proliferation. A scratched wound was created using a 200 μL pipette tip, and PFS extract was added to the cells at desired concentrations in 0.5% FBS supplemented DMEM for treatment. The wounds were treated daily for 48 h, and the healing progression was recorded at three time points: right after scratching (T0), after 24 h of the first treatment (T24), and after 24 h of the second treatment (T48). The gaps of the wounds were measured using Image J software and compared relatively between T0 and T24 or T48 in each treatment group. The results of wound closure were presented as a percentage compared to those of the untreated cells, and kojic acid (1 mM) was used as a positive control [23].
2.10Measurement of mRNA expression
To determine the effect of PFS extract on MMP-1, SIRT1, and EGFR mRNA expression, treated cells were harvested and total RNA was extracted after PFS treatment. For MMP-1, HaCaT cells (1×106 cells/well) were cultured and maintained for 24 h. The cells were then exposed to UVB radiation at 100 mJ/cm2, followed by treatment with PFS extract for 24 h. For SIRT1, HaCaT cells (1×106 cells/well) were cultured and maintained for 24 h and treated with PFS extract for 6 h. For EGFR, the cells were incubated with 10 μM of mitomycin C, followed by wounding. The wounds were then treated with PFS extract for 24 h. After incubation, total RNA was extracted using an RNA extraction kit (Invitrogen, USA), following the manufacturer’s instructions. The total RNA of each condition was measured using a spectrophotometer (Thermo Scientific, USA), and the concentrations were normalized to 100 ng/μL. The normalized total RNA solutions were then converted to cDNA using a ReverstraAce RT-PCR conversion kit (Toyobo, Japan), following the manufacturer’s protocol. qPCR was performed using i-Taq SYBR green RT-PCR master mix (Bio-Rad, USA) and a real-time PCR machine (Thermo Fisher Scientific, Germany). MMP-1, SIRT1, and EGFR were used as target genes, and GAPDH was used as an internal control. The following primers were used as shown in Table 1.
Table 1
Primers used for amplification of the target genes
Genes | Forward (5’-3’) | Reverse (5’-3’) |
MMP-1 | GCTCATGAACTCGGCCATTC | TGGACAGGATTTTGGGAACG |
SIRT1 | GCCTCACATGCAAGCTCTAGTGAC | TTCGAGGATCTGTGCCAATCATAA |
EGFR | TGCGTCTCTTGCCGGAAT | GGCTCACCCTCCAGAAGGTT |
GAPDH | TCATCAATGGAAATCCCATCAACC | TGGACTCCACGACGTACTCAGC |
PCR parameter was set as 95°C, 30 s for denaturation, 40 cycles of denaturation 95°C for 30 s, annealing 55°C for 30 s and extension 72°C for 20 s. The quantity of the amplified mRNA was calculated the 2–ΔΔCt values compared to the internal control GAPDH [24].
2.11Statistical analysis
All experiments were performed in biological triplicates. The results are presented in mean±S.D. Analyses were performed with one-way ANOVA using PASW Statistics 18 software (IBM, USA). Values of p≤0.05 were considered significant.
3Results
3.1PFS extract contained PCT and it reduced UVB-induced cellular oxidant levels, resulting in the attenuation of cellular senescence
Previous studies reported that PFS extract contains stilbene derivatives such as scirpusin B, resveratrol and large amount of PCT hence, PFS extract was investigated for the presence of PCT through HPLC analysis. The chromatogram of the extract displayed a prominent peak of PCT, which was detected at approximately 16.1 minutes. Notably, this retention time matched that of the standard PCT (Fig. 1A). UVB radiation induces various cellular modifications including the generation of cellular oxidants. However, overexposure to UVB radiation can cause cell death. Therefore, we assessed the non-lethal intensity of radiation as well as the non-lethal concentration of PFS extract compared to the untreated cells. We found that the highest intensity of UVB radiation that did not affect cell viability was 100 mJ/cm2. PFS extract showed the highest concentration at 100 μg/mL and kojic acid (KA) was used as a control (Fig. 1B). HaCaT cells were cultured and exposed to UVB radiation (100 mJ/cm2) followed by treatment with PFS extract. We observed that UVB radiation increased cellular 2,7-dichlorofluorescein (DCF) levels, which are indicative of cellular oxidation of DCFH-DA. However, treatment with PFS extract resulted in a dose-dependent reduction of DCF levels. Notably, at a concentration of 100 μg/mL, PFS extract exhibited a significant decrease in DCF levels compared to the UVB-irradiated cells (p≤0.05, Fig. 1C). To investigate cellular senescence, we examined the activity of senescence-associated β-galactosidase (SA-β-gal). We found that UVB radiation significantly increased the number of SA-β-gal positive cells compared to the untreated (UT) cells (p≤0.01). However, treatment with PFS extract resulted in a dose-dependent reduction in the percentage of SA-β-gal positive cells. Notably, the treatment with 100 μg/mL of PFS extract showed a marked reduction in SA-β-gal positive cells compared to the UVB-irradiated cells (p≤0.01, Fig. 1D, E). In this experiment, a positive control was used in the form of 1 mM of kojic acid (KA).
