Vitamin A and cognitive processes
Abstract
Vitamin A and its derivatives, the retinoids, essential for human health, modulate several physiological processes through their interactions with the nuclear retinoic acid receptors (RARs) and retinoid X receptors (RXRs). They are involved in the modulation of cerebral plasticity including hippocampal neurogenesis and synaptic plasticity, known to underlie cognitive processes. Interestingly, retinoic acid (RA) hyposignaling, which contributes to the deterioration of hippocampal plasticity and function during aging, can be reversed by vitamin A supplementation or RA treatment. However, it is still not clear how vitamin A status modulates plasticity and memory. It is commonly accepted that RA, by binding to its specific nuclear receptors, regulates gene expression, including the expression of plasticity-related genes. Moreover, recent studies suggest that vitamin A could participate in the maintenance of neurobiological functions indirectly, through the glucocorticoid pathway. Here, we present data supporting the importance of vitamin A status in the maintenance of memory processes, and the contribution of naturally occurring RA hyposignaling during aging to the etiology of cognitive decline. We propose that vitamin A supplementation or RA treatment could be a potent way to prevent age-related cognitive impairments by maintaining normal vitamin A and glucocorticoid (GC) status in seniors.
1Introduction
Vitamin A (retinol) is an essential liposoluble nutrient for humans. It is present in foods of animal origin such as liver, eggs and dairy products. Provitamin A – carotenoids from plants that are partly converted to retinol by vertebrates – are an alternative source of vitamin A. However, they are an order of magnitude less efficient as a source of retinol. Retinoids, a family of compounds derived from vitamin A, have numerous important functions in many tissues, including vision, the maintenance of epithelial surfaces, immune competence, reproduction, and embryonic growth and development [1]. The majority of these functions (mechanisms underlying the extravisual functions) are performed by the vitamin A metabolite retinoic acid (RA), which binds to receptors of the nuclear receptor superfamily and regulates gene expression.
The importance of vitamin A and RA during the embryonic development of the brain has been extensively studied and their functions, key during this period of life, are well specified. However, it is now recognized that the role of RA in the brain persists into adulthood. Indeed, many studies over the past decade have contributed to the demonstration that RA is involved in the maintenance of neurobiological processes, particularly neuroplasticity, that underlie memory performances throughout life and that the hypoactivity of retinoid signaling participates in age-related cognitive decline. The mechanisms by which retinoids exert their effects on memory are not all well understood. However, it seems clear that in addition to a direct role in neuronal plasticity via its nuclear receptors, RA is also capable of antagonizing the effects of glucocorticoids and thereby exerting a beneficial effect on the maintenance of memory processes.
2Vitamin A metabolism (Fig. 1)
2.1Transport
The capacity to synthesize de novo compounds with vitamin A (retinol) activity is limited to plants and microorganisms. Higher animals must therefore obtain vitamin A from their diet, either in the form of a preformed vitamin, all-trans retinol (the major dietary forms are long-chain fatty acid esters of retinol: retinyl esters or REs) or as provitamin A - carotenoids, such as β-carotene, α-carotene or β-cryptoxanthin, found in plant-derived foods. Although abundant in food, their conversion is not very efficient: 12 μg of β-carotene are converted to only 1 μg of vitamin A [2].
Presently, it is recommended that 60% of vitamin A intake be in the form of carotenoids (plant sources) and 40% in the form of retinols (animal sources). REs must be hydrolyzed prior to intestinal absorption. The efficiency of absorption is higher for retinol (80–90% ) than for carotenoids (50–60% ). Some carotenoids are converted to retinol in the intestinal mucosa via two enzymatic steps. Free retinol is reesterified with long-chain fatty acids in mucosal cells by the enzyme lecithin retinol acyltransferase (LRAT). The resulting REs are incorporated into chylomicrons, absorbed via the lymphatics and delivered to hepatocytes that take up about 70% of dietary retinol [3]. Under normal nutritional conditions, most of the vitamin A is stored in the liver (essentially as REs), partly in the hepatocytes and in higher amounts as lipid droplets in the stellate cells (also called Ito cells), from which vitamin A is mobilized when needed [4]. REs are hydrolyzed prior to mobilization from the liver, and free retinol is bound to retinol-binding protein 4 (RBP4). Approximately 95% of the RBPs circulate in the plasma as macromolecular complexes with the thyroxine carrier, transthyretin (TTR).
2.2Cellular action of retinoids
In tissues, retinol is taken up by target cells through an RBP-binding transporter, STRA6 [5]. Retinol enters the cytoplasm, where it binds to cellular retinol binding protein 1 (CRBP1) and, in a two-step process, is metabolized to all-trans retinoic acid, the active metabolite of vitamin A [6]. Importantly, RA levels in cells and tissues are also controlled by its degradation by hydroxylation, mainly by CYP26A1, an RA-induced p450 enzyme [7].
The physiological action of all-trans RA is mainly mediated by its binding to specific nuclear receptors that function as transcription factors: the retinoic acid receptors (RARs), whose ligands are the all-trans RA and 9-cis RA isomers, and the retinoid X receptors (RXRs), whose ligand is the 9-cis RA isomer [8].
