Aging research using the common marmoset: Focus on aging interventions
Abstract
Traditional animal models have been used to make seminal discoveries in biomedical research including a better understanding of the biology of the aging process. However, translation of these findings from laboratory to clinical populations has likely been hindered due to fundamental biological and physiological differences between common laboratory animals and humans. Non-human primates (NHP) may serve as an effective bridge towards translation, and short-lived NHP like the common marmoset offer many advantages as models for aging research. Here, we address these advantages and discuss what is currently understood about the changes in physiology and pathology that occur with age in the marmoset. In addition, we discuss how aging research might best utilize this model resource, and outline an ongoing study to address whether pharmaceutical intervention can slow aging in the marmoset. With this manuscript, we clarify how common marmosets might assist researchers in geroscience as a potential model for pre-clinical translation.
1Challenges of translation from laboratory animals (mostly rodents) to humans
Animal models have been a critical resource in the quest to evaluate physiological functional decline associated with aging and elucidate the cellular pathways and mechanisms associated with longevity. In general, these studies have focused most of their efforts on delineating the aging process using four primary laboratory organisms: yeast (Saccharomyces cerevisiae), roundworms (Caenorhabditis elegans), fruit flies (Drosophila melanogaster) and rodents including mice and rats (Mus musculus and Rattus norvegicus, respectively) [1]. These species have many practical advantages over other animal models (including humans) as models of aging including their relatively short lifespan, the ability to breed and maintain large numbers of animals in the laboratory, and the ability to control environmental exposure for each individual (or a population). In addition, over the last few decades it has been shown clearly that the relative ease of manipulating genes of interest; i.e., generating organisms with specific genetic mutations to address mechanisms of phenotype including longevity, is a powerful tool for the study of aging. Using these models has been extremely fruitful for geroscience research and has driven discoveries of conserved biochemical pathways associated with longevity, and allowed the comparative evaluation of the effect of aging on metabolism and development, mitochondrial stress pathways, adenosine monophosphate-activated protein kinase, and insulin-like growth factor [2, 3]. In particular, the widespread use of mice, and in particular genetic mutant lines of mice, have driven exponential growth in our understanding of the particular mechanistic pathways on the mammalian aging process [4]. In terms of “translational” approaches to addressing aging and longevity in humans, mice and rats have served a central role in identification and elucidation of interventional and therapeutic treatments on aging and health-span. Most notably, the gold standard for extending lifespan in a model organism is the use of caloric restriction and indeed the seminal studies of caloric restriction have largely been performed using mice and rats [5–9]. In addition, many new drugs of geroscience interest have been tested in rodents and continue to reveal details regarding aging and disease progression, and have yielded novel findings about the anti-aging effect of intervention with pharmaceutical and nutraceutical compounds [10–17]. In many ways, interventional approaches utilizing pharmaceuticals seem to be the most “turn-key” for benefiting human health in terms of their relative ease of translation for clinical consideration.
However, there remain many concerns regarding using rodents as a model of human model of aging (in addition to disease, physiology, pathology, etc.) as a foundation for designing treatments and interventions to improve human health. Unfortunately, a litany of findings in rodent translational research have revealed that many of the drugs tested in rodents do not translate successfully to humans, either having few similar effects or having toxicity in humans [18]. Further, the development of inbred strains of mice has introduced the perpetuation of many traits that are a by-product of selection for breeding, rather than traits of interest. Many of these traits are undetected or unknown and may be detrimental to longevity studies or have unknown impact on the research of interest [19]. Lastly, there is a distinct dichotomy between rodents and humans in terms of the spectrum of diseases that naturally occur with old-age or otherwise. Particularly challenging are the wide-range of diseases and pathologies that plague aging humans that do not naturally exist in rodents (e.g., neurodegenerative diseases including Alzheimer’s disease, cardiovascular disease, diabetes, and a host of others) due to differences between species in their basic physiology, the dramatic differences in their maximum ages, diet and environmental conditions or other unknown variables. Specifically because of such challenges, there has been significant investment in drawing from comparative biology to identify additional animal models that may serve as stronger bridges to clinical translation.
2How non-human primates are useful (critical) to this process
Non-human primate (NHP) models are of particular interest for additional pre-clinical testing towards translation due to their close evolutionary history with humans. Old World monkeys and apes are the closest living relatives to humans and share a closer evolutionary history with humans than other mammals, and thus more similar genetic, biochemical, behavioral and phenotypic outcomes. Old world monkeys split from the ancestors of the human lineage around 25 million years ago, and apes, specifically chimpanzees, split as recently as six million years ago [20, 21]. In the limited studies that have examined the aging characteristics of captive chimpanzees, our closest living relatives, the age-related diseases in this species are described as similar to that of humans including the development of heart disease, cancer, and diabetes with advancing age [22–29]. However, chimpanzees are very long lived, with animals in captivity living up to 60 years, thus making them comparable in difficulty to working with human populations [21]. Additionally, both wild and captive populations of chimpanzees are now classified by the International Union for Conservation of Nature (IUCN) as endangered animalsand the National Institutes of Health (NIH) have effectively ceased funding for invasive research on chimpanzees. In part for these reasons, research using chimpanzees in the laboratory setting has significantly diminished in many countries including the U.S.
Other Old World monkeys, including rhesus macaques, have traditionally been of greater focus for biomedical translational research including aging studies [30–35]. Rhesus macaques have a maximum lifespan of approximately 40 years in captivity and their aging phenotypes have been well characterized [36, 37]. In addition, a means to monitor cognitive functional decline in this species has been developed and is hypothesized to model the similar decline in human cognition with age [32]. The rhesus macaque has also been used to test whether the benefits of caloric restriction on healthy aging translate to NHP species [30]. While there continues to be some discussion on the equivocal nature of caloric restrictions’ effect on NHP lifespan in two ongoing research groups, these studies have largely confirmed that calorie restriction reduces the prevalence of cardiovascular disease, type 2 diabetes, and neoplasias [38–45]. Due in part to the long lifespan of this species and the large time and financial commitment to these studies, it is unlikely that such in depth NHP lifespan studies will be able to be repeated.
In an attempt to reduce the heavy commitments required for aging NHP studies, several shorter-lived NHP species have been suggested for longevity research including the bush baby (Galago senegalensis), the grey mouse lemur (Microcebus murinus), and the common marmoset (Callithrix jacchus) [46, 47]. These species all have relatively shorter lifespans (for each, the maximum lifespan is ∼15–20 years) compared to Old World monkeys and apes, and they offer a number of advantages due to their small size and rapid reproduction [46, 47]. Of note, it was recently reported that the lifespan of the grey mouse lemur could be extended by calorie restriction but that this intervention did not alter cognitive or motor function [48]. However, of these species, the common marmoset has recently emerged as a model for aging related research due to growing investigations on the changes in physiology, pathology and health that occur in this species [49–54].
3Marmosets in aging research
The common marmoset has many characteristics that make it attractive as a non-human primate model for aging research. As mentioned above, this species has a lifespan that is roughly half that of other NHP species commonly used in biomedical research (Fig. 1). While still quite long compared to rodents, this length of time is certainly more amenable to use within the career of a single researcher. Moreover, there is fiduciary benefit to the shorter lifespan (i.e., half the amount of time paying per diems as other long-lived NHP). Along those lines, per diem costs tend to be significantly lower for marmosets than for other NHP, due in part to the smaller cage and space requirements. Their small size also has benefits in terms of pharmaceutical interventions (i.e., less drug is required per animal) as well as in terms of staff safety. Marmosets are also the fastest reproducing anthropoid primate giving birth to fraternal twins on average twice per year [55]. This fast reproduction allows for relatively rapid growth of colonies for use in biomedical research. Further, these characteristics allowed for the development of an specific pathogen free (SPF) barrier maintained colony of marmosets for aging research which to date has displayed extended lifespan when compared to conventionally housed marmosets [56]. These features then support the notion that marmosets offer a unique NHP model with many advantages for studying the physiological changes associated with aging.
Fig.1
Maximum reported lifespans for four primary primate species used in aging research.