Fig. 1
HPLC fingerprint of piceatannol (PCT) contained in passion fruit seed (PFS) extract and its safety and efficacy of PFS extract in reducing UVB-induced cellular oxidant and senescence. (A) The chromatogram of PCT in the PFS extract displayed a peak detected at 16.1 minutes, which was comparable to the peak of the standard PCT, also observed at 16.1 minutes. (B) non-lethal intensities of UVB radiation, concentrations of PFS extract and Kojic acid (KA) which were observed by MTT assay. (C) Cellular oxidant reductive effect of PFS extract which was evaluated by observing the cellular DCF levels via performing DCFH-DA assay in UVB-induced HaCaT cells. (D) Reductive effect of PFS extract on the senescence-associated β-galactosidase (SA-β-gal) in UVB-induced HaCaT cells. The cells were recorded under a microscope at 400x magnification with the light blue stain indicating the SA-β-gal positive cells. (D) The percentage of SA-β-gal positive cells against all cells in the same visual field as a bar graph. The results are manifested as mean±SD. *, ** statistical significance at p≤0.05 and p≤0.01, respectively. a compared to UT, b compared to UVB radiated cells. UT; untreated cells, KA; kojic acid (1 mM) which served as positive control.
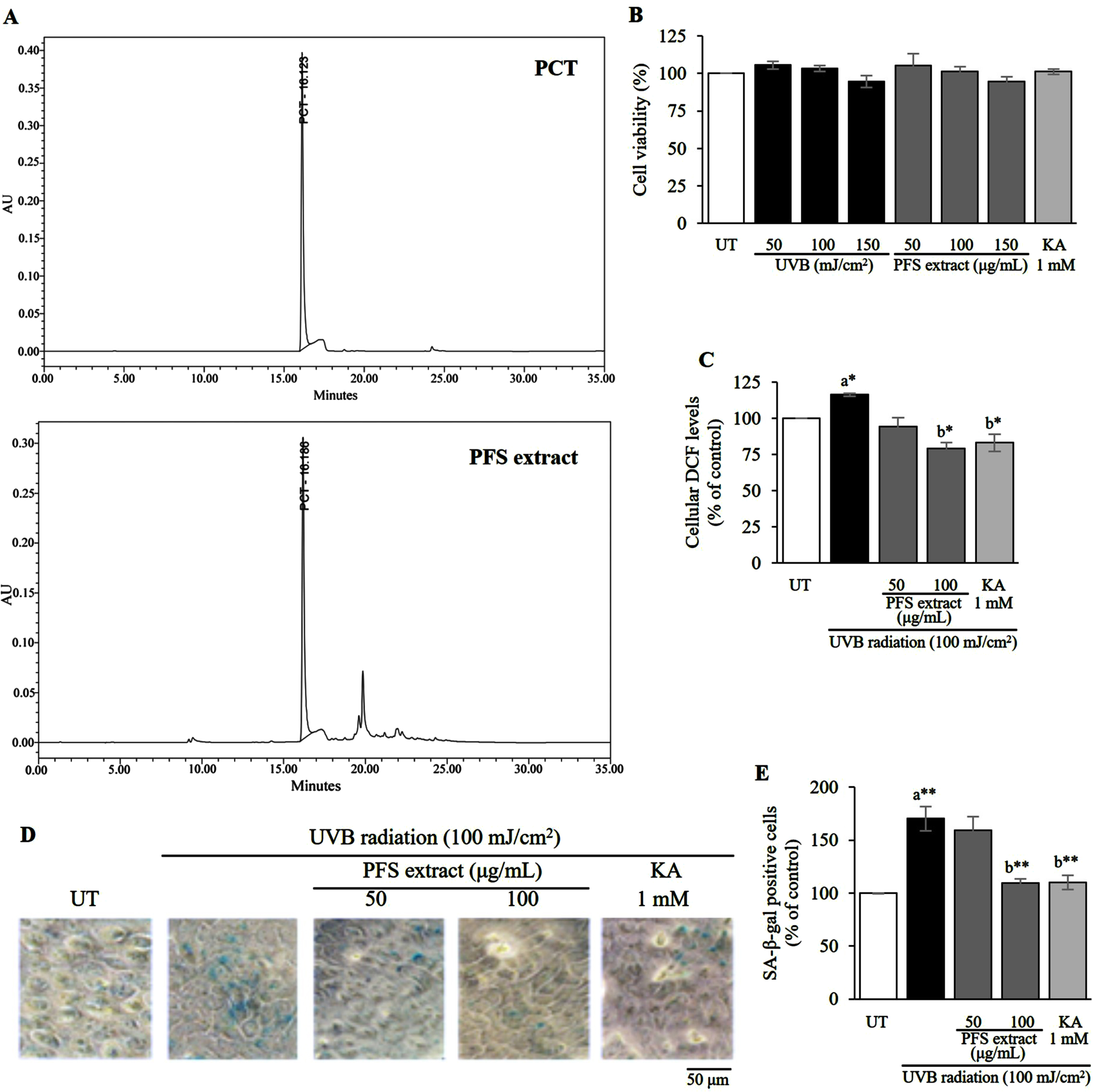
3.2PFS extract reduced UVB-induced matrix metalloproteinase-1 (MMP-1) production and level
An excessive cellular reactive oxygen species (ROS) that is induced by UVB radiation causes the overproduction of MMP-1 and results in skin wrinkles. To investigate the MMP-1 inhibitory effect of PFS extract, we investigated the relative quantitation of MMP-1 induced by UVB irradiation in HaCaT cells using flow cytometry (relative quantitation), immunofluorescence staining (localization), and mRNA expression analysis (relative quantitation). Flow cytometric analysis revealed that the percentage of cells with positive MMP-1 protein at the cells membrane significantly increased in UVB-irradiated cells compared to untreated cells (UT) (p≤0.001). Treatment with PFS extract at a concentration of 100 μg/mL markedly decreased the number of MMP-1-positive cells compared to UVB-irradiated cells (Fig. 2A, B). The criteria for cells with MMP-1-positive was derived from the calibrations and settings of the blank. The percentage of MMP-1-positive cells was derived from the sum of the right upper and right lower quadrants in the histogram. Immunofluorescence staining showed that MMP-1 was highly expressed and localized in the cytoplasmic area of the UVB-exposed cells, however the leveler was reduced when treated with the extract (Fig. 2C). The intensity of MMP-1 in each treatment condition was analyzed and evaluated. Cells exposed to UVB showed a significantly higher intensity of MMP-1 compared to UT (p≤0.001), and treatment with PFS extract at a concentration of 100 μg/mL significantly lowered the intensity compared to UVB-irradiated cells (p≤0.05) (Fig. 2D). Furthermore, we investigated the inhibitory effect of the extract at mRNA level. PFS extract reduced MMP-1 mRNA expression in a similar trend to that revealed by flow cytometry and immunofluorescence staining (Fig. 2E). Therefore, PFS extract lowered UVB-induced MMP-1 production by reducing mRNA expression. Kojic acid (KA, 1 mM) was used as a positive control.