The RA response element (RARE) binds dimers of the receptors. Most often, an RAR heterodimerizes with an RXR, which possesses the capacity to act independently of the presence of its ligand. There are three genes each for the RARs and RXRs (α, β and γ) that encode their α, β and γ isotypes respectively. In addition, for each RAR isotype, there are several isoforms generated by differential promoter use and splicing, greatly increasing the number of variants. Recent advances reveal the complexity of nuclear RA receptor involvement in cellular function through the regulation of gene expression and kinase activity [9]. In addition, some authors have demonstrated non-genomic modes of RA action. These rapid actions include the regulation of gap junctions [10], spinule formation in the retina [11] and effects on dendritic spines in the hippocampus [12]. Another pathway of action is through the repression of AP-1 (Jun, Fos) activity, which involves RAR/RXR dimers but not RARE [13]. In this way, vitamin A and, more precisely, RA, via its specific nuclear receptors, play a critical role in a variety of essential biological processes including reproduction, embryonic development and the modulation of the growth and differentiation of a wide variety of mammalian cell types.
3Vitamin A contributes to the maintenance of neurobiological processes that underlie memory performances throughout life
A large number of epidemiological studies suggest that diet is important for mental health, and inverse correlations between dietary quality and common mental disorders have been reported in adults [14, 15]. Vitamin A and RA, are essential for the developing central nervous system (CNS), where they control neurogenesis and neuronal patterning [16], but also play a key role in the maintenance of diverse functions in the adult brain by regulating neuroplasticity in cerebral structures such as the hippocampus [17–19]. Thus, it is of the first importance to study the effects of the status of vitamin A or RA in the adult brain, in particular during aging.
3.1The role of the RA nuclear receptors, RAR and RXR
There is evidence to suggest that changes in retinoid levels are capable of producing alterations in neuronal target proteins and consequently may affect physiological maintenance processes in the mature brain [20]. More precisely, it has been shown that RARβ and RARβ-RXRγ knockout mice display an alteration of long-term potentiation (LTP) as well as substantial performance deficits in a hippocampus-dependent spatial learning task [21]. RARβ mutations, with mutation in either RXRβ or RXRγ, also result in impaired locomotion typical of abnormal striatal function, possibly related to a decrease in D1 and D2 dopamine receptors in striatal neurons [22]. The ablation of RXRγ in mice leads to depression-like behaviors including increased despair and anhedonia, associated with the reduced expression of the D2 dopamine receptor in the shell of the nucleus accumbens and altered serotonin signaling [23].
Overactivation of the retinoid pathway also leads to the dysregulation of physiological processes in the brain. Thus, the perturbation of RA biosynthesis due to chronic ethanol consumption induces RARβ and RXRβ/γ overexpression in vivo, leading to cognitive deficits. Blocking RARβ activity in this situation has been shown to reverse the alcohol-induced memory deficits. Thus, the overexpression of RA nuclear receptors in the brain also appears to be involved in the memory impairments observed during chronic ethanol consumption [24]. RA of astroglial origin may also be an important signal for neurogenesis in the dentate gyrus of the hippocampus [25]. A recent study has shown that mice that express a dominant-negative form of RARα in the mature forebrain display impaired LTP and AMPA-mediated synaptic transmission in the hippocampus, as well as impaired hippocampus-dependent social recognition and spatial memory abilities [26].
Together, these results suggest that nutritional or physiological situations involving modifications in the level of expression of brain retinoid receptors may lead to considerable neurobiological alterations and mnesic deterioration.
3.2Vitamin A deficiency: A nutritional model that simulates age-related memory deficits
The potential role of retinoids in memory has been well argued in works studying the consequences to brain function of the decreased bioavailability of RA consecutive to a vitamin A deficiency (VAD), the historical nutritional model for the study of the effects of vitamin A. The targeted induction (through VAD) of hypoactivity of the retinoid signaling pathway to the same extent as that described in aged animals leads to cognitive deficits in young adult animals comparable to those linked with age [27, 28]. In other words, there is a relationship between the level of brain activity of the retinoid pathway, the expression of target genes such as neurogranin/RC3 and neuromodulin (GAP43) [29], and memory performance. Moreover, this model has helped reveal the strong link between RA hyposignaling and abnormal hippocampal plasticity: a decrease in hippocampal volume coincident with sick and dying neurons [30], the loss of hippocampal LTP and long-term depression (LTD) [31], and a blockade of hippocampal neurogenesis that can be restored by RA via the induction of RA-target genes, including the neurotrophin receptor tropomyosin receptor kinase A [28]. It has recently been shown that the upregulation of indicators of hippocampal cytoskeletal remodeling and protein damage may at least partly explain the neuronal loss and reduced hippocampal size that occur with VAD [32].
3.3Vitamin A prevents, limits or delays age-related cognitive decline
All neurobiological alterations induced by insufficient vitamin A bioavailability in VAD animals have been shown to be reversible by RA administration (i.e. drug strategy) or vitamin A supplementation (i.e. nutritional intervention).
Age-related alterations in vitamin A metabolism have been reported in both rats and humans, leading to an increased concentration of vitamin A stores in the liver [33, 34]. However, the vitamin A status of healthy aged people, as measured by circulating retinol, does not appear to be abnormal. Indeed, in the elderly, circulating retinol remains precisely regulated to a level close to 2 μmol/L, although it varies slightly depending on recent food intake [35]. No changes have been observed with age with respect to vitamin A absorption, the esterification of retinol or the secretion of REs into chylomicrons. The only apparent dysfunction that takes place with aging is a decrease in retinol mobilization from the liver to the circulation [36]. Interestingly, this report suggests that the relative dose-response test could be a better measure than the levels of serum retinol to evaluate the modifications of vitamin A status in elderly population. However, a possible alteration in the plasma transport of RE in chylomicrons associated with an unchanged or slightly decreased concentration of retinol or RBP has been reported during the postprandial period in middle-aged adults [37]. Together, these data suggest that, during aging, vitamin A is sequestered by the liver but that there are compensatory physiological mechanisms to facilitate its direct use by tissues, particularly the brain, during the postprandial period.