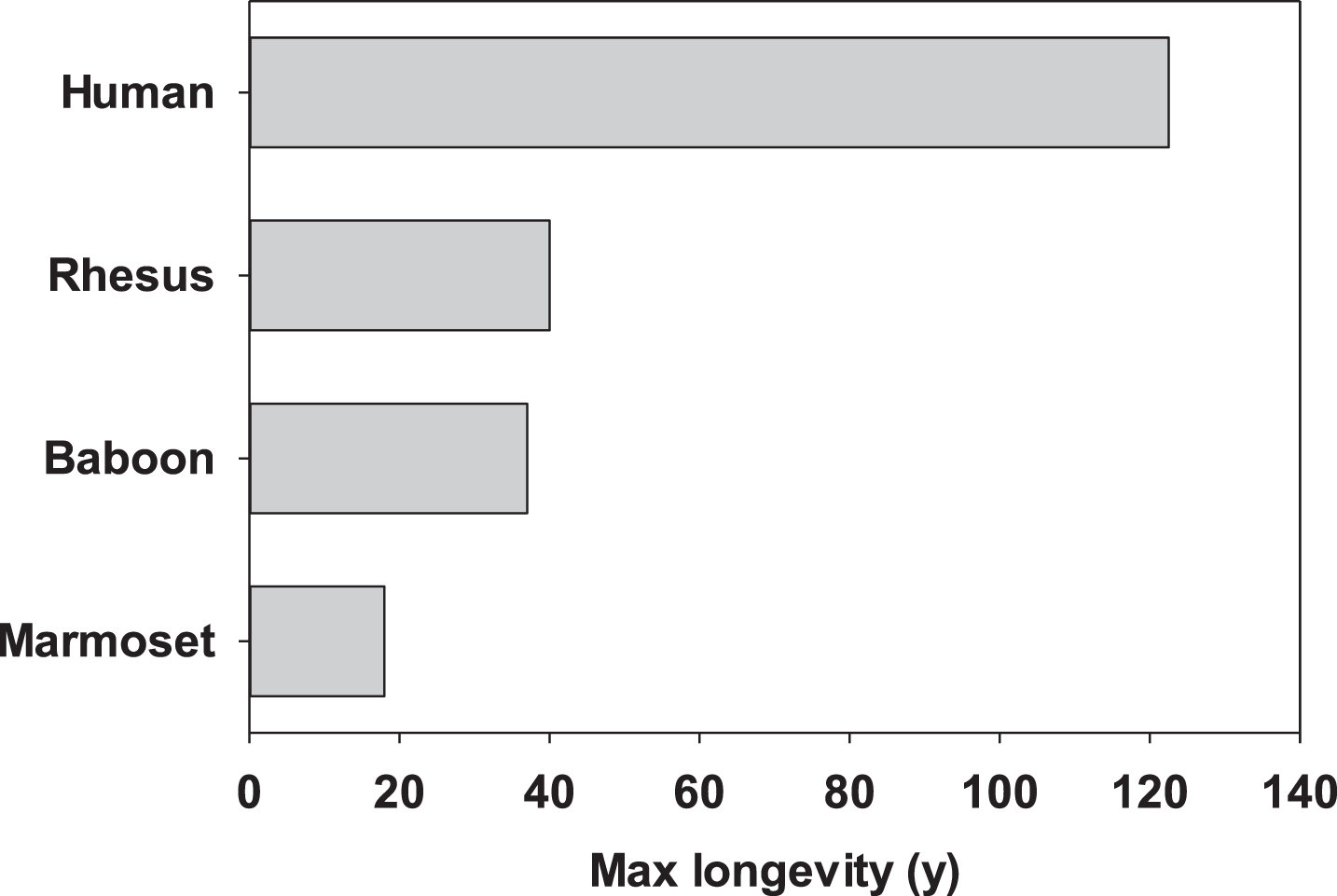
As part of the development of this species as an aging model, there is a growing understanding of the basic aging phenotypes of aging marmosets. For example, a number of skeletal changes associated with aging have been described in marmosets. Marmosets lack adult growth plates and display similar human age related structural changes in skeletal bone [57]. When treated with a bisphosphate therapy, trabecular number and volume increased in marmosets exhibiting age-related bone loss [57]. Further, marmoset bones undergo degeneration of structure associated with bone aging in such bones as the vertebrae [58].
With advancing age, marmosets have been reported to lose weight and show dramatic changes in body composition [54, 59]. These patterns are very similar to the types of changes in body weight and composition that occur in the latter half of normal lifespan in humans [60]. Interestingly, the maximum weight achieved by an individual marmoset is significantly associated with the likelihood of survival in older animals, with animals having a peak mass of less than 400 g having decreased survival after eight years of age [54]. Perhaps related to this, marmosets that exhibit increased rates of stretching behavior have a significantly higher 6-month mortality than animals do not display this behavior [50]. Rather than a beneficial behavior, this type of stretching is hypothesized to be a posture adopted due to discomfort in the marmoset. It is an atypical posture in which the marmosets hang for extended periods of time from their forelimbs rather than maintaining a more typical marmoset quadrupedal positioning, It is still unclear whether this can be attributed to a specific pathology but it would certainly be of further interest to identify how marmoset activity and ambulatory behavior are impacted by physiological declines associated with aging.
There has also been increasing interest in marmosets as a model to evaluate a number of age-related neurological diseases including Parkinson’s disease, Huntington’s disease, Alzheimer’s disease, stroke, multiple sclerosis and spinal cord injury. Marmosets exhibit decreased adult neurogenesis in the dentate gyrus that is evident before the animals would typically be classified as aged [61]. Ultrastructural examinations of the frontal cortex and hippocampus for animals more than 12 years of age found widespread accumulation of lipofuscin in the glial cells, perivascular macrophages and pericytes [62]. A recent study described an increase in α-synuclein aggregations in the olfactory bulb and hippocampus of aged marmosets [63]. β -amyloid deposition has been described in the brain of marmosets over the age of seven [64], and this data is often used as a criterion for defining marmosets as aged when they are eight years old. However, Ridley [65] did not detect β -amyloid in animals under the age of 10 in their colony and very little deposition in older animals. Marmoset brains have also been evaluated for the presence of amyloid beta markers and tau hyperphosphorylation [66] revealing diffuse amyloid plaques throughout the cortex of the aged marmosets, but not in the younger age groups. Conformational changes in tau were detected in all subjects in this study but these changes increased in frequency with aging. Dystrophic microglia were also significantly more likely to exhibit tau hyperphosphorylation than were active microglia in this study. However, the inconsistency between the presence of neurological markers at specific ages in the marmosets suggests that there may be environmental differences associated with the rate of aging between the colonies that have been examined.
As a potential translational model, there have also been numerous attempts to apply standard human clinical biomarkers of aging to marmosets. To date the only reported change in marmoset blood chemistry associated with age has been a significant decrease in albumin with advancing age [50]. Serum albumin decreases are of particular interest as they are a highly predictive of a risk of death in otherwise healthy aging humans [67]. In the largest study to date of age-related changes in marmoset blood chemistry, a cross-sectional analysis of 60 marmosets ranging in age from two to 13 years old identified 2500 metabolites [68, 69]. Connectivity between the metabolites was found to decrease with age and the abundance of several of the metabolites significantly declined with age [70]. With age, marmosets tend to display increased mean arterial pressure and diastolic pressure, but no age effect on the systolic pressure [71]. Finally, testosterone has been found to decrease in aging male marmosets, but they remain capable of reproduction, and no known phenotypic changes are associated with the decreased testosterone [55, 72–74].
An analysis of the pathologies associated with age in marmosets at the New England National Primate Center and the Southwest National Primate Research Center revealed dramatic shifts in the associated causes of death as animals aged in the colony [50, 54]. Deaths in young adults under the age of 6 years were most likely to be due to injury, inflammatory bowel disease (IBD) and infection. Both colonies reported rare occurrences of neoplasia and diabetes. In contrast, for marmosets over the age of 6 years the most likely causes of death were infection and IBD, with increasing rates of pathologies typically thought of as age related pathologies in humans and other model organisms including neoplasia, amyloidosis, diabetes, cardiac and renal failure. It must be noted that IBD is not a primary cause of mortality in humans and this reflects one of the challenges in using the marmoset as an aging model. On the other hand, the etiology of IBD in the marmoset has been difficult to ascertain and may be driven in part by susceptibility to infection in the community housing setting. Supporting this idea, we recently reported that marmosets maintained in SPF conditions showed no deaths associated with inflammatory gastrointestinal or infectious disease unlike marmosets maintained in a standard colony setting [56]. A brief summary and comparison of most likely causes of adult death among mice, marmosets, and humans are provided in Fig. 2.
Fig.2
Common causes of death among laboratory mice, laboratory marmosets, and humans (clipart images from www.istockphotos.com).
