Streblus asper Lour. exerts MAPK and SKN-1 mediated anti-aging, anti-photoaging activities and imparts neuroprotection by ameliorating Aβ in Caenorhabditis elegans
Abstract
BACKGROUND:
Streblus asper Lour., has been reported to have anti-aging and neuroprotective efficacies in vitro.
OBJECTIVE:
To analyze the anti-aging, anti-photoaging and neuroprotective efficacies of S. asper in Caenorhabditis elegans.
METHODS:
C. elegans (wild type and gene specific mutants) were treated with S. asper extract and analyzed for lifespan and other health benefits through physiological assays, fluorescence microscopy, qPCR and Western blot.
RESULTS:
The plant extract was found to increase the lifespan, reduce the accumulation of lipofuscin and modulate the expression of candidate genes. It could extend the lifespan of both daf-16 and daf-2 mutants whereas the pmk-1 mutant showed no effect. The activation of skn-1 was observed in skn-1::GFP transgenic strain and in qPCR expression. Further, the extract can extend the lifespan of UV-A exposed nematodes along with reducing ROS levels. Additionally, the extract also extends lifespan and reduces paralysis in Aβ transgenic strain, apart from reducing Aβ expression.
CONCLUSIONS:
S. asper was able to extend the lifespan and healthspan of C. elegans which was independent of DAF-16 pathway but dependent on SKN-1 and MAPK which could play a vital role in eliciting the anti-aging, anti-photoaging and neuroprotective effects, as the extract could impart oxidative stress resistance and neuroprotection.
1Introduction
Plants have always played a role in human health as a medicine or as an immune enhancer [1, 2]. The use of plants as medicines paved the way for unique treatments for various diseases including headache, dizziness, cold, wounds, cough, asthma and many more which is still being practiced in developing countries [3–5]. The advent of modern medicine was from the foundation laid by traditional medicinal practices [5]. However, the excess use of antibiotics and synthetic drugs has led to various unexpected side effects, leading many researchers to look back into the traditional knowledge for different medicines [1]. A recent report suggests that phytochemicals from plant sources can even act against the coronavirus [6].
Aging is a unique and constant process that eventually leads to age-related diseases, majorly neurodegeneration, and increased oxidative stress. In other words, aging and neurodegeneration are two sides of a coin as both are dependent on each other and are inevitable. Plants can synthesize large amounts of diverse bioactive substances, many of which have the potential to provide substantial antioxidant, neuroprotective and anti-aging benefits. Examples of herbal remedies include green tea, turmeric, cinnamon, ginger, and rosemary, which are widely used across the world due to their health benefits [7, 8]. Essential oils extracted from flowers, leaves, seeds, rhizomes, or barks, of different plants, mainly thyme, clove, eucalyptus, cinnamon leaf, juniper, basil, chamomile, coriander, cumin, could be used for their anti-aging, antioxidant and neuroprotective effects [9].
Streblus asper Lour. belongs to the family Moraceae and the subfamily of Moroideae with the 4th tribe Strebleae along with Taxotrophis, Phyllochlamys, and Maillardia. S. asper was used in traditional medicine in treating cardiac disorders, epilepsy, edema, leprosy, dysentery, elephantiasis, and tuberculous glands. Different parts of the plant including bark, roots, seeds, leaves, and latex, are used for their different medicinal properties, including fever, diarrhea, dysentery, disinfect wounds, toothache, filariasis, leprosy, snakebite, regulating blood pressure, stomach-ache, obesity and other skin disorders [10–12].
The plant has been identified to have different pharmacological and medicinal properties such as anti-cancer [13], antioxidant [14], antibacterial [15], anti-fungal [16], anti-diabetic [17] and anti-inflammatory effects [18]. We have previously reported the neuroprotective efficacies of S. asper in glutamate-induced oxidative toxicity in mouse hippocampal (HT22) cells. Liquid Chromatography-Mass Spectrometry (LC-MS) screening of the extract revealed the presence of andrographolide, carnosic acid, α-linolenic acid, and oleoyl oxazolopyridine along with known compounds such as (+)-3-O-β-D-fucopyranosylperiplogenin, strebluslignanol and magnolignan A, and taxifolin [19]. Additionally, when the extract was separated into acidic, basic and neutral fractions, the acidic fraction exhibited the strongest antioxidant and antibacterial potential, whereas the basic and neutral fractions exhibited neuroprotective effects. Gas chromatography-mass spectrometry (GC-MS) analysis of these fractions confirmed the presence of terpenoids, steroids, phenolics, fatty acids, and lipidic plant hormone [20].
Caenorhabditis elegans, the in vivo model system, has been widely used to study aging, development, reproduction, obesity, stress resistance, neuroprotection and many more diverse mechanisms [21–24]. Small size, short lifespan and life cycle, ease of handling and maintenance, completely sequenced genome are some of the advantages offered by this model, which attracts researchers towards it [23, 25]. The nematodes have been utilized to understand the different compounds or conditions that could impact aging, which could be analyzed using various physiological and molecular parameters [23, 26]. Further, the availability of mutant and transgenic strains allows the model to mimic the pathologies of neurological disorders, making them a preferred model [23].
A variety of plants such as Bacopa monnieri [21], Sonneratia apetala [27], Betula utilis [28], Rehmannia glutinosa [29], Diplocyclos palmatus [30], Cleistocalyx nervosum [31], Caesalpinia mimosoides [32] and Glochidion zeylanicum [33] have been recently identified to exert anti-aging effects in C. elegans. The present study aims to understand the anti-aging, antioxidant and neuroprotective mechanisms of S. asper in C. elegans and the mode of action exhibited by the plant to induce the effect.
2Materials and methods
2.1Chemicals, reagents, and equipment used
The chemicals and reagents utilized in the present study were obtained from Sigma-Aldrich (St. Louis, MO, USA) and HiMedia Laboratories (Mumbai, India). C. elegans strains were kept under UV transilluminator lamp, SANKYO DENKI (F20T10BL), for 4 h for UV-A exposure.
2.2Plant collection and extraction
Leaves of S. asper were collected from the Princess Maha Chakri Sirindhorn Herbal Garden (Rayong Province, Thailand), authenticated [A013419 (BCU)] and deposited in Kasin Suvatabhandhu (Department of Botany, Faculty of Science, Chulalongkorn University, Thailand) herbarium. Dried powdered leaves (35 g) were soaked in absolute ethanol for 48 h, and the resulting supernatant was collected and vacuum dried. The crude ethanol extract (100 mg) was dissolved in DMSO filtered (0.2μm) and stored at –20 °C until use [19].
2.3C. elegans strains used and culture conditions
Wild type strain N2 (Bristol), daf-16 mutant CF1038, daf-2 mutant CB1370, pmk-1 mutant KU25, skn-1::GFP transgenic strain LG333, Aβ transgenic strain CL2006 and the bacterial food source E. coli OP50 were purchased from Caenorhabditis Genetics Center, (University of Minnesota, USA). All strains were grown, maintained and propagated in Nematode growth medium (NGM) at 15 °C as per the standard protocol [34]. Age synchronized young adult worms were used for conducting all the experiments.
2.4Lifespan assay
Analysis of lifespan was performed in liquid media. Briefly, ten age synchronized young adult nematodes (wild type or mutants) were transferred into a 24 well microtiter plate with M9 buffer along with E. coli OP50 and 5-Fluoro-2′-deoxyuridine (FUDR). Different concentrations (1–100μg/ml for wild type and 10–30μg/ml for mutants) of S. asper extracts dissolved in DMSO were added to each well. The worms alive on the well were counted every 24 h. Nematodes were considered to be dead when they did not respond to gentle prodding using the platinum loop. Worms with no extract treatment but only with E. coli OP50 served as the control and DMSO as the vehicle control. The experiments were carried out in five independent trials [31].
2.5Pharyngeal pumping assay
Pharyngeal pumping was measured in young adult staged wild type nematodes (∼10) grouped under control and extract treated, by monitoring the pharyngeal contractions for 30 seconds in every 24 h under a stereomicroscope (Motic SMZ-171) [31].
2.6Fluorescence imaging
Accumulation of lipofuscin in the wild type nematodes and Nuclear factor erythroid 2-related factor 2 (NRF2) green fluorescent protein (GFP) transgenic strain LG333 were monitored using Confocal microscope. Briefly, worms treated with varying S. asper concentrations (10–30μg/ml) consecutively for five days were monitored, with E. coli OP50 fed worms as control. After incubation, the worms were thoroughly washed using M9 buffer several times and then placed on a glass slide into a drop of sodium azide. Fluorescent imaging was performed using a ZEISS LSM 700 Confocal microscope under 10X magnification in 10 nematodes. The images were further analyzed using Image J software, and the relative fluorescence was represented as arbitrary units (AU). The experiment was carried out in three independent trials [31, 35].
2.7Measurement of extracellular ROS using DCF
Briefly, two sets of wild type nematodes were taken for UV-A exposure for 4 h. In the first set, the worms were transferred to the microtitre plate and then treated with varying concentrations of S. asper extracts (10–30μg/ml) and taken for UV-A exposure (pre-treatment). In the second set, the worms were transferred to the microtitre plate and then taken for UV-A exposure followed by treatment with varying concentrations of S. asper extracts (10–30μg/ml) (post-treatment). In both cases, the extract treatment continued for five days under standard conditions and then the worms were washed thoroughly with M9 buffer. The worms were then incubated with DCFH-DA (5μg/ml) for 20 minutes, followed by another wash using M9 buffer to remove the excess of DCFH-DA. The worms were placed in a glass slide and imaged under a confocal microscope. The images were analyzed using Image J software, and the relative fluorescence was represented as arbitrary units (AU). Worms exposed to UV-A for 4 h and did not receive any extract treatment were considered a positive control, whereas worms without UV-A exposure or extract treatment were considered negative control. The experiment was carried out in three independent trials [31].
2.8Superoxide dismutase assay
SOD activity was measured using the protein lysate from control and treated worms obtained by crushing the worms, and the protein concentration was measured using the Bradford method. Protein (50μg) from each sample is mixed with 100 mM Triethanolamine-Diethanolamine buffer (pH-7.4), 2 mM NADPH, 100 mM EDTA-MnCl2 and the reaction was initiated by the addition of 10 mM β-mercaptoethanol. The decrease in absorbance was measured for 5 min at 340 nm, and the enzyme activity was expressed as Units/mg of protein [36].
2.9Analysis of photoaging effect
This experiment was done according to Prasanth et al. [37] with slight modifications. As explained in the previous section, worms were treated with S. asper (10–30μg/ml) before and after UV-A exposure and their lifespan was monitored. Worms exposed to UV-A alone and did not receive any treatment of extracts was used as control. The number of worms alive on the individual wells was scored and counted every 24 h and was plotted, similar to the lifespan assay.
2.10Paralysis assay
The Aβ transgenic strain was treated with different doses of S. asper extract (10–30μg/ml) and closely monitored under the microscope. The worm was considered paralyzed if it does not show any body movements when touched with a platinum loop. Imaging was done using differential interference contrast (DIC) microscopy [38].
2.11Western blotting
The Aβ transgenic strain was treated with different doses of S. asper extract (10–30μg/ml), and then the total protein was isolated using 1 mM PMSF in PBS buffer. Total protein (50 μg) was separated using 12 % sodium dodecyl sulfate-polyacrylamide gel electrophoresis (SDS-PAGE) after it was mixed with a 2×Laemmli buffer (ratio 1:1) and heated at 95 °C for 5 min. The separated proteins were transferred onto polyvinylidene difluoride (PVDF) membranes, which were then blocked with 5 % nonfat milk for 1 h at room temperature. Membranes were incubated with primary antibodies (Aβ (1:2000) or β actin (1:2000)) overnight at 4 °C. After incubation, membranes were washed three times with 1×TBS-Tween 20 (TBST) for 5 min each, then incubated with secondary antibodies (anti-rabbit IgG, HRP-linked antibody) for 45 min at room temperature and washed three times with TBST for 5 min each. Protein bands were visualized by adding an enhanced chemiluminescence detection reagent and exposure to autoradiography film. Each band was normalized against β actin as an internal control [39].