More recently, several signs of the hypoactivation of retinoid signaling pathways have been highlighted during aging. Indeed, several studies have shown that a moderate downregulation of retinoid-mediated transcriptional events occurs naturally with senescence. A decrease in the abundance of RARβ and RXRβ/γ mRNA has effectively been observed in the whole brain and hippocampus of aged mice and rats. In general, the authors have attributed the decrease in nuclear receptor expression to an age-related reduction in the bioavailability of RA [38, 39]. More recently, retinoid hyposignaling, as evidenced by the reduced expression of retinoid receptors, has also been reported in peripheral blood mononuclear cells of aged humans [38]. However, in animal models, the age-related hypoactivity of the retinoid signaling pathway is reversible by chronic treatment with RA, which also very selectively suppresses deficits in relational memory [40] and synaptic plasticity [41]. To date, the potential reversibility of vitamin A hypoactivity in humans remains a major issue.
Interestingly, dietary vitamin A supplementation throughout life is effective in normalizing the memory-encoding-induced recruitment of declarative memory circuits without affecting the activity of the procedural memory system in aged mice, and in alleviating their long-term declarative and working memory impairments [42]. Moreover, recent data confirm these observations, showing that vitamin A supplementation from midlife is sufficient to reverse the age-related deterioration of hippocampal neurogenesis (by enhancing the dendritic arborization of immature neurons, leading to improved neuronal survival and differentiation), and to prevent the related decline in spatial reference memory in the Morris Water Maze test (MWM). Moreover, it appears that the hippocampal expression of CRABP-I, which controls the intracellular availability of RA, may be an important regulator of the neuronal differentiation process in the aged hippocampus.
Taken together, these data suggest that Vitamin A supplementation would represent a preventive strategy to limit or delay the age-related disruption of hippocampal cellular properties induced by retinoid hyposignaling.
4Vitamin A interacts with the glucocorticoid pathway to modulate cognitive processes
A more indirect action of RA on memory processes involves the glucocorticoid (GC) pathway, known to modulate cognitive functions [43]. The hippocampus is a prime target for GCs, which bind to abundant corticosteroid receptors (mineralocorticoid receptors (MR) and glucocorticoid receptors (GR)), known to be involved in the control of hippocampal plasticity and functions [44]. As observed for retinoids, the most widespread view is that GC levels induce inverted-U-shaped dose effects on plasticity and cognitive functions [45–47]. The deleterious effects of a chronic excess of GCs on neuronal function become more marked with aging. Indeed, age-related cognitive deficits in humans [48, 49] and rodents [50, 51] have been construed as being conditioned by excess GC secretion into the plasma. Interestingly, in a subset of aged individuals who hypersecrete GCs, these cognitive dysfunctions are associated with reduced hippocampal volume [48]. Thus, the maintenance of optimal glucocorticoid levels throughout life could prevent the ravages of aging upon hippocampal structure and cognitive function.
In the following sections, we review some of the evidence that vitamin A status acts on GC availability at the peripheral and hippocampal levels, and that the stimulation of the retinoid pathway could be a successful strategy to counteract the deleterious effects of excess GCs on hippocampal function and cognitive alterations during aging.
4.1Vitamin A status modulates glucocorticoid availability: Consequences to cognitive processes
4.1.1Vitamin A status modulates plasma glucocorticoid levels
The effects of VAD on plasma GC status have recently been reported by our team in LOU/C rats [52], which are derived from the Wistar strain and described as a model of healthy aging due to their low level of age-related memory deficits [53]. Indeed, we have observed an increase in the activity of the hypothalamic-pituitary-adrenal (HPA) axis in LOU/C rats with VAD, leading to elevated total plasma corticosterone levels under basal and stress conditions, which can be reestablished by RA treatment [52]. However, contradictory results indicate that chronic treatment with high doses of RA can also induce a dysregulation of the HPA axis along with increased basal plasma corticosterone levels in young rats [54]. Regardless of the specific conditions, both sets of data suggest a relationship between abnormal RA levels (deficiency or excess) and a dysregulation of the HPA axis. However, the mechanisms by which RA signaling can modulate HPA axis activity remain poorly understood. Some recent data suggest that the hypothalamus and the pituitary gland are important retinoid target neuroendocrine areas involved in the control of plasma GC levels.
The hypothalamus is recognized as a key site of RA signaling in the brain [17, 55, 56]. Indeed, RA can be released into the hypothalamus and regulate a number of genes such as corticotropin-releasing hormone (CRH) via its receptors. An interesting recent study has proposed that an excess of RA could induce hypothalamic CRH overexpression through the transcriptional activation of the CRH promoter by RARα [57]. Retinoids can also modulate the HPA axis at the level of the pituitary gland. Cushing’s syndrome patients with a hypersecretion of cortisol due to adrenocorticotropic hormone (ACTH) overproduction by the pituitary exhibit reduced hippocampal volume and impaired performance on hippocampal learning tasks [58]. Interestingly, RA specifically inhibits ACTH production in human pituitary adenoma cells [59]. Thus, RA hyposignaling at the hypothalamic and pituitary levels could activate CRH and ACTH production and thereby stimulate plasma GC levels duringVAD.
