Ceramide dependent lipotoxicity in metabolic diseases
Abstract
Sphingolipids, a major class of lipids in cell membranes, play diverse roles in biology. They are synthesized by a highly conserved biosynthetic pathway that leads to the production of ceramides, the major precursors of most complex sphingolipids. Almost all known stress stimuli including inflammatory agonists, chemotherapeutics, and saturated fatty acids induce the synthesis of ceramide and its metabolites. A panoply of recent studies has implicated ceramides in the development of the metabolic comorbidities of obesity such as diabetes and cardiovascular diseases. In particular, inhibition of ceramide biosynthesis in rodents ameliorates insulin resistance, diabetes, cardiomyopathy, atherosclerosis, and steatohepatitis. These data implicate ceramides as major contributors to the development of metabolic diseases. This review summarizes recent findings on this emerging class of bioactive lipids with an emphasis on studies using in vivo models to understand their role in metabolic disease.
1Introduction
The majority of obese individuals develop insulin resistance, a condition characterized by impaired cellular responses towards insulin [1]. If not controlled, prolonged insulin resistance increases risk for Type 2 Diabetes and cardiovascular disease [2]. A large body of evidence suggests that lipid-induced insults (lipotoxicity) in metabolic tissues drives the initiation and progression of insulin resistance, diabetes and metabolic disorders [3].
As fatty acids enter cells, they are rapidly converted to acyl-CoA’s before undergoing one of three metabolic fates. They can be coupled to (a) glycerol to produce glycerolipids (e.g. triacylglycerol, diacylglycerol, phosphatidylcholine, etc.), (b) carnitine for delivery into mitochondria to produce acyl-CoA for ATP production or cholesterol synthesis, or (c) serine to generate sphingolipids (e.g. ceramide, sphingomyelin). Sphingolipids are the least abundant (∼20% of glycerolipids) [4, 5]. However, when they accumulate above a critical threshold, they impair insulin action in adipose tissue, skeletal muscle, and/or the liver [6, 7] and modulate energy metabolism [7, 8]. Moreover, adiponectin appears to elicit its anti-diabetic and cardioprotective actions by activating receptors with intrinsic ceramidase activity to degrade ceramides [9, 10]. In addition, saturated fatty acids (SFAs) induce their antagonistic effects on insulin signaling in peripheral tissues, such as the skeletal muscle, by enhancing activation of TLR-4 receptor signaling to increase biosynthesis of ceramides [6]. In this review, we provide a perspective on in vivo studies implicating ceramides in the development of metabolic diseases.
2Ceramide synthesis and metabolism
Although ceramides are prevalent in the diet, they are largely degraded in the mammalian intestine [11]. Their production in animal tissues is driven by a conserved de novo ceramide synthesis pathway which begins in the endoplasmic reticulum with the condensation of palmitoyl-CoA and serine, catalyzed by the enzyme serine palmitoyltransferase (Spt 1-3), to produce 3-ketosphinganine (Fig. 1) [12]. Three subsequent reactions follow: 3-ketosphinganine reductase (3Ksn) generates sphinganine, which is then n-acylated by (dihydro) ceramide synthase (Cers 1–6) to produce dihydroceramide. Then, desaturases (Des1 and 2) introduce a distinctive double bond in dihydroceramide to produce ceramides. The diversity in the sphingolipid family results from a family of mammalian Cers (Cers1–6), which add fatty acids of different chain lengths to the sphingoid backbone, leading to the ultimate generation of ceramide with variable acyl chain lengths ranging from 14-carbon to 34-carbon atoms (Fig. 1) [13]. Importantly, ceramides with varying acyl chain compositions are generated in specific tissue and cell types depending on the physiological and pathological state which show differential effects on the development of metabolic diseases [14, 15]. The double bond introduced by dihydroceramide desaturase imparts many of ceramide’s unique biophysical properties [12].
Fig.1
Schematic illustration of ceramide synthesis and its action in metabolic tissues. Free fatty acids, palmitate and inflammatory agonists stimulate the synthesis of ceramides. Excess accumulation of ceramides in insulin responsive tissues inhibits AKT/PKB resulting in reduced insulin response. In addition, ceramides, elicit its deleterious effect by inhibiting mitochondrial function and inducing ER stress. Inhibition of ceramide synthetic pathway improves insulin sensitivity. Similarly, FGF21 and adiponectin exhibits its beneficial effect partially by regulating rates of conversion of ceramide to sphingosine. Abbreviations of enzymes: Cers: Ceramide synthase; Des: desaturase; Ksn: 3-ketosphinganine reductase; Smase: sphingomyelinase; Sptlc: serine palmitoyltransferase.
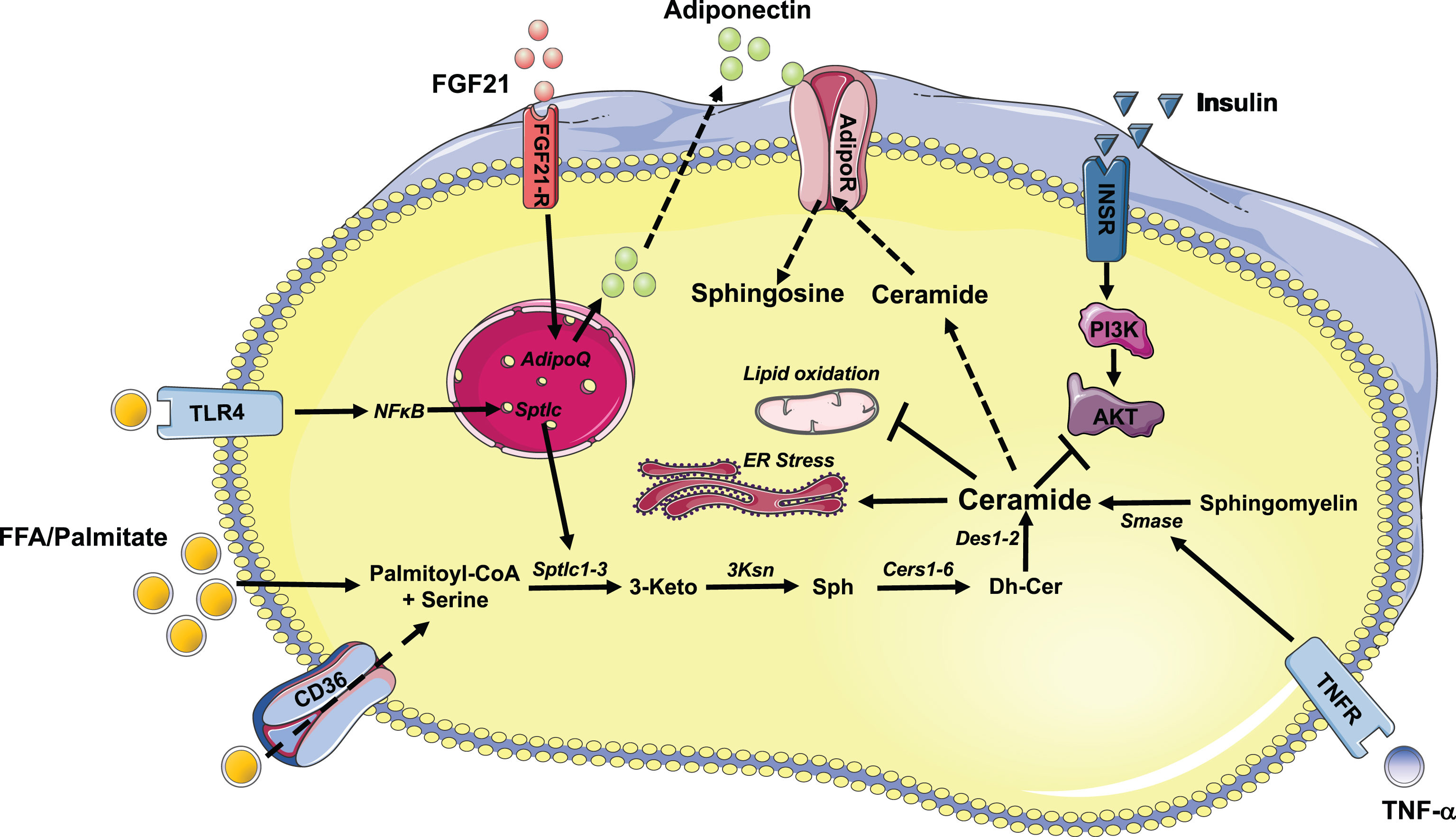
3Regulation of ceramide production in obesity
The question as to how and when ceramides accumulate in obesity has attracted considerable attention. The initial assumption was that increased supply of the substrates palmitate and serine from over-nutrition was the major source of tissue ceramides in obesity. However, recent studies have revealed that tissue ceramides are also regulated by hormonal cues, which modulate rates of ceramide synthesis and degradation [6, 16]. In this section, we will present evidence gained over the years that has led to the refinement of the initial hypothesis.
