Auricularia polytricha extract exerts SKN-1 and DAF-16 mediated longevity and stress resistance against UV-B exposure in Caenorhabditis elegans
Abstract
BACKGROUND:
Auricularia polytricha (AP) is traditionally known for its medicinal properties, and linoleic acid (LA) is the prominent component in AP.
OBJECTIVE:
To understand the anti-aging and stress resistance mechanism induced by AP in Caenorhabditis elegans.
METHODS:
C. elegans (wild-type (N2), transgenic, and mutant strains) were treated with AP and LA and monitored for lifespan and stress resistance through physiological assays, fluorescence microscopy, and qPCR analysis. Molecular docking studies were employed to identify the interaction mode of LA with DAF-16 and SKN-1.
RESULTS:
Ethanol extract of AP (APE) was non-toxic and could induce an anti-aging mechanism, as it could extend the lifespan of nematode worms. This was dependent on PMK-1 and DAF-16 as APE could not extend the lifespan of these gene-specific mutants apart from extending the expression of these genes in wild-type nematodes, which was evident from qPCR analysis. LA, too, had a similar effect on the lifespan of wild-type and mutant worms, which further supported the findings. Molecular docking studies pointed to the role of DAF-16 and SKN-1 in regulating the effect of APE. APE also exhibited antioxidant and anti-inflammatory mechanisms as it significantly extended the lifespan in worms exposed to UV-B-induced oxidative stress, thereby showing a protective effect. APE could regulate SKN-1, which was evident from qPCR analysis and the fluorescence of skn-1:GFP transgenic strain. Further qPCR analysis of candidate regulatory genes exhibited antioxidant mechanisms induced by APE.
CONCLUSION:
APE was observed to induce anti-aging efficacy via SKN-1 and DAF-16.
1Introduction
Increased incidences of degenerative lifestyle diseases have led to the opting for functional foods such as probiotics and plant-based nutritional supplements, which fulfill the normal dietary requirements and have beneficial nutraceutical properties that aid general well-being. A large content of our knowledge about natural therapeutics comes from traditional medicine that comprises various concoctions that utilize extracts of the available flora and fauna of the region. Recently, mushrooms have garnered much interest among researchers and have been studied extensively. Among them, edible mushrooms have been used worldwide as a source of nutrition and have now been widely sought after due to their rich protein content, carbohydrates, vitamins, essential trace elements, and fiber. Most edible mushrooms have medicinal properties and have been used from old times in traditional medicines and culinary delights [1].
Medicinal mushroom research showed the potential therapeutic properties of various species against ailments and infections, including antiviral, antibacterial, antidiabetic, anti-neurodegenerative, hepatoprotective, cardioprotective, and anticancer activities [2]. One among these is Auricularia polytricha (AP), which belongs to the Auriculariaceae family and is commonly known as wood ear or jelly ear mushroom. It is an edible macro-fungus that has been part of traditional culinary and medicinal preparations. It shows several properties such as antioxidant, antimicrobial, immunomodulatory, antitumoral activity, antidiabetic, and hyperlipidemia [3–8]. AP is rich in antioxidants and has also shown effective mitigation of oxidant radicals that lead to macromolecular damage [9], a common pathology in many diseases and in progressive aging [10]. Recently, various cosmetics have come out in the market that promise to slow down the signs of aging. One of the major causes of aging is the constant exposure to sunlight, which comprises UV radiations that trigger the production of oxidant radicals [11].
UV-B is a part of the electromagnetic spectrum having higher energy and shorter wavelength than UV-A. Hence, it can cross the epidermis as well as interact with the molecular components, triggering oxidative stress and DNA damage, activating pathways that cause an increase in connective tissue degeneration, decreased collagen production, and accumulation of senescent cells resulting in wrinkles, hyperpigmentation, photo-immunosuppression, and photo-carcinogenesis. Broad-spectrum sunscreens are most effective in reducing the damage caused by UV radiation to the skin. Currently, sunscreens are incorporated with natural resources that protect the skin and can enhance the overall protective activity of the skin. Therefore, this field offers a larger potential to be explored [11–13].
Recent studies from our team also reported that ethanol extract of AP (APE) showed a reduction in glutamate-induced neuronal toxicity and provided a neuroprotective effect via scavenging of reactive oxygen species (ROS) in mouse hippocampal cells (HT22) [14]. Another study also identified AP extract’s anti-inflammatory and cellular lipid accumulation properties in mouse macrophage cell lines [15]. Additionally, APE enhanced the lifespan and health span in the model system, Caenorhabditis elegans [14].
The genetically tractable soil nematode C. elegans has become an invaluable tool in understanding and deciphering several human diseases and ailments caused by biotic and abiotic stress. Owning to the presence of conserved pathways that regulate the normal homeostasis of eukaryotes, ease of maintenance, and availability of a complete sequenced genome has helped C. elegans gain its importance as a popular system in various fields that includes several aspects of cell and animal biology such as neuronal development, developmental biology, host-pathogen interactions, muscular development, tumorigenesis, apoptosis, toxicology, aging and wound healing [16–20].
The present study intends to analyze the mechanism of action induced by the APE and the major bioactive-compound involved (linoleic acid (LA) for its anti-aging effect and its role in mitigating UV-B induced stress using in vivo model C. elegans.
2Materials and methods
2.1Chemicals, reagents, and equipment’s used
The chemicals and reagents used in the study were obtained from Sigma-Aldrich (St. Louis, MO, USA) and Hi-Media Laboratories (Mumbai, India) unless otherwise specified. UV-B exposure for C. elegans strains was done using UV-B Lamp TL 20 W/01 RS, and intensity was detected using a digital UV radiometer (Solarmeter, Solartech Inc, USA).
2.2Plant collection and extraction
The APE extract used in the current study was from the same batch of extracts used in previous studies [14, 21] obtained from the dried fruiting body of AP macerated in ethanol, which was further dissolved in DMSO, aliquoted and stored in–20°C.
2.3Strains used and culture conditions of C. elegans
The present study used wild-type Bristol N2 as well as mutants KU25 (PMK-1), CF1038 (DAF-16), and transgenic strains LG333 (SKN-1::GFP) and TJ356 (DAF-16::GFP). All were procured from the Caenorhabditis Genetic Centre (CGC), University of Minnesota. The worms were cultured on nematode growth medium (NGM) and food source Escherichia coli OP50 at 20°C. All the studies were performed using age-synchronized L4 worms obtained by isolating eggs from gravid hermaphrodites using standard procedure [22].
2.4Life span assay
C. elegans (wild type or mutants: 10 numbers), UV exposed or unexposed worms treated with different concentrations of APE (30, 40, and 50μg/ml) or LA (60, 70, and 80μM) were transferred into a 24-well plate containing M9 buffer. The live nematodes were counted every 24 h. and calculated for their percentage survival, as explained previously [23].
2.5Analysis of UV-B impact on C. elegans
Wild type C. elegans were exposed to selected intensity of UV-B radiations 60, 100, and 1000 mJ/cm2. For further studies, 60 mJ/cm2 was considered. Age-synchronized L4 worms grown on E. coli OP50 at 20° C were washed thoroughly and placed into a 35 mm petri dish with 1 ml of M9 buffer and exposed to the different UV-B radiation doses and worms were treated with APE extract for 24 h. post UV-B exposure.
2.6Fluorescence imaging
Age-synchronized L4 population of LG333 strain (SKN-1::GFP) and TJ356 (DAF-16::GFP) exposed to UV-B at 60 mJ/cm2 and was treated with the APE extract for 24 h. After the treatment period, the worms from the independent trials were washed thoroughly and imaged with a confocal microscope (OLYMPUS FLUOVIEW FV10i), Centre for Scientific and Technological Research equipment (STREC), Chulalongkorn University and Fluorescent microscope (OLYMPUS BX35), The Science and Educational Co LTD, Bangkok.
2.7Homology modelling
DAF-16 and SKN-1 protein structures were modeled by employing the Homology modeling process, where the three-dimensional (3D) structure of a protein was constructed using the online program trROSETTA (transform-restrained Rosetta) [24], which generates a 3D model of protein via Ab Initio modeling technique. It utilizes the FASTA format of the amino acid sequence of the query protein. The FASTA sequences of both proteins were extracted from the Uniprot database with Uniprot ID – P34707 and O16850.
2.8Protein structure validation
The stereochemical quality of the modeled 3D protein structure was predicted using PDBSum, an online program [25]. This program estimates the polypeptide backbone conformation via the amino acid sequence’s phi/psi torsion angles. If the structure determination is reliable, it implies that most pairs will be located within the core or favored regions of the Ramachandran plot.
2.9Molecular docking analysis
The modeled 3D structure of DAF-16 and SKN-1 proteins and the chemical structures of the compounds of interest were employed for the docking studies. The chemical structures of the reference drugs Epigallocatechin gallate (DAF-16), Luteolin (SKN-1), and Linoleic acid (the major component of APE) were downloaded from PubChem database in 3D conformation with PubChem ID 65064, 5280445 and 5280450 respectively. The molecular docking was performed using a blind docking approach for both the target proteins to estimate the strong binding site of the molecules. DockThor program was used to calculate the binding affinities of the molecules against the target protein. It is a grid-based docking method which computes different binding modes of ligands on the protein structure [26]. Subsequently, the binding position of the potential lead molecule and its 2D interaction studies were done using Biovia Discovery Studio 4.5 (Dassault Systèmes BIOVIA, Discovery Studio Modelling Environment, Release 2017, San Diego: Dassault Systèmes, 2016).
2.10Total RNA isolation and quantitative real-time PCR (qPCR) analysis
As explained above, wild-type C. elegans were exposed to UV-B and/or treated with APE extract. Total RNA was isolated using GENEzolTM reagent using the protocol as prescribed by the manufacturer and further converted to cDNA from the experimental groups based on the manufacturer’s protocol. qPCR analysis was carried out using SYBR Green, RealMODTM Green W2 2xqPCR mix (iNtRon Biotechnology Inc., Korea), in the CFX connect Real-Time Detection system (Bio-Rad) with the help of gene-specific primers as explained previously [23].
2.11Statistical analysis
All the experiments were done in triplicates and expressed as Mean±SD. One-way ANOVA (SPSS 17, SPSS Inc., Chicago, IL) followed by Tukey’s post hoc test was performed to compare control vs. UV-B treated, UV-B treated vs. APE/LA treated, and p < 0.05 was considered statistically significant.
3Results
3.1APE is non-toxic and can improve lifespan in C. elegans
Previously, our group had reported that APE can extend the lifespan of C. elegans at specific concentrations (20 and 40μg/ml) [14]. In the present study, we sought to confirm that APE is not toxic to the nematodes and can extend lifespan. Hence, wild-type nematodes were treated with different concentrations of APE ranging between 10–1000μg/ml. It was observed that none of the doses were toxic to the nematodes, and the doses 20, 30, and 40μg/ml exhibited a maximum lifespan of up to 23 days each, whereas the untreated control survived up to 20 days. DMSO (1%) was used as solvent control, wherein the nematodes survived up to 19 days (Fig. 1A and B).
Fig. 1
Survival analysis of wild type C. elegans (N2) against different concentration of APE extract. Panel A shows the average maximum survival days and panel B represents the survival assay of concentrations (APE 30–50μg/ml) that are taken forward for the further experiments (Significance at p < 0.05 *Control vs APE treated).