4.1.2Vitamin A status modulates glucocorticoid transport
Although elevated plasma levels of GCs have been widely reported to contribute to age-related memory decline [43, 48, 60]), the dynamics of transfer of GCs from the blood to the brain are also important. It has recently been shown that corticosteroid-binding globulin (CBG), the plasma GC-binding protein, plays a critical role in the delivery of GCs to the brain, with an impact on memory retrieval [61, 62]. Indeed, CBG, which is synthesized in the liver and secreted into the blood, acts as a buffer for most systemic GCs by limiting the amount of circulating free hormones that can act on their receptors [63, 64]. We have recently reported that VAD leads to decreased CBG-binding capacity in Wistar rats. This has significant repercussions on the free plasma corticosterone fraction, which is higher in VAD rats compared to controls but normalized by vitamin A supplementation [65].
4.1.3Vitamin A status modulates hippocampal glucocorticoid levels
The magnitude of GC effects on cognitive functions depends not only on their plasma levels but also on the local pre-receptor metabolism of GCs by 11β-hydroxysteroid dehydrogenases (11β-HSDs) [66]. Indeed, 11β-HSD1 regenerates active GCs from their inactive forms in specific areas of the adult brain, such as the hippocampus [67], thereby effectively amplifying intracellular GC signaling [68]. Interestingly, the inhibitory effects of RA or vitamin A supplementation on the expression and activity of 11β-HSD1 have been demonstrated in differentiated C2C12 myotubes [69] and in obese rat livers [70]. Moreover, vitamin A-deficient LOU/C rats display an increase in HPA axis activity associated with an upregulation of the hippocampal expression of 11β-HSD1, which can be normalized by RA treatment [71]. This is of interest since 11β-HSD1 deficiency protects against normal hippocampus-associated cognitive decline both in aged mice [72–74] and in humans [75]. These effects of vitamin A status on hippocampal 11β-HSD1 could be mediated directly through nuclear retinoid receptors, which have been shown to negatively regulate 11β-HSD1 expression in in vitro models [69].
Recently, we demonstrated for the first time that the excess of hippocampal corticosterone in VAD rats is associated with a hyperactivity of hippocampal 11β-HSD1, and contributes to VAD-related spatial memory deficits and alterations of hippocampal plasticity [65]. Moreover, a negative correlation between hippocampal corticosterone levels and spatial memory has been found in VAD rats: the higher the hippocampal corticosterone levels, the poorer the spatial memory performance, suggesting that excess local GC levels result in memory deficits in these animals. One month of vitamin A supplementation corrects the excess hippocampal corticosterone levels and their consequences to spatial memory processes, in addition to reversing the VAD-induced alterations to normal hippocampal neurogenesis [65]. Since excess GCs are known to strongly inhibit adult hippocampal neurogenesis [76], these data suggest that the beneficial effects of vitamin A supplementation on neurogenesis in VAD rats could be mediated through a modulation of the hippocampal GC pathway. This hypothesis is also supported by the fact that 11β-HSD1 is expressed in the dentate gyrus, and increased hippocampal neurogenesis has been found in young 11β-HSD1 knockout mice [77]. Finally, RA minimizes the potentially deleterious effect of GCs on the decreased secretion of brain-derived neurotrophic factor (BDNF) in hippocampal HT22 cells [78], a neurotrophin known to stimulate adult neurogenesis processes and to be involved in the reduction of neurogenesis during chronic exposure to GCs [79].
4.2RA treatment: A strategy to modulate hippocampal GC availability during aging
As already mentioned above, stimulation of the retinoid pathway (by RA or vitamin A) in middle-aged rodents allows memory performance to be normalized. Given the data concerning the relationship between the retinoid pathway and GCs, the question of the involvement of the HPA axis in the mediation of these retinoid effects and their repercussions on memory is crucial. Indeed, it has been recently shown that the rise in intrahippocampal corticosterone levels in middle-aged mice is associated with memory impairments in a hippocampus-dependent memory task [80] known to be affected in midlife [81–86]. RA treatment in middle-aged mice reduces and delays the increase in intrahippocampal corticosterone after exposure to a novel environment, and restores memory performance in this task [87]. Interestingly, this effect is comparable to that of diazepam, which inhibits the stress-induced increase of hippocampal corticosterone levels and in parallel, restores memory performance in a hippocampus-dependent memory task [88]. In contrast to VAD rats, however, this effect of RA on hippocampal corticosterone levels in middle-aged mice is not due to its direct modulation of hippocampal 11β-HSD1, suggesting that other mechanisms are at work. One possibility is that RA treatment in middle-aged mice normalizes the elevated free plasma corticosterone levels, known to impact free hippocampal corticosterone levels with consequences to memory performance [43]. In summary, during aging, impaired RA signaling would induce an overactivation of the HPA axis, leading to excess plasma levels of GCs that can lastingly affect hippocampal plasticity and functions, and contribute to age-related cognitive decline.
Altogether, these recent data demonstrate for the first time a modulation of hippocampal GC availability by vitamin A status, one of the biological mechanisms by which retinoids could exert their effects on hippocampal plasticity and functions. This modulation may also explain the contribution of retinoids to the maintenance of memory processes throughout life and the impact of the age-related decrease in RA bioavailability on the etiology of cognitive decline.