3.1Ceramides in obesity induced inflammation
Obesity is associated with chronic low grade inflammation characterized by increased recruitment and activation of macrophages to adipose tissue, resulting in augmented expression and secretion of inflammatory cytokines [17, 18]. These inflammatory cytokines (e.g. TLR4 agonists, TNF-α, interleukins, etc.) all increase levels of sphingolipids, generally without affecting glycerolipids [19]. The prevailing wisdom is that these inflammatory triggers work in concert with excessive nutrient availability to drive sphingolipid production. Circulating inflammatory cytokines consistently show a particularly tight association with circulating ceramides and insulin resistance [19, 20].
Several findings support the involvement of the innate immunity receptor toll-like receptor 4 (TLR4), which is either stimulated or amplified by saturated fats, as an important modulator of lipid-induced insulin resistance and ceramide synthesis [6]. Indeed, the preponderance of data reveal that TLR4-induced ceramide synthesis is an essential component of fat-induced insulin resistance. Lipopolysaccharide (LPS), a TLR4 agonist, selectively upregulates de novo ceramide synthesis. Moreover, mice lacking TLR4 fail to accumulate ceramide in the presence of elevated saturated fatty acids (Fig. 1) [6, 21, 22]. These data establish TLR4 signaling as an essential component linking saturated fats to the modulation of ceramide synthesis and anabolic metabolism. Mechanistically, these effects are partially mediated by activation of the Nod-like receptor (Nlrp3) inflammasome, which senses ceramides to induce caspase-1 cleavage in macrophages and adipose tissue, and by contributing to the development of insulin resistance by inhibiting AKT activation [23, 24].
3.2FGF21-adiponectin-ceramide axis
The adipokine adiponectin has received considerable attention for its potential anti-diabetic actions. Adiponectin regulates glucose and lipid homeostasis through actions in the liver, adipose, and pancreatic tissue [25–27]. In addition, adiponectin regulates lipid spillover into non-adipose tissue by governing rates of lipid synthesis, oxidation and lipolysis, as well as by inhibiting inflammation. These beneficial effects of adiponectin were previously thought to be mediated by AMPK, a serine/threonine kinase [25]. However, the Scherer group has recently demonstrated that adiponectin receptors AdipoR1 and 2 stimulate deacylation of ceramide, yielding sphingosine that can be converted into sphingosine 1-phosphate (S1P) by sphingosine kinases [9]. The resulting sphingosine and/or S1P prevent apoptosis of pancreatic β-cells and cardiomyocytes and exert an anti-diabetic effect. Moreover, once synthesized, S1P is transported to the extracellular environment and binds to the S1P receptors to activate AMPK [9]. Consistent with this, Tanabe et al. initially showed that crystal structures of human AdipoRs possess a hydrophobic binding pocket potentially resembling that of the ceramidases [28]. More recently, Vasiliauskaite-Brooks et al. showed that purified adiponectin receptors possess inherent ceramidase enzymatic activity (Fig. 1) [10]. Moreover, they solved the crystal structure in the presence of ceramide, obtaining a final entity bound to a fatty acid product of the reaction [10].
FGF21, a member of fibroblast growth factor (FGF), has garnered a considerable amount of attention because of its ability to modulate glucose and lipid homeostasis and whole-animal energy utilization [29, 30]. In a series of elegant studies, Scherer and colleagues recently demonstrated that FGF21 stimulates adiponectin secretion in rodents, thereby decreasing ceramide levels. Interestingly, the deletion of adiponectin renders rodents’ refractory to FGF21, at least with regards to its effects on ceramide levels and energy metabolism. Collectively, these studies demonstrate the presence of an FGF21-adiponectin-ceramide axis that modulates glucose and energy homeostasis (Fig. 1) [31].
3.3Ceramide, gut microbiota, and obesity
Oral transplantation of cecal microbiota derived from obese mice into lean germ free mice leads to an increase in hepatic triglyceride content [32], which demonstrates that alteration of the gut microbiota contributes to the development of obesity and its comorbidities. However, the mechanisms linking gut microbiota to metabolic homeostasis have been elusive. Recently, Gonzalez and colleagues found that gut microbiota regulate a bile acid/intestinal FXR axis to alter ceramide pathways, which may led to hepatic triglyceride accumulation [33]. Moreover, their study goes on to demonstrate that depleting the gut microbiota with antibiotics reduces transcripts encoding for the genes involved in ceramide biosynthesis in the ileum and cecum and lowers serum ceramide levels, an effect mediated by the intestinal FXR receptor. The authors further present data suggesting that improvements in hepatic steatosis result from FXR dependent downregulation of ceramide and hepatic Srebp1c and Cidea [33]. Their work further demonstrated that intestinal FXR modulates ceramides content in the gut to reduce hepatic mitochondrial acetyl-CoA levels and pyruvate carboxylase activities, thereby attenuating hepatic gluconeogenesis [34].
4Ceramides influence glucose homeostasis
Substantive evidence accumulated over the past decade has convincingly demonstrated that sphingolipids, especially ceramides and its metabolites, are key mediators of insulin resistance and related metabolic comorbidities [35]. Early studies identifying roles for ceramide in insulin resistance came from direct application of ceramide analogs to isolated skeletal muscles and cultured adipocytes [36, 37]. These studies revealed that ceramide inhibits insulin-stimulated glucose uptake and glycogen synthesis [38]. Subsequent to that, implementation of pharmacological and genetic strategies to inhibit synthesis of ceramide or glucosylceramides in rodent models of obesity was shown to increase insulin sensitivity [35]. Moreover, profiling studies revealed an inverse relationship between ceramides and insulin sensitivity in rodents, non-human primates and humans [39, 40]. The strength of the relationship is particularly strong when inflammation is considered in concert [6, 22, 41]. Mechanistically, cell-autonomous ceramide accumulation has been shown to inhibit AKT/PKB phosphorylation by activating protein phosphatase 2A, and blocking the translocation of AKT/PKB to the plasma membrane through PKCζ activation (Fig. 1) [42–44]. Studies using lipid infusion or isolated muscles reveal that ceramides are obligate intermediates linking saturated fatty acids, but not unsaturated ones, to the development of insulin resistance [6, 45–47].
In rodents, manipulation of ceramide synthesis or degradation pathways through pharmacologic or genetic means have profound effects on modulating insulin sensitivity [35]. Importantly, pharmacological inhibition of the ceramide biosynthetic enzymes SPT or DES1 using myriocin or fenretinide, respectively, elicits dramatic improvements in insulin action and glucose homeostasis in a high fat fed mice, fructose fed hamsters, leptin or leptin receptor deficient rats or mice, and dexamethasone treated rats or mice. [7, 48–50]. Moreover, mouse models bearing haploinsufficiency of either Sptlc2 or Des1, which are essential for ceramide synthesis, show substantial improvements in insulin sensitivity when exposed to high fat diet and/or dexamethasone [7, 51]. Another approach to reduce ceramide levels in rodents involves the overexpression of acid ceramidase, which converts ceramides into sphingosine. In cultured cells, the transgene negated the inhibitory effects of palmitate on insulin signaling [42, 45]. This approach was also efficacious in vivo, as overexpression of ceramidase in adipose tissue or the liver resolved impaired glucose tolerance [52].
Researchers are starting to obtain greater clarity on the influence of acyl chain length on ceramide action. Much of the work comes from cells or animals lacking one of the six-ceramide synthase enzymes (Cers1–6) that catalyze the n-acylation of sphinganine [13]. Studies involving the ablation of Cers2 and Cers6 in mice came to the common conclusion that C16-ceramides contributed to insulin resistance [14, 15]. First, the Brüning group demonstrated that genetic deletion of Cers6, the enzyme that adds the C16-acyl-chain, protects mice from HFD-induced obesity, glucose tolerance and insulin resistance [14]. Second, Summers and colleagues demonstrated that haploinsufficiency for Cers2 reduced C24-ceramides, but elicited a compensatory increase in C16-ceramides. The elevation of C16-ceramides led to impairments in glucose tolerance and insulin sensitivity [15]. Mechanistic studies suggest that the C16-ceramides impair metabolic homeostasis by inhibiting mitochondrial β−oxidation. Interestingly, genome wide association studies have identified a common Cers2 polymorphism, introducing a single amino acid substitution at position 115, that is strongly associated with insulin resistance [15, 53].