2.12Total RNA isolation and real-time PCR analysis
The nematodes were treated with varying concentrations of S. asper extracts (10–30μg/ml), and after the treatment period total RNA was isolated using Trizol kit (Invitrogen, Carlsbad, CA, USA). From this, 1000 ng was reverse transcribed to cDNA using Accupower RT Premix (Bioneer, Korea) and oligo dT primers through following the manufacturer’s protocol. Gene-specific primers were designed (Table 1) to carry out Real-time PCR using SYBR Green. The Green Star PCR Master Mix (Bioneer, Korea) was used in the Exicycler Real-Time Quantitative Thermal Block (Bioneer, Korea). The expression data were normalized to the internal control actin and then represented as upregulated or downregulated by normalizing with untreated control [31].
Table 1
List of Primers used
Gene Name | Forward Primer | Reverse Primer |
daf-2 | TCGAGCTCTTCCTACGGTGT | CATCTTGTCCACCACGTGTC |
age-1 | ATAGAGCTCCACGGCACTTT | ATAGAGCTCCACGGCACTTT |
utx-1 | GCAGAACACCAGCTCATCAG | ATCAACGCCATTCTTCTCGC |
col-19 | CACACAAATGCTCCACCAAC | CTGGATTTCCCTTCTGTCCA |
egl-8 | CGTATCGTTGCGCTTCTCA | AGTAGTGACACAGCGGTTG |
egl-30 | TCAGAAAGGCGGAAGTGGAT | GGTTCTCGTTGTCACACTCG |
dgk-1 | GTTGGGGAAGTGGTGCAAAT | GCGAGCTTGGATTGGATGAG |
goa-1 | TGTTCGATGTGGGAGGTCAA | TCGTGCATTCGGTTTGTTGT |
skn-1 | ATCCATTCGGTAGAGGACCA | GGCGCTACTGTCGATTTCTC |
sir-2.1 | CGGGGAAGTGCAAGAAATAA | GAGTGGCACCATCATCAAGA |
act-2 | ATCGTCCTCGACTCTGGAGATG | TCACGTCCAGCCAAGTCAAG |
2.13Statistical analysis
One-way ANOVA (SPSS 17) was used to compare each treatment’s mean values in every experiment unless otherwise specified. The data were represented as the average of at least three independent experiments. Significant differences between the means of parameters were determined by using Duncan’s test (P < 0.05) comparing the groups’ control vs treated [37].
3Results
3.1S. asper extended the lifespan of C. elegans
The lifespan extension activity of S. asper extract was tested for varying concentrations ranging from 1–100μg/ml. Almost all the tested concentrations were able to extend the lifespan of wild type C. elegans (Fig. 1) significantly (p < 0.05), in which the doses ranging between 10–70μg/ml exhibited maximum extension of lifespan which was up to 28, 30, 29, 28, 28, 28 and 28 days respectively (Fig. 1). The control worms were fed with E. coli OP50 alone, and the vehicle control, the worms were treated with DMSO. Both the control and vehicle control exhibited similar lifespan as they survived up to 22 days (Fig. 1). This indicates that the solvent-induced no change in mediating lifespan extension by the extract. The concentrations 10–30μg/ml showed the maximum survival rate and were used for further experimental analysis.
Fig. 1
S. asper extract could extend the maximum lifespan in C. elegans. One-way ANOVA (SPSS 17) was used to compare each treatment’s mean values in every experiment, and the data were represented as the average of the independent experiments. (A) Wild type nematodes treated with varying concentrations of S. asper extract (1–100μg/ml) could significantly (p < 0.05) extend the maximum lifespan of the nematodes. Control worms that did not receive any extract treatment and the vehicle control DMSO treated worms survived up to 22 days (B) Doses ranging from 10–30μg/ml that exhibited maximum extension of lifespan were represented wherein they survived up to 28, 30 and 29 days respectively which were used for the further experiments.
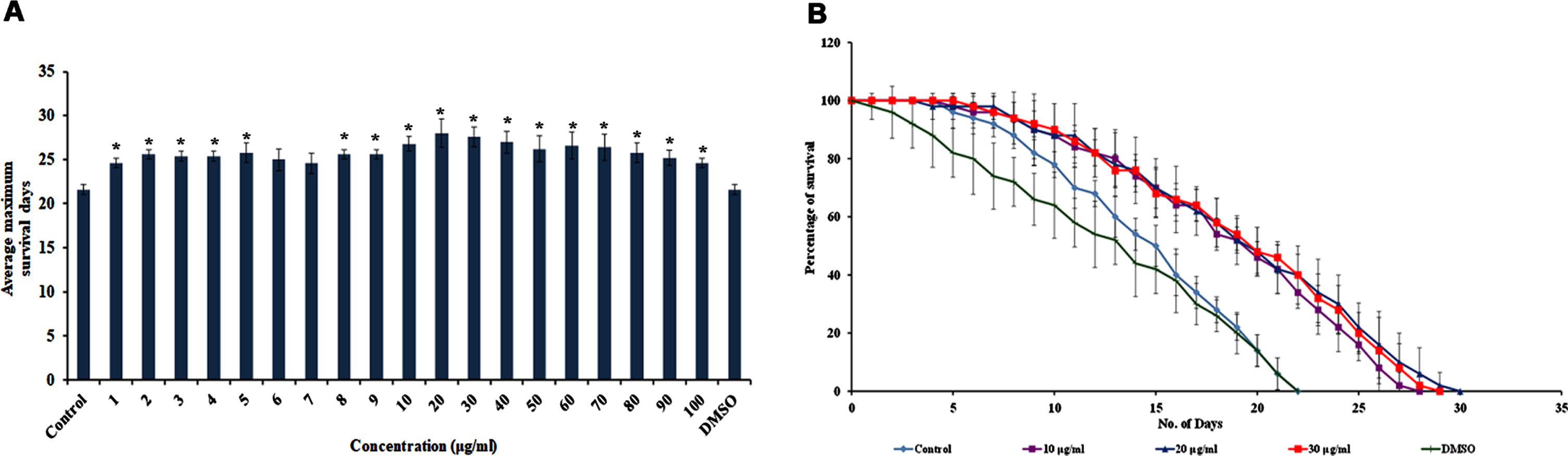
3.2S. asper improved the healthspan of C. elegans
Pharyngeal pumping was analyzed in C. elegans treated with 10–30μg/ml of S. asper extract. It was observed that the extract did not alter the pharyngeal pumping of the nematodes when compared to the untreated control, which was consistent up to 10 days (Fig. 2A).
Fig. 2
S. asper could extend the healthspan of C. elegans (A) Pharyngeal pumping was observed in wild type nematodes fed with 10–30μg/ml of S. asper extract. No significant changes were observed in the feeding pattern of worms treated or untreated with S. asper extract (B) Analysis of the relative fluorescence intensity of nematodes treated with 10–30μg/ml of S. asper extract in which 20μg/ml concentration showing significant (p < 0.05) reduction in fluorescence when compared to control (n = 10) (C) Representative image of wild type nematode with no extract treatment (control) with the level of lipofuscin accumulation (D–F) Representative images of wild type nematodes with 10–30μg/ml of S. asper extract with the level of lipofuscin accumulation (G) Real-Time PCR analysis of col-19, egl-8, egl-30, dgk-1 and goa-1 was done in nematodes treated with 10–30μg/ml of S. asper extract. The expression of col-19, dgk-1 and goa-1 were observed to be downregulated, whereas the expression of egl-8 and egl-30 was upregulated compared to untreated control normalized to the X axis.
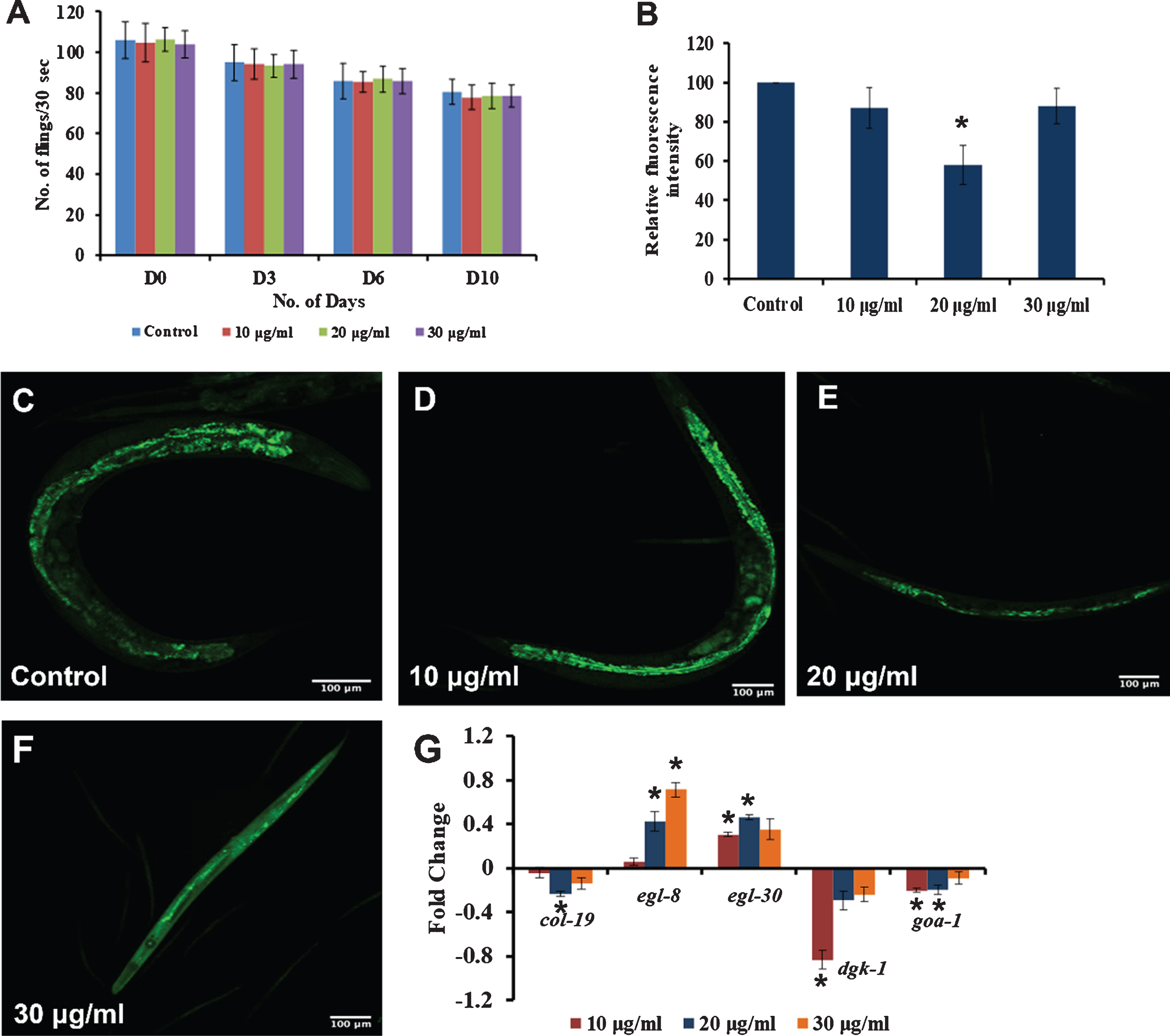
The level of autofluorescent protein, the “age-pigment” lipofuscin, was monitored inside the nematodes after treatment with 10–30μg/ml of S. asper extract. All the tested concentrations showed a reduction in the fluorescence levels and a significant (p < 0.05) reduction was observed in 20μg/ml concentration when compared to control (Fig. 2B), indicating that the extract was successful in slowing down the aging process, as it could reduce the accumulation of lipofuscin.