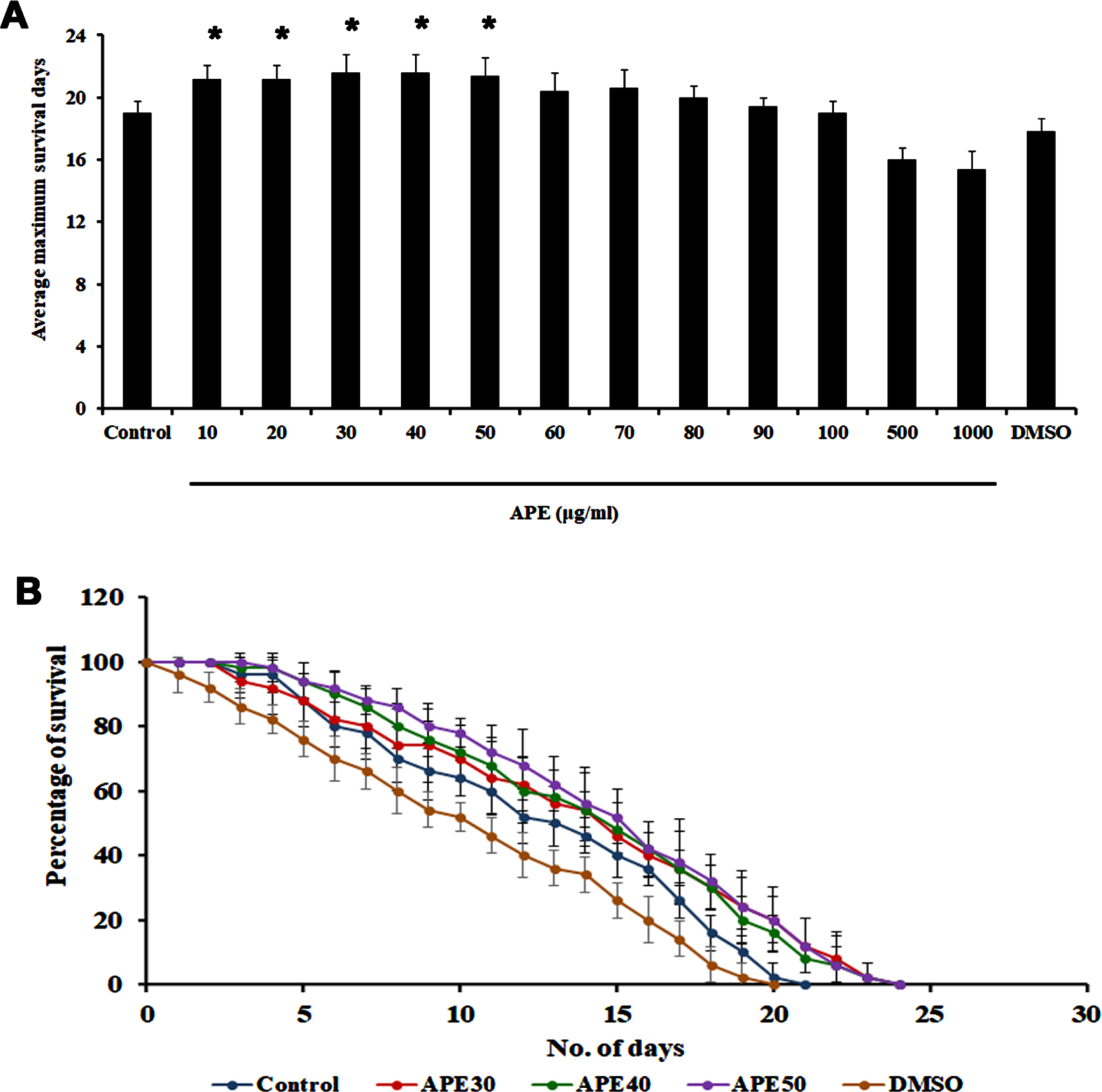
3.2APE-mediated lifespan extension was dependent on the MAPK pathway
To identify the mode of action of APE-mediated lifespan extension, mutants of pmk-1, a key player in the MAPK pathway were treated with 30–50μg/ml of APE extract. Interestingly, the extract was not able to induce any significant changes in lifespan, as the mutants survived up to 18.4, 18.8, 18.6, and 19 (control, APE 30μg, APE 40μg, APE 50μg, respectively) days each, similar to that of the untreated control (Fig. 2A and B). This indicates the importance of pmk-1 in exerting the lifespan-extending effects of APE.
Fig. 2
Panel A and B shows the survival analysis of pmk-1 mutant (KU25) representing average maximum survival days and percentage of survival respectively. Panel C shows the increase in mRNA expression of pmk-1 in wild type C. elegans on treatment with APE at different concentrations (APE 30–50μg/ml) (Significance at p < 0.05 *Control vs APE treated).
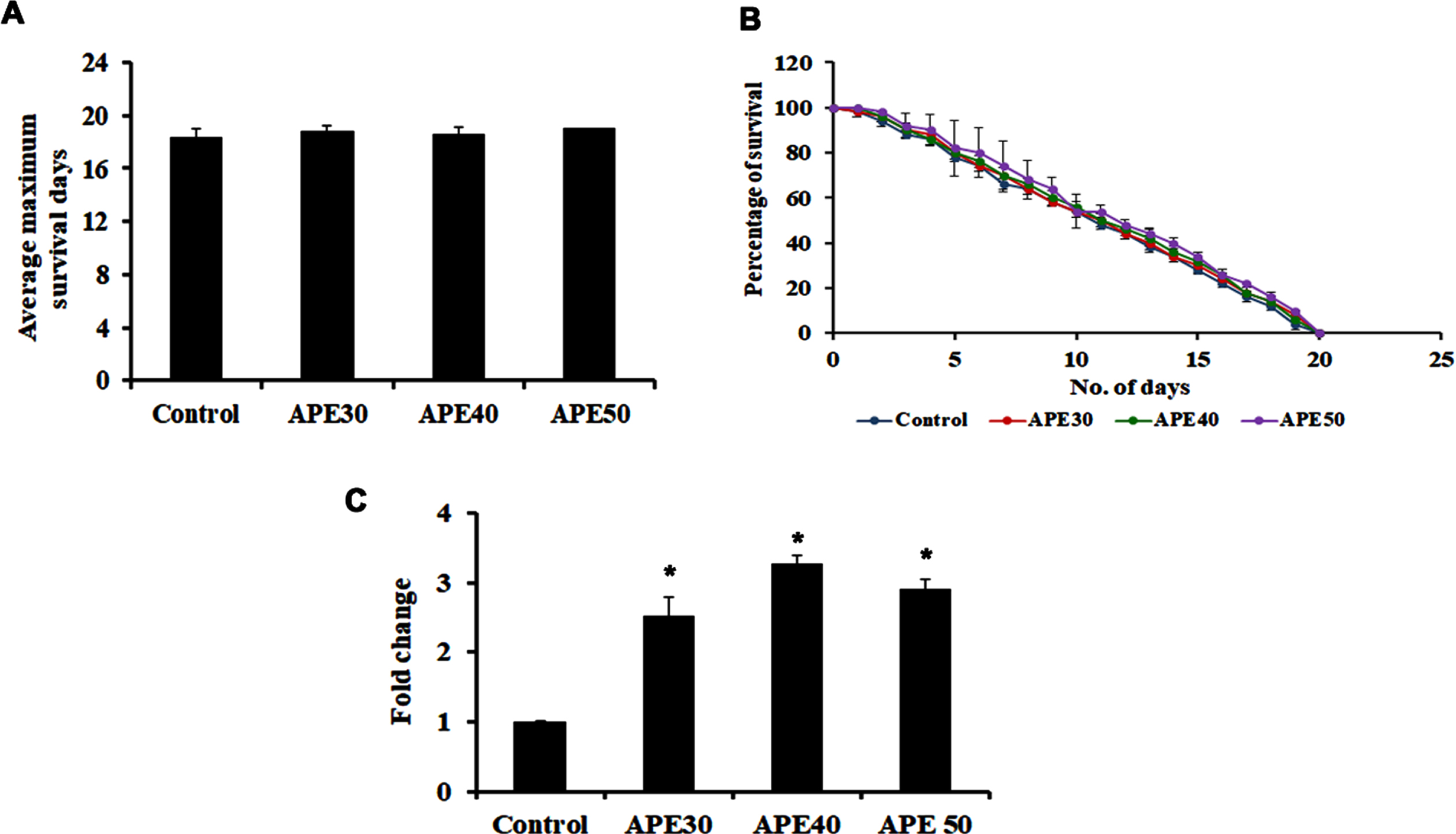
Additionally, qPCR analysis of pmk-1 was analyzed in wild-type nematodes after treatment with APE, which showed significant upregulation, indicating the pathway’s involvement in mediating APE-induced anti-aging activity (Fig. 2C).
3.3APE mediated lifespan extension was dependent on DAF-16 mediated pathway
DAF-16 mediated pathway is a significant pathway that regulates lifespan and activates anti-aging in C. elegans. On that note, the role of this pathway in APE-mediated lifespan extension was analyzed by treating 30–50μg/ml of APE extract to daf-16 mutants. Interestingly, APE could not induce any significant changes in the lifespan of these mutants, as the worms survived up to 16, 16, and 16 days, respectively, wherein the untreated control worms survived up to 15 days (Fig. 3A and B).
Fig. 3
Panel A and B shows the survival analysis of daf-16 mutant (CF1038) representing average maximum survival days and percentage of survival respectively. Panel C shows a decrease in mRNA expression of daf-2 and age-1 in wild type C. elegans on treatment with APE at different concentrations (APE 30–50μg/ml) (Significance at p < 0.05 *Control vs APE treated).