4.1Ceramides in adipose tissue
We recently completed a study doing a careful analysis of the role of ceramides in adipose tissue in vivo. The work was an offshoot of our studies with myriocin, a potent inhibitor of the enzyme SPT isolated from the fungus Isaria Sinclairii. The reagent has been used in a series of studies to reduce ceramides in rodents, which ameliorates insulin resistance and various other metabolic disorders in obese mice, rats, and hamsters [7, 48, 49, 54–57]. We found that it induced a broad spectrum of changes in the adipose bed including reduced adipocyte size, increase recruitment of M2 macrophages, and elevated numbers of brown/beige adipocytes in white adipose tissue, particularly in the subcutaneous depot [58]. We then found that adipose-specific ablation of Sptlc2 recapitulated the effects of myriocin including the improvement in insulin sensitivity and glucose tolerance, resolution of hepatic steatosis, recruitment of beige adipocyte in the adipose tissue, and improved mitochondrial function [58]. These adipose-specific changes were sufficient to increase whole-body energy expenditure. Based on these findings, we proposed that ceramides act as nutrient signals that direct the adipocyte towards a hypo-metabolic, rather than thermogenic phenotype (Fig. 2).
Fig.2
Model for Ceramide sensing in adipocytes and its systemic effects. (a) Ceramides are essential for the differentiation of pre-adipocytes into adipocytes. Ablation of Sptlc1/2 and pharmacological inhibition of ceramides biosynthesis in pre-adipocytes blocks differentiation. (b) Adipocyte ceramides serve as nutritional determinants to promote lipid storage and inhibit thermogenic capacity hence promoting “whitening” rather than “beigeing/britening” of adipocytes. Ablation of ceramide synthesis in mature adipocytes of obese mice promotes beigeing/britening of adipocytes which improves insulin sensitivity and mitochondrial function in adipocytes and has systemic effects on improving mitochondrial function, energy expenditure, glucose homeostasis and resolving hepatic steatosis.
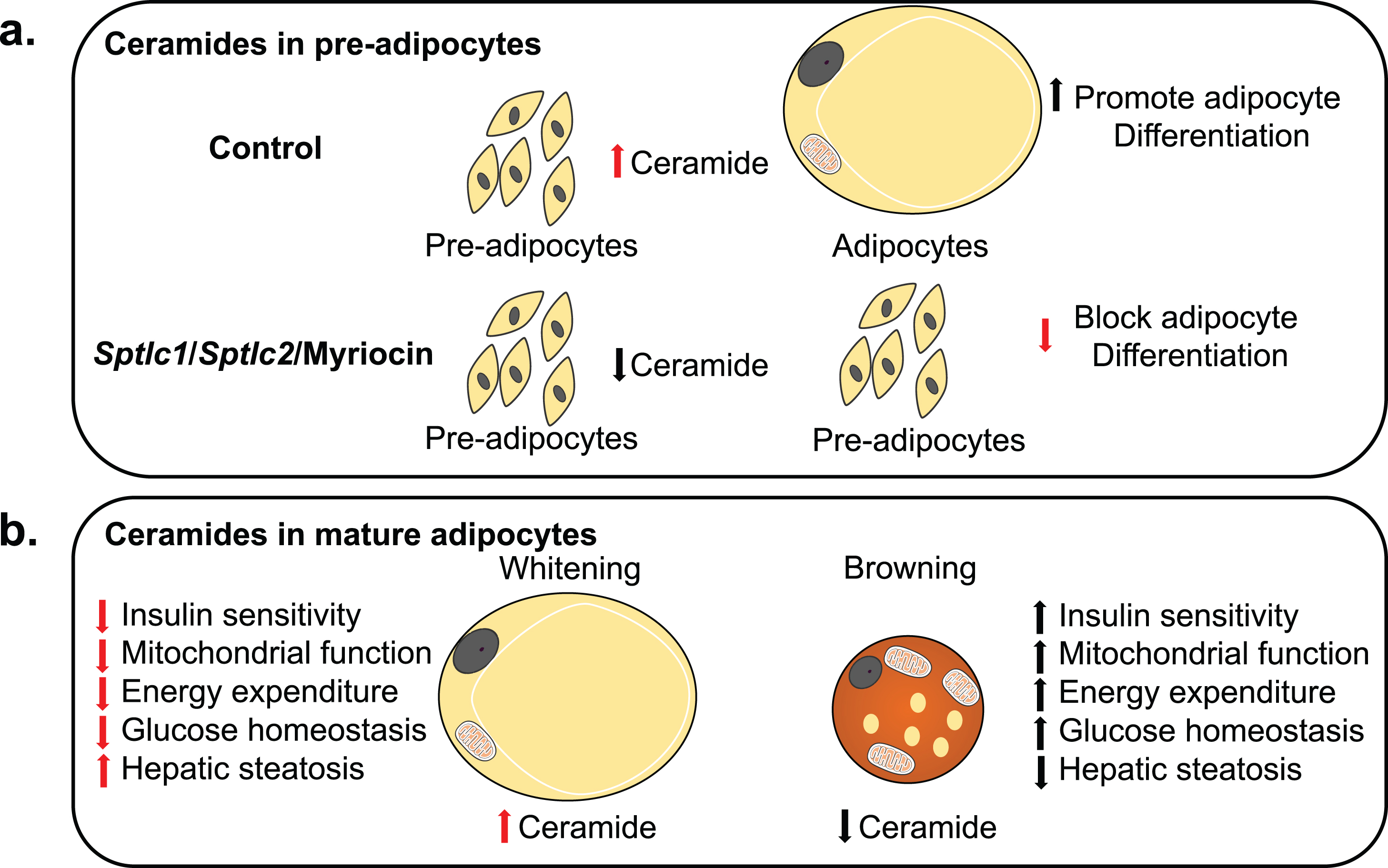
In congruence with these findings, Jiang et al. demonstrated that ectopic ceramides inhibit the browning of beige adipocytes, suggesting that endogenous ceramides could be autonomous regulators of adipocyte function [59]. We applied a similar approach in the aforementioned study, using various pharmacological reagents to manipulate levels of endogenous ceramides. Collectively, the work shows that the actions on the adipocyte were cell-autonomous and driven by ceramides, but not other sphingolipids.
Scherer and colleagues [52] used another approach to selectively reduce adipose ceramides. Specifically, they overexpressed acid ceramidase (Asah1) in adipose tissue. Though they did not report effects on adipose tissue browning/beiging, transgene induction in adipose tissue quickly (i.e. within 3 days) resolved hepatic steatosis and improved glucose tolerance, an effect that was similar to that observed with Sptlc2 ablation in adipose tissue [58].
Of note, recent papers by the Proia and Park laboratories found that ablation of either Sptlc1 or 2, respectively, in adipose tissue impaired adipose differentiation and elicited a lipodystrophic phenotype [60, 61]. In these studies, the authors used an adiponectin-Cre-recombinase line from Jackson Laboratories that expresses the transgene earlier in development [62]. We hypothesize that this accounts for the difference in phenotype. In concordance with their work, our studies in primary cells show that myriocin is a potent inhibitor of adipocyte differentiation [58].
Adipose tissue preferentially expresses a ceramide synthase (i.e. Cers6) that makes the deleterious C16-ceramides. Associations between adipose C16-ceramides and metabolic dysfunction have been observed [14]. Moreover, Cers6 expression is dramatically increased in obese individuals [14]. Turpin et al. generated mice lacking Cers6 in brown adipose tissue [14]. BAT-specific inhibition of Cers6 resolved hepatic steatosis, improved glucose tolerance, and enhanced mitochondrial β-oxidation and energy expenditure. These studies further highlight the importance of ceramide accumulation in BAT in regulating systemic metabolic homeostasis.
5Ceramides in the liver
Nonalcoholic fatty liver disease is characterized by an increased accumulation of triglycerides in hepatocytes. The condition is a major health problem that predisposes individuals to cardiovascular disease, liver cancer and cirrhosis [63]. The condition results from insulin resistance in adipose tissue, leading to increased lipolysis that liberates fatty acids destined for the liver, causing impairments in hepatic lipid oxidation. [64]. In mice and humans, the degree of hepatic steatosis and/or insulin resistance positively correlates with hepatic ceramides [65, 66]. Pharmacological inhibition of SPT by myriocin or DES1 by fenretinide in rodent models of obesity reduces hepatic lipid accumulation [49, 50].
The aforementioned studies on the CERS enzymes again support roles for C16-ceramides in promoting fat deposition in the liver via impaired lipid oxidation. In particular, Turpin et al. [14] demonstrated depletion of Cers6 from the liver protected obese mice from steatohepatitis and insulin resistance. Conversely, Cers2 depletion led to compensatory increases in CerS6 and C16-ceramides and predisposed mice to diet-induced steatohepatitis [15]. A subsequent study showed that mice lacking Cers5, which also contributes to C16-ceramide synthesis, exhibit reduced C16-ceramide content in liver, improved glucose metabolism and insulin sensitivity, and protection from hepatic steatosis [67].