Fig. 2
PFS extract affects negatively the level of MMP-1 after UVB induction. (A) Flow cytometric analysis indicates the percentage of UVB-induced MMP-1 positive cells. (B) MMP-1 positive cells in each treatment group were calculated, and the results are presented as mean±SD relative to the control. (C) Immunofluorescence staining image of UVB-exposed HaCaT cells with anti-MMP-1 (red) and the nuclei were counterstained with Hoechst-33342 (blue), scale = 50 μm. (D) The intensity of MMP-1 in each treatment group was analyzed, calculated and expressed as a bar graph compared to the untreated cells, and the results are presented as mean±SD. (E) The expression of MMP-1 mRNA in each treatment group was calculated using the 2–ΔΔCt values and expressed as a bar graph relative to the untreated cells. The results are presented as mean±SD. *, **, *** Statistical significance at p≤0.05, p≤0.01, and p≤0.001, respectively. a compared to UT, b compared to UVB radiated cells. UT; untreated cells, KA; kojic acid (1 mM) served as positive control.
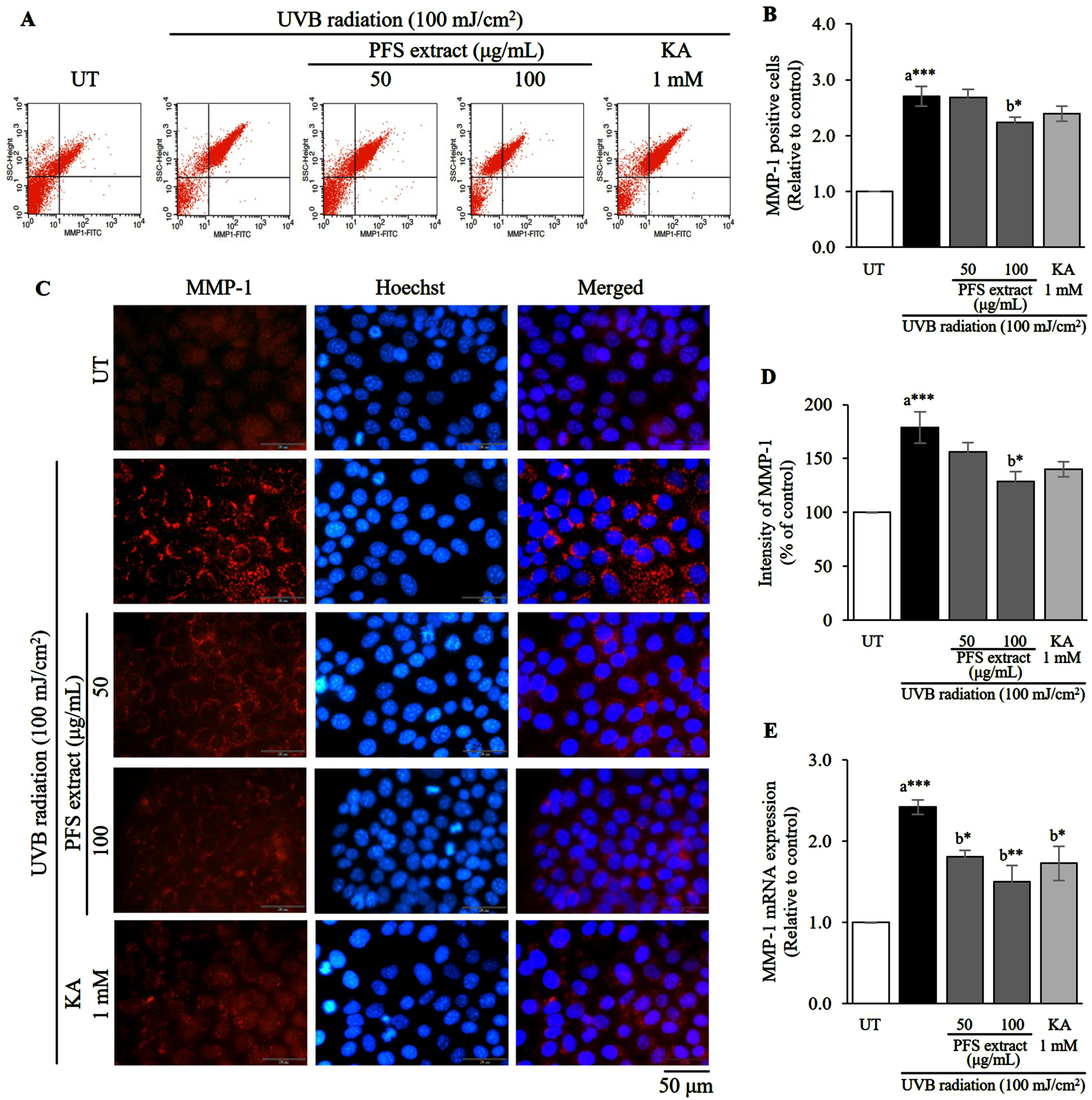
3.3PFS extract induced EGFR and promoted the wound-healing
This study aimed to examine the potential of PFS extract in expediting the closure of wounds. HaCaT cells were utilized to observe cell migration, wherein they were treated with 10 μM mitomycin C to halt proliferation and then subjected to scratching to simulate a wound. The findings revealed that treatment with the extract at concentrations of 50 and 100 μg/mL for 24 h moderately reduced the wound gap, showing a dose-dependent relationship. The most remarkable improvement in healing was observed when the cells were treated with 100 μg/mL of PFS extract for 48 h, resulting in a narrower wound gap compared to untreated cells (UT) (p≤0.05) (Fig. 3A, B). Since the expression of EGFR directly affects cell migration, the impact of PFS extract on promoting cell migration in wound closure was investigated. The results indicated that PFS extract stimulated the expression of EGFR mRNA in a similar pattern to wound healing, with significant effects observed at the 100 μg/mL treatment (p≤0.01) (Fig. 3C). Kojic acid (KA, 1 mM) was employed as a positive control. These findings suggest that the PFS extract facilitates cell migration by enhancing the expression of EGFR mRNA, thereby accelerating wound healing.