5Conclusion
In order to determine whether nutritional intervention is a feasible strategy for the prevention or alleviation of age-related cognitive decline, it is of the first importance to better understand the involvement of the hypoactivity of the vitamin A signaling pathway in the genesis of cognitive impairment. Although the elderly possess a larger stock of vitamin A in the liver, all the experimental data from aged animals indicate that aging is accompanied by impaired vitamin A metabolism, leading to reduced RA synthesis and to retinoid hyposignaling in target tissues. In the brain, this metabolic perturbation induces alterations in neuronal plasticity and contributes to memory process impairments. Moreover, it has recently emerged that vitamin A status modulates the production of GC, both at the plasma and the hippocampal levels, and this antagonistic effect of Vitamin A on GC signaling seems to contribute positively to the maintenance of cognitive performance. Interestingly, experimental studies have demonstrated that RA could be pharmacologically effective in correcting age-related cognitive deficits, and some data obtained in animals also suggest that nutritional vitamin A supplementation might be a good approach to reduce or at least delay the onset of “normal” age-related cognitive decline. However, future investigations and additional prevention efforts are still needed to better determine the dietary forms and/or the doses of vitamin A that could be recommended in human population.
Acknowledgments
We thank the Aquitaine regional council for its financial support.
Glossary
ABBREVIATIONS
Abbreviation | Expansion |
---|---|
ADH | alcohol dehydrogenase |
ACTH | adrenocorticotropic hormone |
BDNF | brain-derived neurotrophic factor |
CBG | corticosteroid-binding globulin |
CNS | central nervous system |
CRABP | cellular retinoic acid binding protein |
CRBP | cellular retinol binding protein |
CRH | corticotropin-releasing hormone |
GC | glucocorticoid |
GR | glucocorticoid receptor |
HPA | hypothalamic-pituitary-adrenal |
11β-HSDs | 11β-hydroxysteroid dehydrogenase |
LRAT | lecithin retinol acyltransferase |
LTP | long-term potentiation |
LTD | long-term depression |
MR | mineralocorticoid receptor |
RA | retinoic acid |
RALDH | retinal dehydrogenase |
RAR | retinoic acid receptor |
RARE | RA response element |
RBP | retinol-binding protein |
RE | retinyl esters |
RXR | retinoid X receptor |
TR | thyroid hormone receptor |
TTR | transthyretin |
VAD | vitamin A deficiency |
References
[1] | Blomhoff R, Blomhoff HK(2006) Overview of retinoid metabolism and functionJ Neurobiol66: 606630 |
[2] | Allen SP, Maden M, Price JSA(2002) role for retinoic acid in regulating the regeneration of deer antlersDev Biol251: 409423 |
[3] | Harrison EH(1821) Mechanisms involved in the intestinal absorption of dietary vitamin A and provitamin A carotenoidsBiochim Biophys Acta7077 |
[4] | Bellovino D, Apreda M, Gragnoli S, Massimi M, Gaetani S(2003) Vitamin A transport: in vitro models for the study of RBP secretionMol Aspects Med24: 411420 |
[5] | Kawaguchi R, Yu J, Honda J, Hu J, Whitelegge J, Ping P, Wiita P, Bok D, Sun HA(2007) Membrane receptor for retinol binding protein mediates cellular uptake of vitamin AScience315: 820825 |
[6] | D’Ambrosio DN, Clugston RD, Blaner WS(2011) Vitamin A metabolism: An updateNutrients3: 63103 |
[7] | Thatcher JE, Isoherranen N(2009) The role of CYP26 enzymes in retinoic acid clearanceExpert Opin Drug Metab Toxicol5: 875886 |
[8] | Bastien J, Rochette-Egly C(2004) Nuclear retinoid receptors and the transcription of retinoid-target genes Gene328: 116 |
[9] | Benbrook DM, Chambon P, Rochette-Egly C, Asson-Batres MA(2014) History of retinoic Acid receptorsSubcell Biochem70: 120 |
[10] | Zhang DQ, McMahon DG(2000) Direct gating by retinoic acid of retinal electrical synapsesProc Natl Acad Sci U S A97: 1475414759 |
[11] | Dirks P, Tieding S, Schneider I, Mey J, Weiler R(2004) Characterization of retinoic acid neuromodulation in the carp retinaJ Neurosci Res78: 177185 |
[12] | Chen N, Napoli JL(2008) All-trans-retinoic acid stimulates translation and induces spine formation in hippocampal neurons through a membrane-associated RARalphaFaseb J22: 236245 |
[13] | Li JJ, Dong Z, Dawson MI, Colburn NH(1996) Inhibition of tumor promoter-induced transformation by retinoids that transrepress AP-1 without transactivating retinoic acid response elementCancer Res56: 483489 |
[14] | Jacka FN, Kremer PJ, Leslie ER, Berk M, Patton GC, Toumbourou JW, Williams JW(2010) Associations between diet quality and depressed mood in adolescents: Results from the Australian Healthy Neighbourhoods StudyAust N Z J Psychiatry44: 435442 |