The Scherer lab used the aforementioned system enabling inducible expression of acid ceramidase to study the role of ceramides in the liver. Overexpression of acid ceramidase (Asah1), which results in decreased hepatic ceramides, protected the animals from hepatic steatosis and improved insulin sensitivity [52]. This protection appeared to result from changes in hepatic lipid uptake, as they found that ceramide-induced translocation of the lipid transport protein CD36 to the cell membrane. PKCζ was an obligate intermediate in this newly identified ceramide action. Similarly, in liver specific inducible overexpression of adiponectin receptor (AdipoR), adiponectin decreased hepatic ceramide content, improved hepatic insulin resistance and protected against hepatic steatosis by increasing AdipoR-induced ceramidase activation [68].
6Ceramides in muscle
Despite the abundance of data from interventional studies indicating that ceramides improve insulin sensitivity, the relative importance of ceramides as regulatory factors in skeletal muscle metabolism has been contentious. The controversy stems from discordance in lipidomic profiling studies, as some groups have shown strong associations between muscle ceramides and insulin resistance [69–76], while others have found no such relationship [77–80]. Indeed, this issue has been discussed further in a recently published Crosstalk “debate” sponsored by the Journal of Physiology where investigators took oppositional positions about the roles for ceramides as modulators of muscle function [81, 82]. Despite this contention, the initial in vitro studies convincingly demonstrated that increasing ceramide content in skeletal muscle cells potently inhibits insulin signaling [42, 83, 84]. Conversely, pharmacological inhibition of ceramide synthesis or increased degradation of ceramides in myotubes, attenuates palmitate induced inhibition of insulin signaling [85]. Moreover, inhibiting ceramide synthesis was shown to negate palmitate-induced insulin resistance in isolated muscle strips and lipid-infused rodents [6, 7]. Nonetheless, muscle-specific manipulations of ceramide content have not been conducted. To further hone the tissue-specific role of ceramides in muscle, these studies should be pursued in the future.
7Ceramides in beta cells
Blocking ceramide production prevents the destruction of beta cells in rodent models of type 2 diabetes. Whether this is due to autonomous actions within the beta cell or is a consequence of its insulin-sensitizing properties is unclear. However, studies in cultured cells suggest that ceramide may have autonomous actions within the cell type. In particular, fatty acids have been shown to impair insulin secretion and insulin gene transcription, in addition to inducing apoptosis. The chronic adverse effects of FFAs on β-cell function and viability are potentiated in the presence of hyperglycemia, a phenomenon that has been termed gluco-lipotoxicity [24]. Ceramide has been shown to accumulate in β-cells exposed to either saturated fatty acids (lipotoxicity) or to hyper-physiologic glucose environment [86]. Moreover, ceramides are capable of inhibiting insulin gene expression, blocking proliferation, and inducing apoptosis both in mouse and human islets [87–96]. Glycosylated derivatives of ceramide (i.e. gangliosides) have been identified as putative antigens that contribute to the auto-immune response [97–100]. These in vitro studies suggest that ceramides could contribute to the decline in β-cell function that underlies diabetes. However, their roles in vivo (e.g. with tissue specific knockouts) have not been studied in sufficient detail and are essential for delineating the roles of ceramides in β-cell function.
8Ceramides in central nervous system
Data gleaned over the past decade have established the role of hypothalamic insulin and leptin signaling in modulating energy and glucose homeostasis [101, 102]. In particular, the Clegg laboratory found that introducing saturated fatty acids into the brain disrupts insulin signaling in the hypothalamus at the level of AKT/PKB [103]. In addition, the Summers group reported that ceramides accumulate in hypothalamus following either high fat diet feeding or acute lipid infusion [6]. Taken together these studies raise the interesting possibility that ceramides accumulate in the hypothalamus to modulate energy homeostasis. In support of this hypothesis, Contreras and colleagues recently demonstrated ceramide induced lipotoxicity in the hypothalamus modulates weight gain by reducing brown adipose tissue thermogenesis [104].
Additional studies suggest that glucosylated ceramides within the CNS may modulate peripheral metabolism. In particular, neuronal expression of glucosylceramide synthase (GCS), which regulates the synthesis of ceramide metabolite glucosylceramide, has also been implicated as a modulator of body weight and energy homeostasis [105]. Mice deficient in glycosphingolipids in the hypothalamus developed progressive obesity and displayed a decrease in sympathetically mediated thermogenesis [105]. Moreover, rAAV-mediated Ugcg (encoding for GCS) delivery to the hypothalamic arcuate nucleus led to ensuing elevations in nuclear glucosylceramides which ameliorated obesity. Mechanistically, GCS-depleted neurons displayed inadequate leptin receptor [42] activation, requiring neuronal gangliosides GM1 and GD1a to be recruited to the ObR upon ligand stimulation [105]. Although these observations suggest the essential requirement of GCS in regulating food intake, we cannot distinguish whether the obese phenotype in this animal model results from the lack of glucosylceramides or the accumulation of ceramides.
Although nascent, studies accumulated in recent years have refined our understanding of ceramide-mediated lipotoxicity in the hypothalamus in regulating energy homeostasis. These data raise interesting questions as to which orexigenic signals are modulated by ceramides or its metabolites.
9Ceramides in the heart and vasculature
An estimated 65% of people that die a cardiovascular death have either impaired glucose tolerance or diabetes [106]. Interestingly, inhibition of ceramide biosynthesis shows beneficial effects in several rodent models of cardiovascular diseases, including atherosclerosis, hypertension and cardiomyopathy [107–110]. Of note, ceramide has been identified as surrogate biomarkers that predict cardiovascular events, and clinical tests are being made available to patients [111].
Whether this protective effect of ceramide depletion interventions is due to improvements in glucose homeostasis or a result of autonomous effects in the vasculature or heart is unclear [24]. For example, the atherogenic effects of ceramide could also be due to autonomous effects on the vessel wall. Ceramides also induce transcytosis of oxidized low-density lipoproteins across endothelial cells, leading to the retention of lipids in the vascular wall [112] and promote monocyte adhesion to vessel walls [113], which provides a mechanism that could contribute to plaque formation. Furthermore, vascular dysfunction critically underlies cardiovascular diseases, including hypertension. Both myriocin and haploinsufficency for Des1 protect mice from diet-induced impairment in vascular function, negating hypertension [114]. Studies in isolated vessels exposed to palmitate imply that ceramides may also have autonomous actions in the vessel [114]. Ceramide was an obligate intermediate linking palmitate to the impairment in vascular reactivity. These effects were due to ceramide induced co-localization of protein phosphatase 2A (PP2A) with eNOS, leading to the inhibition of eNOS phosohorylation and its dissociation from AKT/PKB [114].
Obesity is associated with an increased incidence of cardiac dysfunction and cardiomyopathy that contributes to morbidity and mortality from cardiac infarction. Ceramides have been found in pathological specimens and worsen lipotoxicity induced cardiomyopathy [115]. Pharmacological or genetic inhibition of ceramide synthesis in a model of lipotoxic cardiomyopathy (i.e. cardiac specific overexpression of human lipoprotein lipase) abrogates apoptosis and cardiac contraction [115]. However, heart-specific deletion of Sptlc2 impairs cardiac function [116], making it difficult to ascertain whether ceramides that regulate cardio-lipotoxicity were generated within the cardiomyocyte.
10Conclusion
The redundancy of approaches utilized in rodent models so far strongly suggests that therapeutic strategies that reduce pathological ceramides should improve insulin sensitivity and help patients achieve better glycemic control. Moreover, such clinical interventions should delay or prevent the various co-morbidities of obesity, such as diabetes and heart disease. Nonetheless, a number of questions still remain [24, 117]. Firstly, our understanding of the tissue-specific roles of ceramides in disease etiology is still not fully defined. To this end, the advent of novel mouse tools enabling tissue-specific manipulation of ceramides will help to identify which tissues are most sensitive to ceramide accumulation. Secondly, though initial studies identified a couple of key mechanisms (i.e. regulation of AKT) for ceramide actions, the plethora of effects elicited by ceramide seems to be unlikely to be fully explained solely by this PP2A-AKT axis. Identifying additional molecular mechanisms will be crucial for understanding the roles of ceramides. Thirdly, ceramides are intermediate metabolites of the complex sphingolipids which are not in a static state and have a high degree of turnover. A better understanding the regulatory nodes in the ceramide biosynthetic pathway that are modulated during metabolic abnormalities could lead to the identification of better therapeutic targets. Despite these questions, the data thus far obtained place ceramides at the nexus of a nutrient signaling network that has profound effects on a wide variety of metabolic disease processes.