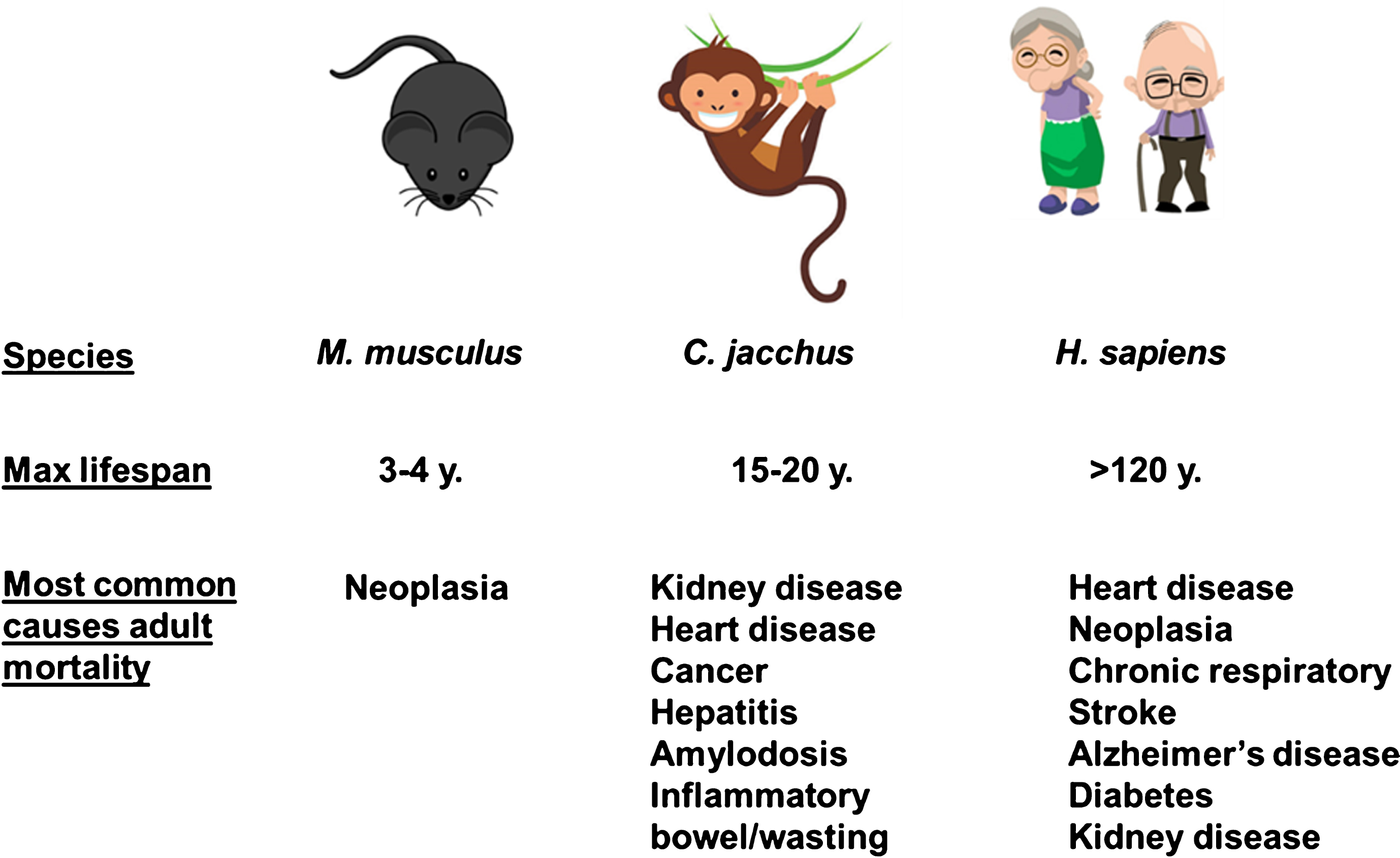
While the extent of physiological and behavioral markers that have been evaluated for changes with aging is ever expanding, many things remain to be examined. For example, a common evaluation to assess frailty or health in geriatric patients is the use of walking speed and grip strength. Translating these relatively simple human assessments to marmosets has proven to be challenging. Other indicators of physiological and social resilience are also still lacking and need further refinement and development to determine their trajectories with age. Tools to assess cognitive health are not as varied and detailed in the marmoset as they are for human evaluations. Many of the cognitive tools used to evaluate Old World monkeys or apes are beyond the ability of the marmoset to be trained for or to complete, and interpretations of results can be controversial. Development of new cognitive tests and adaptations of human, monkey and mouse assessments are ongoing developments.
4Scientific approaches to study aging in marmosets
It is now clear that longevity can be altered using three primary means in traditional laboratory animals: genes, diet, and pharmaceutical interventions [13, 75, 76]. These interventional methods are invaluable tools for studying the basic biology of aging and there is growing evidence that they can be translated to marmoset studies with some considerations.
While genetic modifications have been valuable tools in invertebrate and mouse models of aging, one of the biggest disadvantages historically for NHP biomedical studies has been the inability to produce genetically modified individuals to examine targeted areas of interest in ways that are either relatively cheap or easy (or hopefully both). There are a few reports of transgenic Old World monkeys that exist but the production of a single living offspring in these species is generally extraordinarily costly both financially and in terms of time commitment [77, 78]. Moreover, in terms of aging studies the phenotypic symptoms of the gene of interest may not appear until mid- to old-age which could be on the orders of decades. However, transgenic marmosets have existed for nearly a decade with the production of the lentiviral-induced GFP transgenic marmosets first reported in 2009 [79]. In this mutant monkey, the GFP transgene was distributed throughout the somatic cells in addition to a successful germ-line transmission that was verified within 2 years of the birth of the initial infants. Since this first report, a number of facilities have produced transgenic lines of marmosets using lentiviral transduction, CRISPR/Cas and Tet-on systems. A process that has a great deal of potential for the production of transgenic marmosets is somatic cell nuclear transfer (SCNT) because it may decrease the likelihood of producing mosaic individuals, but to date these attempts have not been successful [80]. Regarding aging research, many of the transgenic lines proposed and developed using marmosets have focused on neurologic disease and modeling of neurodegenerative disorders such as Alzheimer’s and Parkinson’s. Lentiviral transgenic induction of Tet-on human ataxin 3 genes has produced young marmosets with neurodegenerative disease phenotype [81, 82]. Recently, marmosets that express transgenic induction of intracellular calcium indicators have been developed in order to greatly advance the technology and imaging possibilities [83]. While the developing transgenic marmoset models is still certainly much more expensive and time consuming than developing similar rodent models, the ability to examine neurodegenerative disorders and cognitive deficit in a NHP will greatly advance our understanding of these processes in human aging. Further refinement of transgenic marmoset generation will also help expand the scientific focus of such models to other areas of aging research.
As mentioned above, calorie restriction has been one of most important tools used in aging research to understand the basic mechanisms of animal aging. Dietary interventions, including both dietary restriction and over-nutrition, have been adapted to marmoset studies to test the physiological outcomes of such interventions [84, 85]. However, these effects have not been evaluated in association with aging or age- related disease to date. Dietary restriction in marmosets has been limited to evaluations of the timing of caloric restriction during pregnancy and lactation to infant outcome [84]. While it is possible to restrict marmosets calorically, for some short studies such as measuring food intake or evaluation of daily food patterning, it is extremely challenging to use this intervention under normal housing conditions for this animal; i.e., social housing. Marmosets are one of the few primates that has been found to actively share food with other family members and with infants [86]. This particular trait of marmosets makes it difficult to account for food taken from a hopper by an individual animal because even though they remove it from a dish, they are not necessarily eating the food by themselves. Single housing animals, as would typically be done in rodent calorie restriction, is not preferred in marmosets due to the social habits (and requirements) of this species. One potential approach to food restriction might be separating the individual of interest during feeding and then removing all food from the family housing prior to returning the animal. However, this technique is extremely labor intensive and it is likely that the entire group’s feeding patterns would be shifted in ways that would be challenging to document. Another possible approach would be to use time-restricted feeding or intermittent fasting, though the status of these approaches in comparison to “traditional” calorie restriction is still equivocal. Thus, there is still opportunity to develop appropriate caloric restriction paradigms in the marmoset for future testing.