Further, qPCR analysis was performed on candidate genes that could mediate healthspan. Candidate genes of the Diacylglycerol (DAG) pathway were monitored, namely egl-8 and egl-30 that positively regulate healthspan and dgk-1 and goa-1, negatively regulate healthspan. Additionally, the level of expression of an adult-specific marker, col-19 was also observed. The expression of egl-8 and egl-30 were observed to be upregulated significantly (p < 0.05) whereas the expression of col-19, dgk-1 and goa-1 were downregulated significantly (p < 0.05) in the worms treated with 10–30μg/ml of S. asper extract when compared to control (Fig. 2G). The results indicate that S. asper extract could positively improve the healthspan of the nematodes.
3.3S. asper mediated extension of lifespan and healthspan is independent of DAF-16 pathway
Some of the key players of the DAF-16 mediated pathway were analyzed in nematodes treated with 10–30μg/ml of S. asper extracts using qPCR analysis. It was observed that the expression of daf-2, age-1 and utx-1 were upregulated significantly (p < 0.05) in a concentration-dependent manner when compared to control, suggesting that the DAF-16 mediated pathway may not play a role in S. asper mediated extension of lifespan (Fig. 3A).
Fig. 3
S. asper mediated lifespan extension is independent of DAF-16 pathway (A) Real-Time PCR analysis of daf-2, age-1 and utx-1 was done in nematodes treated with 10–30μg/ml of S. asper extract. All the genes were upregulated in a dose-dependent manner when compared to untreated control which was normalized to the X-axis (B) Maximum lifespan of daf-16 mutants (mu86) were significantly (p < 0.05) extended when treated with 10–30μg/ml of S. asper extract at 15 °C (C) Graph representing the average of maximum lifespan extension of daf-16 mutants when treated with 10–30μg/ml of S. asper extract (D) Maximum lifespan of daf-2 mutants (e1370) were significantly (p < 0.05) extended when treated with 10–30μg/ml of S. asper extract at 15 °C (E) Graph representing the average of maximum lifespan extension of daf-2 mutants when treated with 10–30μg/ml of S. asper extract.
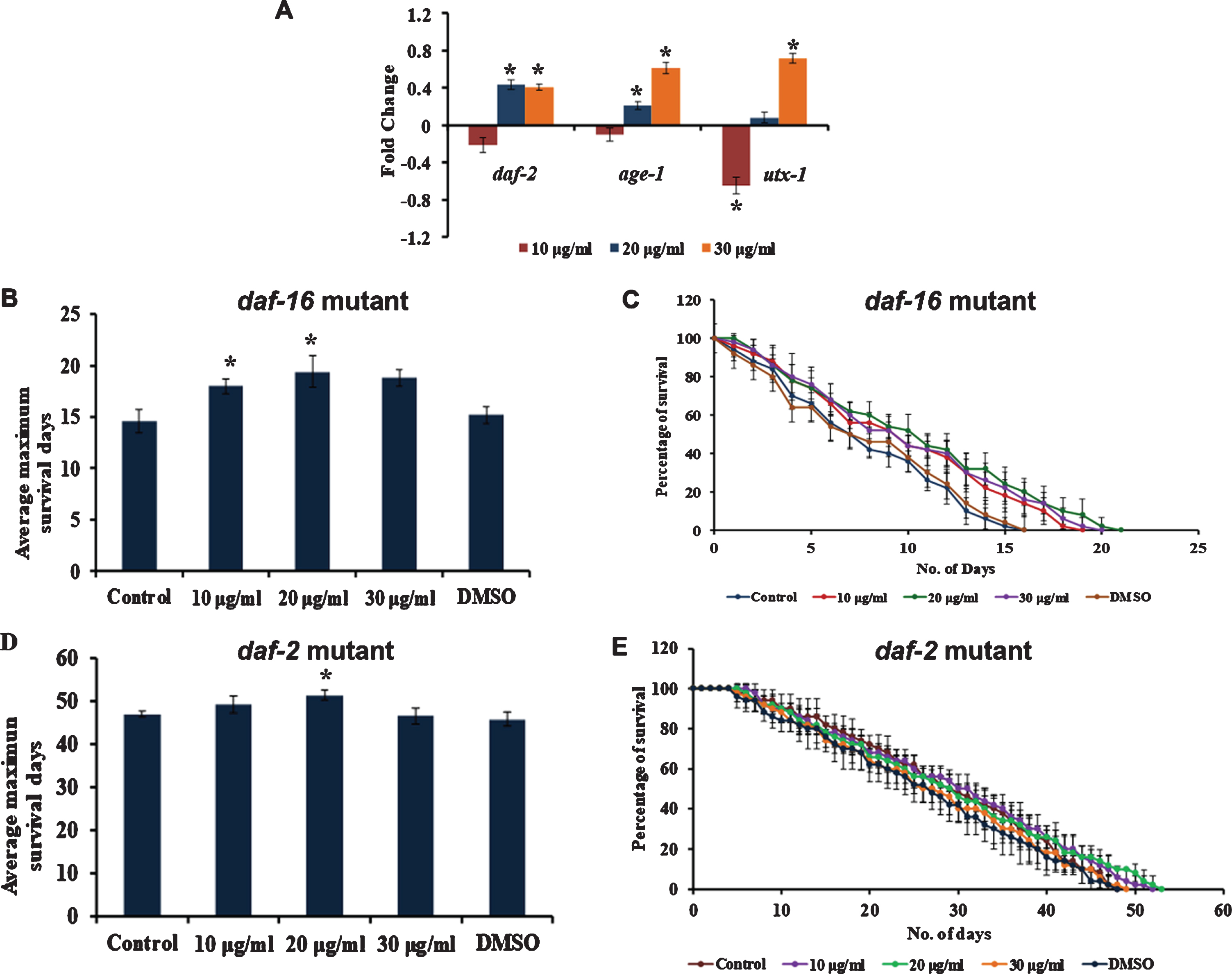
To further confirm this, mutants of DAF-16 and DAF-2 were treated with 10–30μg/ml of S. asper extract, and the survival rate was monitored. It was observed that there was a significant (p < 0.05) increase in the lifespan in both DAF-16 mutants at 10 and 20μg/ml as they survived up to 19, 21 and 20 days respectively when compared to 16 days survived by control (Fig. 3B-C) as well as in DAF-2 mutants at 20μg/ml as they survived up to 52, 53 and 49 days respectively when compared to 48 days survived by control (Fig. 3D-E) worms. This suggests that the lifespan extension exhibited by S. asper extracts was not mediated by DAF-16 pathway, and there could be some other mechanism mediating the effect.
3.4S. asper mediated lifespan extension, and stress resistance is mediated by MAPK pathway
Mitogen-Activated Protein Kinase (MAPK) pathway plays a major role in stress resistance and extending the nematodes’ lifespan. To analyze whether S. asper has a role in activating MAPK signalling, mutants of PMK-1 were treated with S. asper extracts. It was observed that the extract could not induce any significant change in the lifespan of the mutant strains (Fig. 4), indicating the importance of PMK-1 in mediating the effect of S. asper extracts in the nematodes.
Fig. 4
S. asper mediated lifespan extension is dependent of MAPK pathway (A) Maximum lifespan of pmk-1 mutants were showing no significant change when treated with 10–30μg/ml of S. asper extract (B) Graph representing the average of maximum lifespan extension of pmk-1 mutants when treated with 10–30μg/ml of S. asper extract.
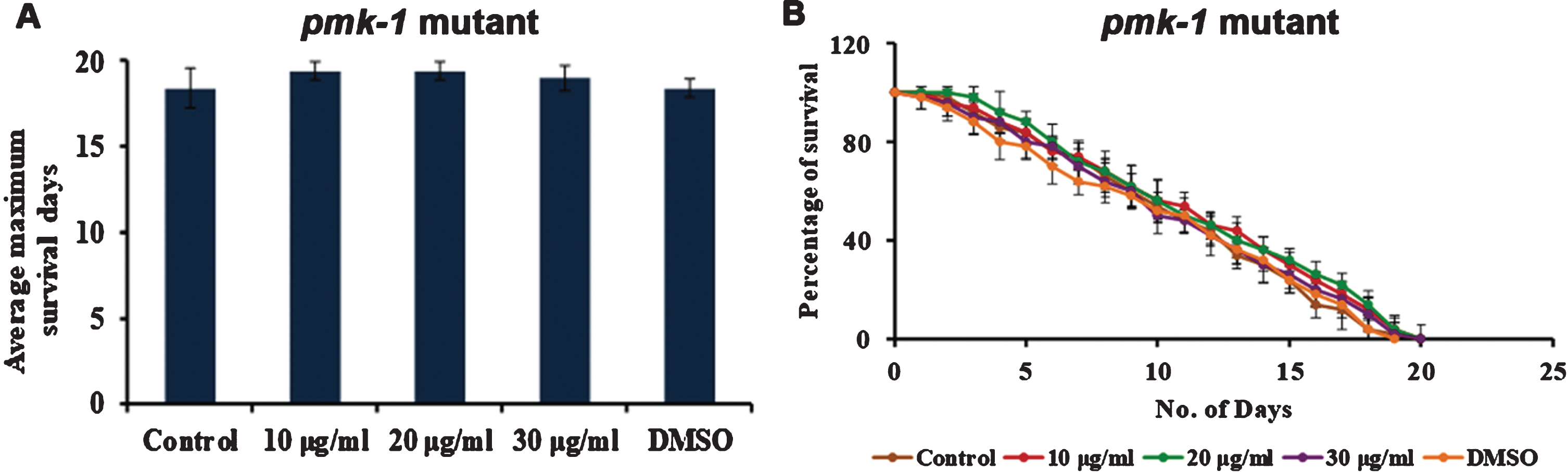
3.5S. asper can induce antioxidant potential in C. elegans
Since S. asper has been reported to possess antioxidant potential in vitro, [19], the extract’s ability to induce the same under in vivo conditions was analyzed through qPCR analysis of candidate genes, skn-1 and sir-2.1, that are responsible for the antioxidant mechanism in C. elegans. The significant (p < 0.05) upregulation of both the genes at 10 and 20μg/ml upon treatment with 10–30μg/ml of S. asper extract treatment suggest that the extract could activate the antioxidant mechanism in the nematode (Fig. 5).
Fig. 5
S. asper extract can activate antioxidant potential in C. elegans. (A) Representative image of skn-1::GFP transgenic strain (Control) (B-D) Representative image of skn-1::GFP transgenic strain treated with 10–30μg/ml of S. asper extract (E) Quantification of fluorescence wherein 20μg/ml showed maximum expression (F) Real-Time PCR analysis of skn-1 and sir-2.1 was done in nematodes treated with 10–30μg/ml of S. asper extract that showed significant (p < 0.05) upregulation at 10 and 20μg/ml concentrations when compared to untreated control which was normalized to the X-axis.