Acknowledgments
We wish to thank Prof. Scott A Summers for the critical reading of the manuscript. This work was supported by a grant from the Vice President Research Office, Funding Seed Grant Incentive, University of Utah (To BC). We apologize for the many excellent studies that were not discussed because of limited space. The figures in this manuscript were partially adapted from Servier Medical Art.
References
[1] | Kahn SE , Hull RL , Utzschneider KM . Mechanisms linking obesity to insulin resistance and type 2 diabetes. Nature. (2006) ;444: (7121):840–6. |
[2] | Meigs JB , Rutter MK , Sullivan LM , Fox CS , D’Agostino RB Sr , Wilson PW . Impact of insulin resistance on risk of type 2 diabetes andcardiovascular disease in people with metabolic syndrome. DiabetesCare. (2007) ;30: (5):1219–25. |
[3] | Unger RH , Scherer PE . Gluttony, sloth and the metabolic syndrome: A roadmap to lipotoxicity. Trends Endocrinol Metab. (2010) ;21: (6):345–52. |
[4] | Meikle PJ , Wong G , Tan R , Giral P , Robillard P , Orsoni A , et al. Statin action favors normalization of the plasma lipidome in theatherogenic mixed dyslipidemia of MetS: Potential relevance tostatin-associated dysglycemia. J Lipid Res. (2015) ;56: (12):2381–92. |
[5] | Wentworth JM , Naselli G , Ngui K , Smyth GK , Liu R , O’Brien PE , et al. GM3 ganglioside and phosphatidylethanolamine-containinglipids are adipose tissue markers of insulin resistance in obesewomen. Int J Obes (Lond). (2016) ;40: (4):706–13. |
[6] | Holland WL , Bikman BT , Wang LP , Yuguang G , Sargent KM , Bulchand S , et al. Lipid-induced insulin resistance mediated by theproinflammatory receptor TLR4 requires saturated fattyacid-induced ceramide biosynthesis in mice. J Clin Invest. (2011) ;121: (5):1858–70. |
[7] | Holland WL , Brozinick JT , Wang LP , Hawkins ED , Sargent KM , Liu Y , et al. Inhibition of ceramide synthesis amelioratesglucocorticoid-, saturated-fat-, and obesity-induced insulinresistance. Cell Metab. (2007) ;5: (3):167–79. |
[8] | Guenther GG , Edinger AL . A new take on ceramide: Starving cells by cutting off the nutrient supply. Cell Cycle. (2009) ;8: (8):1122–6. |
[9] | Holland WL , Miller RA , Wang ZV , Sun K , Barth BM , Bui HH , et al. Receptor-mediated activation of ceramidase activity initiates thepleiotropic actions of adiponectin. Nat Med. (2011) ;17: (1):55–63. |
[10] | Vasiliauskaite-Brooks I , Sounier R , Rochaix P , Bellot G , Fortier M , Hoh F , et al. Structural insights into adiponectin receptorssuggest ceramidase activity. Nature. (2017) ;544(7648):120–3. |
[11] | Vesper H , Schmelz EM , Nikolova-Karakashian MN , Dillehay DL , Lynch DV , Merrill AH Jr . Sphingolipids in food and the emerging importance of sphingolipids to nutrition. J Nutr (1999) ;129: (7):1239–50. |
[12] | Merrill AH Jr . De novo sphingolipid biosynthesis: A necessary, but dangerous, pathway. J Biol Chem (2002) ;277: (29):25843–6. |
[13] | Park JW , Park WJ , Futerman AH . Ceramide synthases as potential targets for therapeutic intervention in human diseases. Biochim Biophys Acta. (2014) ;1841: (5):671–81. |
[14] | Turpin SM , Nicholls HT , Willmes DM , Mourier A , Brodesser S , Wunderlich CM , et al. Obesity-induced CerS6-dependent C0ceramide production promotes weight gain and glucose intolerance. Cell Metab. (2014) ;20: (4):678–86. |
[15] | Raichur S , Wang ST , Chan PW , Li Y , Ching J , Chaurasia B , et al. CerS2 haploinsufficiency inhibits beta-oxidation and conferssusceptibility to diet-induced steatohepatitis and insulinresistance. Cell Metab. (2014) ;20: (4):687–95. |
[16] | Bikman BT , Summers SA . Ceramides as modulators of cellular and whole-body metabolism. The Journal of clinical investigation. (2011) ;121: (11):4222–30. |
[17] | Hotamisligil GS . Inflammation and metabolic disorders. Nature. (2006) ;444: (7121):860–7. |
[18] | Hotamisligil GS , Murray DL , Choy LN , Spiegelman BM . Tumor necrosis factor alpha inhibits signaling from the insulin receptor. Proceedings of the National Academy of Sciences of the United States of America. (1994) ;91: (11):4854–8. |
[19] | de Mello VD , Lankinen M , Schwab U , Kolehmainen M , Lehto S , Seppanen-Laakso T , et al. Link between plasma ceramides,inflammation and insulin resistance: Association with serum IL-6concentration in patients with coronary heart disease. Diabetologia. (2009) ;52: (12):2612–5. |
[20] | Majumdar I , Mastrandrea LD . Serum sphingolipids and inflammatory mediators in adolescents at risk for metabolic syndrome. Endocrine. (2012) ;41: (3):442–9. |
[21] | Sims K , Haynes CA , Kelly S , Allegood JC , Wang E , Momin A , et al. Kdo2-lipid A, a TLR4-specific agonist, induces de novosphingolipid biosynthesis in RAW264.7 macrophages, which isessential for induction of autophagy. The Journal of biologicalchemistry. (2010) ;285: (49):38568–79. |
[22] | Schilling JD , Machkovech HM , He L , Sidhu R , Fujiwara H , Weber K , et al. Palmitate and lipopolysaccharide trigger synergisticceramide production in primary macrophages. The Journal ofbiological chemistry. (2013) ;288: (5):2923–32. |
[23] | Vandanmagsar B , Youm YH , Ravussin A , Galgani JE , Stadler K , Mynatt RL , et al. The NLRP3 inflammasome instigates obesity-inducedinflammation and insulin resistance. Nature medicine. (2011) ;17: (2):179–88. |
[24] | Chaurasia B , Summers SA . Ceramides - Lipotoxic Inducers of Metabolic Disorders. Trends Endocrinol Metab. (2015) ;26: (10):538–50. |
[25] | Yamauchi T , Kamon J , Minokoshi Y , Ito Y , Waki H , Uchida S , et al. Adiponectin stimulates glucose utilization and fatty-acidoxidation by activating AMP-activated protein kinase. Naturemedicine. (2002) ;8: (11):1288–95. |
[26] | Ouchi N , Kihara S , Arita Y , Okamoto Y , Maeda K , Kuriyama H , et al. Adiponectin, an adipocyte-derived plasma protein, inhibitsendothelial NF-kappaB signaling through a cAMP-dependent pathway. Circulation. (2000) ;102: (11):1296–301. |
[27] | Kadowaki T , Yamauchi T , Kubota N . The physiological and pathophysiological role of adiponectin and adiponectin receptors in the peripheral tissues and CNS. FEBS letters. (2008) ;582: (1):74–80. |
[28] | Tanabe H , Fujii Y , Okada-Iwabu M , Iwabu M , Nakamura Y , Hosaka T , et al. Crystal structures of the human adiponectin receptors. Nature. (2015) ;520: (7547):312–6. |
[29] | Kharitonenkov A , Larsen P . FGF21 reloaded: Challenges of a rapidly growing field. Trends in endocrinology and metabolism: TEM. (2011) ;22: (3):81–6. |
[30] | Kharitonenkov A , Shiyanova TL , Koester A , Ford AM , Micanovic R , Galbreath EJ , et al. FGF-21 as a novel metabolic regulator. TheJournal of Clinical Investigation. (2005) ;115: (6):1627–35. |
[31] | Holland WL , Adams AC , Brozinick JT , Bui HH , Miyauchi Y , Kusminski CM , et al. An FGF21-adiponectin-ceramide axis controls energyexpenditure and insulin action in mice. Cell Metabolism. (2013) ;17: (5):790–7. |
[32] | Backhed F , Ding H , Wang T , Hooper LV , Koh GY , Nagy A , et al. Thegut microbiota as an environmental factor that regulates fatstorage. Proceedings of the National Academy of Sciences of theUnited States of America. (2004) ;101: (44):15718–23. |