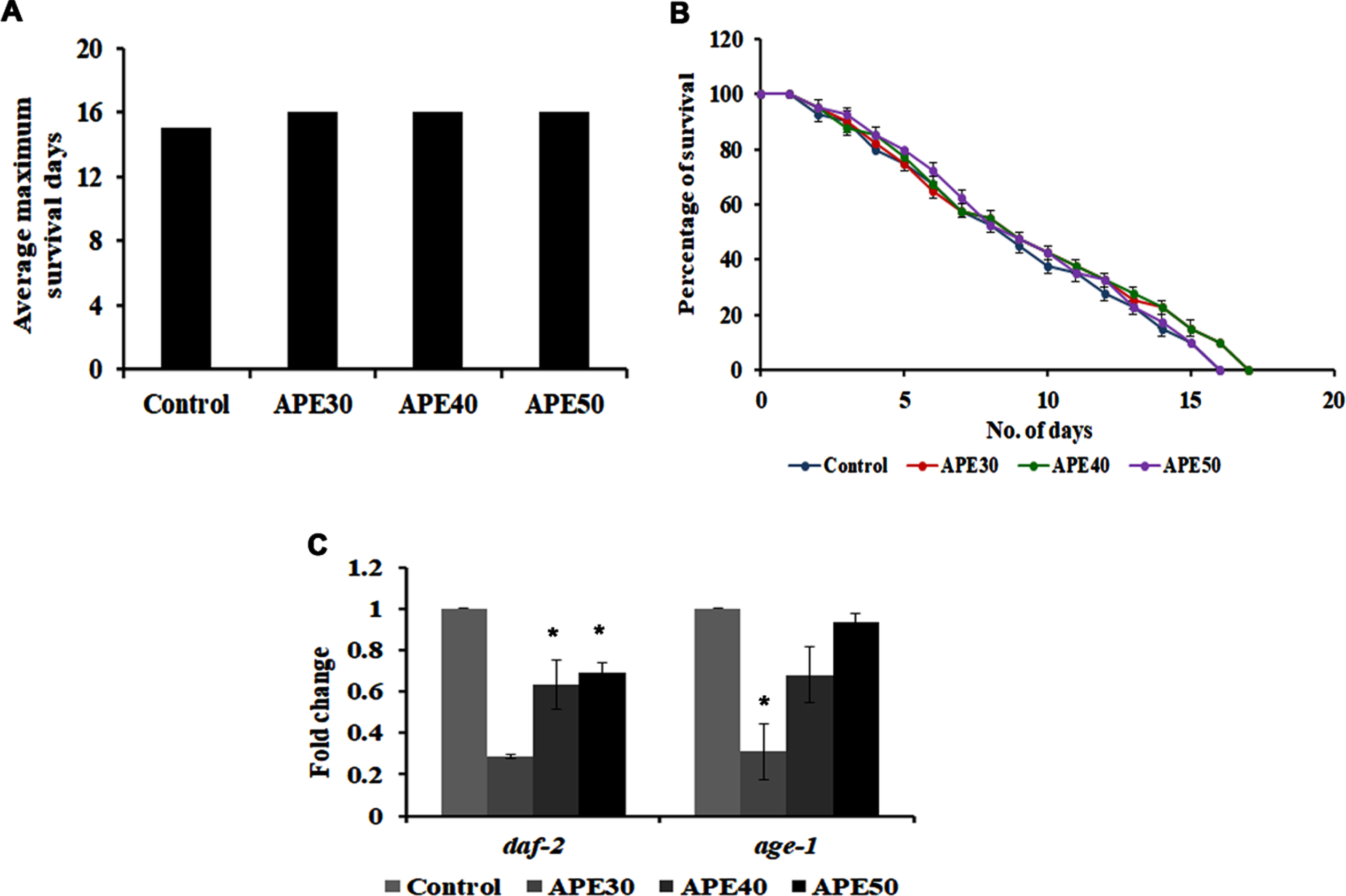
Furthermore, daf-2 and age-1, the candidate genes that regulate the pathway, were analyzed using qPCR analysis in wild-type nematodes. Their expression was found to be negatively regulated, indicating the pathway’s involvement in mediating APE-induced anti-aging activity (Fig. 3C).
3.4Linoleic acid, one of the significant lipophilic compounds in APE, can also extend the lifespan of C. elegans
In the previous study, our group had identified through GC/MS analysis that LA is the most predominant lipophilic compound present in APE, which may be responsible for the positive effects induced by the extract [14]. Hence, the present study also seeks to identify the effect of LA on the lifespan of wild-type nematodes. Different concentrations of LA varying from 10–100μM were treated with the nematodes, and it was observed that the compound could significantly extend the lifespan of C. elegans (Fig. 4A and B). Interestingly, the compound was not able to induce any significant changes in the lifespan of both pmk-1 (Fig. 4C and D) and daf-16 mutants (Fig. 4E and F), similar to that of APE, implying that LA activates the anti-aging mechanism in C. elegans through both pmk-1 and daf-16 mediated pathways.
Fig. 4
Panel A shows the average maximum survival of wild type C. elegans when treated with Linoleic acid with varying concentrations (LA 10–100μM) showed an increase in lifespan, panel B shows the percentage of survival in wild type C. elegans showing the lifespan extension in the selected dose (LA 60–80μM) which was used for further analysis. Lifespan extension of LA mediated through both MAPK and DAF-16 pathways, both pmk-1 (KU25, panel C and D) and daf-16 (CF1038, panel E and F) mutant did not show any significant changes on admiration of LA (Significance at p < 0.05 *Control vs LA treated).
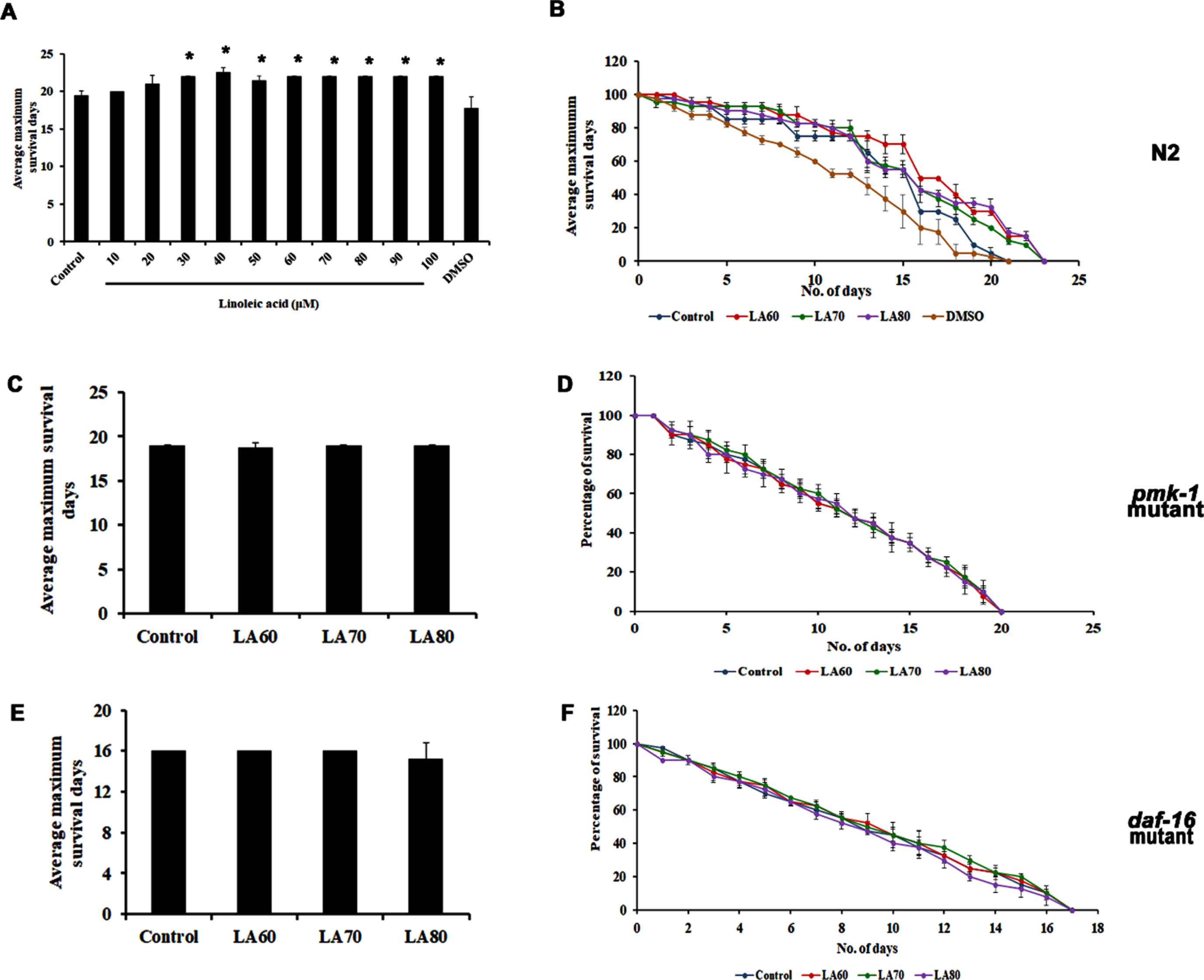
3.5Molecular docking studies further confirm the role of LA
3.5.1Ramachandran plot validation for modeled proteins
The 3D structures of the modeled proteins DAF-16 and SKN-1, generated by trROSETTA, were evaluated using PDBSUM for quality check. Ramachandran plot is represented in (Fig. 5A and B) the graphs obtained for each protein showed that the psi and phi angles are mainly located in the favored and allowed regions of the Ramachandran plot, indicating both proteins as reliable models.
Fig. 5
Ramachandran plot for DAF-16 (A) and SKN-1 (B).
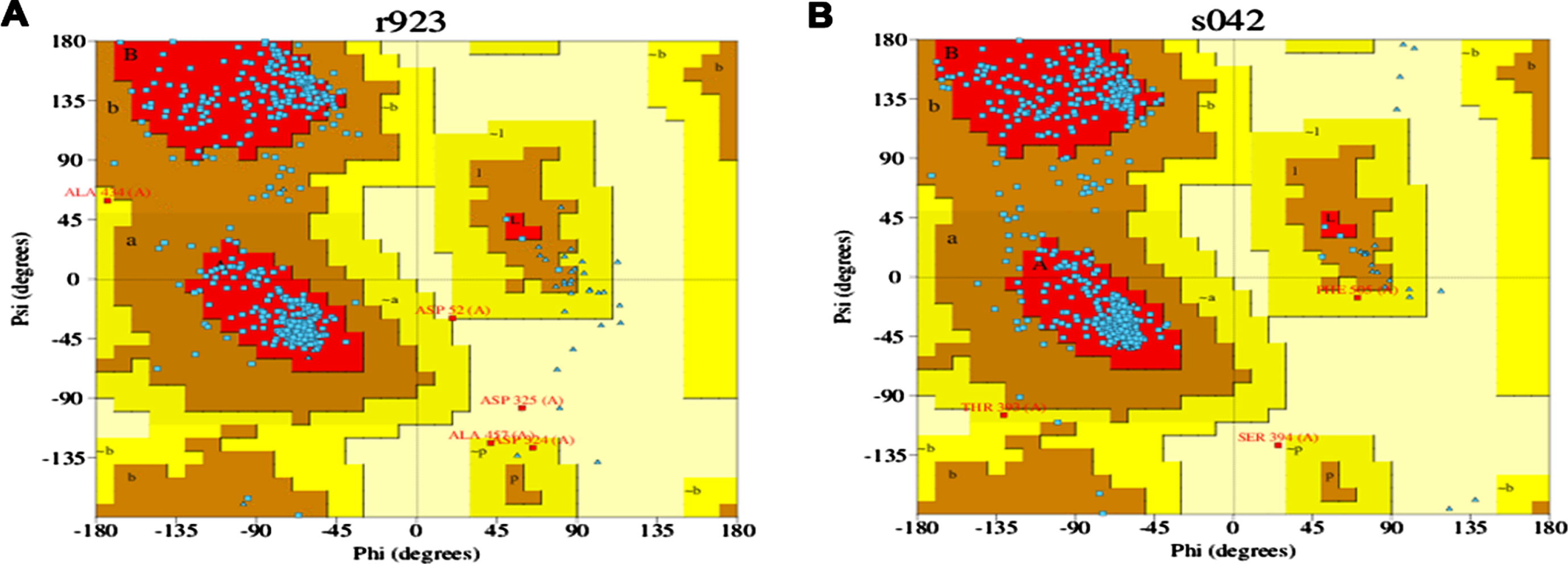
3.5.2Docking analysis
The modeled protein structures were taken for docking analysis using the respective reference compounds Epigallocatechin gallate (an inducer of DAF-16), Luteolin (inhibitor of SKN-1) [27], and LA using the DockThor algorithm to study the binding affinity and its modulatory action against both the target proteins. Luteolin was earlier reported to modulate skin aging and inflammation [28, 29]. The energy score obtained from DockThor for Epigallocatechin gallate was –7.53 kcal/mol against DAF16 (Fig. 6C), and Luteolin showed –7.99 kcal/mol against SKN-1 (Fig. 6F). In contrast, LA showed binding scores of –7.68 and –6.88 kcal/mol for DAF-16 and SKN-1 respectively. Overall, the results from our docking study reveal that the drug-target binding energy of the reference drugs and linoleic acid have almost similar docking scores, thus supporting our experimental studies. The results clearly indicate the strong binding ability of LA towards the target proteins and modulate their activities.