[15] | Nanri A, Kimura Y, Matsushita Y, Ohta M, Sato M, Mishima N, Sasaki S, Mizoue T(2010) Dietary patterns and depressive symptoms among Japanese men and womenEur J Clin Nutr64: 832839 |
[16] | Maden M(2007) Retinoic acid in the development, regeneration and maintenance of the nervous systemNat Rev Neurosci8: 755765 |
[17] | Shearer KD, Stoney PN, Morgan PJ, McCaffery PJA(2012) Vitamin for the brainTrends Neurosci35: 733741 |
[18] | Lane MA, Bailey SJ(2005) Role of retinoid signalling in the adult brainProg Neurobiol75: 275293 |
[19] | Wang HL, Zhang Z, Hintze M, Chen L(2011) Decrease in calcium concentration triggers neuronal retinoic acid synthesis during homeostatic synaptic plasticityJ Neurosci31: 1776417771 |
[20] | McCaffery P, Zhang J, Crandall JE(2006) Retinoic acid signaling and function in the adult hippocampusJ Neurobiol66: 780791 |
[21] | Chiang MY, Misner D, Kempermann G, Schikorski T, Giguere V, Sucov HM, Gage FH, Stevens CF, Evans RM(1998) An essential role for retinoid receptors RARbeta and RXRgamma in long-term potentiation and depressionNeuron21: 13531361 |
[22] | Krezel W, Ghyselinck N, Samad TA, Dupe V, Kastner P, Borrelli E, Chambon P(1998) Impaired locomotion and dopamine signaling in retinoid receptor mutant miceScience279: 863867 |
[23] | Krzyzosiak A, Szyszka-Niagolov M, Wietrzych M, Gobaille S, Muramatsu S, Krezel W(2010) Retinoid x receptor gamma control of affective behaviors involves dopaminergic signaling in miceNeuron66: 908920 |
[24] | Alfos S, Boucheron C, Pallet V, Higueret D, Enderlin V, Beracochea D, Jaffard R, Higueret P(2001) A retinoic acid receptor antagonist suppresses brain retinoic acid receptor overexpression and reverses a working memory deficit induced by chronic ethanol consumption in miceAlcohol Clin Exp Res25: 15061514 |
[25] | Kornyei Z, Gocza E, Ruhl R, Orsolits B, Voros E, Szabo B, Vagovits B, Madarasz E(2007) Astroglia-derived retinoic acid is a key factor in glia-induced neurogenesisFaseb J.21: 24962509 |
[26] | Nomoto M, Takeda Y, Uchida S, Mitsuda K, Enomoto H, Saito K, Choi T, Watabe AM, Kobayashi S, Masushige S, Manabe T, Kida S(2012) Dysfunction of the RAR/RXR signaling pathway in the forebrain impairs hippocampal memory and synaptic plasticityMol Brain5: 8 |
[27] | Etchamendy N, Enderlin V, Marighetto A, Pallet V, Higueret P, Jaffard R(2003) Vitamin A deficiency and relational memory deficit in adult mice: Relationships with changes in brain retinoid signallingBehav Brain Res145: 3749 |
[28] | Bonnet E, Touyarot K, Alfos S, Pallet V, Higueret P, Abrous DN(2008) Retinoic acid restores adult hippocampal neurogenesis and reverses spatial memory deficit in vitamin A deprived ratsPLoS ONE3: e3487 |
[29] | Husson M, Enderlin V, Alfos S, Boucheron C, Pallet V, Higueret P(2004) Expression of neurogranin and neuromodulin is affected in the striatum of vitamin A-deprived ratsBrain Res Mol Brain Res123: 717 |
[30] | Ghenimi N, Beauvieux MC, Biran M, Pallet V, Higueret P, Gallis JL(2009) Vitamin A deficiency in rats induces anatomic and metabolic changes comparable with those of neurodegenerative disordersJ Nutr139: 696702 |
[31] | Misner DL, Jacobs S, Shimizu Y, de Urquiza AM, Solomin L, Perlmann T, De Luca LM, Stevens CF, Evans RM(2001) Vitamin A deprivation results in reversible loss of hippocampal long-term synapticplasticityProc Natl Acad Sci U S A98: 1171411719 |
[32] | Zhang M, Huang K, Zhang Z, Ji B, Zhu H, Zhou K, Li Y, Yang J, Sun L, Wei Z, He G, Gao L, He L, Wan C(2011) Proteome alterations of cortex and hippocampus tissues in mice subjected to vitamin A depletionJ Nutr Biochem22: 10031008 |
[33] | Borel P, Mekki N, Boirie Y, Partier A, Alexandre-Gouabau MC, Grolier P, Beaufrere B, Portugal H, Lairon D, Azais-Braesco V(1998) Comparison of the postprandial plasma vitamin A response in young and older adultsJ Gerontol A Biol Sci Med Sci.53: B133B140 |
[34] | van der Loo B, Labugger R, Aebischer CP, Bachschmid M, Spitzer V, Kilo J, Altwegg L, Ullrich V, Luscher TF(2004) Age-related changes of vitamin A statusJ Cardiovasc Pharmacol43: 2630 |
[35] | Feart C, Siewe A, Samieri C, Peuchant E, Helmer C, Alfos S, Pallet V, Barberger-Gateau P(2010) Plasma retinol and association with socio-demographic and dietary characteristics of free-living older persons: The Bordeaux sample of the three-city studyInt J Vitam Nutr Res80: 3244 |
[36] | Azais-Braesco V, Moriniere C, Guesne B, Partier A, Bellenand P, Baguelin D, Grolier P, Alix E(1995) Vitamin A status in the institutionalized elderly. Critical analysis of four evaluation criteria: Dietary vitamin A intake, serum retinol, relative dose-response test (RDR) and impression cytology with transfer (ICT)Int J Vitam Nutr Res65: 151161 |