[33] | Jiang C , Xie C , Li F , Zhang L , Nichols RG , Krausz KW , et al. Intestinal farnesoid X receptor signaling promotes nonalcoholicfatty liver disease. The Journal of Clinical Investigation.(2014) . |
[34] | Xie C , Jiang C , Shi J , Gao X , Sun D , Sun L , et al. An IntestinalFarnesoid X Receptor-Ceramide Signaling Axis Modulates HepaticGluconeogenesis in Mice. Diabetes. (2017) ;66: (3):613–26. |
[35] | Chavez JA , Summers SA . A ceramide-centric view of insulin resistance. Cell metabolism. (2012) ;15: (5):585–94. |
[36] | Wang CN , O’Brien L , Brindley DN . Effects of cell-permeable ceramides and tumor necrosis factor-alpha on insulin signaling and glucose uptake in 3T3-L1 adipocytes. Diabetes. (1998) ;47: (1):24–31. |
[37] | Summers SA , Garza LA , Zhou H , Birnbaum MJ . Regulation of insulin-stimulated glucose transporter GLUT4 translocation and Akt kinase activity by ceramide. Mol Cell Biol. (1998) ;18: (9):5457–64. |
[38] | Galadari S , Rahman A , Pallichankandy S , Galadari A , Thayyullathil F . Role of ceramide in diabetes mellitus: Evidence and mechanisms. Lipids in Health and Disease. (2013) ;12: :98. |
[39] | Brozinick JT , Hawkins E , Hoang Bui H , Kuo MS , Tan B , Kievit P , et al. Plasma sphingolipids are biomarkers of metabolic syndromein non-human primates maintained on a Western-style diet. International Journal of Obesity. (2013) ;37: (8):1064–70. |
[40] | Haus JM , Kashyap SR , Kasumov T , Zhang R , Kelly KR , Defronzo RA , et al. Plasma ceramides are elevated in obese subjects with type 2diabetes and correlate with the severity of insulin resistance. Diabetes. (2009) ;58: (2):337–43. |
[41] | Samad F , Hester KD , Yang G , Hannun YA , Bielawski J . Altered adipose and plasma sphingolipid metabolism in obesity: A potential mechanism for cardiovascular and metabolic risk. Diabetes. (2006) ;55: (9):2579–87. |
[42] | Chavez JA , Knotts TA , Wang LP , Li G , Dobrowsky RT , Florant GL , et al. A role for ceramide, but not diacylglycerol, in theantagonism of insulin signal transduction by saturated fattyacids. J Biol Chem. (2003) ;278: (12):10297–303. |
[43] | Salinas M , Lopez-Valdaliso R , Martin D , Alvarez A , Cuadrado A . Inhibition of PKB/Akt1 by C2-ceramide involves activation of ceramide-activated protein phosphatase in PC12 cells. Molecular and Cellular Neurosciences. (2000) ;15: (2):156–69. |
[44] | Stratford S , DeWald DB , Summers SA . Ceramide dissociates 3’-phosphoinositide production from pleckstrin homology domain translocation. The Biochemical Journal. (2001) ;354: (Pt 2):359–68. |
[45] | Powell DJ , Turban S , Gray A , Hajduch E , Hundal HS . Intracellular ceramide synthesis and protein kinase Czeta activation play an essential role in palmitate-induced insulin resistance in rat L6 skeletal muscle cells. The Biochemical Journal. (2004) ;382: (Pt 2):619–29. |
[46] | Watson ML , Coghlan M , Hundal HS . Modulating serine palmitoyl transferase (SPT) expression and activity unveils a crucial role in lipid-induced insulin resistance in rat skeletal muscle cells. The Biochemical Journal. (2009) ;417: (3):791–801. |
[47] | Hu W , Ross J , Geng T , Brice SE , Cowart LA . Differential regulation of dihydroceramide desaturase by palmitate versus monounsaturated fatty acids: Implications for insulin resistance. The Journal of Biological Chemistry. (2011) ;286: (19):16596–605. |
[48] | Ussher JR , Koves TR , Cadete VJ , Zhang L , Jaswal JS , Swyrd SJ , et al. Inhibition of de novo ceramide synthesis reversesdiet-induced insulin resistance and enhances whole-body oxygenconsumption. Diabetes. (2010) ;59: (10):2453–64. |
[49] | Yang G , Badeanlou L , Bielawski J , Roberts AJ , Hannun YA , Samad F . Central role of ceramide biosynthesis in body weight regulation, energy metabolism, and the metabolic syndrome. Am J Physiol Endocrinol Metab. (2009) ;297: (1):E211–24. |
[50] | Bikman BT , Guan Y , Shui G , Siddique MM , Holland WL , Kim JY , et al. Fenretinide prevents lipid-induced insulin resistance by blockingceramide biosynthesis. J Biol Chem. (2012) ;287: (21):17426–37. |
[51] | Li Z , Zhang H , Liu J , Liang CP , Li Y , Li Y , et al. Reducing plasmamembrane sphingomyelin increases insulin sensitivity. Mol CellBiol. (2011) ;31: (20):4205–18. |
[52] | Xia JY , Holland WL , Kusminski CM , Sun K , Sharma AX , Pearson MJ , et al. Targeted Induction of Ceramide Degradation Leads toImproved Systemic Metabolism and Reduced Hepatic Steatosis. CellMetab. (2015) ;22: (2):266–78. |
[53] | Eleanor Wheeler AL , Liu C-T , Hivert M-F , Strawbridge RJ , Podmore C , Li M , Yao J , Sim X , Hong J , Chu AY , Zhang W , Wang X , Chen P , Maruthur NM , Porneala BC , Sharp SJ , Jia Y , Kabagambe EK , Chang L-C , Chen W-M , Elks CE , Evans DS , Fan Q , Giulianini F , Go MJ , Hottenga J-J , Hu Y , Jackson AU , Kanoni S , Kim YJ , Kleber ME , Ladenvall C , Lecoeur C , Lim S-H , Lu Y , Mahajan A , Marzi C , Nalls MA , Navarro P , Nolte IM , Rose LM , Rybin DV , Sanna S , Shi Y , Stram DO , Takeuchi F , Tan SP , van der Most PJ , Van Vliet-Ostaptchouk JV , Wong A , Yengo L , Zhao W , Goel A , Larrad MTM , Radke D , Salo P , Tanaka T , van Iperen EPA , Abecasis G , Afaq S , Alizadeh BZ , Bertoni AG , Bonnefond A , Böttcher Y , Bottinger EP , Campbell H , Carlson OD , Chen C-H , Cho YS , Timothy Garvey W , Gieger C , Goodarzi MO , Grallert H , Hamsten A , Hartman CA , Herder 7C , Hsiung CA , Huang J , Igase M , Isono M , Katsuya T , Khor C-C , Kiess W , Kohara K , Kovacs P , Lee J , Lee W-J , Lehne B , Li H , Liu J , Lobbens S , Luan J , Lyssenko V , Meitinger T , Miki T , Miljkovic I , Moon S , Mulas A , Müller G , Müller-Nurasyid M , Nagaraja R , Nauck M , Pankow JS , Polasek O , Prokopenko I , Ramos PS , Rasmussen-Torvik L , Rathmann W , Rich SS , Robertson NR , Roden M , Roussel R , Rudan I , Scott RA , Scott WR , Sennblad B , Siscovick DS , Strauch K , Sun L , Swertz M , Tajuddin SM , Taylor KD , Teo Y-Y , Tham YC , Tönjes A , Wareham NJ , Willemsen G , Wilsgaard T , Hingorani AD , EPIC-CVDConsortium, EPIC-InterAct Consortium, Lifelines Cohort Study, Egan J , Ferrucci L , Kees Hovingh G , Jula A , Kivimaki M , Kumari M , Njølstad I , Palmer CNA , Ríos MS , Stumvoll M , Watkins H , Aung T , Blüher M , Boehnke M , Boomsma DI , Bornstein SR , Chambers JC , Chasman DI , Ida Chen Y-D , Chen Y-T , Cheng C-Y , Cucca F , de Geus EJC , Deloukas P , Evans MK , Fornage M , Friedlander Y , Froguel P , Groop L , Gross MD , Harris TB , Hayward C , Heng C-K , Ingelsson E , Kato N , Kim B-J , Koh W-P , Kooner JS , Körner A , Kuh D , Kuusisto J , Laakso M , Lin X , Liu Y , Loos RJF , Magnusson PKE , März W , McCarthy MI , Oldehinkel AJ , Ong KK , Pedersen NL , Pereira MA , Peters A , Ridker PM , Sabanayagam C , Sale M , Saleheen D , Saltevo J , Schwarz PEH , Sheu WHH , Snieder H , Spector TD , Tabara Y , Tuomilehto J , van Dam RM , Wilson JG , Wilson JF , Wolffenbuttel BHR , Wong TY , Wu J-Y , Yuan J-M , Zonderman AB , Soranzo N , Guo X , Roberts DJ , Florez JC , Sladek R , Dupuis J , Morris AP , Tai E-S , Selvin E , Rotter JI , Langenberg C , Barroso I , Meigs JB . Impact of common genetic determinants of hemoglobin A1c on Type 2 diabetesrisk and diagnosis in ancestrally diverse populations: Atransethnic genome-wide meta-analysis. PLOS Medicine. (2017) . |