Fig. 6
Interaction sites of epigallocatechin gallate and Linoleic acid towards DAF-16 (A, B and C) and Luteolin and Linoleic acid interaction towards SKN-1 (D, E and F).
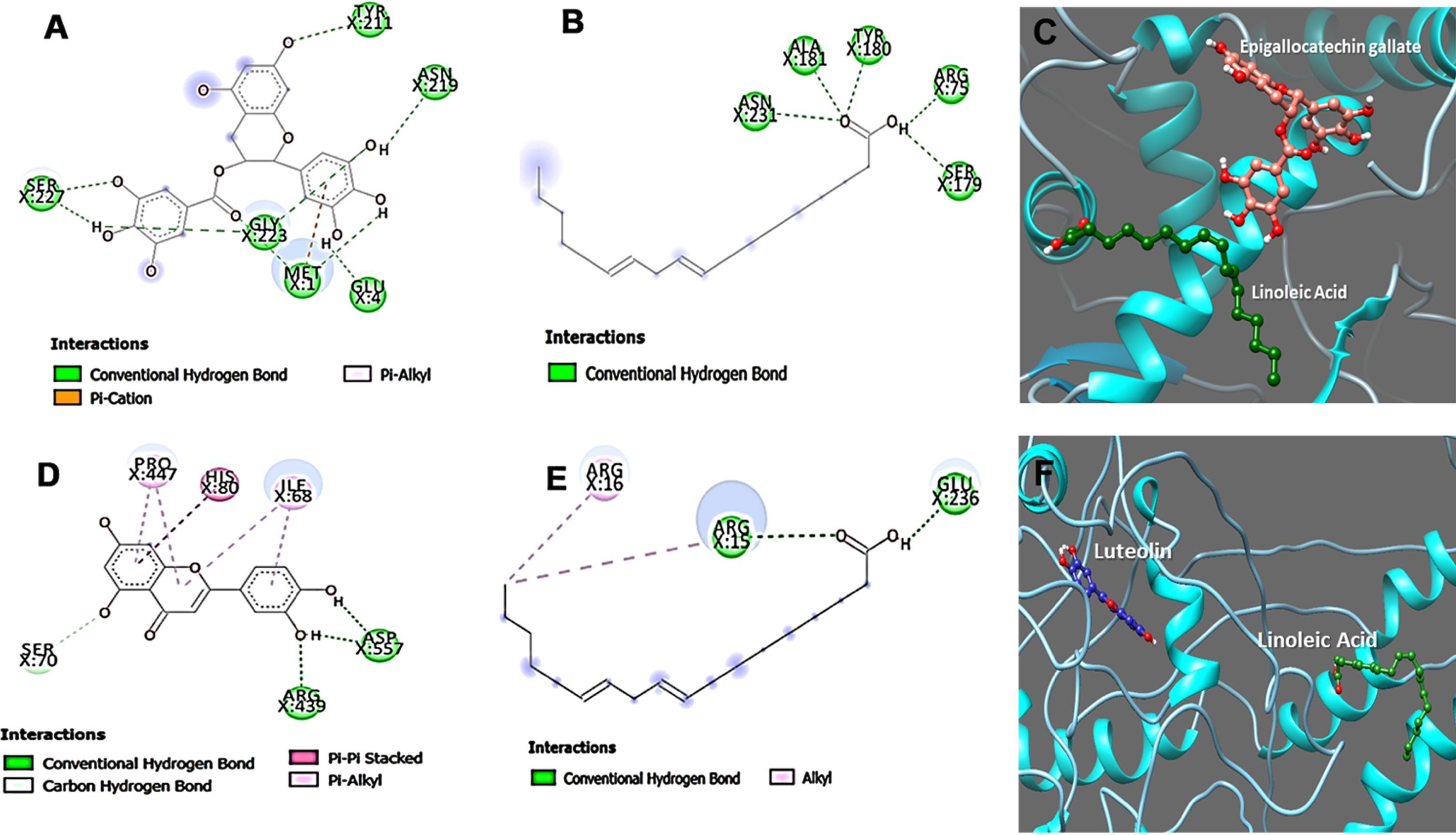
Further, the ligand interaction for both proteins was studied for Epigallocatechin gallate, Luteolin, and Linoleic acid to understand the binding mechanism of the interacting residues of the proteins. The protein-ligand interaction images were represented in the figure interaction. The interaction studies display different kinds of bond formation between ligand molecules and target protein, including conventional hydrogen bonds, alkyl bonds, π-sigma, π-cation, and π-alkyl bonds, as represented in Fig. 6. Linoleic acid can maintain Conventional hydrogen bonds interaction with Arg75, Ser179, Tyr180, Ala181, and Asn231 amino acid residues of DAF-16 protein whereas, in case of Epigallocatechin gallate the amino acid contributing for the conventional hydrogen bonds interactions are with residues Met1, Glu4, Tyr211, Asn219, Gly223 and Ser227 also a π-cation and π-alkyl interactions were formed with Met1(Fig. 6A and B). In addition, the interactions maintained by amino acid residues of SKN-1 protein with Linoleic acid are with residues Arg15, Glu236 (Conventional hydrogen bonds), whereas Arg16 made Alkyl bond. On the other hand, Luteolin can form π-π stacked bond with His80 and π-alkyl with Pro447 and Ile68, and we observed conventional hydrogen bond interactions with Arg439 and Asp557 (Fig. 6D and E). We conclude that hydrogen bonds contribute to the efficient binding of Epigallocatechin gallate, Luteolin, and Linoleic acid with the protein DAF-16 and SKN-1.
3.6APE can impart protection against UV-B-induced stress in C. elegans
APE was subjected to analysis of its effect in mediating oxidative stress in C. elegans. UV is a known source to induce oxidative stress and a series of other damages to the nematode. In the present study, C. elegans were exposed to UV-B in different intensities, 60, 100, and 1000 mJ/cm2. The wild-type worms were observed to survive up to an average of 18, 17, and 9 days, respectively, whereas the untreated control survived up to 21 days (Fig. 7A and B).
Fig. 7
Impact of UV-B of varying intensities on C. elegans and rescue by APE. Panel A and B show the average maximum survival and percentage of C. elegans exposed to varying intensities of 60 mJ/cm2, 100 mJ/cm2 and 1000 mJ/cm2. The Average maximum days of survival of the exposed worms found to be significantly reduced (p < 0.005). APE (30–50μg/ml) was found to rescue and extend lifespan in wild type C. elegans from the effects of various intensities of UV- B exposure compared to untreated group (Panel C, D, E, F, G and H). # Represents significance (p < 0.05) between control and UV B/APE extract treated, *represents significance (p < 0.05) between UV-B exposed and treated groups.
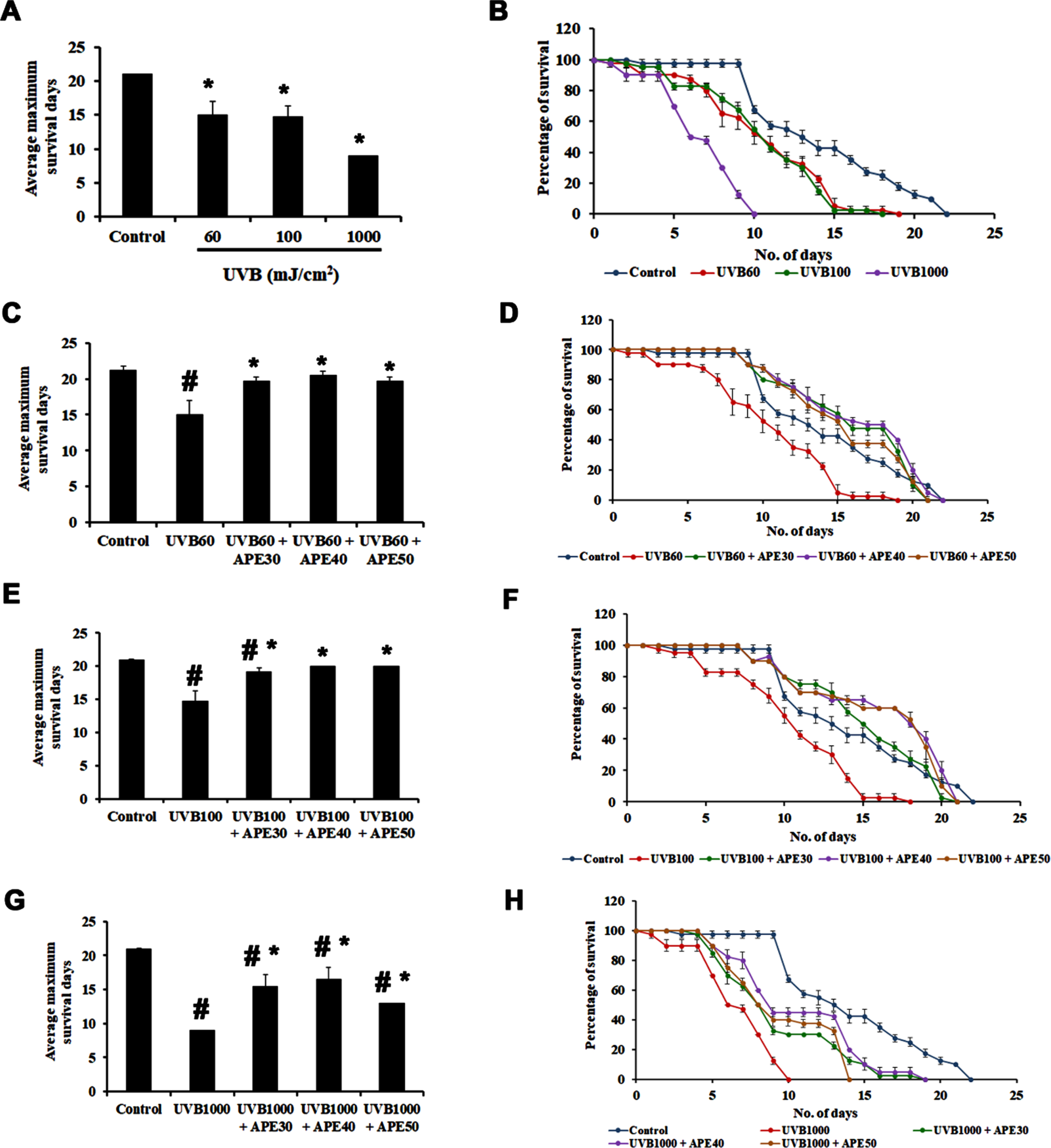
Further, worms exposed to 60 mJ/cm2 of UV-B were treated post UV-B exposure with different concentrations of APE (30–50μg/ml). It was observed that the worms survived up to an average of 20, 21, and 20 days, respectively, compared to the worms exposed to UV-B alone, surviving for 18 days (Fig. 7C and D). Similarly, worms exposed to 100 mJ/cm2 of UV-B when treated post UV-B exposure with different concentrations of APE (30–50μg/ml) survived up to 20, 20, and 20 days respectively when compared to the worms exposed to UV-B alone surviving for 17 days (Fig. 7E and F) and those exposed to 1000 mJ/cm2 of UV-B, on post-exposure treatment with different concentrations of APE (30–50μg/ml) survived up to 18, 18 and 13 days respectively when compared to the worms exposed to UV-B alone surviving for 9 days (Fig. 7G and H), indicating the protective effect of APE.