Fig. 3
Wound healing acceleration and EGFR mRNA expression enhancement effects of PFS extract. (A) The monolayer of HaCaT cells that was scratched to mimic a wound and treated daily with PFS extract (50 or 100 μg/mL). The wound gap was recorded after 0, 24, and 48 h after initiation of the wound. (B) The wound gap was analyzed by Image J software and calculated to the percentage by compared to the gap at 0 h in each treatment group. The per cent values were plotted in a line chart compared to the untreated cells (UT) at each time point (24 and 48 h) and the results are presented as mean±SD. (C) The expression of EGFR mRNA in each treatment group was calculated using the 2–ΔΔCt values and expressed as a bar graph relative to the untreated cells. The results are presented as mean±SD. *, ** Statistical significance at p≤0.05 and p≤0.01, respectively. a compared to UT. UT; untreated cells, KA; kojic acid (1 mM) served as positive control.
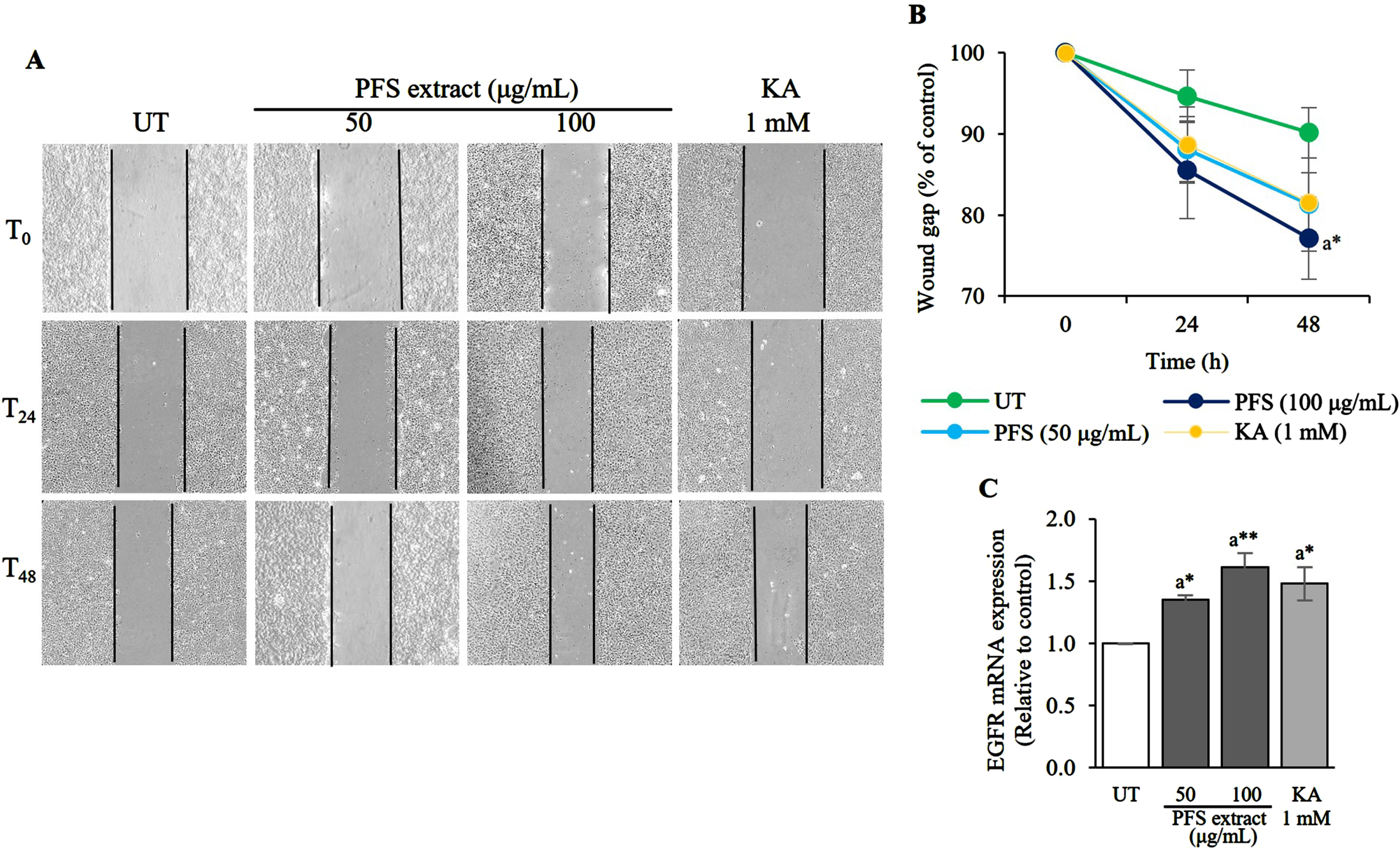
3.4PFS extract enhanced the level of SIRT1
Flow cytometry, immunofluorescence staining, and evaluation of SIRT1 mRNA expression were employed to assess the levels of SIRT1 in HaCaT cells treated with PFS extract. After 6 h of PFS treatment, the cells were collected and analyzed. The proportion of SIRT1 positive cells in the PFS extract treatment exhibited a dose-dependent increase and was significantly higher than that of the untreated cells (UT) (p≤0.01) (Fig. 4A, B). Additionally, the fluorescence intensity of SIRT1 was notably higher in the 100 μg/mL treatment group with p≤0.01 compared to the UT (Fig. 4C, D). Furthermore, the expression of SIRT1 mRNA was measured, and as expected, PFS extract increased the expression of SIRT1 mRNA, with the highest level observed in the 100 μg/mL treatment group (p≤0.01) compared to the UT (Fig. 4E). A positive control using 1 mM kojic acid was included. Overall, these findings indicate that the PFS extract enhances the synthesis of SIRT1 protein by stimulating its mRNA expression.