Pharmaceutical interventions are by far the most easily translated to marmoset studies of aging and age-related disease. The marmoset is already a valuable NHP model for evaluating preclinical drug pharmacokinetics, teratology and toxicity in part due to its small size and thus need for smaller doses of drugs compared to other NHP [87–89]. Their metabolic profile and primate physiology allows drugs to be tested in the same delivery modality as will be used in human dosing, making translation to clinical trials smoother. Marmosets have been key to the development of drugs for use in neuroscience, immunology and infectious disease [89]. Drug development and testing for multiple sclerosis, Parkinson’s, stroke, spinal injury, amyloidosis, hepatitis, encephalitis, viral hemorrhagic fevers and bone disease have all relied on marmosets [89]. Thus, an easy case can be made for using this particular approach as the “low-hanging fruit” for refinement of the marmoset as a valuable NHP for studying the basic biology of aging. In this regard, we have put together an aging cohort of marmosets to test the very idea of whether drug intervention can delay the aging process in this NHP.
5Designing a study to address whether rapamycin slows aging in marmosets
The work of the Interventions Testing Program and others has identified rapamycin as one of the most consistent (so far) pharmaceutical interventions capable of extending lifespan and improving health in mice [13–15, 17, 90]. Rapamycin is an inhibitor of mTOR (mechanistic target of rapamycin) signaling and this compound, along with some of its analogues, have been shown to delay (if not rejuvenate) the physiological dysfunction and pathologies associated with aging across multiple organs in the aging mouse [90–96]. Collectively, these outcomes have provided the first proof of principle that longevity in mammals can be extended, if not slowed outright, by administration of a pharmaceutical agent. As described above, there are significant challenges in applying the results of intervention testing in rodents towards the benefit of human health. There is some evidence that rapamycin or its orthologs might provide some benefit to generally healthy older human populations with limited side-effects [97, 98]. While clinical based trial may reveal additional benefit, they are unlikely in the near future to be capable of determining whether this intervention increases longevity or slows aging among humans directly. As discussed here, there then is potentially tremendous benefit in addressing whether interventions that extend longevity in mice, such as rapamycin, similarly extend lifespan in a NHP species.
To this end, we have enrolled a cohort of middle-aged marmosets into a long-term study to test the effect of rapamycin on longevity and healthy aging in this species. During the development of this project, we previously characterized the pharmacokinetics of daily oral treatment with rapamycin encapsulated in a slow-release enteric coating (as used in the Intervention Testing Program’s mouse studies). We reported that marmosets treated with an oral dose of 1.0 mg/kg body weight/day rapamycin showed circulating trough rapamycin concentrations similar to those reported in mouse lifespan studies as well as similar to the reported therapeutic ranges for humans given rapamycin as chemotherapy [99]. Moreover, we showed this dose of rapamycin was sufficient to inhibit mTOR signaling in vivo both in the short (weeks) and long (14 months) term. In this pilot study, we also reported little to no signs of intolerability among animals treated with rapamycin (total 7 animals of mixed sexes) and no additional veterinary interventions were required of animals who had taken daily treatment with this drug for approximately 14 months.
Our results from this pilot study in large part confirmed that we could deliver rapamycin to marmosets in such a way to at least achieve similar blood concentrations (and in vivo mTOR inhibition) similar to that reported in mouse longevity studies using this drug. While this suggests the basic biochemical properties of this drug intervention can be translated between these species, other results from our pilot study support the notion that physiological outcomes of such an intervention may differ between species. For example, one of the relatively consistent ancillary effects of rapamycin treatment in mice has been development of glucose intolerance, likely due to an inhibitory effect of rapamycin on mTORC2 signaling in the liver [15, 100–102]. However, in marmosets treated with rapamycin we found no evidence of glucose intolerance measured by oral glucose tolerance tests or indices of insulin resistance [103]. It is unclear exactly why we found these discrepancies between species, though one possibility might be basic anatomical differences between mice and NHP. For example, the liver, which has been reported to be at least partially responsible for rapamycin-mediated glucose intolerance, makes up a greater contribution of the total mass of mice in comparison to marmosets. In this regard, marmoset relative liver mass is much more similar to humans than to mice. While this provides no assurance that results from marmosets will directly translate to humans, it does hint at the potential value of utilizing a model species with basic biological characteristics similar to humans in biomedical research.
In a similar vein, there has been speculation that at least part of the beneficial effects of rapamycin on longevity are mediated through mTOR’s effects on protein homeostasis (or proteostasis). While the relationships between mTOR and autophagy and mTOR and protein translation are well-understood, there is also growing evidence that mTOR may regulate proteostasis through other pathways such as ubiquitin-proteosome and protein chaperone activation. Indeed, previous reports have suggested that chronic rapamycin treatment can stimulate autophagy, induce proteosome activity and increase expression of protein chaperones [104–107]. In marmosets treated with rapamycin, we found evidence for mild stimulation of autophagy in some, though not all, tissues compared to control animals though little to no evidence that other protein degradation pathways, including proteasome and protein chaperones, were affected [108]. These data then suggest that if rapamycin has an effect on healthy aging in the marmoset, at least some of this effect might be contributed to activation of autophagy and provides a mechanistic target of action for further study.
The goal of our ongoing marmoset study is to directly address the question of whether intervention with rapamycin will benefit longevity or healthy aging in this species. As mentioned above, we have recruited and enrolled a cohort of middle-aged marmosets into a long-term study to test this question. We have designed this experiment starting with older animals (approximately 5–7 years of age in this species) for three main reasons. 1) This provides a reasonable chance to see effects on longevity in this species over the next 5–10 years. 2) This is similar to the original Interventions Testing Program report on rapamycin which started in middle-aged mice and showed a beneficial effect of this drug on longevity [13]. 3) It is reasonable to assume that translation of aging interventions in humans might target those individuals that have reached a period considered later in life [97]. Our cohort is of mixed sex with roughly equivalent numbers of male and female animals. We are treating half of the cohort with rapamycin administered daily at dose of 1 mg/kg body weight which we have shown is comparable to doses known to extend mouse lifespan [99], the other half of this cohort is control (i.e., treated daily with the agent used to encapsulate rapamycin only). We are tracking physiological and behavioral phenotypes as well as health outcomes and longevity over the next several years.
While our primary goal is to assess effects of this intervention on longevity in the marmoset, we also have the opportunity to examine the effects of rapamycin on “healthy aging” or the progression of age-related physiological decline. In mice, rapamycin has been suggested to delay this progression (or even reverse age-related loss) across several organ systems with cardiac [91, 93], hematopoietic stem cells [96], the immune system [94], and periodontal bone [109] benefits among the many reported positive outcomes. In our cohort of aging marmosets, we are testing the effect of both advancing age and rapamycin intervention across multiple minimally-invasive assessments of animal health, frailty and resilience. For example, changes in blood chemistry, complete blood counts, blood lipids, etc. are being tested through regular blood draws similar to what would be performed during routine physical assessments in a clinical setting. In addition, we have defined scheduled tests of glucose metabolism, cardiovascular function, musculoskeletal function and inflammatory processes outlined for repeated testing throughout the remaining lifetime of this cohort. These repeated longitudinal tests also give us an opportunity to address long-term administration of rapamycin to a relatively healthy population has similar potential for side-effects as in clinical use to treat specific disease conditions. For example, the most commonly cited potential side effects of this drug are metabolic dysfunction (including new-onset type 2 diabetes and dyslipidemia) and immunological suppression. As mentioned above, we have found so far no evidence to suggest rapamycin is impairing glucose metabolism at least through approximately 1 year of treatment and no evidence for dyslipidemia [51]. We continue to monitor these outcomes in our long-term treatment cohort. While our design does not allow us to directly test changes in immune response with age and rapamycin in the marmoset, we do monitor changes in blood cell count (including subsets of leukocytes) during our semi-annual health checkups. Again, to date we have found little evidence that chronic rapamycin significantly alters these parameters. Functional tests of immune cell function in vivo would at least tangentially address whether rapamycin inherently alters the ability of the immune cells to address challenge.
At death, animals will undergo full pathological assessment as well as to tissue collection to assess the effect of chronic intervention with rapamycin on multiple markers that represent the “pillars of aging” [110]. Thus, over the course of this study we should develop a complete picture of not only the broad effects of aging on the common marmoset but also whether pharmaceutical interventions known to extend lifespan in mice have similar effects in a NHP species.
6Conclusions
Marmosets offer a unique non-human primate model in which to evaluate both cross-sectional and longitudinal effects of aging on measures of longevity and healthspan. While areas of interest remain for which there are currently no assessment tools for use in the marmoset, recent developments have rapidly expanded tools that are available. Marmosets have significant advantages including relatively short life span and ease of handling that make them ideal for this type of work. We look forward to the continued expansion of tools and knowledge that are becoming available for the marmosets as models for aging research.
Funding
The authors report no funding.
Conflict of interest
The authors have no conflict of interest to report.