3.7SKN-1 plays a role in mediating the rescue of UV-B-exposed C. elegans
SKN-1, an ortholog of mammalian NF-E2-related transcription factor (NRF-2), plays a major role in activating antioxidant activity. The regulation of skn-1 was monitored using a transgenic strain LG333. An overall SKN-1 expression was found to be increased in UV-B (60 mJ/cm2) exposed worms compared to control, in comparison with the C. elegans treated with APE post-UV-B exposure (Fig. 8B-E). Also, the qPCR data corroborated these findings (Fig. 8A). The regulation of daf-16 was observed using transgenic strain TJ356. DAF-16 expression was found to be increased in UV-B exposed in treated worms compared to APE-treated C. elegans (Fig. 9).
Fig. 8
Panel A shows the qPCR analysis of skn-1 in UV-B exposed wildtype C. elegans at 24 h with or without APE treatment. Panel B quantified expression of level of SKN-1. SKN-1 expression in transgenic strain in LG333 (C) Control, (D) UV-B exposed, (E) APE treated worms exposed to UV-B at 24 h. # represents significance (p < 0.05) between control and UV-B/APE extract treated groups, *represents significance (p < 0.05) between UV-B exposed and treated groups.
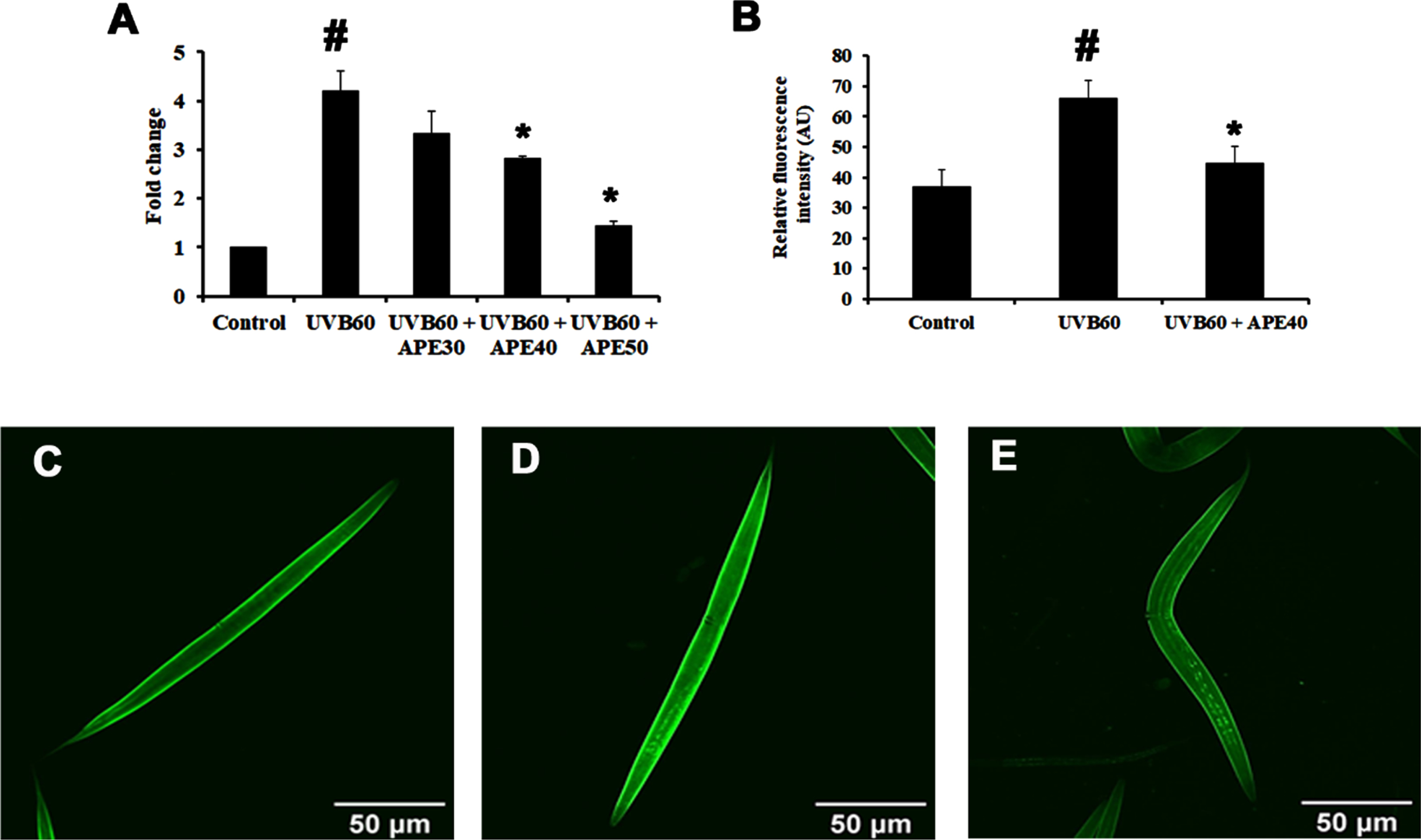
Fig. 9
DAF-16 translocation in transgenic strain TJ356 (A) Control (B) UV-B exposed (C) APE treated worms exposed to UV-B at 24 h. The arrows show the expression and translocation of DAF-16. Panel D shows the quantified level of DAF-16. # represents significance (p < 0.05) between control and UV-B/APE extract treated groups, *represents significance (p < 0.05) between UV-B exposed and treated groups.
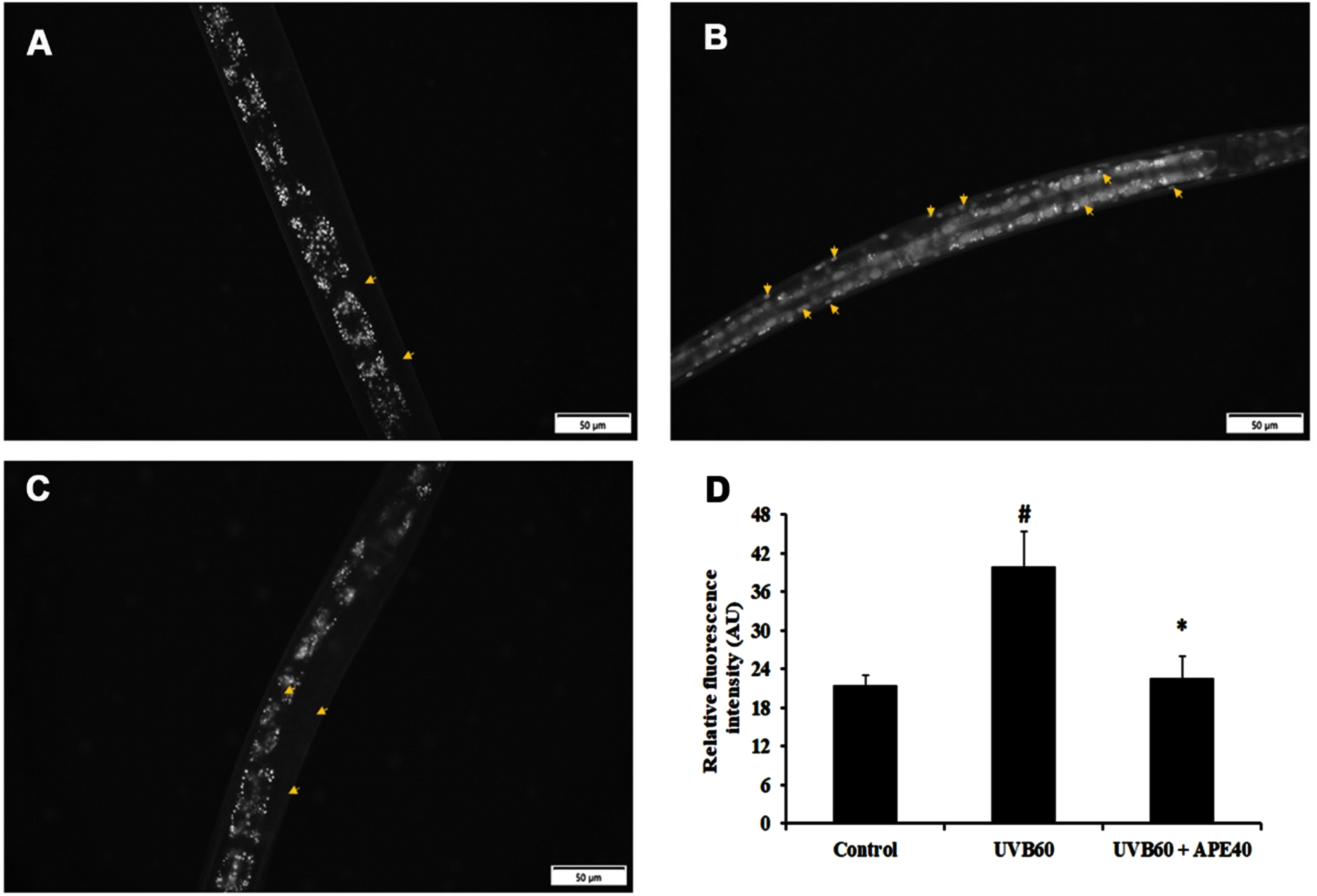
Furthermore, qPCR analysis of antioxidant genes such as sod-1 and gst-4 showed an increase in expression when treated with APE for 24 h post-UV-B exposure (Fig. 10). Additionally, the expression of col-19, an adult marker for collagen and responsible for the maintenance of adult worm cuticle was also observed. Previous studies reported that disruption of COL-19 can lead to morphological defects in alae [30]. It was found that APE 30–50μg/ml treatment post-UV-B exposure exhibited an increase in col-19 expression compared to the UV-B exposed untreated N2 worms (Fig. 10).
Fig. 10
qPCR analysis of mRNA expression of col-19, gst-4 and sod-1 post exposure UV-B with or without treatment with APE. Treated group showed increased mRNA expression in comparison with UV-B exposure alone. # represents significance (p < 0.05) between control and UV-B/APE extract treated, *represents significance (p < 0.05) between UV-B exposed and treated groups.