Fig. 4
SIRT1 enhancement effect of PFS extract. (A) Flow cytometric analysis indicates the percentage of SIRT positive cells. (B) SIRT1 positive cells in each treatment group was calculated, and the results are presented as mean±SD. (C) Immunofluorescence staining image of PFS-treated HaCaT cells with anti-SIRT1 (green) and the nuclei were counterstained with Hoechst-33342 (blue), scale = 50 μm. (D) The intensity of SIRT1 in each treatment group was analyzed, calculated and expressed as a bar graph compared to the untreated cells, and the results are presented as mean±SD. (E) The expression of SIRT1 mRNA in each treatment group was calculated using the 2–ΔΔCt values and expressed as a bar graph relative to the untreated cells. The results are presented as mean±SD. *, ** Statistical significance at p≤0.05 and p≤0.01, respectively. a compared to UT. UT; untreated cells, KA; kojic acid (1 mM) served as positive control.
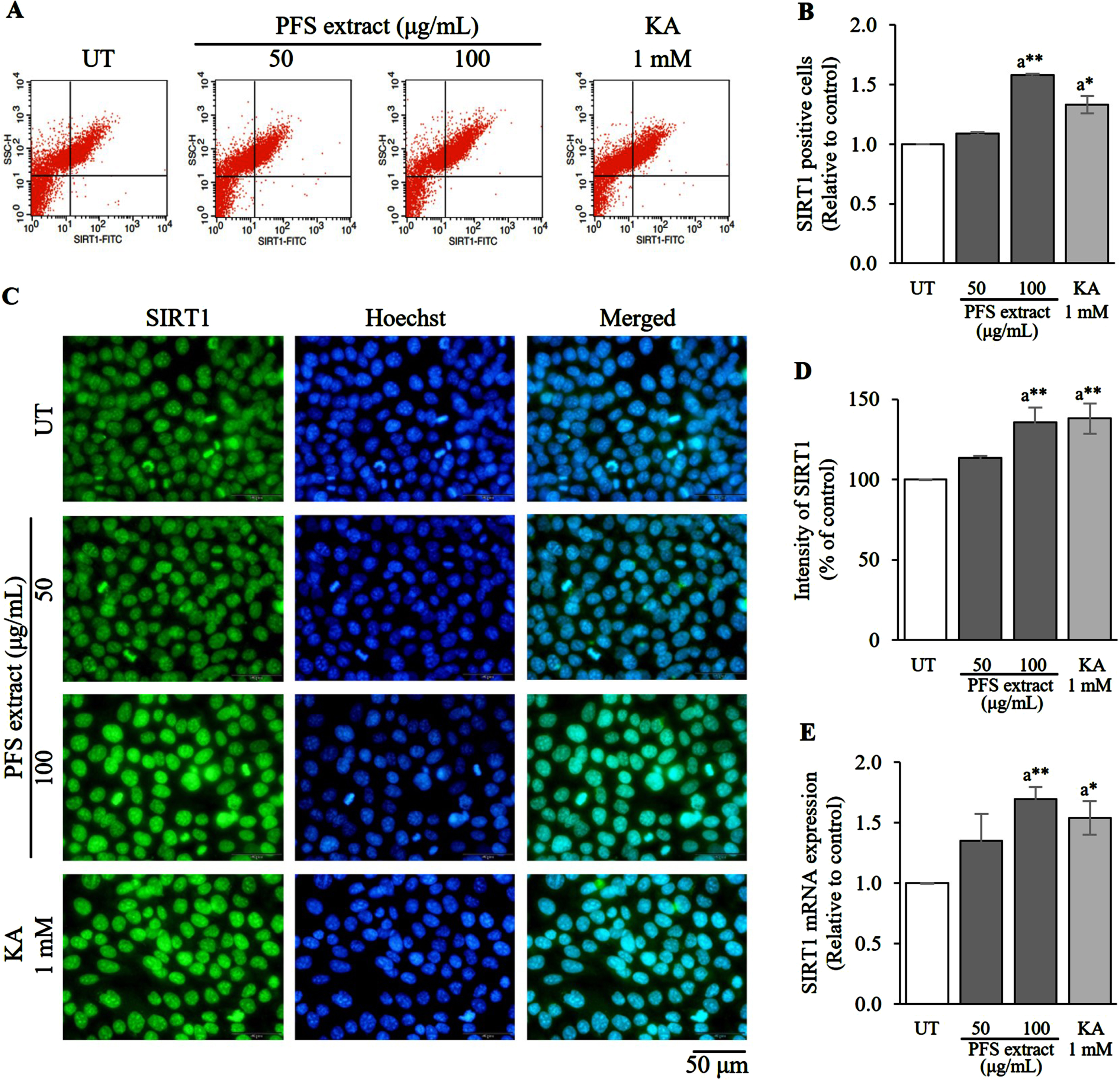
4Discussion
Naturally, skin cells undergo senescence over time, but exposure to UVB radiation speeds up this process by generating an excess of reactive oxygen species (ROS) [1]. While cells possess antioxidant enzymes to eliminate surplus ROS, when the cellular defense system fails to do so, it leads to accelerated aging and impaired cell functions [9, 11]. Senescence-associated β-galactosidase (SA-β-gal) is an enzyme used to indicate cellular aging, and its activity is higher in senescent cells due to increased expression of lysosomal β-galactosidase [25]. In this study, X-gal was used as a substrate to detect β-galactosidase activity, which hydrolyzes X-gal into monosaccharide, producing blue stains in cellular cytoplasmic areas [26]. Treatment with passion fruit seed (PFS) extract reduced X-gal conversion in a dose-dependent manner, significantly lower than that of UVB-irradiated cells. Additionally, PFS extract reduced UVB-induced cellular oxidants. Overall, PFS extract mitigated UVB-induced senescence in human keratinocytes by lowering cellular oxidants.