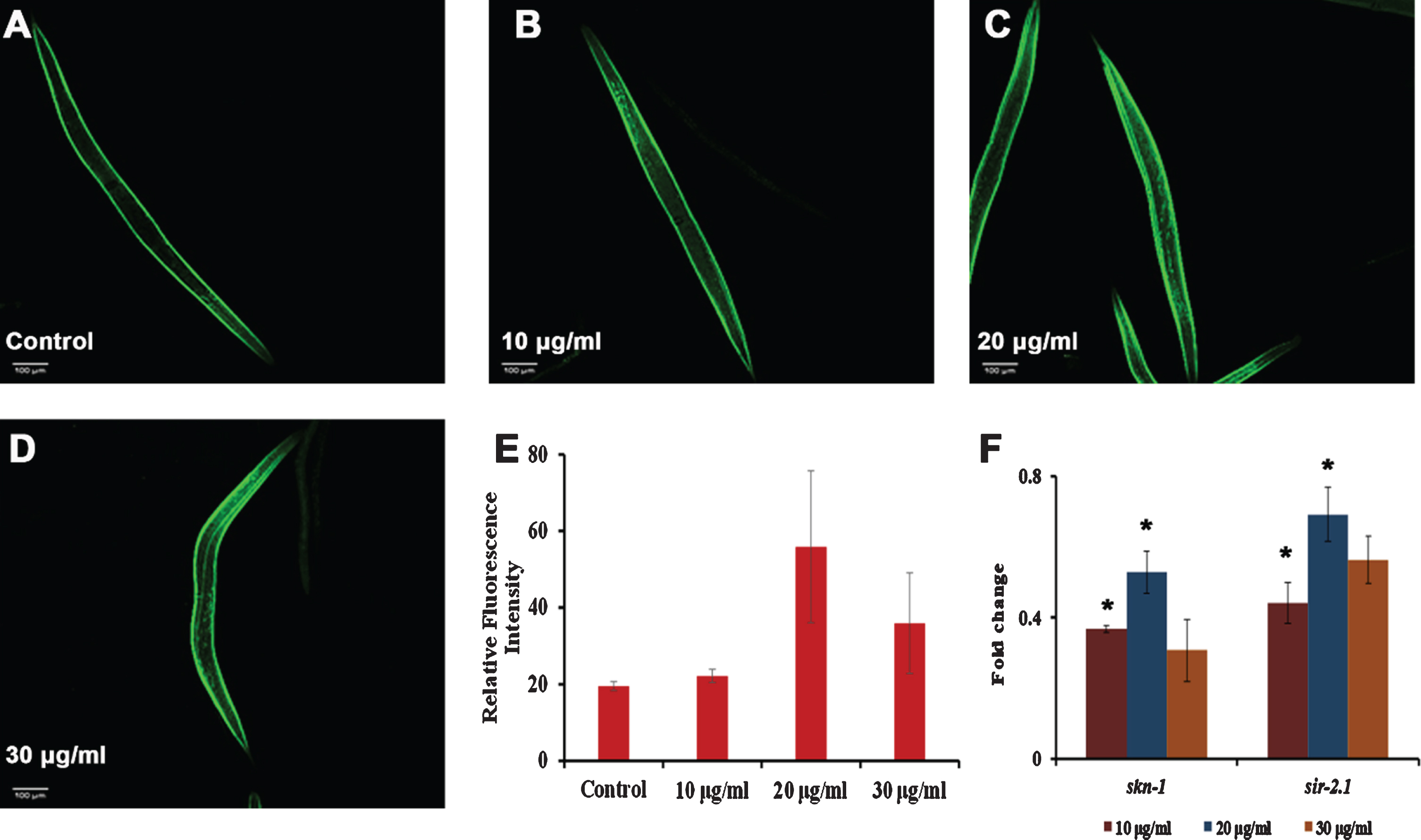
Additionally, to analyze the level of skn-1 in the nematode after S. asper treatment, skn-1::GFP transgenic strains were monitored for their fluorescence intensity. It was observed that the extract treatment improved the fluorescence intensity when compared to the control wherein 20 and 30μg/ml of S. asper extract showed maximum effect (Fig. 5).
3.6S. asper can reduce the level of photoaging in C. elegans
The anti-photoaging effect was monitored by analyzing the effect of S. asper extracts on the lifespan of UV-A exposed worms. The extract was analyzed for its protection and repair effects by treating the extracts before and after the exposure to UV-A individually. There was a significant (p < 0.05) extension of lifespan in worms pre-treated and post-treated with the extracts when compared to the worms which were exposed to UV-A without any treatment (Fig. 6A-C). This suggests that the extract possesses anti-photoaging activity along with antioxidant potential.
Fig. 6
S. asper extract could activate antioxidant and anti-photoaging potential (A) Wild type nematodes were exposed to UV-A. S. asper extract was treated before (pre-treatment) or after (post-treatment) UV-A exposure. Significant (p < 0.05) extension of lifespan was observed in both kind of treatments when compared to nematodes exposed to UV-A without any treatment (B) Graph representing the average maximum lifespan of nematodes pre-treated with UV-A exposure (C) Graph representing the average maximum lifespan of nematodes post-treated with UV-A exposure (D) Representative image of worm showing the level of ROS that was not exposed to UV-A and did not receive any treatment (negative control) (E) Representative image of worm showing the level of ROS that was exposed to UV-A and did not receive any treatment (positive control) (F–H) Representative image of worm showing the level of ROS that was exposed to UV-A and was treated with 10–30μg/ml of S. asper extract before exposure (pre-treatment) (I–K) Representative image of worm showing the level of ROS that was exposed to UV-A and was treated with 10–30μg/ml of S. asper extract after exposure (post-treatment) (L) Analysis of the relative fluorescence intensity of nematodes pre and post-treated with 10–30μg/ml of S. asper extract (n = 10).
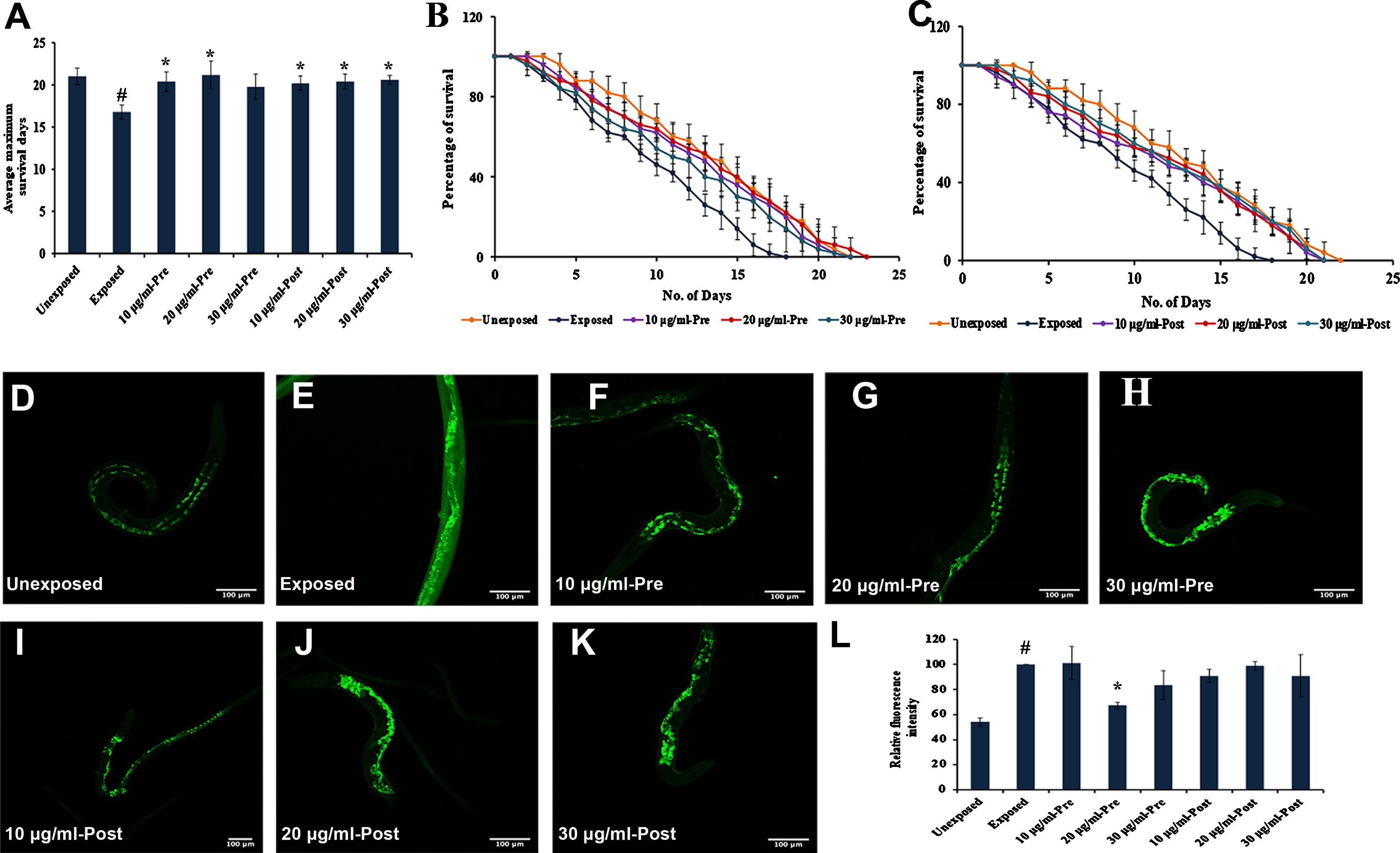
As UV-A causes ROS induced photoaging, oxidative stress was induced in C. elegans by exposing to UV-A for 4 h [40]. The extract was analyzed for its protection and repair effects by treating the extracts to nematodes before and after UV-A exposure individually. A significant (p < 0.05) reduction in the level of ROS was observed, which could be evident from the reduction in fluorescence intensity in the extract pre-treated with 20μg/ml of S. asper extract (Fig. 6L). The post-treated group also showed a reduction in fluorescence which is not as significant as the pre-exposed ones. The results indicate that the extract exhibits substantial antioxidant properties and could protect the nematodes from UV-A induced photoaging.
Additionally, the superoxide dismutase (SOD) level was analyzed in the whole protein of C. elegans exposed to UV-A pre- and post-treated with S. asper extract as described above. It was observed that UV-A triggered the level of SOD inside the nematode, which was reduced by the treatment of S. asper wherein 20μg/ml pre-treatment exhibited significant reduction (Fig. 7).
Fig. 7
S. asper extract can regulate the level of SOD in C. elegans. Wild type nematodes were exposed to UV-A. S. asper extract was treated before (pre-treatment) or after (post-treatment) UV-A exposure. Analysis of the relative fluorescence intensity of worm lysate (50μg) pre- and post-treated with 10–30μg/ml of S. asper extract. 20μg/ml pre-exposed concentration showed a significant (p < 0.05) reduction in the level of SOD (# control vs UV exposed; *UV exposed vs extract treated).