[37] | Mino M, Tamai H, Tanabe T, Morinobu T, Ohsawa N, Takamatsu J, Miyata S, Hirahara F(1993) Nutritional status of antioxidant vitamins (A, E, and beta-carotene) in elderly JapaneseJ Nutr Sci Vitaminol (Tokyo)39 SupplS67S74 |
[38] | Feart C, Pallet V, Boucheron C, Higueret D, Alfos S, Letenneur L, Dartigues JF, Higueret P(2005) Aging affects the retinoic acid and the triiodothyronine nuclear receptor mRNA expression in human peripheral blood mononuclear cellsEur J Endocrinol152: 449458 |
[39] | Pallet V, Azais-Braesco V, Enderlin V, Grolier P, Noel-Suberville C, Garcin H, Higueret P(1997) Aging decreases retinoic acid and triiodothyronine nuclear expression in rat liver: Exogenous retinol and retinoic acid differentially modulate this decreased expressionMech Ageing Dev99: 123136 |
[40] | Etchamendy N, Enderlin V, Marighetto A, Vouimba RM, Pallet V, Jaffard R, Higueret P(2001) Alleviation of a selective age-related relational memory deficit in mice by pharmacologically induced normalization of brain retinoid signalingJ Neurosci21: 64236429 |
[41] | Féart C, Mingaud F, Enderlin V, Husson M, Alfos S, Higueret P, Pallet V(2005) Differential effect of retinoic acid and triiodothyronine on the age-related hypo-expression of neurogranin in ratNeurobiol Aging26: 729738 |
[42] | Mingaud F, Mormede C, Etchamendy N, Mons N, Niedergang B, Wietrzych M, Pallet V, Jaffard R, Krezel W, Higueret P, Marighetto A(2008) Retinoid hyposignaling contributes to aging-related decline in hippocampal function in short-term/working memory organization and long-term declarative memory encoding in miceJ Neurosci28: 279291 |
[43] | Yau JL, Seckl JR(2012) Local amplification of glucocorticoids in the aging brain and impaired spatial memoryFront Aging Neurosci4: 24 |
[44] | Oitzl MS, de Kloet ER(1992) Selective corticosteroid antagonists modulate specific aspects of spatial orientation learningBehav Neurosci106: 6271 |
[45] | Sandi C, Pinelo-Nava MT(2007) Stress and memory: Behavioral effects and neurobiological mechanismsNeural Plast78970 |
[46] | Joels M, Pu Z, Wiegert O, Oitzl MS, Krugers HJ(2006) Learning under stress: How does it work?Trends Cogn Sci10: 152158 |
[47] | Sandi C(2011) Glucocorticoids act on glutamatergic pathways to affect memory processesTrends Neurosci34: 165176 |
[48] | Lupien SJ, de Leon M, de Santi S, Convit A, Tarshish C, Nair NP, Thakur M, McEwen BS, Hauger RL, Meaney MJ(1998) Cortisol levels during human aging predict hippocampal atrophy and memory deficitsNat Neurosci1: 6973 |
[49] | Meaney MJ, O’Donnell D, Rowe W, Tannenbaum B, Steverman A, Walker M, Nair NP, Lupien S(1995) Individual differences in hypothalamic-pituitary-adrenal activity in later life and hippocampal agingExp Gerontol.30: 229251 |
[50] | Issa AM, Rowe W, Gauthier S, Meaney MJ(1990) Hypothalamic-pituitary-adrenal activity in aged, cognitively impaired and cognitively unimpaired ratsJ Neurosci10: 32473254 |
[51] | Yau JL, Olsson T, Morris RG, Meaney MJ, Seckl JR(1995) Glucocorticoids, hippocampal corticosteroid receptor gene expression and antidepressant treatment: Relationship with spatial learning in young and aged ratsNeuroscience66: 571581 |
[52] | Arvy N, Hamiani R, Richard E, Moisan MP, Pallet V(2013) Vitamin A regulates HPA axis status in LOU/C ratsJ Endocrinol219: 12127 |
[53] | Alliot J, Boghossian S, Jourdan D, Veyrat-Durebex C, Pickering G, Meynial-Denis D, Gaumet N(2002) The LOU/c/jall rat as an animal model of healthy aging?J Gerontol A Biol Sci Med Sci57: B312B320 |
[54] | Cai L, Yan XB, Chen XN, Meng QY, Zhou JN(2010) Chronic all-trans retinoic acid administration induced hyperactivity of HPA axis and behavioral changes in young ratsEur Neuropsychopharmacol20: 839847 |
[55] | Shearer KD, Goodman TH, Ross AW, Reilly L, Morgan PJ, McCaffery PJ(2010) Photoperiodic regulation of retinoic acid signaling in the hypothalamusJ Neurochem112: 246257 |
[56] | Helfer G, Ross AW, Russell L, Thomson LM, Shearer KD, Goodman TH, McCaffery PJ, Morgan PJ(2012) Photoperiod regulates vitamin A and Wnt/beta-catenin signaling in F344 ratsEndocrinology153: 815824 |
[57] | Hu P, Liu J, Zhao J, Qi XR, Qi CC, Lucassen PJ, Zhou JN(2013) All-trans retinoic acid-induced hypothalamus-pituitary-adrenal hyperactivity involves glucocorticoid receptor dysregulationTransl Psychiatry3: e336 |
[58] | Starkman MN, Gebarski SS, Berent S, Schteingart DE(1992) Hippocampal formation volume, memory dysfunction, and cortisol levels in patients with Cushing’s syndromeBiol Psychiatry32: 756765 |
[59] | Paez-Pereda M, Kovalovsky D, Hopfner U, Theodoropoulou M, Pagotto U, Uhl E, Losa M, Stalla J, Grubler Y, Missale C, Arzt E, Stalla GK(2001) Retinoic acid prevents experimental Cushing syndromeJ Clin Invest108: 11231131 |