The underlying molecular mechanism responsible for the suppression of MMP1 in HaCaT cells in the presence of UVB radiation remains unclear. However, it has been demonstrated that the accumulation of ROS stimulates the production of collagen MMP1 [27]. Specifically, in various cellular systems, ROS triggers the activation of the MAPK pathway, leading to the phosphorylation of ERK and JNK. Consequently, this activation results in the stimulation of c-JUN and c-FOS, two transcription factors that induce the transcription of AP1, ultimately leading to MMP1 expression [28]. After conducting the investigation, it was observed that cells exposed to UVB irradiation exhibited a significantly higher level of MMP-1 compared to untreated cells. However, when treated with PFS extract, the MMP-1 level decreased in a dose-dependent manner. The maximum reduction in MMP-1 was observed at a concentration of 100 μg/ml of PFS extract. This reduction in MMP-1 can be attributed to the suppressive effect of PFS extract on cellular oxidants, along with its ability to attenuate UVB-induced MMP-1 levels in human keratinocytes by reducing cellular oxidants.
Delayed wound healing serves as an indicator of aged skin [29], and it can be evaluated in vitro. The process of wound healing relies on the proliferation and migration of cells. Cell migration is governed by various molecular processes, including the interaction between epidermal growth factor (EGF) and its receptor (EGFR). The activation of EGFR triggers the MAPK pathway, which is known to regulate cell migration [30]. When PFS extract was introduced, the wound healing process became more efficient, even though mitomycin C, an inhibitor of proliferation, was added. Additionally, PFS extract significantly induced the expression of EGFR. Taken together, these findings suggest that PFS extract promotes wound healing by activating EGFR.
It has been reported that the accumulation of ROS over time can shorten the cellular lifespan [31]. Silent information regulator 1 (SIRT1), a member of the mammalian sirtuin family and a nicotinamide adenine dinucleotide (NAD+)-dependent histone deacetylase, has been elucidated to enhance cellular ROS detoxification by regulating related enzymes such as superoxide dismutase (SOD) and glutathione peroxidase (GSH-PX), leading to an extension of cellular lifespan [9]. As mentioned earlier, the regulation of the MAPK pathway by EGFR is transient. However, it has been observed that EGFR can also be regulated in the long term through epigenetic modifications. One such factor is SIRT1. Treatment with PFS has been shown to induce the expression of SIRT1. In turn, SIRT1 is capable of deacetylating and activating SP1, a transcription factor that can bind to the promoter region of EGFR and promote its transcription. This mechanism has been reported in previous studies [32, 33]. Additionally, SIRT1 is known to downregulate the levels of miR-34a, which is a transcription factor that inhibits the translation of EGFR. Therefore, in the absence of miR-34a, the expression of EGFR is increased [34]. Collectively, the PFS extract possesses properties that have the potential to impact on aging and wound healing processes.
The quality of PFS extract can vary depending on various factors, including the growth conditions and genetics of the passion fruit. Therefore, it is crucial to identify the main components responsible for its actions, quantify their presence, and investigate potential chemical modifications for future applications. Another interesting study that should be conducted is to investigate the concentrations of PFS extract to address aging in a realistic skin model, as well as study the accumulation of the extract and/or the potent compound in cells and their metabolism. PFS extract has been reported to contain piceatannol (PCT), a structural analog of resveratrol with a similar chemical composition [12]. PCT possesses an additional hydroxyl (–OH) group attached to one of its phenol rings, making it a more potent antioxidant compared to resveratrol. This additional hydroxyl group allows PCT to donate its 4’-H atom to free radical molecules, thereby exhibiting stronger antioxidant properties. The structure of piceatannol can be found in Supplement 1 (Figure S1) [12].
Based on the presence of piceatannol, it is reasonable to suggest that PFS extract, containing PCT, has the potential to reduce cellular oxidants induced by UVB radiation. This reduction in cellular oxidants contributes to the attenuation of cellular senescence, suppression of MMP-1-mediated collagen degradation, and enhancement of EGFR expression, which is associated with cell migration and wound closure acceleration. These beneficial effects are likely linked to the increased expression and production of SIRT1, as mentioned previously.