Acknowledgments
During the preparation of this manuscript, CNR received support from a grant from the National Institute of Health (P30 AG013319); ABS received support from grants from the National Institute of Health (R01 AG050797 and R01 AG057431), the San Antonio Nathan Shock Center and Claude A. Pepper Center, and from the Geriatric Research, Education and Clinical Center of the South Texas Veterans Health Care System. This material is the result of work supported with resources and the use of facilities at South Texas Veterans Health Care System, San Antonio, Texas. The contents do not represent the views of the U.S. Department of Veterans Affairs or the United States Government.
References
[1] | Wang AM , Promislow DE , Kaeberlein M .Fertile waters for aging research. Cell.. (2015) ;160: (5):814–5. doi: 10.1016/j.cell.2015.02.026. PubMed PMID: 25723160. |
[2] | Bitto A , Wang AM , Bennett CF , Kaeberlein M .Biochemical geneticpathways that modulate aging in multiple species. Cold SpringHarbor Perspectives in Medicine.. (2015) ;5: (11). doi: 10.1101/cshperspect.a025114. |
[3] | Santulli G , Borras C , Bousquet J , Calzá L , Cano A , Illario M , et al., Models for preclinical studies in aging-related disorders:One is not for all. Translational Medicine @ UniSa. (2015) ;13: . PubMed PMID: PMC4811343. |
[4] | Yuan R , Peters LL , Paigen B .Mice as a mammalian model for research on the genetics of aging. ILAR J. (2011) ;52: (1). PubMed PMID: 21411853; PubMed Central PMCID: PMCPMC3074346. |
[5] | Koopman JJ , van Heemst D , van Bodegom D , Bonkowski MS , Sun LY , Bartke A .Measuring aging rates of mice subjected to caloric restriction and genetic disruption of growth hormone signaling. Aging (Albany NY).539-46. (2016) ;8: (3). doi: 10.18632/aging.100919. PubMed PMID: 26959761; PubMed Central PMCID: PMCPMC4833144. |
[6] | Morselli E , Maiuri MC , Markaki M , Megalou E , Pasparaki A , Palikaras K , et al., Caloric restriction and resveratrol promote longevity through the Sirtuin-1-dependent induction of autophagy. Cell Death Dis. (2010) ;1: : e10doi: 10.1038/cddis.2009.8. PubMed PMID: 21364612; PubMed Central PMCID: PMCPMC3032517. |
[7] | Wuttke D , Connor R , Vora C , Craig T , Li Y , Wood S , et al., Dissecting the gene network of dietary restriction to identifyevolutionarily conserved pathways and new functional genes. PLoSGenet. (2012) ;8: (8): e834. doi: 10.1371/journal.pgen.1002834. PubMed PMID: 22912585; PubMed Central PMCID:PMCPMC3415404. |
[8] | McDonald RB , Ramsey JJ .Honoring clive McCay and 75 years of calorie restriction research. The Journal of Nutrition. (2010) ;140: (7): 1205–10. doi: 10.3945/jn.110.122804. PubMed PMID: PMC2884327. |
[9] | Yu BP , Masoro EJ , Murata I , Bertrand HA , Lynd FT .Life span study of SPF Fischer 344 male rats fed ad libitum or restricted diets: Longevity, growth, lean body mass and disease. Journal of gerontology. (1982) ;37: (2): 130–41. PubMed PMID: 7056998. |
[10] | Hasty P , Livi CB , Dodds SG , Jones D , Strong R , Javors M , et al., eRapa restores a normal life span in a FAP mouse model. Cancer Prevention Research. (2014) ;7: (1): 169–78. doi: 10.1158/1940-6207.CAPR-13-0299. PubMed PMID: 24282255; PubMed Central PMCID: PMC4058993. |
[11] | Kennedy BK , Pennypacker JK .Drugs that modulate aging: The promising yet difficult path ahead. Transl Res. (2014) ;163: (5):456–65. doi: 10.1016/j.trsl.2013.11.007. PubMed PMID: 24316383; PubMed Central PMCID: PMCPMC4004650. |
[12] | Sharp ZD , Bartke A .Evidence for down-regulation of phosphoinositide 3-kinase/Akt/mammalian target of rapamycin (PI3K/Akt/mTOR)-dependent translation regulatory signaling pathways in Ames dwarf mice. J Gerontol A Biol Sci Med Sci.. (2005) ;60: (3):293–300. PubMed PMID: 15860463. |
[13] | Harrison DE , Strong R , Sharp ZD , Nelson JF , Astle CM , Flurkey K , et al., Rapamycin fed late in life extends lifespan in geneticallyheterogeneous mice. Nature. (2009) ;460: (7253):392–5. doi: 10.1038/nature08221. PubMed PMID: 19587680; PubMed Central PMCID: PMC2786175. |
[14] | Miller RA , Harrison DE , Astle CM , Baur JA , Boyd AR , de Cabo R , et al., Rapamycin, but not resveratrol or simvastatin, extends life span of genetically heterogeneous mice. The Journals of Gerontology Series A: Biological Sciences and Medical Sciences. (2011) 2:191–201. doi: 10.1093/gerona/glq178. PubMed Central PMCID: PMC3021372. |
[15] | Miller RA , Harrison DE , Astle CM , Fernandez E , Flurkey K , Han M , et al., Rapamycin-mediated lifespan increase in mice is dose and sex dependent and metabolically distinct from dietary restriction. Aging Cell.468-77. (2014) ;13: (3):468–77. doi: 10.1111/ace1.12194. PubMed Central PMCID: PMC4032600. |
[16] | Harrison DE , Strong R , Allison DB , Ames BN , Astle CM , Atamna H , et al., Acarbose, 17-alpha-estradiol, and nordihydroguaiaretic acid extend mouse lifespan preferentially in males. Aging Cell. (2014) ;13: (2):273–82. doi: 10.1111/acel.12170. PubMed PMID: 24245565; PubMed Central PMCID: PMC3954939. |
[17] | Strong R , Miller RA , Antebi A , Astle CM , Bogue M , Denzel MS , et al.,Longer lifespan in male mice treated with a weakly estrogenicagonist, an antioxidant, an alpha-glucosidase inhibitor or aNrf2-inducer. Aging Cell.. (2016) ;15: (5):872–84. doi: 10.1111/acel.12496. PubMed PMID: 27312235. |
[18] | BrackenMB.Why animal studies are often poor predictors of humanreactions to exposure. Journal of the Royal Society of Medicine. (2009) ;102: (3):120–2. doi: 10.1258/jrsm.2008.08k033. PubMed PMID: PMC2746847. |
[19] | Zeiss CJ , Allore HG , Beck AP .Established patterns of animal study design undermine translation of disease-modifying therapies for Parkinson’s disease. PLoS One. (2017) ;12: (2):e0171790. doi: 10.1371/journal.pone.0171790. PubMed PMID: 28182759. |
[20] | Chatterjee HJ , Ho SYW , Barnes I , Groves C .Estimating thephylogeny and divergence times of primates using a supermatrixapproach. BMC Evolutionary Biology.. (2009) ;9: (1):259. doi: 10.1186/1471-2148-9-259 |
[21] | Finch C , Austad SN .Primate aging in the mammalian scheme: The puzzle of extreme variation in brain aging. AGE. (2012) ;34: , 1075–91. |
[22] | Bellino FL , Wise PM .Nonhuman primate models of menopause workshoBiol Reprod. Biol Reprod.. (2003) ;68: (1):10–8. PubMed PMID: 12493689. |
[23] | Chilton J , Wilcox A , Lammey M , Meyer D .Characterization of acardiorenal-like syndrome in aged chimpanzees (Pan troglodytes). Vet Pathol.. (2016) ;53: (2):417–24. doi: 10.1177/0300985815618435. PubMed PMID: 26792841. |
[24] | Ely JJ , Zavaskis T , Lammey ML .Hypertension increases with aging and obesity in chimpanzees (Pan troglodytes). Zoo Biol.. (2013) ;32: (1):79–87. doi: 10.1002/zoo.21044. PubMed PMID: 22968757; PubMed Central PMCID: PMCPMC3537917. |
[25] | Gilissen EP , Leroy K , Yilmaz Z , Kovari E , Bouras C , Boom A , et al., A neuronal aging pattern unique to humans and common chimpanzees. Brain Struct Funct. (2016) ;221: (1):647–64. doi: 10.1007/s00429-014-0931-5. PubMed PMID: 25381006. |