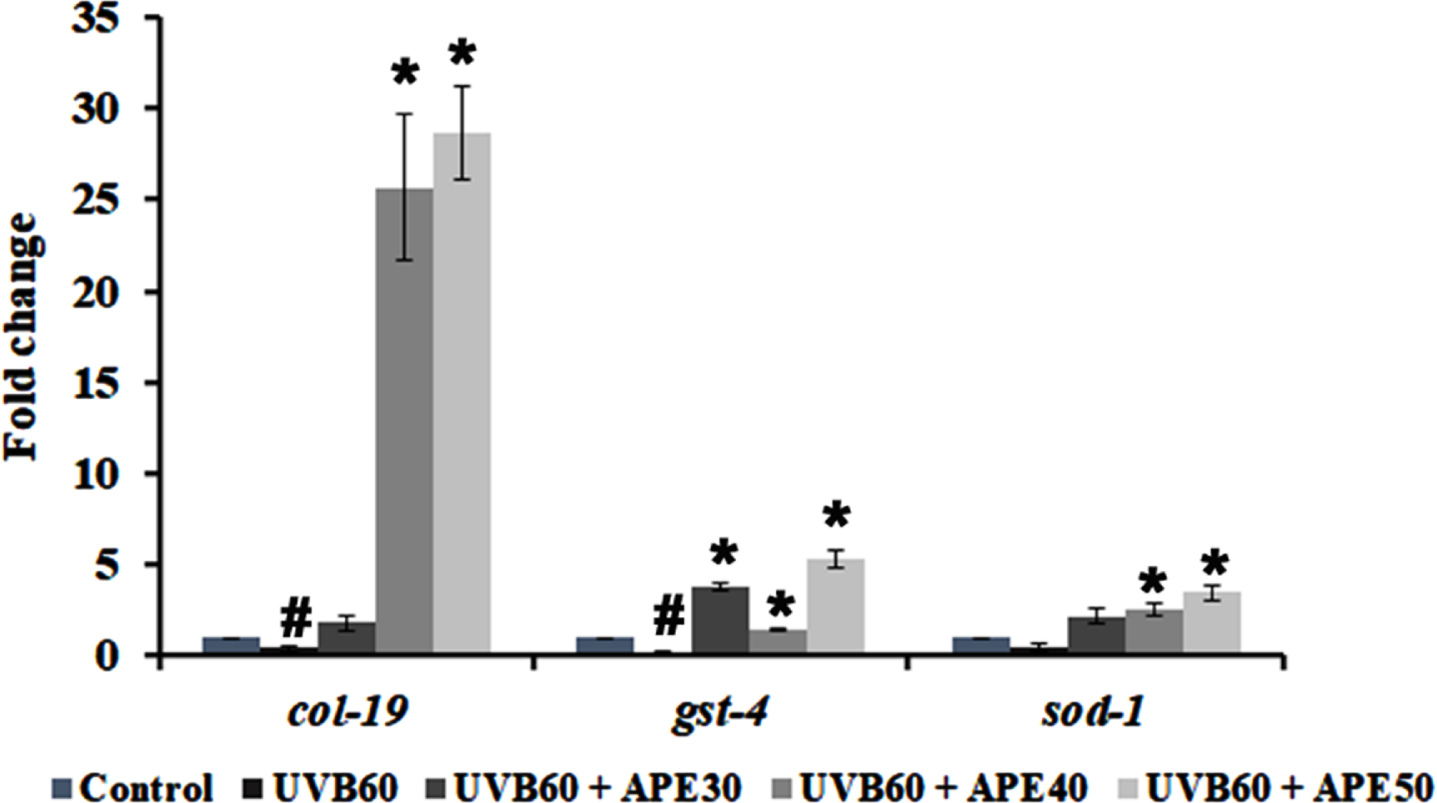
4Discussion
Biological and environmental stresses are detrimental to the induction and acceleration of the normal aging process [31–35]. Skin, the largest body organ, forms a protective barrier against the external environment is also a visible indicator of aging. Hence, it is essential to take extra measures to protect it from solar radiation and pollutants that tend to cause premature aging [36]. Several cellular and molecular factors, including reactive oxygen species (ROS) production, dysregulation of signal transduction, and mutation, are involved in the process, wherein these phenomena can be counteracted by acting appropriately by balancing behavioral and nutritional factors [32, 34]. Hence, several natural resources such as plants, mushrooms, and marine organisms that may or may not be a part of traditional medicinal practices have been currently explored to identify derivatives that may possess antiaging properties [2, 32, 37, 38].
Auricularia polytricha is an edible medicinal mushroom used in various traditional preparations and cuisines around the globe. Crude extracts from AP reportedly exhibited properties beneficial for overall health [15]. The non-toxic APE extract exhibited an extension of lifespan and health span in wild-type C. elegans (Fig. 1 and B), indicating its antiaging potential [14]. Interestingly, the mRNA expressions of daf-2, which encodes for receptor tyrosine kinase and its downstream player age-1, a phosphatidyl inositol 3-kinase (PI3K) homolog, were found to be downregulated during treatment with APE extract in the N2 worms (Fig. 2C), whereas pmk-1 expression was found to be increased (Fig. 3C). Moreover, no extension in lifespan was found in daf-16 (Fig. 3A and B) and pmk-1 (Fig. 2A and B) mutants when treated with APE extract, suggesting the involvement of both DAF-2/DAF-16 insulin and PMK-1/p38 MAPK pathway mediated regulation of lifespan extension in C. elegans treated with APE (Figs. 1–3).
Fig. 11
A schematic representation of the protective effect of APE against UV-B radiation in C. elegans.
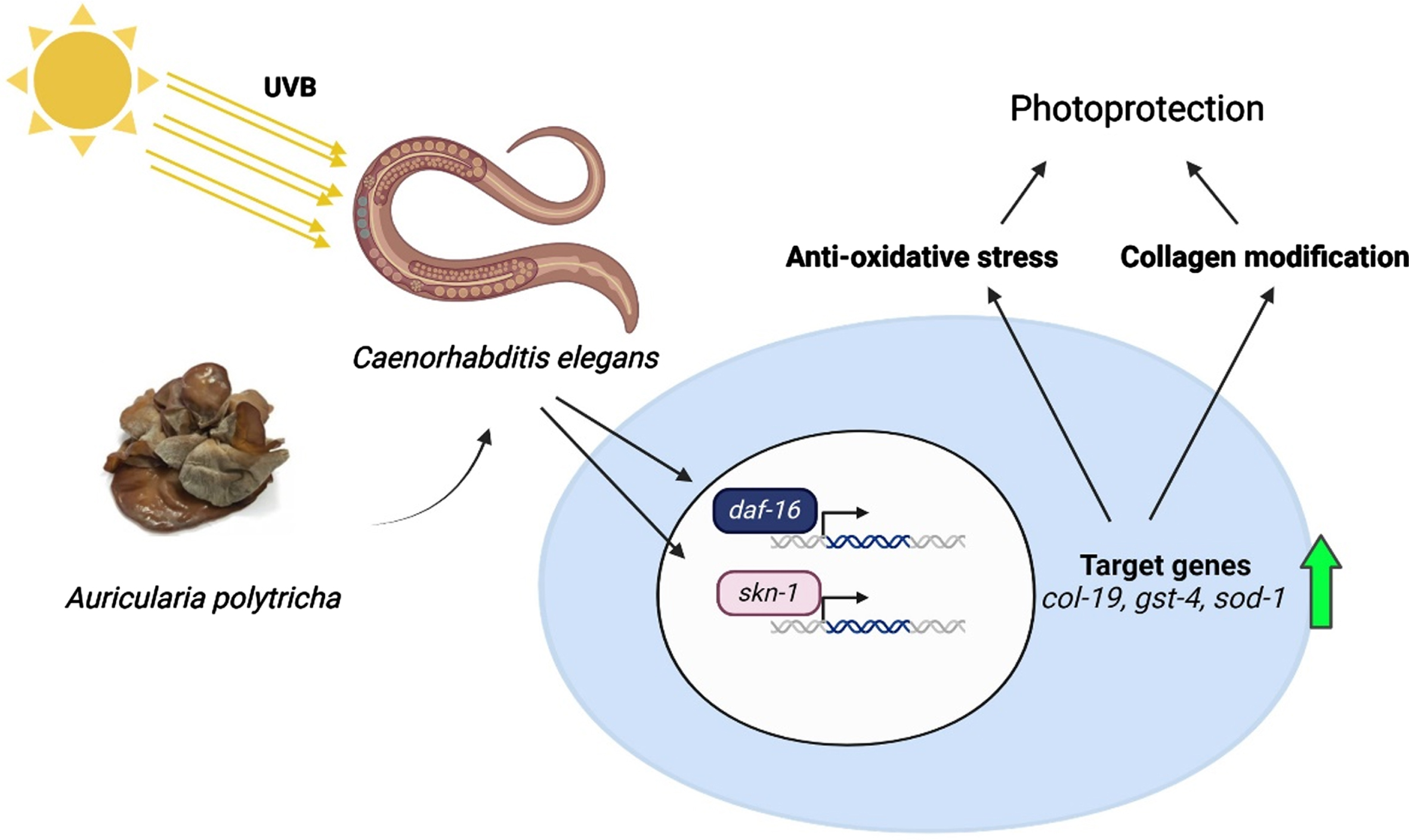
Previous studies show DAF-2/DAF-16 insulin/insulin-like signaling pathway regulates lifespan extension and aging in C. elegans by the translocation of DAF-16, an ortholog of FOXO-1 in humans, from the cytoplasm to nucleus mediated through the reduction of DAF-2 activation [39, 40]. It was also reported that during both internal and external stress conditions in C. elegans, the lifespan extension by DAF-16 was meditated by its interaction with other regulatory pathways and players, including p38 MAPK pathway [41], SKN-1, and HSF-1 [39].
The APE extract was previously reported to show antioxidant activity [14]. Hence, the impact of UV-B radiation of various intensities 60 mJ/cm2, 100 mJ/cm2, and 1000 mJ/cm2 on wild-type C. elegans and the protective effect of APE against it were analyzed. UV-B exposure to N2 worms at all the given intensities resulted in a severe lifespan reduction compared to the unexposed control group (Fig. 7A and B). In contrast, the APE (30, 40, and 50μg/ml) treatment showed a rescue and extension of lifespan in all of the UV-B exposed groups (Fig. 7C-H), indicating that APE can reduce the oxidative stress induced by UV-B.