In comparison to the positive control of kojic acid at a concentration of 1 mM used in this study, the efficacy of PFS extract in anti-aging and wound healing was more pronounced at a concentration of 100 μg/mL. It should be noted that higher concentrations of kojic acid resulted in cell lethality in HaCaT cells, as indicated in Supplement 1 (Figure S2). The findings of this study are significant as they highlight the potential value of utilizing a waste product, such as passion fruit seeds, which contain abundant piceatannol, as an agro-waste byproduct in the fruit juice industry.
Acknowledgments
Department of Pathobiology, Faculty of Science, Mahidol University, and Normal and Pathological Development of Melanocytes, Institut Curie provided reagents and instruments for this project.
Funding
This research was funded by Research and Researchers for Industries (RRI), Thailand Science Research and Innovation (TSRI) with cooperation of Detox Thailand Co., Ltd. (Grant number PHD 61I0034).
Author contributions
Conceptualization, P.S. and P.K.; methodology, P.K., W.P., V.P., and P.S.; formal analysis, P.K., W.P., and P.S.; investigation, P.K., W.P., V.P., and S.C.; resources, W.P., P.S., and L.L.; data curation, P.K. and P.S.; writing original draft preparation, P.K.; writing review and editing, W.P., V.P., L.L., and P.S.; visualization, P.K.; supervision, L.L., and P.S.; project administration, P.S.; funding acquisition, W.P., L.L., and P.S. All authors have read and agreed to the published version of the manuscript.
Conflict of interest
The authors declare no conflict of interest.
Supplementary material
[1] The supplementary material is available in the electronic version of this article: https://dx.doi.org/10.3233/NHA-231518.
References
[1] | Lephart ED . Skin aging and oxidative stress: Equol’s anti-aging effects via biochemical and molecular mechanisms. Ageing Res Rev. (2016) ;31: :36–54. |
[2] | Csekes E , Račková L . Skin aging, cellular senescence and natural polyphenols. Int J Mol Sci. (2021) ;22: (23):12641. doi: 10.3390/ijms222312641. |
[3] | Ho CY , Dreesen O . Faces of cellular senescence in skin aging. Mechanisms of Ageing and Development. (2012) ;198: :111525. doi: 10.1016/j.mad.2021.111525. |
[4] | Mesa-Arango AC , Flórez-Muñoz SV , Sanclemente G . Mechanisms of skin aging. Iatreia. (2017) ;30: (2):160–70. |
[5] | Gosain A , DiPietro LA . Aging and wound healing. World J Surg. (2004) ;28: :321–26. |
[6] | Boucher I , Kehasse A , Marcincin M , Rich C , Rahimi N , Trinkaus-Randall V . Distinct activation of epidermal growth factor receptor by UTP contributes to epithelial cell wound repair. Am J Pathol. (2011) ;178: (3):1092–105. |
[7] | Shiraha H , Gupta K , Drabik K , Wells A . Aging fibroblasts present reduced epidermal growth factor (EGF) responsiveness due to preferential loss of EGF receptors. J Biol Chem. (2000) ;275: (25):19343–51. |
[8] | Gillbro JM , Olsson MJ . The melanogenesis and mechanisms of skin-lightening agents – existing and new approaches. Int J Cos Sci. (2011) ;33: :210–21. |
[9] | Olmos Y , Sánchez-Gómez FJ , Wild B , García-Quintans N , Cabezudo S , Lamas S , Monsalve M . SirT1 regulation of antioxidant genes is dependent on the formation of a FoxO3a/PGC-1α complex. Antioxid Redox Signal. (2013) ;19: (13):1507–21. |
[10] | KEGG pathway database [homepage on the Internet] Homo sapiens (human). Longevity regulating pathway. [updated 2021 May 8; cited 2022 Dec 4]. Available from: https://www.genome.jp/pathway/hsa04211+3265. |
[11] | Poljsak B , Suput D , Irina Milisav I . Achieving the balance between ROS and antioxidants: When to use the synthetic antioxidant. Oxid Med Cell Longev. (2013) ;956792. doi: 10.1155/2013/956792. |
[12] | Rossi M , Caruso F , Opazo C , Salciccioli J . Crystal and molecular structure of piceatannol; scavenging features of resveratrol and piceatannol on hydroxyl and peroxyl radicals and docking with transthyretin. J Agric Food Chem. (2008) ;56: (22):10557–66. |
[13] | Talcott SP , Moore SP , Charity JC . Phytochemical composition and antioxidant stability of fortified yellow passion fruit (Passiflora edulis). J Agr Food Chem. (2003) ;51: :935–41. |
[14] | Ashikawa K , Majumdar S , Banerjee S , Bharti AC , Shishodia S , Aggarwal BB . Piceatannol inhibits TNF-induced NF-κB activation and NF-κB-mediated gene expression through suppression of IκBα kinase and p65 phosphorylation. J Immunol. (2002) ;169: (11):6490–7. |
[15] | Mahmoud Moustafa E , Rashed ER , Rashed RR , Omar NN . Piceatannol promotes hepatic and renal AMPK/SIRT1/PGC-1α mitochondrial pathway in rats exposed to reserpine or gamma-radiation. Int J Immunopathol Pharmacol. (2021) ;35: :20587384211016194. doi: 10.1177/20587384211016194. |