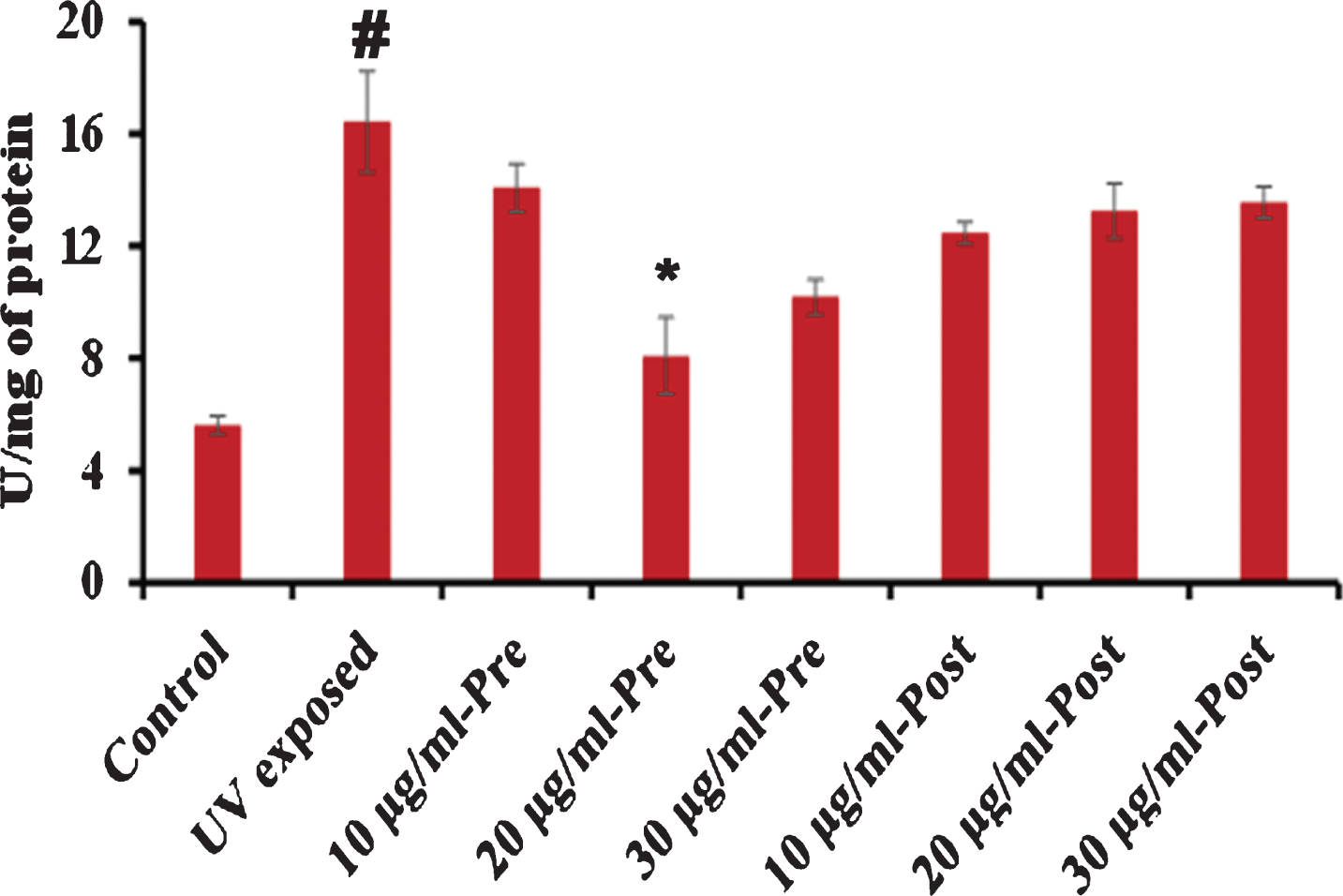
3.7S. asper imparts neuroprotection in transgenic C. elegans
As antioxidants are known to impart neuroprotection, the neuroprotective effect of S. asper extract was analyzed using a transgenic strain of C. elegans, CL2006 that expresses Aβ1–42 constitutively by treating with 10–30μg/ml of S. asper extract to monitor its survival. The extract was observed to significantly (p < 0.05) extend the lifespan of the transgenic nematodes at 20 and 30μg/ml as they survived up to 25, 26 and 26 days when compared to control which survived up to 23 days. Additionally, the extract was able to protect the worms from being paralyzed due to the accumulation of Aβ inside the worm. Western blot analysis further suggests a significant reduction in Aβ load inside the worm after the treatment with 20μg/ml S. asper extract (Fig. 8) suggesting the neuroprotective potential of the extract.
Fig. 8
S. asper extract can impart neuroprotection in C. elegans (A) Maximum lifespan of Aβ transgenic strain were significantly (p < 0.05) extended at 20 and 30μg/ml when treated with 10–30μg/ml of S. asper extract (B) Graph representing the average of maximum lifespan extension of Aβ transgenic strain when treated with 10–30μg/ml of S. asper extract (C) Representative image of Aβ transgenic strain which is paralyzed (control) (D-F) Representative image of Aβ transgenic strain treated with 10–30μg/ml of S. asper extract which is not paralyzed (Paralysed worm –straight line; non-paralysed worm –“C” or “S”- shaped) (G) S. asper extract significantly reduced the expression of Aβ oligomer. Lane 1 (control) Lane 2 (10μg/ml S. asper extract) Lane 3 (20μg/ml S. asper extract) Lane 4 (30μg/ml S. asper extract) (H) Quantitative measurement of the relative density of Aβ to β-actin expression (*P < 0.05 compared between control and S. asper treated group).
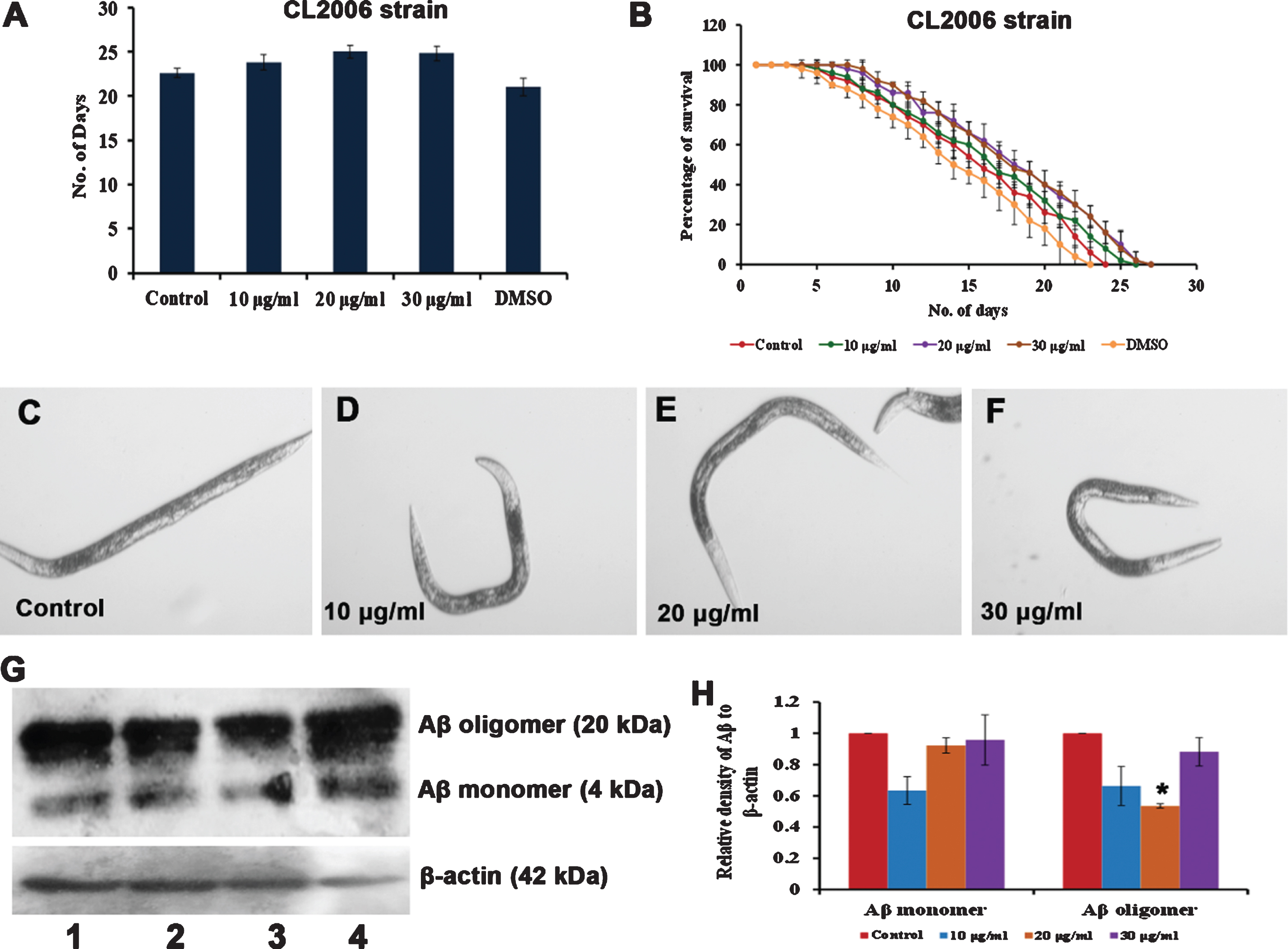
4Discussion
Aging is an irreversible, progressive and degenerative process causing damage to tissues and organs, leading to an increased level of oxidative stress coupled with different age-associated diseases, eventually leading to death [23, 26]. This has always led researchers to strive hard to identify molecules that can target the molecular pathways to delay aging and its associated diseases, which further improves the overall health of the organism, thereby improving lifespan [41]. Since many natural sources such as plants and their derivatives pose limited to negligible side effects, they are gaining more attention to be a strong contender for anti-aging research [9, 42]. S. asper, a plant used in traditional medicine, has been identified to have neuroprotective effects against glutamate-induced cytotoxicity in HT22 cells by reducing ROS level via activation of Nrf2. Additionally, the extract was also able to extend the lifespan of the larval stages of C. elegans, indicating its anti-aging potential [19].
Extension of lifespan and healthspan is essential for any formulation to claim its anti-aging potential. S. asper has already been reported to be used in Thai traditional formula for longevity and antioxidant potential [43]. As in previous reports, C. elegans fed with 1–100μg/ml of S. asper extract were observed to have extended the nematode’s lifespan (Fig. 1). The extension in lifespan may be due to the phytochemicals andrographolide, carnosic acid, α-linolenic acid, and oleoyl oxazolopyridine and other phytochemicals in the extract [19, 44, 45]. Carnosic acid and α-linolenic acid have been reported to elicit anti-aging potential in C. elegans, which could be dependent on SKN-1 [46, 47].
Dietary restriction is a critical mechanism that can also extend lifespan in different models, including C. elegans by mediating signalling pathways and maintaining the cellular energy balance [48]. A pharyngeal pumping assay was performed to confirm that the worms feed on S. asper and the lifespan extension was not because of dietary restriction. It was observed that the worms were able to feed upon the extract in a similar way to that of its E. coli OP50 and there was no significant change in the pumping rate between the control and extract-treated group (Fig. 2A), indicating that the worms could consume the extract and that the extension of lifespan was not induced by dietary restriction process.
The autofluorescent age pigment, lipofuscin gets accumulated in C. elegans during the aging process; wherein, the accumulation will initially start in the intestinal tract and uterus area and further develop into other parts of the body [49, 50]. A reduction of the level of lipofuscin by small molecules indicates the reversal of aging [51, 52]. It is understood that during aging, an increase in lipofuscin is further involved in limiting the removal of oxidized proteins and induce the formation of ROS and leading to apoptosis [53]. Recent studies of plant extracts also correlate the reduction of lipofuscin level to anti-aging potential [31, 54, 55]. Corroborating to the previous reports, S. asper extract was found to exhibit anti-aging potential in C. elegans, as it could significantly reduce the level of lipofuscin inside the nematode (Fig. 2B-F).
Further, to validate the activation of healthspan by S. asper extract, qPCR analysis of candidate genes that could mediate the nematode’s overall health was monitored. The adult-specific marker of C. elegans, col-19 [24, 56] was observed to be downregulated significantly (p < 0.05) (Fig. 2G) suggesting the delay in the aging process. Candidate genes of the Diacylglycerol (DAG) pathway that mediates healthspan, including pharyngeal pumping, locomotion and egg-laying [24, 57] were monitored. The significant (p < 0.05) upregulation of egl-8 and egl-30 along with the significant (p < 0.05) downregulation of dgk-1 and goa-1 (Fig. 2G) in line with their role in the aging mechanism. Both egl-8 and egl-30 are essential for the activation of healthspan, whereas dgk-1 and goa-1 negatively regulate healthspan [58–62]. This suggests the activation of healthspan inside the nematodes treated with S. asper, which goes in parallel to a recent report from our group [31].
Further, the study focused on understanding the molecular mechanism by which S. asper mediates the anti-aging effects. The conserved insulin/IGF-1 signalling (IIS) pathway, which is generally known as DAF-16 pathway in C. elegans is a well-known pathway to mediate anti-aging and stress resistance in nematodes [28, 54, 55, 63]. The regulation of candidate genes of the pathway, daf-2, age-1 and utx-1, which could negatively regulate anti-aging and stress resistance in C. elegans [24, 54, 64] were analyzed through qPCR approach. Both daf-2 and age-1 are negative regulators of lifespan and the histone demethylase utx-1 acts upstream of daf-2 and negatively regulates lifespan [25, 64, 65]. It was observed that all three genes were upregulated significantly (p < 0.05) when treated with S. asper extract in a dose-dependent manner (Fig. 3A) indicating that the anti-aging mechanism exhibited by S. asper could be independent of the pathway.