[26] | Lowenstine LJ , McManamon R , Terio KA .Comparative pathology ofaging great apes: Bonobos, chimpanzees, gorillas, and orangutans. Vet Pathol.250-76. (2016) ;53: (2):250–76. doi: 10.1177/0300985815612154. PubMed PMID: 26721908. |
[27] | Nunamaker EA , Lee DR , Lammey ML .Chronic diseases in captive geriatric female Chimpanzees (Pan troglodytes). Comp Med. (2012) ;62: (2):131–6. PubMed PMID: 22546920; PubMed Central PMCID: PMCPMC3318251. |
[28] | Sleeper MM , Drobatz K , Lee DR , Lammey ML .Echocardiography parameters of clinically normal adult captive chimpanzees (Pan troglodytes). J Am Vet Med Assoc. (2014) ;244: (8):956–60. doi: 10.2460/javma.244.8.956. PubMed PMID: 24697773. |
[29] | Videan EN , Fritz J , Murphy J .Effects of aging on hematology and serum clinical chemistry in chimpanzees (Pan troglodytes). Am J Primatol.327-38. (2008) ;70: (4):327–38. doi: 10.1002/ajp.20494. PubMed PMID: 17943981. |
[30] | Didier ES , MacLean AG , Mohan M , Didier PJ , Lackner AA , Kuroda MJ .Contributions of nonhuman primates to research on aging. Vet Pathol.277-90. (2016) ;53: (2):277–90. doi: 10.1177/0300985815622974. PubMed PMID: 26869153; PubMed Central PMCID: PMCPMC5027759. |
[31] | Finch C , Austad S .Primate models for human brain aging andneurological diseases. Annual Review of Gerontology andGeriatrics.. (2014) ;34: (1):139–70. doi: 10.1891/0198-8794.34.139. |
[32] | Lacreuse A , Herndon JG .Nonhuman primate models of cognitiveaging, (2009) :1-30. doi: 10.1007/978-1-59745-422-32. |
[33] | Lane MA.Nonhuman primate models in biogerontology. Exp Gerontol. (2000) ;35: (5):533–41. PubMed PMID: 848 10978676. |
[34] | Mattison JA , Vaughan KL .An overview of nonhuman primates in aging research. Exp Gerontol. (2016) . doi: 10.1016/j.exger.2016.12.005. PubMed PMID: 27956088. |
[35] | Verdier JM , Acquatella I , Lautier C , Devau G , Trouche S , Lasbleiz C , et al., Lessons from the analysis of nonhuman primates for understanding human aging and neurodegenerative diseases. Front Neurosci.. (2015) ;9: :64. doi: 10.3389/fnins.2015.00064. PubMed PMID: 25788873; PubMed Central PMCID: PMCPMC4349082. |
[36] | Hoffman CL , Higham JP , Mas-Rivera A , Ayala JE , Maestripieri D .Terminal investment and senescence in rhesus macaques (Macaca mulatta) on Cayo Santiago. Behav Ecol.. (2010) ;21: (5):972–8. doi: 10.1093/beheco/arq098. PubMed PMID: 22475990; PubMed Central PMCID: PMCPMC2920293. |
[37] | Maestripieri D , Hoffman CL .Chronic stress, allostatic load, and aging in nonhuman primates. Dev Psychopathol.. (2011) ;23: (4):1187–95. doi: 10.1017/S0954579411000551. PubMed PMID: 22018089; PubMed Central PMCID: PMCPMC3942660. |
[38] | Colman RJ , Anderson RM .Nonhuman primate calorie restriction. Antioxid Redox Signal.. (2011) ;14: (2):229–39. doi: 10.1089/ars.2010.3224. PubMed PMID: 20698791; PubMed Central PMCID: PMCPMC3000242. |
[39] | Colman RJ , Beasley TM , Allison DB , Weindruch R .Attenuation of sarcopenia by dietary restriction in rhesus monkeys. J Gerontol A Biol Sci Med Sci.. (2008) ;63: (6):556–9. PubMed PMID: 18559628; PubMed Central PMCID: PMCPMC2812805. |
[40] | Colman RJ , Beasley TM , Allison DB , Weindruch R .Skeletal effects of long-term caloric restriction in rhesus monkeys. Age (Dordr). (2012) ;34: (5):1133–43. doi: 10.1007/s11357-011-9354-x. PubMed PMID: 22189911; PubMed Central PMCID: PMCPMC3448987. |
[41] | Colman RJ , Beasley TM , Kemnitz JW , Johnson SC , Weindruch R , Anderson RM .Caloric restriction reduces age-related and all-cause mortality in rhesus monkeys. Nat Commun. (2014) ;5: :3557. doi: 10.1038/ncomms4557. PubMed PMID: 24691430; PubMed Central PMCID: PMCPMC3988801. |
[42] | Kemnitz JW.Calorie restriction and aging in nonhuman primates. Institute for Laboratory Animal Research Journal. (2011) ;52: (1), 66–77. |
[43] | Mattison JA , Colman RJ , Beasley TM , Allison DB , Kemnitz JW , Roth GS , et al., Caloric restriction improves health and survival of rhesus monkeys. Nat Commun.. (2017) ;8: :14063. doi: 10.1038/ncomms14063. PubMed PMID: 28094793. |
[44] | Mattison JA , Roth GS , Beasley TM , Tilmont EM , Handy AM , Herbert RL , et al., Impact of caloric restriction on health and survival in rhesus monkeys from the NIA study. Nature.. (2012) :318–21. doi: 10.1038/nature11432. PubMed PMID: 22932268; PubMed Central PMCID: PMCPMC3832985. |
[45] | Smucny DA , Allison DB , Ingram DK , Roth GS , Kemnitz JW , Kohama SG , et al., Changes in blood chemistry and hematology variables during aging in captive rhesus macaques (Macaca mulatta). Journal of Medical Primatology. (2001) ;30: , 161–73. |
[46] | AustadSN.Small nonhuman primates as potential models of human aging. Institute for Laboratory Animal Research Journal. (1997) ;38: (3), 142–7. |
[47] | Fischer KE , Austad SN .The development of small primate models for aging research. Institute for Laboratory Animal Research Journal. (2011) ;52: (1), 78–88. |
[48] | Pifferi F , Terrien J , Marchal J , Dal-Pan A , Djelti F , Hardy I , et al.,Caloric restriction increases lifespan but affects brainintegrity in grey mouse lemur primates. Communications Biology.. (2018) ;1: (1):30. doi: 10.1038/s42003-018-0024-8. |
[49] | MansfieldKG.Marmoset models commonly used in biomedical research. Comparative Medicine. (2003) ;53: (4), 383–92. |
[50] | Ross CN , Davis K , Dobek G , Tardif SD .Aging phenotypes of common marmosets (Callithrix jacchus). Journal of Aging Research. (2012) ;2012: :567143. |
[51] | Ross CN , Salmon AB , Strong R , Fernandez E , Javors M , Richardson A , et al., Metabolic consequences of long-term rapamycin exposure on common marmoset monkeys (Callithrix jacchus). Aging. (2015) ;7: (11), 1–10. |
[52] | Salmon AB.Moving toward ‘common’ use of the marmoset as a non-human primate aging model. Pathobiol Aging Age Relat Dis.. (2016) ;6: :32758. doi: 10.3402/pba.v6.32758. PubMed PMID: 27452489; PubMed Central PMCID: PMCPMC4958916. |
[53] | Tardif SD , Abee CR , Mansfield KG .Workshop summary: Neoptropical primates in biomedical research. Institute for Laboratory Animal Research Journal. (2011) ;52: (3), 386–92. |
[54] | Tardif SD , Mansfield KG , Ratnam R , Ross CN , Ziegler TE .The marmoset as a model of aging and age-related disease. Institute for Laboratory Animal Research Journal. (2011) ;52: (1), 54–65. |
[55] | Tardif SD , Arauajuo A , Arruda MF , French JA , Sousa MB , Yamamoto K Reproduction and aging in marmosels and tamarins. In: Atsalis S, Margulis SW, editors. Primate Reproductive Aging: Interdisciplinary topics in gerontology and geriatrics: Karger; (2008) . pp. 29–48. |
[56] | Ross CN , Austad S , Brasky K , Brown CJ , Forney LJ , Gelfond JA , et al., The development of a specific pathogen free (SPF) barrier colony of marmosets (Callithrix jacchus) for aging research. Aging (Albany NY).. (2017) ;9: (12):2544–58. doi: 10.18632/aging.101340. PubMed PMID: 29227963; PubMed Central PMCID: PMCPMC5764392. |