SKN-1, the C. elegans ortholog of mammalian NRF-2, plays a significant role in mounting response to diverse stress factors to maintain the homeostasis of the host, including oxidative and xenobiotic stress [42, 43]. The stress response can have progressive effects on the aging process. Furthermore, it can result in the accumulation of functional losses that culminate in many age-related clinical conditions that result in diseases, including pulmonary disorder, cancer, and neurodegenerative disorders [44]. Exposure to UV radiations (A, B or C) can induce severe oxidative damage that results in the progression of aging and related pathologies [45]. In line with the reports, SKN-1 expression was found to be increased in LG333 strains at 24 h post-exposure of UV-B compared to the control. Although SKN-1 is associated with regulating homeostasis upon stress response [46], previous study has revealed that constitutive activation of SKN-1 can be detrimental in C. elegans as it impairs the ability of the nematodes to resist stress and increase ROS levels [43, 47, 48]. In studies with SKN-1 gain-of-function, nematodes showed age-dependent somatic depletion (ASDF) of fats and shortened lifespan, corroborating the observed results [49, 50]. However, the exposed worms treated with APE were found to reduce the SKN-1 activity (Fig. 8B and C), which was further validated through the qPCR analysis of skn-1 in N2 worms (Fig. 8A). Studies have reported that SKN-1 regulation can be mediated by both DAF-16 and PMK-1 mediated pathways [45, 51]. Interestingly, dietary supplementation of PUFAs (LA) and antioxidants can rescue worms under ASDF condition triggered by increase in SKN-1 under oxidative stress [49], which corroborates with findings of the present study (Fig. 8). The protective effect thus observed by APE against UV-B can be attributed to the regulatory activity of SKN-1 through PMK-1 and DAF-16-mediated pathways (Figs. 2 and 3A-B), as well as the presence of LA in the extract, which could act as a negative regulator of SKN-1 as observed from docking analysis. Additionally, qPCR analysis of antioxidant genes gst-4 and sod-1 was upregulated in the APE-treated group of C. elegans post UV-B exposure compared to untreated worms (Fig. 10), suggesting SKN-1 mediated antioxidant gene regulation.
COL-19 is a cuticle collagen and forms an integral part of the C. elegans exoskeleton. The cuticle protects the worms from the external environment and impacts [52, 53]. The disruption of COL-19 was found to cause morphological changes in C. elegans [30]. Previous studies report that UV radiation damages extracellular matrix elements such as elastin and collagen [54]. Additionally, a study reported the downregulation of mRNA expression of col-19 during UV-A exposure in C. elegans [55]. The present study shows an increased fold in mRNA expression of col-19 when treated with APE extracts post UV-B exposure (Fig. 10), suggesting the activation of conserved and C. elegans-specific pathway activation in re-establishing the exoskeletal integrity [56].
Edible mushrooms are widely known for the presence of essential fatty acids such as linoleic, oleic, and linolenic, which are important for the human diet, compared to other food sources, making it commercially highly valuable [57]. LA is one of the predominant components identified through GC/MS analysis in APE extract [14] and belongs to a group of polyunsaturated fatty acids (omega-6 PUFAs) that are well known for their health-promoting beneficial effects, including suppression of aging [58, 59]. Interestingly, C. elegans, unlike mammals, is capable of de novo synthesis of essential fatty acids due to the presence of Δ12 desaturase and ω3 desaturase enzymes [60]. Studies have reported that supplementation of LA played a role in rescuing mutant worms with loss of function of HLH-30, which plays a major role in survival during starvation, as well as SBP-1 mutant in restoration of body size and brood size and also modulation of p38 MAPK pathway [59–61]. Studies also suggest that supplementation of ω6 fatty acids can promote longevity. In the present study, LA exhibited longevity towards N2 worms at varying concentrations, and this lifespan extension was mediated through both PMK-1 and DAF-16 pathways (Fig. 4). Reportedly, deuterated PUFAs improve in vivo redox conditions under both normal and oxidative stress conditions, leading to lifespan extension [62]. Furthermore, the in silico docking analysis also showed strong binding activity of LA towards DAF-16 and SKN-1 (Figs. 5 and 6). Additionally, various studies have reported the induction of DAF-16 expression upon UV-mediated damages in C. elegans, which acts as an adaptive mechanism in the nematodes to combat stress [63, 64]. Further, DAF-16 is also involved in regulating fat homeostasis, which could be observed from the activation of the gene upon reduction in fat accumulation in fat-related gene RNAi worms [65]. APE was found to reduce the nuclear translocation of DAF-16 upon UV-B exposure (Fig. 9) in the current study, which could be due to the early antioxidant effects of the extract against UV-B-mediated changes.
5Conclusion
In summary, the antioxidants, including LA present in APE, play a significant regulatory role in the modulation of influential players such as PMK-1, DAF-16, and SKN-1, resulting in the rescue and extension of life span in C. elegans post UV-B exposure. AP extracts have also previously shown neuroprotective effects, and mushrooms are abundant sources of antioxidants and PUFAs. Hence, it could be used in the food and cosmetic industry as an additive to promote healthcare, especially in an aging population.
Acknowledgments
RS wishes to thank the support of the Second Century Fund (C2F) Postdoctoral Fellowship, Chulalongkorn University, Thailand. We also Thank Dr. James M. Brimson (Research Unit for Innovation and International Affairs, Faculty of Allied Health Sciences, Chulalongkorn University) for his proofreading and language editing of this manuscript. We sincerely thank Dr.Mani Iyer Prasanth for technical assistance and critical comments. In addition, we would like to thank Sunita Nilkhet for producing figure using BioRender.
Funding
This research was funded by Thailand Science Research and Innovation Fund, Chulalongkorn University (CU_FRB65_hea (78) _173_37_03).
Conflicts of interest
The authors declare no conflicts of interest.
References
[1] | Das AK , Nanda PK , Dandapat P , Bandyopadhyay S , Gullón P , Sivaraman GK , McClements DJ , Gullón B , Lorenzo JM . Edible mushrooms as functional ingredients for development of healthier and more sustainable muscle foods: A flexitarian approach. Molecules. (2021) ;26: (9):2463. |
[2] | Abebaw G . Review on: Nutrtional Value and Health Benefits of Edible Mushroom. Journal of Engineering and Applied Sciences Technology. SRC/JEAST-122. (2020) ;DOI:https://doi.org/10.47363/JEAST/2020 (2), 113. |
[3] | Yu M , Xu X , Qing Y , Luo X , Yang Z , Zheng L . Isolation of an anti-tumor polysaccharide from Auricularia polytricha (jew’s ear) and its effects on macrophage activation. Eur Food Res Technol. (2009) ;228: , 477–485. |
[4] | Song G , Du Q . Structure characterization and antitumor activity of an α β-glucan polysaccharide from Auricularia polytricha . Nat Prod Res. (2012) ;26: (21):1963–70. |
[5] | Emre AVCI , Cagatay G , Avci GA , Suiçmez M , Cevher SC . An edible mushroom with medicinal significance; Auricularia polytricha . Hittite Journal of Science and Engineering. (2016) ;3: (2):111–116. |
[6] | Budinastiti R , Sunoko HR , Widiastiti NS . The Effect of Cloud Ear Fungus (Auricularia polytricha) on Serum Total Cholesterol, LDL And HDL Levels on Wistar Rats Induced by Reused Cooking Oil. In E3S Web of Conferences (Vol. 31, p. 06006). EDP Sciences. (2018) . |
[7] | Fang D , Wang D , Ma G , Ji Y , Zheng H , Chen H , Zhao M , Hu Q , Zhao L . Auricularia polytricha noodles prevent hyperlipemia and modulate gut microbiota in high-fat diet fed mice. Food Science and Human Wellness. (2021) ;10: (4):431–441. |
[8] | Liu Q , Ma R , Li S , Fei Y , Lei J , Li R , Pan Y , Liu S , Wang L . Dietary Supplementation of Auricularia auricula-judae Polysaccharides Alleviate Nutritional Obesity in Mice via Regulating Inflammatory Response and Lipid Metabolism. Foods. (2022) ;11: (7):942. |
[9] | Hossen M , Billah Prince M , Tanvir EM , Chowdhury M , Rahman M , Alam F , Paul S , Saha M , Ali M , Bhoumik NC , Karim N , Gan SH , Khalil MI . Ganoderma lucidum and Auricularia polytricha mushrooms protect against Carbofuran-induced toxicity in rats. Evid Based Complement Alternat Med. (2018) )6254929. |
[10] | Hajam YA , Rani R , Ganie SY , Sheikh TA , Javaid D , Qadri SS , Pramodh S , Alsulimani A , Alkhanani MF , Harakeh S , Hussain A , Haque S , Reshi MS . Oxidative Stress in Human Pathology and Aging: Molecular Mechanisms and Perspectives. Cells. (2022) ;11: (3):552. |
[11] | Amaro-Ortiz A , Yan B , D’Orazio JA . Ultraviolet radiation, aging and the skin: prevention of damage by topical cAMP manipulation. Molecules. (2014) ;19: (5):6202–6219 . |
[12] | Moyal DD , Fourtanier AM . Broad-spectrum sunscreens provide better protection from solar ultraviolet– simulated radiation and natural sunlight– induced immunosuppression in human beings. J Am Acad Dermatol. (2008) ;58: (5 Suppl 2):S149–54. |
[13] | Cavinato M , Waltenberger B , Baraldo G , Grade CVC , Stuppner H , Jansen-Dürr P . Plant extracts and natural compounds used against UVB-induced photoaging. Biogerontology. (2017) ;18: (4):499–516. |
[14] | Sillapachaiyaporn C , Rangsinth P , Nilkhet S , Ung AT , Chuchawankul S , Tencomnao T . Neuroprotective effects against Glutamate-Induced HT-22 hippocampal cell damage and Caenorhabditis elegans lifespan/healthspan enhancing activity of Auricularia polytricha mushroom extracts. Pharmaceuticals (Basel). (2021) ;14: (10):1001. |
[15] | Sangphech N , Sillapachaiyaporn C , Nilkhet S , Chuchawankul S . Auricularia polytricha ethanol crude extract from sequential maceration induces lipid accumulation and inflammatory suppression in RAW264. 7 macrophages. Food Funct. (2021) ;12: (21):10563–10570. |
[16] | Ellis HM , Horvitz HR . Genetic control of programmed cell death in the nematode C. elegans . Cell. (1986) ;44: (6):817–29. |