The qPCR analysis results were further confirmed with studies using daf-16 and daf-2 mutants short-lived and long-lived, respectively [65]. The significant (p < 0.05) increase in lifespan of both the mutants treated with S. asper (Fig. 3B-E) further confirm that the anti-aging effect could be independent of DAF-16 pathway. A traditional uyghur medicine, aiweixin, was identified to extend lifespan independent of DAF-16 [66]. Another report suggests that lifespan extension by tryptophan in C. elegans was independent of DAF-16 pathway [67].
To identify the alternate pathway through which S. asper exerts its anti-aging effects, the MAPK pathway in C. elegans was analyzed using mutants of pmk-1, the downstream effector of MAPK pathway. It was recently reported that the MAPK pathway could elicit anti-aging effect in C. elegans exposed to UV-A [68]. Several extracts and phytochemicals have already been reported to extend longevity in nematodes by acting through MAPK pathway. Mulberry anthocyanins and sesamin were observed to extend lifespan in C. elegans, dependent on MAPK mediated by pmk-1 and skn-1 [69, 70]. In a similar fashion to these reports, the extract could not induce any significant change in the lifespan of the nematode (Fig. 4) indicating the role of MAPK in exerting the anti-aging properties for S. asper in C. elegans. Andrographolide, the antioxidant present in S. asper has been previously reported to activate the JNK MAPK pathway in human neuroblastoma cells against Parkinson’s disease [71].
The mammalian Nrf-2 acts as a transcription factor of MAPK. On that note, qPCR analysis of the genes skn-1 and sir-2.1, the mammalian orthologs of Nrf-2 and SIRT-1 respectively were analyzed. Previous reports suggest that SKN-1 acts to extend longevity and oxidative stress resistance [72–74], whereas sir-2.1 was crucial in eliciting antioxidant potential by green tea [37] and black tea [75]. It was observed that both the genes were significantly (p < 0.05) upregulated (Fig. 5), suggesting the activation of the antioxidant mechanism. Additionally, the skn-1::GFP strains showed an increase in fluorescence when treated with S. asper extract, indicating the activation of skn-1 by the extract, which can reduce the level of oxidative stress [35, 76]. It is also important to note that MAPK aids in the nuclear localization of SKN-1 during oxidative stress response [77]. Previously, S. asper extract has been reported to induce the expression of Nrf-2 in HT22 cells and protect cells from glutamate-induced oxidative stress [19]. Further the bioactive compounds in S. asper including, carnosic acid and taxifolin were also found to activate Nrf-2 [78–80]. In the present study, the activation of skn-1 could have played a key role in preventing the ROS formation and increasing the nematodes’ lifespan.
C. elegans are prone to a spike in oxidative stress when exposed to UV-A, leading to photoaging, which is dependent on MAPK and SKN-1 [40, 68]. To monitor S. asper extract’s efficacy in protecting and treating the nematode from oxidative stress, C. elegans were treated with the extracts before and after exposure individually. A significant (p < 0.05) reduction of fluorescence in the nematodes pre-treated with the extract was observed (Fig. 6D-L) suggesting that the extract could protect the nematode from oxidative stress. Similarly, another study showed that a water-soluble form of andrographolide suppressed the harmful effects of UV exposure in mice skin and protected the accumulation of oxidative stress [81]. Taxifolin in black rice extract reduced the UV induced ROS level and collagen damage, which is mediated by JNK in HaCaT cells [82].
Parallel to this, nematodes exposed to UV-A and treated with the extract before and after exposure individually were monitored for their overall lifespan. It was observed that the nematodes treated with the extract before or after the exposure to UV-A could significantly (p < 0.05) extend the lifespan of the nematode when compared to the worms exposed to UV-A without any treatment (Fig. 6A-C). Interestingly, the worms pre-treated with the extract exhibited longer lifespan than the worms that received post-treatment, in line with the previous data, indicating that the protective effect of S. asper against oxidative stress was much better than the treatment effect.
The increase in the level of oxidative stress led to an increase in the level of SOD inside the worms. This could be attributed to the host’s counteractive mechanism to reduce the level of oxidative stress [36, 83]. In the present study, the UV-A exposed worms showed an increase in the level of SOD, which was significantly reduced by the 20μg/ml pre-treated S. asper extract (Fig. 7). A recent study in C. elegans suggests a decrease in the level of SOD could be because of oxidative stress detoxification [84].
Aging dependent oxidative stress poses one of the major threats for neurodegenerative diseases such as Alzheimer’s disease. The use of antioxidant-based therapies is thus gaining prominence in treating these diseases [85]. A recent study in Neuro2a cells highlights the importance of Nrf2 in reducing the toxicity induced by Aβ [86]. Nutritional interventions using antioxidants in diet could positively affect the health of older people susceptible to age-related neurodegenerative diseases [87]. Neuroprotective efficacy of S. asper against glutamate-induced oxidative toxicity has already been reported in vitro [19]. In the present study, a transgenic strain of C. elegans, with neurological defects induced by Aβ were treated with S. asper extracts. It was observed that the extract could significantly (p < 0.05) extend the lifespan of the transgenic strain and reduce the rate of paralysis. The transgenic strain for the Alzheimer’s disease CL2006 expresses and accumulates intracellular Aβ leading to enhanced paralysis [38, 88]. S. asper extract downregulated the expression of Aβ in the nematodes thereby delaying the paralysis contributing to the neuroprotective effects and increased lifespan. The lifespan extension property of the extract can be attributed to its antioxidant activity, as the antioxidants identified in S. asper such as andrographolide, α-linolenic acid and strebluslignanol were able to impart neuroprotection in different models [71, 89–91]. Moreover, andrographolide was also able to reduce the total Aβ load and astrogliosis and interleukin-6 levels in the brains of aged Octodon degus [92].
The present study can be of potential interest in considering S. asper as an emerging candidate for anti-aging and neuroprotection in higher model systems and humans. However, the overall pharmacokinetic activity, including the absorption and metabolism of the compounds in the plant also needs to be analyzed. Available literature suggests the activity of some of the compounds present in S. asper in different systems, which could help in a better understanding of the role of the compounds inside the host.
Andrographolide was quickly and almost completely absorbed into the blood in rats following the oral administration of Andrographis paniculata which was dose dependent [93]. Among this, the highest concentration was observed in kidney, followed by heart, lungs, brain and plasma [94]. Carnosic acid is absorbed into the bloodstream of rats after oral administration which makes it bioavailable in intestine, liver and muscle tissues of abdomen and legs and was eventually eliminated through fecus [95]. The naturally enriched goat diary fat containing alpha-linoleic acid when administered orally in rats, the maximum bioavailability was observed in liver followed by plasma and erythrocytes [96]. Taxifolin can be absorbed into the blood of rats after oral administration, however hepatic fibrosis affects the pharmacokinetics of taxifolin indicating personalized dosage must be followed for individuals with liver diseases [97]. From the available reports, it can be said that the phytochemicals of S. asper are rapidly absorbed and distributed throughout various tissues via circulation to exert systemic effects. Further, earlier reports on the acute (2000 mg/kg body weight) and sub-chronic toxicity (400 mg/kg body weight) studies have revealed that S. asper extract is devoid of any toxicities indicating its safety [98].
Overall, the results indicate that S. asper can exert multi-faceted effects and play a major role in extending lifespan and healthspan apart from improving stress resistance and neuroprotection in C. elegans from photoaging and Aβ induced toxicity. A recent study in C. elegans reported that carnosic acid, a major phytochemical in S. asper, can mediate lifespan, healthspan, stress resistance and neuroprotection via SKN-1 and SEK-1 (another player in MAPK), but independent of DAF-16 pathway [46]. Another polyphenol, α-linolenic acid, was also reported to extend lifespan and extend neuroprotection which was dependent on both NHR-49 and SKN-1 transcription factors [47, 99] suggesting the importance of SKN-1 in S. asper mediated health benefits.
5Conclusions
To summarize, S. asper was observed to be non-toxic and could extend lifespan and healthspan in C. elegans. It also protected the nematodes from aging and photoaging via MAPK pathway and SKN-1. Also, the extract offered neuroprotection against Aβ induced toxicity in nematodes. Our results suggest that S. asper acts as a multi-factorial agent and can be considered as a promising lead in anti-aging and neuroprotective studies. Further studies in this area will shed more light on the mechanisms through which S. asper acts in extending health benefits to the host.
Acknowledgments
M.I.P. and J.M.B. wish to thank the Ratchadaphiseksomphot Endowment Fund for Postdoctoral Fellowship and Chulalongkorn University, Thailand, for the support. D.S.M wishes to thank the Second Century Fund (C2F), Chulalongkorn University, Thailand, for the support. The study was partially supported by Grants for Development of New Faculty Staff, Ratchadaphiseksomphot Endowment Fund, Chulalongkorn University, Thailand. The authors would like to thank Natural Products for Neuroprotection and Anti-ageing Research Unit and Ratchadaphiseksomphot Endowment Fund for the support.
Funding
This study was supported by a Grant from the Ratchadaphiseksomphot Endowment Fund (TT).
Conflict of interest
The authors declare no conflict of interest.