[16] | Kawakami S , Kinoshita Y , Maruki-Uchida H , Yanae K , Sai M , Ito T . Piceatannol and its metabolite, isorhapontigenin, induce SIRT1 expression in THP-1 human monocytic cell line. Nutrients. (2014) ;6: (11):4794–804. doi: 10.3390/nu6114794. |
[17] | Chalortham N , Povichit N , Kreawsa S , Moonsawat K , Yasamoot D , Jaisit N , Saefong C , Leepatanakul L , Pongtakam C , Na Lamphun J , Suwannalert P , Ezure Y . Comparison of piceatannol content in seed coat and embryo of passion fruit. Thai Bull Pharm Sci. (2019) ;14: (1):35–48. |
[18] | López-García J , Lehocký M , Humpolíček P , Sáha P . HaCaT keratinocytes response on antimicrobial atelocollagen substrates: Extent of cytotoxicity, cell viability and proliferation. J Funct Biomater. (2014) ;5: (2):43–57. doi: 10.3390/jfb5020043. |
[19] | Lee H , Yeom M , Shin S , Jeon K , Park D , Jung E . Protective effects of aqueous extract of Mentha suaveolens against oxidative stress-induced damages in human keratinocyte HaCaT cells. Evid Based Complement Alternat Med. (2019) ;2019: :5045491. doi: 10.1155/2019/5045491. |
[20] | Dimri GP , Lee X , Basile G , Acosta M , Scott G , Roskelley C , Medrano E E , Linskens M , Rubelj I , Pereira-Smith O . A biomarker that identifies senescent human cells in culture and in aging skin in vivo . PNAS. (1995) ;92: (20):9363–67. |
[21] | Oh JH , Lee JI , Karadeniz F , Park SY , Seo Y , Kong CS . Antiphotoaging effects of 3,5-dicaffeoyl-epi-quinic acid via inhibition of matrix metalloproteinases in UVB-irradiated human keratinocytes. Evid-based Complement Altern Med. (2020) ;8949272. doi: 10.1155/2020/8949272. |
[22] | Kim JH , Kang DJ , Bae JS , Lee JH , Jeon S , Choi HD , Kim N , Kim HG , Kim HR . Activation of matrix metalloproteinases and FoxO3a in HaCaT keratinocytes by radiofrequency electromagnetic field exposure. Sci Rep. (2021) ;11: (1):7680. doi: 10.1038/s41598-021-87263-2. |
[23] | Di Grazia A , Luca V , Segev-Zarko LA , Shai Y , Mangoni ML . Temporins A and B stimulate migration of HaCaT keratinocytes and kill intracellular Staphylococcus aureus . Antimicrob Agents Chemother. (2014) ;58: (5):2520–27. |
[24] | Dziadziuszko R , Witta SE , Cappuzzo F , Park SJ , Tanaka K , Danenberg PV , Baron AE , Crino L , Franklin WA , Bunn PAJ , Varella-Garcia M , Danenberg KD , Hirsch FR . Epidermal growth factor receptor messenger RNA expression, gene dosage, and gefitinib sensitivity in non-small cell lung cancer. Clin Cancer Res. (2006) ;12: (10):3078–84. |
[25] | Dimri GP , Lee X , Basile G , Acosta M , Scott G , Roskelley C , Medrano E E , Linskens M , Rubelj I , Pereira-Smith O . A biomarker that identifies senescent human cells in culture and in aging skin in vivo . PNAS. (1995) ;92: (20):9363–67. |
[26] | Yang J , Liu M , Hong D , Zeng M , Zhang X . The paradoxical role of cellular senescence in cancer. Front Cell Dev Biol. (2021) ;9: :722205. doi: 10.3389/fcell.2021.722205. |
[27] | Xiao ZB , Yang ST , Chen JL , Li CY , Zhou CX , Hong PZ , Sun SL , Qian ZJ . Trehalose against UVB-induced skin photoaging by suppressing MMP expression and enhancing procollagen I synthesis in HaCaT cells. Journal of Functional Foods. (2020) ;74: :104198. |
[28] | Merecz-Sadowska A , Sitarek P , Kucharska E , Kowalczyk T , Zajdel K , Cegliński T , Zajdel R . Antioxidant properties of plant-derived phenolic compounds and their effect on skin fibroblast cells. Antioxidants. (2021) ;10. 10.3390/antiox10050726. |
[29] | Khalid KA , Nawi AFM , Zulkifli N , Barkat MA , Hadi H . Aging and wound healing of the skin: A review of clinical and pathophysiological hallmarks. Life (Basel). (2022) ;12: (12):2142. doi: 10.3390/life12122142. |
[30] | Association for Diagnostics & Laboratory Medicine [homepage on the Internet]. Mutations in the EGFR pathway-clinical utility and testing strategies. [updated 2013 Oct 1; cited 2022 Dec 4].Available from: https://www.aacc.org/cln/articles/2013/october/egfr-mutations. |
[31] | Amano H , Chaudhury A , Rodriguez-Aguayo C , Lu L , Akhanov V , Catic A , Popov YV , Verdin E , Johnson H , Stossi F , Sinclair DA , Ogiso EN , Berestein GL , Chang JT , Neilson JR , Meeker A , Finegold M , Baur JA , Sahin E . Telomere dysfunction induces sirtuin repression that drives telomere dependent disease. Cell Metabolism. (2019) ;29: (6):1274–90. |
[32] | Kim YH , Park JW . SIRT1 deacetylates Sp1 and promotes its ubiquitin-dependent degradation in mycotoxin-induced cytotoxicity. Toxicology. (2014) ;316: (2):75–82. doi: 10.1016/j.tox.2013.11.004. |
[33] | Guo C , Zhang X , Pfeifer K , Hu H , Xi J , Sun Y , Liu H , Ma D , Lu J . Sp1 regulates the transcription of EGFR in cervical cancer cells. Oncology Reports. (2016) ;36: (4):2351–8. doi: 10.3892/or.2016.5069. |
[34] | Liang Y , Liu H , Zhu J , Song N , Lu Z , Fang Y , Teng J , Dai Y , Ding X . Inhibition of p53/miR-34a/SIRT1 axis ameliorates podocyte injury in diabetic nephropathy. Biochem Biophys Res Commun. (2021) ;559: :48–55. doi: 10.1016/j.bbrc.2021.04.025. |