[57] | Bagi CM , Volberg M , Moalli M , Shen V , Olson E , Hanson N , et al., Age-related changes in marmoset trabecular and cortical bone and response to alendronate therapy resemble human bone physiology and architecture. Anat Rec (Hoboken).. (2007) ;290: (8):1005–16. doi: 10.1002/ar.20561. PubMed PMID: 17610276. |
[58] | Bernick S , Cailliet R , Levy BM .The maturation and aging of the vertebrae of marmosets. Spine. (1980) ;5: (6), 519–24. |
[59] | Power RA , Power ML , Layne DG , Jaquish CE , Oftedal OT , Tardif SD .Relations among measures of body composition, age, and sex in the common marmoset monkey (Callithrix jacchus). Comparative Medicine. (2001) ;51: (3), 281–23. |
[60] | Frisard MI , Broussard A , Davies SS , Roberts LJ , Rood J , de Jonge L , et al., Aging, resting metabolic rate, and oxidative damage: Results from the louisiana healthy aging study. The Journals of Gerontology Series A, Biological Sciences and Medical Sciences.. (2007) ;62: (7):752–9. PubMed PMID: PMC2692620. |
[61] | Leuner B , Kozorovitskiy Y , Gross CG , Gould E .Diminished adult neurogenesis in the marmoset brain precedes old age. Proc Natl Acad Sci U S A.. (2007) ;104: (43):17169–73. doi: 10.1073/pnas.0708228104. PubMed PMID: 17940008; PubMed Central PMCID: PMCPMC2040400. |
[62] | Honavar M , Lantos PL .Ultrastructural changes in the frontal cortex and hippocampus in the aging marmoset. Mechanisms of Aging and Development. (1987) ;41: , 161–75. |
[63] | Kobayashi R , Takahashi-Fujigasaki J , Shiozawa S , Hara-Miyauchi C , Inoue T , Okano HJ , et al., Alpha-Synuclein aggregation in the olfactory bulb of middle-aged common marmoset. Neurosci Res.. (2016) ;106: :55–61. doi: 10.1016/j.neures.2015.11.006. PubMed PMID: 26643383. |
[64] | Geula C , Nagykery N , Wu C-K .Amyloid-B deposits in the cerebral cortex of the aged common marmoset (Callithrix jacchus): Incidence and chemical composition. Acta Neuropathol. (2002) ;103: , 48–58. |
[65] | Ridley RM , Baker HF , Windle CP , Cummings RM .Very long term studies of the seeding of beta-amyloidosis in primates. Journal of Neural Transmission. (2006) ;113: , 1243–51. |
[66] | Rodriguez-Callejas JD , Fuchs E , Perez-Cruz C .Evidence of Tau hyperphosphorylation and dystrophic microglia in the common marmoset. Frontiers in Aging Neuroscience. (2016) ;8: , 315. |
[67] | Klonoff-Cohen H , Barrett-Connor EL , Edelstein SL .Albumin levels as a predictor of mortality in the healthy elderly. J Clin Epidemiol.. (1992) ;45: (3):207–12. PubMed PMID: 1569417. |
[68] | Go YM , Liang Y , Uppal K , Soltow QA , Promislow DE , Wachtman LM , et al.,Metabolic characterization of the common marmoset (CallithrixJacchus). PLoS One.. (2015) ;10: (11):e0142916. doi: 10.1371/journal.pone.0142916. PubMed PMID: 26581102; PubMed Central PMCID: PMCPMC4651467. |
[69] | Hoffman JM , Tran V , Wachtman LM , Green CL , Jones DP , Promislow DE .A longitudinal analysis of the effects of age on the blood plasma metabolome in the common marmoset, Callithrix jacchus. Exp Gerontol.. (2016) ;76: :17–24. doi: 10.1016/j.exger.2016.01.007. PubMed PMID: 1007 26805607; PubMed Central PMCID: PMCPMC4775367. |
[70] | Soltow QA , Jones DP , Promislow DE .A netweork persepective on metabolism and aging. Integrative and Comparative Biology. (2010) ;50: (5), 844–54. |
[71] | Mietsch M , Baldauf K , Reitemeier S , Suchowski M , Schoon HA , Einspanier A .Blood pressure as prognostic marker for body condition, cardiovascular, and metabolic diseases in the common marmoset (Callithrix jacchus). J Med Primatol.. (2016) ;45: (3):126–38. doi: 10.1111/jmp.12215. PubMed 1016 PMID: 27144322. |
[72] | Kelnar CJ , McKinnell C , Walker M , Morris KD , Wallace WH , Saunders PT , et al., Testicular changes during infantile ‘quiescence’ in the marmoset and their gonadotrophin dependence: A model for investigating susceptibility of the prepubertal human testis to cancer therapy? Hum Reprod. (2002) ;17: (5):1367–78. PubMed PMID: 11980767. |
[73] | Kholkute SD , Aitken RJ , Lunn SF .Plasma testosterone response to hCG stimulation in the male marmoset monkey (Callithrix jacchus jacchus). J Reprod Fertil. (1983) ;67: (2):457–63. PubMed PMID: 6403699. |
[74] | Nunes S , Fite JE , French JA .Variation in steroid hormones associated with infant care behaviour and experience in male marmosets (Callithrix kuhlii). Anim Behav.. (2000) ;60: (6):857–65. doi: 10.1006/anbe.2000.1524. PubMed PMID: 11124885. |
[75] | KenyonCJ.The genetics of ageing. Nature.. (2010) ;464: :504. doi: 10.1038/nature08980 |
[76] | Weindruch R , Walford RL , Fligiel S , Guthrie D .The retardation ofaging in mice by dietary restriction: Longevity, cancer, immunityand lifetime energy intake. The Journal of Nutrition.. (1986) ;116: (4). |
[77] | Yang SH , Cheng PH , Banta H , Piotrowska-Nitsche K , Yang JJ , Cheng EC , et al., Towards a transgenic model of Huntington’s disease in a non-human primate. Nature. (2008) :921–4. doi: 10.1038/nature06975. PubMed PMID: 18488016; PubMed Central PMCID: PMC2652570. |
[78] | Seita Y , Tsukiyama T , Iwatani C , Tsuchiya H , Matsushita J , Azami T , et al., Generation of transgenic cynomolgus monkeys that express green fluorescent protein throughout the whole body. Scientific Reports.. (2016) ;6: :24868. doi: 10.1038/srep24868 https://www.nature.com/articles/srep 24868#supplementary-information. |
[79] | Sasaki E , Suemizu H , Shimada A , Hanazawa K , Oiwa R , Kamioka M , et al., Generation of transgenic non-human primates with germline transmission. Nature.. (2009) :523–7. doi: 10.1038/nature08090. PubMed PMID: 19478777. |
[80] | Sasaki E.Prospects for genetically modified non-human primate models, including the common marmoset. Neurosci Res.. (2015) ;93: :110–5. doi: 10.1016/j.neures.2015. 01.011. PubMed PMID: 25683291. |
[81] | Tomioka I , Ishibashi H , Minakawa EN , Motohashi HH , Takayama O , Saito Y , et al., Transgenic monkey model of the polyglutamine diseases recapitulating progressive neurological symptoms. eNeuro. (2017) ;4: (2). doi: 10.1523/ENEURO.0250-16.2017. PubMed PMID: 28374014; PubMed Central PMCID: PMCPMC5368386. |
[82] | Tomioka I , Nogami N , Nakatani T , Owari K , Fujita N , Motohashi H , et al., Generation of transgenic marmosets using a tetracyclin-inducible transgene expression system as a neurodegenerative disease model. Biol Reprod.. (2017) ;97: (5):772–80. doi: 10.1093/biolre/iox129. PubMed PMID: 29045563. |
[83] | Park JE , Zhang XF , Choi SH , Okahara J , Sasaki E , Silva AC .Generation of transgenic marmosets expressing genetically encoded calcium indicators. Sci Re1. (2016) ;6: :34931. doi: 10.1038/srep34931. PubMed PMID: 27725685; PubMed Central PMCID: PMCPMC5057151. |
[84] | Tardif S , Power M , Layne D , Smucny D , Ziegler T .Energy restriction initiated at different gestational ages has varying effects on maternal weight gain and pregnancy outcome in common marmoset monkeys (Callithrix jacchus). The British journal of nutrition.. (2004) ;92: (5):841–9. PubMed PMID: 15533274. |