References
[1] | Nielsen E , Temporiti MEE , Cella R . Improvement of phytochemical production by plant cells and organ culture and by genetic engineering. Plant Cell Rep. (2019) ;38: (10):1199–1215. |
[2] | Schaal B . Plants and people: Our shared history and future. Plants People Planet. (2019) ;1: :14–9. |
[3] | Van Wyk BE . A family-level floristic inventory and analysis of medicinal plants used in Traditional African Medicine. J Ethnopharmacol. (2020) ;249: :112351. |
[4] | Kumar A , Aswal S , Chauhan A , Semwal RB , Kumar A , Semwal DK . Ethnomedicinal Investigation of Medicinal Plants of Chakrata Region (Uttarakhand) Used in the Traditional Medicine for Diabetes by Jaunsari Tribe. Nat Prod Bioprospect. (2019) ;9: (3):175–200. |
[5] | Lemonnier N , Zhou GB , Prasher B , Mukerji M , Chen Z , Brahmachari SK , Noble D , Boutron B , Auffray C , Sagner M . Traditional knowledge-based medicine: A review of history, principles, and relevance in the present context of P4 systems medicine. Prog Prev Med. (2017) ;2: :e0011. |
[6] | Mani JS , Johnson JB , Steel JC , Broszczak DA , Neilsen PM , Walsh KB , Naiker M . Natural product-derived phytochemicals as potential agents against coronaviruses: a review. Virus Res. (2020) ;284: :197989. |
[7] | Hügel HM . Brain Food for Alzheimer-Free Ageing: Focus on Herbal Medicines. Adv Exp Med Biol. (2015) ;863: :95–116. |
[8] | Prasanth MI , Sivamaruthi BS , Chaiyasut C , Tencomnao T . A review of the role of green tea (Camellia sinensis) in antiphotoaging, stress resistance, neuroprotection, and autophagy. Nutrients. (2019) ;11: (2):pii:E474. |
[9] | Ayaz M , Sadiq A , Junaid M , Ullah F , Subhan F , Ahmed J . Neuroprotective and Anti-Aging Potentials of Essential Oils from Aromatic and Medicinal Plants. Front Aging Neurosci. (2017) ;9: :168. |
[10] | Datta SK , Datta PC . Pharmacognosy of Streblus Bark Drug. Int. J. Crude Drug Res. (1984) ;22: :61–71. |
[11] | Rastogi S , Kulshreshtha DK , Rawat AK . Streblus asper Lour. (Shakhotaka): a review of its chemical, pharmacological and Ethnomedicinal properties. Evid Based Complement Alternat Med. (2006) ;3: (2):217–22. |
[12] | Verma NK , Singh SP , Singh AP , Singh R , Rai PK , Tripathi AK . A brief study on Strebulus asper L. -a review. Research Journal of Phytomedicine. (2015) ;1: :65–71. |
[13] | Ganu UK , Khandalekar DD , Gokhale SV . Potentiation of anticancer activity by extracts of Streblus asper against p388 murine leukemia. Indian Drugs. (1991) ;28: :504–6. |
[14] | Choudhury MK , Venkatraman S , Upadhyay L . Analysis of in-vitro antioxidant potential of Streblus asper leaves water extract. Biomedicine (India). (2009) ;29: :259–62. |
[15] | Mahida Y , Mohan JSS . Screening of Indian plant extracts for antibacterial activity. Pharm Biol. (2006) ;44: :627–31. |
[16] | Davenport JC . The oral distribution of candida in denture stomatitis. Br Dent J. (1970) ;129: (4):151–6. |
[17] | Choudhury MK , Venkatraman S , Upadhyay L . Phytochemical analysis and peripheral glucose utilization activity determination of Steblus asper. Asian Pac J Trop Biomed. (2012) ;2: :S656–S661. |
[18] | Sripanidkulchai B , Junlatat J , Wara-aswapati N , Hormdee D . Anti-inflammatory effect of Streblus asper leaf extract in rats and its modulation on inflammation-associated genes expression in RAW 264.7 macrophage cells. J Ethnopharmacol. (2009) ;124: (3):566–70. |
[19] | Prasansuklab A , Meemon K , Sobhon P , Tencomnao T . Ethanolic extract of Streblus asper leaves protects against glutamate-induced toxicity in HT22 hippocampal neuronal cells and extends lifespan of Caenorhabditis elegans. BMC Complement Altern Med. (2017) ;17: (1):551. |
[20] | Prasansuklab A , Theerasri A , Payne M , Ung AT , Tencomnao T . Acid-base fractions separated from Streblus asper leaf ethanolic extract exhibited antibacterial, antioxidant, anti-acetylcholinesterase, and neuroprotective activities. BMC Complement Altern Med. (2018) ;18: (1):223. |
[21] | Brimson JM , Prasanth MI , Plaingam W , Tencomnao T . Bacopa monnieri (L.) wettst. extract protects against glutamate toxicity and increases the longevity of Caenorhabditis elegans. J Tradit Complement Med. (2019) ;10: (5):460–70. |
[22] | Sharika R , Subbaiah P , Balamurugan K . Studies on reproductive stress caused by candidate Gram positive and Gram negative bacteria using model organism, Caenorhabditis elegans. Gene. (2018) ;649: :113–126. |
[23] | Shen P , Yue Y , Zheng J , Park Y . Caenorhabditis elegans: A Convenient In Vivo Model for Assessing the Impact of Food Bioactive Compounds on Obesity, Aging, and Alzheimer’s Disease. Annu Rev Food Sci Technol. (2018) ;9: :1–22. |
[24] | Prasanth MI , Santoshram GS , Bhaskar JP , Balamurugan K . Ultraviolet-A triggers photoaging in model nematode Caenorhabditis elegans in a DAF-16 dependent pathway. Age (Dordr). (2016) ;38: (1):27. |
[25] | Kenyon CJ . The genetics of ageing. Nature. (2010) ;464: (7288):504–12. |
[26] | Collins JJ , Huang C , Hughes S , Kornfeld K . The measurement and analysis of age-related changes in Caenorhabditis elegans. WormBook. 2008;1-21. |
[27] | Yi X , Jiang S , Qin M , Liu K , Cao P , Chen S , Deng J , Gao C . Compounds from the fruits of mangrove Sonneratia apetala: Isolation, molecular docking and anti-aging effects using a Caenorhabditis elegans model. Bioorg Chem. (2020) ;99: :103813. |
[28] | Pandey S , Phulara SC , Mishra SK , Bajpai R , Kumar A , Niranjan A , Lehri A , Upreti DK , Chauhan PS . Betula utilis extract prolongs life expectancy, protects against amyloid-β toxicity and reduces Alpha Synuclien in Caenorhabditis elegans via DAF-16 and SKN-1. Comp Biochem Physiol C Toxicol Pharmacol. (2020) ;228: :108647. |
[29] | Yuan Y , Kang N , Li Q , Zhang Y , Liu Y , Tan P . Study of the Effect of Neutral Polysaccharides from Rehmannia glutinosa on Lifespan of Caenorhabditis elegans . Molecules. (2019) ;24: (24):pii:E4592. |
[30] | Alexpandi R , Prasanth MI , Ravi AV , Balamurugan K , Durgadevi R , Srinivasan R , De Mesquita JF , Pandian SK . Protective effect of neglected plant Diplocyclos palmatus on quorum sensing mediated infection of Serratia marcescens and UV-A induced photoaging in model Caenorhabditis elegans. J Photochem Photobiol B. (2019) ;201: :111637. |
[31] | Prasanth MI , Brimson JM , Chuchawankul S , Sukprasansap M , Tencomnao T . Antiaging, Stress Resistance, and Neuroprotective Efficacies of Cleistocalyx nervosum var. paniala Fruit Extracts Using Caenorhabditis elegans Model. Oxid Med Cell Longev. (2019) ;2019: :7024785. |
[32] | Rangsinth P , Prasansuklab A , Duangjan C , Gu X , Meemon K , Wink M , Tencomnao T . Leaf extract of Caesalpinia mimosoides enhances oxidative stress resistance and prolongs lifespan in Caenorhabditis elegans. BMC Complement Altern Med. (2019) ;19: (1):164. |
[33] | Duangjan C , Rangsinth P , Gu X , Zhang S , Wink M , Tencomnao T . Glochidion zeylanicum leaf extracts exhibit lifespan extending and oxidative stress resistance properties in Caenorhabditis elegans via DAF-16/FoxO and SKN-1/Nrf-2 signalling pathways. Phytomedicine. (2019) ;64: :153061. |
[34] | Brenner S . The genetics of Caenorhabditis elegans . Genetics. (1974) ;77: (1):71–94. |
[35] | Brimson JM , Prasanth MI , Isidoro C , Sukprasansap M , Tencomnao T . Cleistocalyx nervosum var. paniala seed extracts exhibit sigma-1 antagonist sensitive neuroprotective effects in PC12 cells and protect C. elegans from stress via the SKN-1/NRF-2 pathway. Nutr. Healthy Aging. 2021;1-16. doi: 10.3233/NHA-200108. |
[36] | Suganthy N , Malar DS , Devi KP . Rhizophora mucronata attenuates Beta-amyloid induced cognitive dysfunction, oxidative stress and cholinergic deficit in Alzheimer’s disease animal model. Metab Brain Dis. (2016) ;31: (4):937–49. |
[37] | Prasanth MI , Gayathri S , Bhaskar JP , Krishnan V , Balamurugan K . Analyzing the Synergistic Effects of Antioxidants in Combating Photoaging Using Model Nematode, Caenorhabditis elegans. Photochem Photobiol. (2020) ;96: (1):139–47. |
[38] | Malar DS , Prasanth MI , Jeyakumar M , Balamurugan K , Devi KP . Vitexin prevents Aβ proteotoxicity in transgenic Caenorhabditis elegans model of Alzheimer’s disease by modulating unfolded protein response. J Biochem Mol Toxicol. (2021) ;35: (1):e22632. |
[39] | Pattarachotanant N , Tencomnao T . Citrus hystrix Extracts Protect Human Neuronal Cells against High Glucose-Induced Senescence. Pharmaceuticals (Basel). (2020) ;13: (10):283. |
[40] | Prasanth MI , Venkatesh D , Murali D , Bhaskar JP , Krishnan V , Balamurugan K . Understanding the role of DAF-16 mediated pathway in Caenorhabditis elegans during UV-A mediated photoaging process. Arch Gerontol Geriatr. (2019) ;82: :279–85. |
[41] | Niccoli T , Partridge L . Ageing as a risk factor for disease. Curr Biol. (2012) ;22: (17):R741–52. |
[42] | Xu DP , Li Y , Meng X , Zhou T , Zhou Y , Zheng J , Zhang JJ , Li HB . Natural Antioxidants in Foods and Medicinal Plants: Extraction, Assessment and Resources. Int J Mol Sci. (2017) ;18: (1):pii:E96. |
[43] | Luanchoy S , Tiangkul S , Wongkrajang Y , Temsiririrkkul R , Peungvicha P , Nakornchai S . Antioxidant activity of a Thai traditional formula for longevity. Mahidol J Pharm Sci. (2014) ;41: :15. |
[44] | Peixoto H , Roxo M , Koolen H , da Silva F , Silva E , Braun MS , Wang X , Wink M . Calycophyllum spruceanum (Benth.), the Amazonian “Tree of Youth” Prolongs Longevity and Enhances Stress Resistance in Caenorhabditis elegans. Molecules. (2018) ;23: (3):534. |
[45] | Spindler SR , Mote PL , Flegal JM , Teter B . Influence on longevity of blueberry, cinnamon, green and black tea, pomegranate, sesame, curcumin, morin, pycnogenol, quercetin, and taxifolin fed iso-calorically to long-lived, F1 hybrid mice. Rejuvenation Res. (2013) ;16: (2):143–51. |
[46] | Lin C , Zhang X , Xiao J , Zhong Q , Kuang Y , Cao Y , Chen Y . Effects on longevity extension and mechanism of action of carnosic acid in Caenorhabditis elegans. Food Funct. (2019) ;10: (3):1398–410. |
[47] | Qi W , Gutierrez GE , Gao X , Dixon H , McDonough JA , Marini AM , Fisher AL . The ω-3 fatty acid α-linolenic acid extends Caenorhabditis elegans lifespan via NHR-49/PPARα and oxidation to oxylipins. Aging Cell. (2017) ;16: (5):1125–35. |
[48] | von Frieling J , Roeder T . Factors that affect the translation of dietary restriction into a longer life. IUBMB Life. (2020) ;72: (5):814–24. |
[49] | Teuscher AC , Ewald CY . Overcoming Autofluorescence to Assess GFP Expression During Normal Physiology and Aging in Caenorhabditis elegans . Bio Protoc. (2018) ;8: (14):e2940. |
[50] | Pincus Z , Mazer TC , Slack FJ . Autofluorescence as a measure of senescence in C. elegans: look to red, not blue or green. Aging (Albany NY). (2016) ;8: (5):889–98. |
[51] | Liao VH , Yu CW , Chu YJ , Li WH , Hsieh YC , Wang TT . Curcumin-mediated lifespan extension in Caenorhabditis elegans. Mech. Ageing Dev. (2011) ;132: (10):480–7. |
[52] | Pincus Z , Slack FJ . Developmental biomarkers of aging in Caenorhabditis elegans . Dev Dyn. (2010) ;239: (5):1306–14. |