[17] | Hengartner MO , Ellis R , Horvitz R . Caenorhabditis elegans gene ced-9 protects cells from programmed cell death. Nature. (1992) ;356: (6369):494–9. |
[18] | Ono S . Regulation of structure and function of sarcomeric actin filaments in striated muscle of the nematode Caenorhabditis elegans . Anat Rec (Hoboken). (2014) ;297: (9):1548–59. |
[19] | Ruijtenberg S , van den Heuvel S . G1/S inhibitors and the SWI/SNF complex control cell-cycle exit during muscle differentiation. Cell. (2015) ;162: (2):300–313. |
[20] | Elkabti AB , Issi L , Rao RP . Caenorhabditis elegans as a model host to monitor the Candida infection processes. J Fungi (Basel). (2018) ;4: (4):123. |
[21] | Sillapachaiyaporn C , Nilkhet S , Ung AT , Chuchawankul S . Anti-HIV-1 protease activity of the crude extracts and isolated compounds from Auricularia polytricha . BMC Complementary Altern Med. (2019) ;19: , 351. |
[22] | Brenner S . The genetics of Caenorhabditis elegans . Genetics. (1974) ;77: (1):71–94. |
[23] | Prasanth MI , Malar DS , Brimson JM , Verma K , Tonsomboon A , Plaingam W , Tencomnao T . DAF-16 and SKN-1 mediate Anti-aging and Neuroprotective efficacies of “thai ginseng” Kaempferia parviflora Rhizome extract in Caenorhabditis elegans . Nutr Healthy Aging. (2022) ;1–16. |
[24] | Yang J , Anishchenko I , Park H , Peng Z , Ovchinnikov S , Baker D . Improved protein structure prediction using predicted interresidue orientations. Proc Natl Acad Sci U S A. (2020) ;117: (3):1496–1503. |
[25] | Laskowski RA , Hutchinson EG , Michie AD , Wallace AC , Jones ML , Thornton JM . PDBsum: a Web-based database of summaries and analyses of all PDB structures. Trends Biochem Sci. (1997) ;22: (12):488–90. |
[26] | Santos KB , Guedes IA , Karl AL , Dardenne LE . Highly flexible ligand docking: benchmarking of the DockThor program on the LEADS-PEP protein– peptide data set. J Chem Inf Model. (2020) ;60: (2):667–683. |
[27] | Chian S , Thapa R , Chi Z , Wang XJ , Tang X . Luteolin inhibits the Nrf2 signaling pathway and tumor growth in vivo . Biochem Biophys Res Commun. (2014) ;447: (4):602–8. |
[28] | Gendrisch F , Esser PR , Schempp CM , Wölfle U . Luteolin as a modulator of skin aging and inflammation. Biofactors. (2021) ;47: (2):170–180. |
[29] | Chen J , Li Y , Zhu Q , Li T , Lu H , Wei N , Huang Y , Shi R , Ma X , Wang X , Sheng J . Anti-skin-aging effect of epigallocatechin gallate by regulating epidermal growth factor receptor pathway on aging mouse model induced by d-Galactose. Mech Ageing Dev. (2017) ;164: , 1–7. |
[30] | Thein MC , McCormack G , Winter AD , Johnstone IL , Shoemaker CB , Page AP . Caenorhabditis elegans exoskeleton collagen COL-19: An adult-specific marker for collagen modification and assembly, and the analysis of organismal morphology. Dev Dyn. (2003) ;226: (3):523–39. |
[31] | Lavretsky H , Newhouse PA . Stress, inflammation, and aging. Am J Geriatr Psychiatry. (2012) ;20: (9):729–33. |
[32] | Prasanth MI , Brimson JM , Chuchawankul S , Sukprasansap M , Tencomnao T . Antiaging, stress resistance, and neuroprotective efficacies of Cleistocalyx nervosum var. paniala fruit extracts using Caenorhabditis elegans model. Oxid Med Cell Longev. (2019) ;2019;7024785. |
[33] | Yegorov YE , Poznyak AV , Nikiforov NG , Sobenin IA , Orekhov AN . The link between chronic stress and accelerated aging. Biomedicines. (2020) ;8: (7):198. |
[34] | Burke KE Environmental aging of the skin; new insights. Plast Aesthet Res. (2020) ;7: , 59 |
[35] | Ganguly B , Hota M , Pradhan J . Skin Aging: Implications of UV Radiation, Reactive Oxygen Species and Natural Antioxidants. In Reactive Oxygen Species. IntechOpen.2021. |
[36] | Farage MA , Miller KW , Elsner P , Maibach HI . Characteristics of the aging skin. Adv Wound Care (New Rochelle). (2013) ;2: (1):5–10. |
[37] | Wang X , Zhang Z , Zhang S , Yang F , Yang M , Zhou J , Hu Z , Xu X , Mao G , Chen G , Xiang W , Sun X , Xu N . Antiaging compounds from marine organisms. Food Res Int. (2021) ;143: , 110313. |
[38] | Resende DI , Ferreira M , Magalhães C , Lobo JS , Sousa E , Almeida IF . Trends in the use of marine ingredients in anti-aging cosmetics. Algal Res. (2021) ;55: , 102273. |
[39] | Zeˇci´c A , Braeckman BP . DAF-16/FoxO in Caenorhabditis elegans and Its Role in Metabolic Remodeling. Cells. (2020) ;9: (1):109. |
[40] | Zhang YP , Zhang WH , Zhang P , Li Q , Sun Y , Wang JW , Zhang SO , Cai T , Zhan C , Dong MQ . Intestine-specific removal of DAF-2 nearly doubles lifespan in Caenorhabditis elegans with little fitness cost. Nat Commun. (2022) ;13: (1):6339. |
[41] | Troemel ER , Chu SW , Reinke V , Lee SS , Ausubel FM , Kim DH . p38 MAPK regulates expression of immune response genes and contributes to longevity in C. elegans . PLoS Genet. (2006) ;2: (11):e183. |
[42] | Yoon DS , Choi Y , Cha DS , Zhang P , Choi SM , Alfhili MA , Polli JR , Pendergrass D , Taki FA , Kapalavavi B , Pan X , Zhang B , Blackwell TK , Lee JW , Lee MH . Triclosan Disrupts SKN-1/Nrf2-Mediated Oxidative Stress Response in C. elegans and Human Mesenchymal Stem Cells. Sci Re. (2017) ;7: (1):12592. |
[43] | Nhan JD , Turner CD , Anderson SM , Yen CA , Dalton HM , Cheesman HK , Ruter DL , Naresh NU , Haynes CM , Soukas AA , Pukkila-Worley R , Curran SP . Redirection of SKN-1 abates the negative metabolic outcomes of a perceived pathogen infection. Proc Natl Acad Sci U S A. (2019) ;116: (44):22322–22330. |
[44] | Liguori I , Russo G , Curcio F , Bulli G , Aran L , Della-Morte D , Gargiulo G , Testa G , Cacciatore F , Bonaduce D , Abete P . Oxidative stress, aging, and diseases. Clin Interv Aging. (2018) ;13: , 757–772. |
[45] | Prasanth MI , Gayathri S , Bhaskar JP , Krishnan V , Balamurugan K . Understanding the role of p38 and JNK mediated MAPK pathway in response to UV-A induced photoaging in Caenorhabditis elegans. Journal of Photochemistry and Photobiology B: Biology. Apr 1. (2020) ;205: , 111844. |
[46] | Blackwell TK , Steinbaugh MJ , Hourihan JM , Ewald CY , Isik M . SKN-1/Nrf, stress responses, and aging in Caenorhabditis elegans . Free Radic Biol Med. (2015) ;88: (Pt B):290–301. |
[47] | An JH , Blackwell TK . SKN-1 links C. elegans mesendodermal specification to a conserved oxidative stress response. Genes & development. (2003) ;17: (15):1882–93. |
[48] | Deng J , Dai Y , Tang H , Pang S . SKN-1 is a negative regulator of DAF-16 and somatic stress resistance in Caenorhabditis elegans . G3 (Bethesda). (2020) ;10: (5):1707–1712. |
[49] | Lynn DA , Dalton HM , Sowa JN , Wang MC , Soukas AA , Curran SP . Omega-3 and-6 fatty acids allocate somatic and germline lipids to ensure fitness during nutrient and oxidative stress in Caenorhabditis elegans . Proc Natl Acad Sci U S A. (2015) ;112: (50):15378–83. |
[50] | Paek J , Lo JY , Narasimhan SD , Nguyen TN , Glover-Cutter K , Robida-Stubbs S , Suzuki T , Yamamoto M , Blackwell TK , Curran SP . Mitochondrial SKN-1/Nrf mediates a conserved starvation response. Cell metabolism. (2012) ;16: (4):526–37. |
[51] | Keshet A , Mertenskötter A , Winter SA , Brinkmann V , Dölling R , Paul RJ . PMK-1 p38 MAPK promotes cadmium stress resistance, the expression of SKN-1/Nrf and DAF-16 target genes, and protein biosynthesis in Caenorhabditis elegans . Mol Genet Genomics. (2017) ;292: (6):1341–1361. |
[52] | Taffoni C , Pujol N . Mechanisms of innate immunity in C. elegans epidermis. Tissue Barriers. (2015) ;3: (4):e1078432. |
[53] | Sandhu A , Badal D , Sheokand R , Tyagi S , Singh V . Specific collagens maintain the cuticle permeability barrier in Caenorhabditis elegans . Genetics. (2021) ;217: (3):iyaa047. |
[54] | Dhital B , Durlik P , Rathod P , Gul-E-Noor F , Wang Z , Sun C , Chang EJ , Itin B , Boutis GS . Ultraviolet radiation reduces desmosine cross-links in elastin. Biochem Biophys Re. (2017) ;10: , 172–177. |
[55] | Prasanth MI , Santoshram GS , Bhaskar JP , Balamurugan K . Ultraviolet-A triggers photoaging in model nematode Caenorhabditis elegans in a DAF-16 dependent pathway. Age. (2016) ;38: (1):1–13. |
[56] | Tong A , Lynn G , Ngo V , Wong D , Moseley SL , Ewbank JJ , Goncharov A , Wu YC , Pujol N , Chisholm AD . Negative regulation of Caenorhabditis elegans epidermal damage responses by death-associated protein kinase. Proc Natl Acad Sci U S A. (2009) ;106: (5):1457–61. |
[57] | Sande D , de Oliveira GP , Moura MAFE , de Almeida Martins B , Lima MTNS , Takahashi JA . Edible mushrooms as a ubiquitous source of essential fatty acids. Food Res Int. (2019) ;125: , 108524. |
[58] | Chen J , Wei Y , Chen X , Jiao J , Zhang Y . Polyunsaturated fatty acids ameliorate aging via redox-telomere-antioncogene axis. Oncotarget. (2017) ;8: (5):7301–7314. |
[59] | Murphy JT , Liu H , Ma X , Shaver A , Egan BM , Oh C , Boyko A , Mazer T , Ang S , Khopkar R , Javaheri A , Kumar S , Jiang X , Ory D , Mani K , Matkovich SJ , Kornfeld K , Diwan A . Simple nutrients bypass the requirement for HLH-30 in coupling lysosomal nutrient sensing to survival. PLoS Biol. (2019) ;17: (5):e3000245. |
[60] | Watts JL . Using Caenorhabditis elegans to Uncover Conserved Functions of Omega-3 and Omega-6 Fatty Acids. J Clin Med. (2016) ;5: (2):19. |
[61] | Nandakumar M , Tan MW . Gamma-linolenic and stearidonic acids are required for basal immunity in Caenorhabditis elegans through their effects on p38 MAP kinase activity. PLoS Genet. (2008) ;4: (11):e1000273. |
[62] | Beaudoin-Chabot C , Wang L , Smarun AV , Vidović D , Shchepinov MS , Thibault G . Deuterated polyunsaturated fatty acids reduce oxidative stress and extend the lifespan of C. elegans . Front Physiol. (2019) ;10: :641. |
[63] | Bianco JN , Schumacher B . MPK-1/ERK pathway regulates DNA damage response during development through DAF-16/FOXO. Nucleic Acids Research. (2018) ;46: (12):6129–39. |
[64] | Mueller MM , Castells-Roca L , Babu V , Ermolaeva MA , Müller RU , Frommolt P , Williams AB , Greiss S , Schneider JI , Benzing T , Schermer B . DAF-16/FOXO and EGL-27/GATA promote developmental growth in response to persistent somatic DNA damage. Nature Cell Biology. (2014) ;16: (12):1168–79. |
[65] | Horikawa M , Sakamoto K . Polyunsaturated fatty acids are involved in regulatory mechanism of fatty acid homeostasis via daf-2/insulin signaling in Caenorhabditis elegans . Mol Cell Endocrinol. (2010) ;323: (2):183–92. |