[85] | Tardif SD , Power ML , Ross CN , Rutherford JN , Layne-Colon DG , Paulik MA .Characterization of obese phenotypes in a small nonhuman primate, the common marmoset (Callithrix jacchus). Obesity (Silver Spring).. (2009) ;17: (8):1499–505. doi: 10.1038/oby.2009.77. PubMed PMID: 19325546; PubMed Central PMCID: PMCPMC3823549. |
[86] | Miller CT , Freiwald WA , Leopold DA , Mitchell JF , Silva AC , Wang X .Marmosets: A neuroscientific model of human social behavior. Neuron.219-33. (2016) ;90: (2):219–33. doi: 10.1016/j.neuron.2016.03.018. PubMed PMID: PMC4840471. |
[87] | Soars MG , Riley RJ , Burchell B .Evaluation of the marmoset as amodel species for drug glucuronidation. Xenobiotica; the Fate ofForeign Compounds in Biological Systems.. (2001) ;31: (12):849–60. doi: 10.1080/00498250110069690. PubMed PMID: 11780760. |
[88] | VanderVeen N , Paran C , Appelhans A , Krasinkiewicz J , Lemons R , Appelman H , et al., Marmosets as a preclinical model for testing “off-label” use of doxycycline to turn on Flt3L expression from high-capacity adenovirus vectors. Molecular Therapy — Methods & Clinical Development.. (2014) ;1. doi: 10.1038/mtm.2013.10 http://www.nature.com/articles/mtm201310#supplementary-information. PubMed Central PMCID: PMC4111110. |
[89] | Orsi A , Rees D , Andreini I , Venturella S , Cinelli S , Oberto G .Overview of the marmoset as a model in nonclinical development ofpharmaceutical products. Regulatory Toxicology and Pharmacology.. (2011) ;59: (1):19–27. doi: https://doi.org/10.1016/j.yrtph.2010.12.003 |
[90] | Wilkinson JE , Burmeister L , Brooks SV , Chan C-C , Friedline S , Harrison DE , et al., Rapamycin slows aging in mice. Aging Cell. (2012) ;11: (4):675–82. doi: 10.1111/j.1474-9726.2012.00832.x. |
[91] | Flynn JM , O’Leary MN , Zambataro CA , Academia EC , Presley MP , Garrett BJ , et al., Late-life rapamycin treatment reverses age-related heart dysfunction. Aging Cell.851-62. (2013) ;12: (5):851–62. doi: 10.1111/acel.12109. PubMed Central PMCID: PMC4098908. |
[92] | Zhang Y , Bokov A , Gelfond J , Soto V , Ikeno Y , Hubbard G , et al., Rapamycin extends life and health in C57BL/6 mice. The Journals of Gerontology Series A: Biological Sciences and Medical Sciences.. (2014) 2:119–30. doi: 10.1093/gerona/glt056. PubMed Central PMCID: PMC4038246. |
[93] | Dai D-F , Karunadharma PP , Chiao YA , Basisty N , Crispin D , Hsieh EJ , et al., Altered proteome turnover and remodeling by short-term caloric restriction or rapamycin rejuvenate the aging heart. Aging Cell.. (2014) ;13: (3):529–39. doi: 10.1111/acel.12203. PubMed Central PMCID: PMC4040127. |
[94] | Hurez V , Dao V , Liu A , Pandeswara S , Gelfond J , Sun L , et al., Chronic mTOR inhibition in mice with rapamycin alters T, B, myeloid, and innate lymphoid cells and gut flora and prolongs life of immune-deficient mice. Aging Cell.945-56. (2015) ;14: (6):945–56. doi: 10.1111/acel.12380. PubMed PMID: 26315673; PubMed Central PMCID: PMC4693453. |
[95] | Chiao YA , Kolwicz SC , Basisty N , Gagnidze A , Zhang J , Gu H , et al., Rapamycin transiently induces mitochondrial remodeling to reprogram energy metabolism in old hearts. Aging (Albany NY).. (2016) ;8: (2):314–27. PubMed PMID: 26872208; PubMed Central PMCID: PMC4789585. |
[96] | Chen C , Liu Y , Liu Y , Zheng P .mTOR regulation and therapeuticrejuvenation of aging hematopoietic stem cells. Sci Signal.. (2009) ;2: (98):ra75. doi: 10.1126/scisignal.2000559. |
[97] | Mannick JB , Del Giudice G , Lattanzi M , Valiante NM , Praestgaard J , Huang B , et al., mTOR inhibition improves immune function in theelderly. Science Translational Medicine.. (2014) ;6: (268):268ra179. doi: 10.1126/sci-translmed.3009892. |
[98] | Kraig E , Linehan LA , Liang H , Romo TQ , Liu Q , Wu Y , et al., A randomized control trial to establish the feasibility and safety of rapamycin treatment in an older human cohort: Immunological, physical performance, and cognitive effects. Exp Gerontol.. (2018) ;105: :53–69. doi: 10.1016/j.exger.2017.12.026. PubMed PMID: 29408453; PubMed Central PMCID: PMC5869166. |
[99] | Tardif S , Ross C , Bergman P , Fernandez E , Javors M , Salmon A , et al., Testing efficacy of administration of the antiaging drug rapamycin in a nonhuman primate, the common marmoset. J Gerontol A Biol Sci Med Sci.. (2015) ;70: (5):577–87. doi: 10.1093/gerona/glu101. PubMed PMID: 25038772; PubMed Central PMCID: PMC4400395. |
[100] | Liu Y , Diaz V , Fernandez E , Strong R , Ye L , Baur JA , et al., Rapamycin-induced metabolic defects are reversible in both lean and obese mice. Aging (Albany NY).. (2014) ;6: (9):742–54. PubMed PMID: 25324470. |
[101] | Lamming DW , Ye L , Katajisto P , Goncalves MD , Saitoh M , Stevens DM , et al., Rapamycin-induced insulin resistance is mediated by mTORC2 loss and uncoupled from longevity. Science.. (2012) :1638–43. doi: 10.1126/science.1215135. PubMed Central PMCID: PMC3324089. |
[102] | Weiss R , Fernandez E , Liu Y , Strong R , Salmon AB .Metformin reduces glucose intolerance caused by rapamycin treatment in genetically heterogeneous female mice. Aging (Albany NY). (2018) . doi: 10.18632/aging.101401. PubMed PMID: 29579736. |
[103] | Ross C , Salmon A , Strong R , Fernandez E , Javors M , Richardson A , et al., Metabolic consequences of long-term rapamycin exposure on common marmoset monkeys (Callithrix jacchus). Aging (Albany NY).. (2015) ;7: (11):964–73. PubMed PMID: 26568298; PubMed Central PMCID: PMC4694066. |
[104] | Zhao J , Zhai B , Gygi SP , Goldberg AL .mTOR inhibition activatesoverall protein degradation by the ubiquitin proteasome system aswell as by autophagy. Proc Natl Acad Sci U S A.. (2015) ;112: (52):15790–7. doi: 10.1073/pnas.1521919112. PubMed PMID: 26669439; PubMed Central PMCID: PMC4703015. |
[105] | Diaz-Troya S , Perez-Perez ME , Florencio FJ , Crespo JL .The role of TOR in autophagy regulation from yeast to plants and mammals. Autophagy.. (2008) ;4: (7):851–65. PubMed PMID: 18670193. |
[106] | Conn CS , Qian SB .mTOR signaling in protein homeostasis: Less is more? Cell Cycle. (2011) ;10: (12):1940–7. PubMed PMID: 21555915; PubMed Central PMCID: PMC3154417. |
[107] | Zhang Y , Nicholatos J , Dreier JR , Ricoult SJ , Widenmaier SB , Hotamisligil GS , et al., Coordinated regulation of protein synthesis and degradation by mTORC1. Nature. (2014) :440–3. doi: 10.1038/nature13492. PubMed PMID: 25043031; PubMed Central PMCID: PMC4402229. |
[108] | Lelegren M , Liu Y , Ross C , Tardif S , Salmon AB .Pharmaceutical inhibition of mTOR in the common marmoset: Effect of rapamycin on regulators of proteostasis in a non-human primate. Pathobiol Aging Age Relat Dis.. (2016) ;6: :31793. doi: 10.3402/pba.v6.31793. PubMed PMID: 27341957; PubMed Central PMCID: PMC4920937. |
[109] | An JY , Quarles EK , Mekvanich S , Kang A , Liu A , Santos D , et al., Rapamycin treatment attenuates age-associated periodontitis in mice. GeroScience. (2017) ;39: (4):457–63. doi: 10.1007/s11357-017-9994-6. PubMed PMID: PMC5636779. |
[110] | López-Otín C , Blasco MA , Partridge L , Serrano M , KroemerG.The hallmarks of aging. Cell. 153: (6):1194–217. doi: 10.1016/j.cell.2013.05.039. |