[53] | Höhn A , Jung T , Grimm S , Grune T . Lipofuscin-bound iron is a major intracellular source of oxidants: role in senescent cells. Free Radic. Biol. Med. (2010) ;48: (8):1100–8. |
[54] | Song B , Zheng B , Li T , Liu RH . Raspberry extract promoted longevity and stress tolerance via the insulin/IGF signaling pathway and DAF-16 in Caenorhabditis elegans. Food Funct. (2020) ;11: (4):3598–609. |
[55] | Wang H , Liu J , Li T , Liu RH . Blueberry extract promotes longevity and stress tolerance via DAF-16 in Caenorhabditis elegans. Food Funct. (2018) ;9: (10):5273–82. |
[56] | Thein MC , McCormack G , Winter AD , Johnstone IL , Shoemaker CB , Page AP . Caenorhabditis elegans exoskeleton collagen COL- an adult-specific marker for collagen modification and assembly, and the analysis of organismal morphology. Dev Dyn. (2003) ;226: (3):523–39. |
[57] | Hofer AM . Another dimension to calcium signaling: a look at extracellular calcium. J Cell Sci. (2005) ;118: (Pt 5):855–62. |
[58] | Miller KG , Emerson MD , Rand JB . Goalpha and diacylglycerol kinase negatively regulate the Gqalpha pathway in C. elegans. Neuron. (1999) ;24: (2):323–33. |
[59] | Matsuki M , Kunitomo H , Iino Y . Goα regulates olfactory adaptation by antagonizing Gqα-DAG signaling in Caenorhabditis elegans. Proc Natl Acad Sci USA. (2006) ;103: (4):1112–7. |
[60] | Papaioannou S , Holden-Dye L , Walker RJ . Evidence for a role for cyclic AMP in modulating the action of 5-HT and an excitatory neuropeptide, FLP17A, in the pharyngeal muscle of Caenorhabditis elegans . Invert Neurosci.. (2008) ;8: (2):91–100. |
[61] | Govorunova EG , Moussaif M , Kullyev A , Nguyen KCQ , McDonald TV , Hall DH , Sze JY . A homolog of FHM2 is involved in modulation of excitatory neurotransmission by serotonin in C. elegans. PLoS One. (2010) ;5: (4):e10368. |
[62] | Avery L , You YJ . C. elegans Feeding. WormBook. (2012) ;21: :1–23. |
[63] | Novakovic S , Molesworth LW , Gourley TE , Boag PR , Davis GM . Zinc transporters maintain longevity by influencing insulin/IGF-1 activity in Caenorhabditis elegans. FEBS Lett. (2020) ;594: (9):1424–32. |
[64] | Jin C , Li J , Green CD , Yu X , Tang X , Han D , Xian B , Wang D , Huang X , Cao X , Yan Z , Hou L , Liu J , Shukeir N , Khaitovich P , Chen CD , Zhang H , Jenuwein T , Han JD . Histone demethylase UTX-1 regulates C. elegans life span by targeting the insulin/IGF-1 signaling pathway. Cell Metab. (2011) ;14: (2):161–72. |
[65] | Kenyon C . The first long-lived mutants: discovery of the insulin/IGF-1 pathway for ageing. Philos Trans R Soc Lond B Biol Sci. (2011) ;366: (1561):9–16. |
[66] | Zhu B , Jo K , Yang P , Tohti J , Fei J , Abudukerim K . Aiweixin, a Traditional Uyghur Medicinal Formula, Extends the Lifespan of Caenorhabditis elegans. Evid Based Complement Alternat Med. (2019) ;2019: :3684601. |
[67] | Edwards C , Canfield J , Copes N , Brito A , Rehan M , Lipps D , Brunquell J , Westerheide SD , Bradshaw PC . Mechanisms of amino acid-mediated lifespan extension in Caenorhabditis elegans. BMC Genet. (2015) ;16: (1):8. |
[68] | Prasanth MI , Gayathri S , Bhaskar JP , Krishnan V , Balamurugan K . Understanding the role of p38 and JNK mediated MAPK pathway in response to UV-A induced photoaging in Caenorhabditis elegans. J Photochem Photobiol B. (2020) ;205: :111844. |
[69] | Yan F , Chen Y , Azat R , Zheng X . Mulberry Anthocyanin Extract Ameliorates Oxidative Damage in HepG2 Cells and Prolongs the Lifespan of Caenorhabditis elegans through MAPK and Nrf2 Pathways. Oxid Med Cell Longev. (2017) ;2017: :7956158. |
[70] | Yaguchi Y , Komura T , Kashima N , Tamura M , Kage-Nakadai E , Saeki S , Terao K , Nishikawa Y . Influence of oral supplementation with sesamin on longevity of Caenorhabditis elegans and the host defense. Eur J Nutr. (2014) ;53: (8):1659–68. |
[71] | Ketterman AJ , Wongtrakul J , Saisawang C . Phytochemical andrographolide modulates NF-kappaB and JNK in human neuroblastoma SH-SY5Y cells, a cell model for Parkinson’s disease. Heliyon. (2020) ;6: (6):e04121. |
[72] | An JH , Blackwell TK . SKN-1 links C. elegans mesendodermal specification to a conserved oxidative stress response. Genes Dev. (2003) ;17: (15):1882–93. |
[73] | An JH , Vranas K , Lucke M , Inoue H , Hisamoto N , Matsumoto K , Blackwell TK . Regulation of the Caenorhabditis elegans oxidative stress defense protein SKN-1 by glycogen synthase kinase-3. Proc Natl Acad Sci U S A. (2005) ;102: (45):16275–80. |
[74] | Tullet JM , Hertweck M , An JH , Baker J , Hwang JY , Liu S , Oliveira RP , Baumeister R , Blackwell TK . Direct inhibition of the longevity-promoting factor SKN-1 by insulin-like signaling in C. elegans. Cell. (2008) ;132: (6):1025–38. |
[75] | Xiong LG , Huang JA , Li J , Yu PH , Xiong Z , Zhang JW , Gong YS , Liu ZH , Chen JH . Black tea increased survival of Caenorhabditis elegans under stress. J Agric Food Chem. (2014) ;62: (46):11163–9. |
[76] | Song B , Zheng B , Li T , Liu RH . SKN-1 is involved in combination of apple peels and blueberry extracts synergistically protecting against oxidative stress in Caenorhabditis elegans. Food Funct. (2020) ;11: (6):5409–19. |
[77] | Inoue H , Hisamoto N , An JH , Oliveira RP , Nishida E , Blackwell TK , Matsumoto K . The C. elegans p38 MAPK pathway regulates nuclear localization of the transcription factor SKN-1 in oxidative stress response. Genes Dev. (2005) ;19: (19):2278–83. |
[78] | Lipton SA , Rezaie T , Nutter A , Lopez KM , Parker J , Kosaka K , Satoh T , McKercher SR , Masliah E , Nakanishi N . Therapeutic advantage of pro-electrophilic drugs to activate the Nrf2/ARE pathway in Alzheimer’s disease models. Cell Death Dis. (2016) ;7: (12):e2499. |
[79] | Hybertson BM , Gao B , Bose S , McCord JM . Phytochemical Combination PB125 Activates the Nrf2 Pathway and Induces Cellular Protection against Oxidative Injury. Antioxidants (Basel). (2019) ;8: (5):119. |
[80] | Zhao M , Chen J , Zhu P , Fujino M , Takahara T , Toyama S , Tomita A , Zhao L , Yang Z , Hei M , Zhong L , Zhuang J , Kimura S , Li XK . Dihydroquercetin (DHQ) ameliorated concanavalin A-induced mouse experimental fulminant hepatitis and enhanced HO-1 expression through MAPK/Nrf2 antioxidant pathway in RAW cells. Int Immunopharmacol. (2015) ;28: (2):938–44. |
[81] | Zhan JY , Wang XF , Liu YH , Zhang ZB , Wang L , Chen JN , Huang S , Zeng HF , Lai XP . Andrographolide Sodium Bisulfate Prevents UV-Induced Skin Photoaging through Inhibiting Oxidative Stress and Inflammation. Mediators Inflamm. (2016) ;2016: :3271451. |
[82] | Han M , Bae JS , Ban JJ , Shin HS , Lee DH , Chung JH . Black rice (Oryza sativa L.) extract modulates ultraviolet-induced expression of matrix metalloproteinases and procollagen in a skin cell model. Int J Mol Med.. (2018) ;41: (5):3073–80. |
[83] | Soto ME , Soria-Castro E , Lans VG , Ontiveros EM , Mejía BI , Hernandez HJ , García RB , Herrera V , Pérez-Torres I . Analysis of oxidative stress enzymes and structural and functional proteins on human aortic tissue from different aortopathies. Oxid Med Cell Longev. (2014) ;2014: :760694. |
[84] | Azevedo BC , Roxo M , Borges MC , Peixoto H , Crevelin EJ , Bertoni BW , Contini SHT , Lopes AA , França SC , Pereira AMS , Wink M . Antioxidant Activity of an Aqueous Leaf Extract from Uncaria tomentosa and Its Major Alkaloids Mitraphylline and Isomitraphylline in Caenorhabditis elegans. Molecules. (2019) ;24: (18):3299. |
[85] | Singh E , Devasahayam G . Neurodegeneration by oxidative stress: a review on prospective use of small molecules for neuroprotection. Mol Biol Rep. (2020) ;47: (4):3133–40. |
[86] | Malar DS , Suryanarayanan V , Prasanth MI , Singh SK , Balamurugan K , Devi KP . Vitexin inhibits Aβ2535 induced toxicity in Neuro-2a cells by augmenting Nrf-2/HO-1 dependent antioxidant pathway and regulating lipid homeostasis by the activation of LXR-α. Toxicol In Vitro. (2018) ;50: :160–71. |
[87] | Grodzicki W , Dziendzikowska K . The Role of Selected Bioactive Compounds in the Prevention of Alzheimer’s Disease. Antioxidants (Basel). (2020) ;9: (3):pii:E229. |
[88] | Link C . Expression of human beta-amyloid peptide in transgenic Caenorhabditis elegans. Proc Natl Acad Sci USA. (1995) ;92: (20):9368–72. |
[89] | Cisternas P , Oliva CA , Torres VI , Barrera DP , Inestrosa NC . Presymptomatic Treatment With Andrographolide Improves Brain Metabolic Markers and Cognitive Behavior in a Model of Early-Onset Alzheimer’s Disease. Front Cell Neurosci. (2019) ;13: :295. |
[90] | Shashikumar S , Pradeep H , Chinnu S , Rajini PS , Rajanikant GK . Alpha-linolenic acid suppresses dopaminergic neurodegeneration induced by 6-OHDA in C. elegans. Physiol Behav. (2015) ;151: :563–9. |
[91] | Zhang H , Yan ZY , Wang YX , Bai M , Wang XB , Huang XX , Song SJ . Network pharmacology-based screening of the active ingredients and potential targets of the genus of Pithecellobium marthae (Britton & Killip) Niezgoda & Nevl for application to Alzheimer’s disease. Nat Prod Res. (2019) ;33: (16):2368–71. |
[92] | Lindsay CB , Zolezzi JM , Rivera DS , Cisternas P , Bozinovic F , Inestrosa NC . Andrographolide Reduces Neuroinflammation and Oxidative Stress in Aged Octodon degus. Mol Neurobiol. (2020) ;57: (2):1131–45. |
[93] | Panossian A , Hovhannisyan A , Mamikonyan G , Abrahamian H , Hambardzumyan E , Gabrielian E , Goukasova G , Wikman G , Wagner H . Pharmacokinetic and oral bioavailability of andrographolide from Andrographis paniculata fixed combination Kan Jang in rats and human. Phytomedicine. (2000) ;7: (5):351–64. |
[94] | Bera R , Ahmed SK , Sarkar L , Sen T , Karmakar S . Pharmacokinetic analysis and tissue distribution of andrographolide in rat by a validated LC-MS/MS method. Pharm Biol. (2014) ;52: (3):321–9. |
[95] | Doolaege EH , Raes K , De Vos F , Verhé R , De Smet S . Absorption, distribution and elimination of carnosic acid, a natural antioxidant from Rosmarinus officinalis, in rats. Plant Foods Hum Nutr. (2011) ;66: (2):196–202. |
[96] | Rodríguez-Alcalá LM , Ares I , Fontecha J , Juarez M , Castellano V , Martínez-Larrañaga MR , Anadón A , Martínez MA . Oral Absorption and Disposition of alpha-Linolenic, Rumenic and Vaccenic Acids After Administration as a Naturally Enriched Goat Dairy Fat to Rats. Lipids. (2015) ;50: (7):659–66. |
[97] | Wei F , Guo L , Xu Y , Chen D , Gong M . Comparative Pharmacokinetic Study of Taxifolin after Oral Administration of Fructus Polygoni Orientalis Extract in Normal and Fibrotic Rats by UPLC-MS/MS. Evid Based Complement Alternat Med. (2019) ;2019: :9348075. |
[98] | Kumar RBS , Puratchikodi A , Prasanna A , Dolai N , Majumder P , Mazumder UK , Haldar PK . Pre-clinical studies of Streblus asper Lour in terms of behavioural safety and toxicity. Orient Pharm Exp Med. 2011;DOI:10.1007/s13596-011-0040-4. |
[99] | Lee AY , Lee MH , Lee S , Cho EJ . Neuroprotective Effect of Alpha-Linolenic Acid against Abeta-Mediated Inflammatory Responses in C6 Glial Cell. J Agric Food Chem. (2018) ;66: (19):4853–61. |