[54] | Correnti JM , Juskeviciute E , Swarup A , Hoek JB . Pharmacological ceramide reduction alleviates alcohol-induced steatosis and hepatomegaly in adiponectin knockout mice. Am J Physiol Gastrointest Liver Physiol. (2014) ;306: (11):G959–73. |
[55] | Kurek K , Piotrowska DM , Wiesiolek-Kurek P , Lukaszuk B , Chabowski A , Gorski J , et al. Inhibition of ceramide de novo synthesisreduces liver lipid accumulation in rats with nonalcoholic fattyliver disease. Liver Int. (2014) ;34: (7):1074–83. |
[56] | Kurek K , Wiesiolek-Kurek P , Piotrowska DM , Lukaszuk B , Chabowski A , Zendzianendzian-Piotrowska M . Inhibition of ceramide de novo synthesis with myriocin affects lipid metabolism in the liver of rats with streptozotocin-induced type 1 diabetes. Biomed Res Int. (2014) ;2014: :980815. |
[57] | Dekker MJ , Baker C , Naples M , Samsoondar J , Zhang R , Qiu W , et al. Inhibition of sphingolipid synthesis improves dyslipidemia in thediet-induced hamster model of insulin resistance: Evidence for therole of sphingosine and sphinganine in hepatic VLDL-apoB100overproduction. Atherosclerosis. (2013) ;228: (1):98–109. |
[58] | Chaurasia B , Kaddai VA , Lancaster GI , Henstridge DC , Sriram S , Galam DL , et al. Adipocyte ceramides regulate subcutaneous adiposebrowning, inflammation, and metabolism. Cell Metab. (2016) ;24: (6):820–34. |
[59] | Jiang C , Xie C , Lv Y , Li J , Krausz KW , Shi J , et al. Intestine-selective farnesoid X receptor inhibition improvesobesity-related metabolic dysfunction. Nat Commun. (2015) ;6: :10166. |
[60] | Alexaki A , Clarke BA , Gavrilova O , Ma Y , Zhu H , Ma X , et al. Denovo sphingolipid biosynthesis is required for adipocyte survivaland metabolic homeostasis. J Biol Chem. (2017) ;292: (9):3929–39. |
[61] | Lee SY , Lee HY , Song JH , Kim GT , Jeon S , Song YJ , et al. Adipocyte-specific deficiency of de novo sphingolipid biosynthesisleads to lipodystrophy and insulin resistance. Diabetes. (2017) . |
[62] | Eguchi J , Wang X , Yu S , Kershaw EE , Chiu PC , Dushay J , et al. Transcriptional control of adipose lipid handling by IRF4. Cell Metab. (2011) ;13: (3):249–59. |
[63] | Neuschwander-Tetri BA , Caldwell SH . Nonalcoholic steatohepatitis: Summary of an AASLD single topic conference. Hepatology. (2003) ;37: (5):1202–19. |
[64] | Kim CH , Younossi ZM . Nonalcoholic fatty liver disease: A manifestation of the metabolic syndrome. Cleveland Clinic Journal of Medicine. (2008) ;75: (10):721–8. |
[65] | Yetukuri L , Katajamaa M , Medina-Gomez G , Seppanen-Laakso T , Vidal-Puig A , Oresic M . Bioinformatics strategies for lipidomics analysis: Characterization of obesity related hepatic steatosis. BMC Systems Biology. (2007) ;1: :12. |
[66] | Luukkonen PK , Zhou Y , Sadevirta S , Leivonen M , Arola J , Oresic M , et al. Hepatic ceramides dissociate steatosis and insulinresistance in patients with non-alcoholic fatty liver disease. J Hepatol. (2016) ;64: (5):1167–75. |
[67] | Gosejacob D , Jager PS , Vom Dorp K , Frejno M , Carstensen AC , Kohnke M , et al. Ceramide Synthase 5 Is Essential to MaintainC0-Ceramide Pools and Contributes to the Development ofDiet-induced Obesity. J Biol Chem. (2016) ;291: (13):6989–7003. |
[68] | Holland WL , Xia JY , Johnson JA , Sun K , Pearson MJ , Sharma AX , et al. Inducible overexpression of adiponectin receptors highlightthe roles of adiponectin-induced ceramidase signaling in lipid andglucose homeostasis. Mol Metab. (2017) ;6: (3):267–75. |
[69] | Amati F , Dube JJ , Alvarez-Carnero E , Edreira MM , Chomentowski P , Coen PM , et al. Skeletal muscle triglycerides, diacylglycerols,and ceramides in insulin resistance: Another paradox inendurance-trained athletes? Diabetes (2011) ;60: (10):2588–97. |
[70] | Coen PM , Hames KC , Leachman EM , DeLany JP , Ritov VB , Menshikova EV , et al. Reduced skeletal muscle oxidative capacity and elevatedceramide but not diacylglycerol content in severe obesity. Obesity(Silver Spring). (2013) ;21: (11):2362–71. |
[71] | de la Maza MP , Rodriguez JM , Hirsch S , Leiva L , Barrera G , Bunout D . Skeletal muscle ceramide species in men with abdominal obesity. J Nutr Health Aging. (2015) ;19: (4):389–96. |
[72] | Coen PM , Dube JJ , Amati F , Stefanovic-Racic M , Ferrell RE , Toledo FG , et al. Insulin resistance is associated with higherintramyocellular triglycerides in type I but not type II myocytesconcomitant with higher ceramide content. Diabetes. (2010) ;59: (1):80–8. |
[73] | Adams JM 2nd , Pratipanawatr T , Berria R , Wang E , DeFronzo RA , Sullards MC , et al. Ceramide content is increased in skeletalmuscle from obese insulin-resistant humans. Diabetes. (2004) ;53: (1):25–31. |
[74] | Dube JJ , Amati F , Toledo FG , Stefanovic-Racic M , Rossi A , Coen P , et al. Effects of weight loss and exercise on insulin resistance,and intramyocellular triacylglycerol, diacylglycerol and ceramide. Diabetologia. (2011) ;54: (5):1147–56. |
[75] | Dube JJ , Amati F , Stefanovic-Racic M , Toledo FG , Sauers SE , Goodpaster BH . Exercise-induced alterations in intramyocellularlipids and insulin resistance: The athlete’s paradoxrevisited. Am J Physiol Endocrinol Metab. (2008) ;294: (5):E882–8. |
[76] | Coen PM , Menshikova EV , Distefano G , Zheng D , Tanner CJ , Standley RA , et al. Exercise and weight loss improve muscle mitochondrialrespiration, lipid partitioning and insulin sensitivity followinggastric bypass surgery. Diabetes. (2015) . |
[77] | Itani SI , Rudderman NB , Schmieder F , Boden G . Lipid-induced insulin resistance in human muscle is associated with changes in diacylglycerol, protein kinase C and IkB-a. Diabetes. (2002) ;51: (7):2005–11. |
[78] | Szendroedi J , Yoshimura T , Phielix E , Koliaki C , Marcucci M , Zhang D , et al. Role of diacylglycerol activation of PKCtheta inlipid-induced muscle insulin resistance in humans. Proc Natl AcadSci USA. (2014) ;111: (26):9597–602. |
[79] | Nowotny B , Zahiragic L , Krog D , Nowotny PJ , Herder C , Carstensen M , et al. Mechanisms underlying the onset of oral lipid-inducedskeletal muscle insulin resistance in humans. Diabetes. (2013) ;62: (7):2240–8. |
[80] | Skovbro M , Baranowski M , Skov-Jensen C , Flint A , Dela F , Gorski J , et al. Human skeletal muscle ceramide content is not a majorfactor in muscle insulin sensitivity. Diabetologia. (2008) ;51: (7):1253–60. |
[81] | Summers SA , Goodpaster BH . CrossTalk proposal: Intramyocellular ceramide accumulation does modulate insulin resistance. J Physiol. (2016) ;594: (12):3167–70. |
[82] | Petersen MC , Jurczak MJ . CrossTalk opposing view: Intramyocellular ceramide accumulation does not modulate insulin resistance. J Physiol. (2016) ;594: (12):3171–4. |
[83] | Chavez JA , Summers SA . Characterizing the effects of saturated fatty acids on insulin signaling and ceramide and diacylglycerol accumulation in 3T3-L1 adipocytes and C2C12 myotubes. Arch Biochem Biophys. (2003) ;419: (2):101–9. |
[84] | Park M , Kaddai V , Ching J , Fridianto KT , Sieli RJ , Sugii S , et al. A role for ceramides, but not sphingomyelins, as antagonists ofinsulin signaling and mitochondrial metabolism in C2C12 myotubes. J Biol Chem. (2016) ;291: (46):23978–88. |
[85] | Chavez JA , Holland WL , Bar J , Sandhoff K , Summers SA . Acid ceramidase overexpression prevents the inhibitory effects of saturated fatty acids on insulin signaling. J Biol Chem. (2005) ;280: (20):20148–53. |
[86] | El-Assaad W , Buteau J , Peyot ML , Nolan C , Roduit R , Hardy S , et al. Saturated fatty acids synergize with elevated glucose tocause pancreatic beta-cell death. Endocrinology. (2003) ;144: (9):4154–63. |
[87] | Kelpe CL , Moore PC , Parazzoli SD , Wicksteed B , Rhodes CJ , Poitout V . Palmitate inhibition of insulin gene expression is mediated at the transcriptional level via ceramide synthesis. J Biol Chem. (2003) ;278: (32):30015–21. |
[88] | Maedler K , Oberholzer J , Bucher P , Spinas GA , Donath MY . Monounsaturated fatty acids prevent the deleterious effects of palmitate and high glucose on human pancreatic beta-cell turnover and function. Diabetes. (2003) ;52: (3):726–33. |
[89] | Ishizuka N , Yagui K , Tokuyama Y , Yamada K , Suzuki Y , Miyazaki J , et al. Tumor necrosis factor alpha signaling pathway and apoptosisin pancreatic beta cells. Metabolism. (1999) ;48: (12):1485–92. |
[90] | Shimabukuro M , Higa M , Zhou YT , Wang MY , Newgard CB , Unger RH . Lipoapoptosis in beta-cells of obese prediabetic fa/fa rats. Roleof serine palmitoyltransferase overexpression. J Biol Chem. (1998) ;273: (49):32487–90. |
[91] | Sjoholm A . Ceramide inhibits pancreatic beta-cell insulin production and mitogenesis and mimics the actions of interleukin-1 beta. FEBS Lett. (1995) ;367: (3):283–6. |
[92] | Lang F , Ullrich S , Gulbins E . Ceramide formation as a target in beta-cell survival and function. Expert Opin Ther Targets. (2011) ;15: (9):1061–71. |
[93] | Zhu Q , Shan X , Miao H , Lu Y , Xu J , You N , et al. Acute activationof acid ceramidase affects cytokine-induced cytotoxicity in ratislet beta-cells. FEBS Lett. (2009) ;583: (12):2136–41. |
[94] | Bellini L , Campana M , Mahfouz R , Carlier A , Veret J , Magnan C , et al. Targeting sphingolipid metabolism in the treatment ofobesity/type 2 diabetes. Expert Opin Ther Targets. (2015) ;19: (8):1037–50. |
[95] | Veret J , Bellini L , Giussani P , Ng C , Magnan C , Le Stunff H . Roles of sphingolipid metabolism in pancreatic beta cell dysfunction induced by lipotoxicity. J Clin Med. (2014) ;3: (2):646–62. |
[96] | Boslem E , Meikle PJ , Biden TJ . Roles of ceramide and sphingolipids in pancreatic beta-cell function and dysfunction. Islets. (2012) ;4: (3):177–87. |
[97] | Lucchetta M , Rudilosso S , Costa S , Bruttomesso D , Ruggero S , Toffanin E , et al. Anti-ganglioside autoantibodies in type 1diabetes. Muscle Nerve. (2010) ;41: (1):50–3. |
[98] | Dotta F , Falorni A , Tiberti C , Dionisi S , Anastasi E , Torresi P , et al. Autoantibodies to the GM2-1 islet ganglioside and to GAD-65at type 1 diabetes onset. J Autoimmun. (1997) ;10: (6):585–8. |
[99] | Misasi R , Dionisi S , Farilla L , Carabba B , Lenti L , Di Mario U , et al. Gangliosides and autoimmune diabetes. Diabetes Metab Rev. (1997) ;13: (3):163–79. |
[100] | Dionisi S , Dotta F , Diaz-Horta O , Carabba B , Viglietta V , Di Mario U . Target antigens in autoimmune diabetes: Pancreatic gangliosides. Ann Ist Super Sanita. (1997) ;33: (3):433–5. |
[101] | Gelling RW , Morton GJ , Morrison CD , Niswender KD , Myers MG Jr , Rhodes CJ , et al. Insulin action in the brain contributes toglucose lowering during insulin treatment of diabetes. Cell Metabolism. (2006) ;3: (1):67–73. |
[102] | Vogt MC , Bruning JC . CNS insulin signaling in the control of energy homeostasis and glucose metabolism - from embryo to old age. Trends in Endocrinology and Metabolism: TEM. (2013) ;24: (2):76–84. |
[103] | Benoit SC , Kemp CJ , Elias CF , Abplanalp W , Herman JP , Migrenne S , et al. Palmitic acid mediates hypothalamic insulin resistance byaltering PKC-theta subcellular localization in rodents. TheJournal of Clinical Investigation. (2009) ;119: (9):2577–89. |
[104] | Contreras C , Gonzalez-Garcia I , Martinez-Sanchez N , Seoane-Collazo P , Jacas J , Morgan DA , et al. Central ceramide-inducedhypothalamic lipotoxicity and ER stress regulate energy balance. Cell Re. (2014) ;9: (1):366–77. |
[105] | Nordstrom V , Willershauser M , Herzer S , Rozman J , von Bohlen UndHalbach O , Meldner S , et al. Neuronalexpression of glucosylceramide synthase in central nervous systemregulates body weight and energy homeostasis. PLoS Biol. (2013) ;11: (3):e1001506. |
[106] | Summers SA , Nelson DH . A role for sphingolipids in producing the common features of type 2 diabetes, metabolic syndrome X, and Cushing’s syndrome. Diabetes. (2005) ;54: (3):591–602. |
[107] | Chun L , Junlin Z , Aimin W , Niansheng L , Benmei C , Minxiang L . Inhibition of ceramide synthesis reverses endothelial dysfunction and atherosclerosis in streptozotocin-induced diabetic rats. Diabetes Res Clin Pract. (2011) ;93: (1):77–85. |
[108] | Hojjati MR , Li Z , Zhou H , Tang S , Huan C , Ooi E , et al. Effect ofmyriocin on plasma sphingolipid metabolism and atherosclerosis inapoE-deficient mice. J Biol Chem. (2005) ;280: (11):10284–9. |
[109] | Park TS , Panek RL , Mueller SB , Hanselman JC , Rosebury WS , Robertson AW , et al. Inhibition of sphingomyelin synthesis reducesatherogenesis in apolipoprotein E-knockout mice. Circulation. (2004) ;110: (22):3465–71. |
[110] | Park TS , Rosebury W , Kindt EK , Kowala MC , Panek RL . Serine palmitoyltransferase inhibitor myriocin induces the regression of atherosclerotic plaques in hyperlipidemic ApoE-deficient mice. Pharmacol Res. (2008) ;58: (1):45–51. |
[111] | Laaksonen R , Ekroos K , Sysi-Aho M , Hilvo M , Vihervaara T , Kauhanen D , et al. Plasma ceramides predict cardiovascular death inpatients with stable coronary artery disease and acute coronarysyndromes beyond LDL-cholesterol. Eur Heart J. (2016) ;37: (25):1967–76. |
[112] | Li W , Yang X , Xing S , Bian F , Yao W , Bai X , et al. Endogenousceramide contributes to the transcytosis of oxLDL acrossendothelial cells and promotes its subendothelial retention invascular wall. Oxidative Medicine and Cellular Longevity. (2014) ;2014: :823071. |
[113] | Gao D , Pararasa C , Dunston CR , Bailey CJ , Griffiths HR . Palmitate promotes monocyte atherogenicity via de novo ceramide synthesis. Free Radical Biology & Medicine. (2012) ;53: (4):796–806. |
[114] | Zhang QJ , Holland WL , Wilson L , Tanner JM , Kearns D , Cahoon JM , et al. Ceramide mediates vascular dysfunction in diet-inducedobesity by PP2A-mediated dephosphorylation of the eNOS-Aktcomplex. Diabetes. (2012) ;61: (7):1848–59. |
[115] | Park TS , Hu Y , Noh HL , Drosatos K , Okajima K , Buchanan J , et al. Ceramide is a cardiotoxin in lipotoxic cardiomyopathy. J Lipid Res. (2008) ;49: (10):2101–12. |
[116] | Lee SY , Kim JR , Hu Y , Khan R , Kim SJ , Bharadwaj KG , et al. Cardiomyocyte specific deficiency of serine palmitoyltransferase subunit 2 reduces ceramide but leads to cardiac dysfunction. J Biol Chem. (2012) ;287: (22):18429–39. |
[117] | Summers SA . Sphingolipids and insulin resistance: The five Ws. Curr Opin Lipidol. (2010) ;21: (2):128–35. |