[60] | Joels M, Karst H, Alfarez D, Heine VM, Qin Y, van Riel E, Verkuyl M, Lucassen PJ, Krugers HJ(2004) Effects of chronic stress on structure and cell function in rat hippocampus and hypothalamusStress7: 221231 |
[61] | Breuner CW, Orchinik M(2002) Plasma binding proteins as mediators of corticosteroid action in vertebratesJ Endocrinol175: 99112 |
[62] | Minni AM, Dorey R, Pierard C, Dominguez G, Helbling JC, Foury A, Beracochea D, Moisan MP (2012) Critical role of plasma corticosteroid-binding-globulin during stress to promote glucocorticoid delivery to the brain: Impact on memory retrieval Endocrinology 153: 4766 4774 |
[63] | Richard EM, Helbling JC, Tridon C, Desmedt A, Minni AM, Cador M, Pourtau L, Konsman JP, Mormede P, Moisan MP(2010) Plasma transcortin influences endocrine and behavioral stress responses in miceEndocrinology151: 649659 |
[64] | Moisan MP(2010) Genotype-phenotype associations in understanding the role of corticosteroid-binding globulin in health and disease animal modelsMol Cell Endocrinol316: 3541 |
[65] | Bonhomme D, Minni AM, Alfos S, Roux P, Richard E, Higueret P, Moisan MP, Pallet V, Touyarot K(2014) Vitamin A status regulates glucocorticoid availability in Wistar rats: Consequences on cognitive functions and hippocampal neurogenesis?Front Behav Neurosci8: 20 |
[66] | Seckl JR(1997) 11beta-Hydroxysteroid dehydrogenase in the brain: A novel regulator of glucocorticoid action?Front Neuroendocrinol18: 4999 |
[67] | Moisan MP, Seckl JR, Edwards CR(1990) 11 beta-hydroxysteroid dehydrogenase bioactivity and messenger RNA expression in rat forebrain: Localization in hypothalamus, hippocampus, and cortexEndocrinology127: 14501455 |
[68] | Holmes MC, Yau JL, Kotelevtsev Y, Mullins JJ, Seckl JR(1007) 11 Beta-hydroxysteroid dehydrogenases in the brain: Two enzymes two rolesAnn N Y Acad Sci1007: 35766 |
[69] | Aubry EM, Odermatt A(2009) Retinoic acid reduces glucocorticoid sensitivity in C2C12 myotubes by decreasing 11beta-hydroxysteroid dehydrogenase type 1 and glucocorticoid receptor activitiesEndocrinology150: 27002708 |
[70] | Sakamuri VP, Ananthathmakula P, Veettil GN, Ayyalasomayajula V(2011) Vitamin A decreases pre-receptor amplification of glucocorticoids in obesity: Study on the effect of vitamin A on 11beta-hydroxysteroid dehydrogenase type 1 activity in liver and visceral fat of WNIN/Ob obese ratsNutr J10: 70 |
[71] | Marissal-Arvy N, Hamiani R, Richard E, Moisan MP, Pallet V(2013) Vitamin A regulates hypothalamic-pituitary-adrenal axis status in LOU/C ratsJ Endocrinol219: 2127 |
[72] | Yau JL, Noble J, Kenyon CJ, Hibberd C, Kotelevtsev Y, Mullins JJ, Seckl JR(2001) Lack of tissue glucocorticoid reactivation in 11beta-hydroxysteroid dehydrogenase type 1 knockout mice ameliorates age-related learning impairmentsProc Natl Acad Sci U S A98: 47164721 |
[73] | Sooy K, Webster SP, Noble J, Binnie M, Walker BR, Seckl JR, Yau JL(2010) Partial deficiency or short-term inhibition of 11beta-hydroxysteroid dehydrogenase type 1 improves cognitive function in aging miceJ Neurosci30: 1386713872 |
[74] | Mohler EG, Browman KE, Roderwald VA, Cronin EA, Markosyan S, Scott Bitner R, Strakhova MI, Drescher KU, Hornberger W, Rohde JJ, Brune ME, Jacobson PB, Rueter LE(2011) Acute inhibition of 11beta-hydroxysteroid dehydrogenase type-1 improves memory in rodent models of cognitionJ Neurosci31: 54065413 |
[75] | Sandeep TC, Yau JL, MacLullich AM, Noble J, Deary IJ, Walker BR, Seckl JR(2004) 11Beta-hydroxysteroid dehydrogenase inhibition improves cognitive function in healthy elderly men and type 2 diabeticsProc Natl Acad Sci U S A101: 67346739 |
[76] | Gould E, Tanapat P(1999) Stress and hippocampal neurogenesisBiol Psychiatry46: 14721479 |
[77] | Yau JL, McNair KM, Noble J, Brownstein D, Hibberd C, Morton N, Mullins JJ, Morris RG, Cobb S, Seckl JR(2007) Enhanced hippocampal long-term potentiation and spatial learning in aged 11beta-hydroxysteroid dehydrogenase type 1 knock-out miceJ Neurosci27: 1048710496 |
[78] | Brossaud J, Roumes H, Moisan MP, Pallet V, Redonnet A, Corcuff JB(2013) Retinoids and glucocorticoids target common genes in hippocampal HT22 cellsJ Neurochem125: 518531 |
[79] | Duman RS, Monteggia LM(2006) A neurotrophic model for stress-related mood disordersBiol Psychiatry59: 11161127 |
[80] | Chauveau F, Pierard C, Tronche C, Coutan M, Drouet I, Liscia P, Beracochea D(2009) The hippocampus and prefrontal cortex are differentially involved in serial memory retrieval in non-stress and stress conditionsNeurobiol Learn Mem91: 447455 |
[81] | Tronche C, Pierard C, Coutan M, Chauveau F, Liscia P, Beracochea D(2010) Increased stress-induced intra-hippocampus corticosterone rise associated with memory impairments in middle-aged miceNeurobiol Learn Mem93: 343351 |
[82] | Beracochea D, Boucard A, Trocme-Thibierge C, Morain P(2008) Improvement of contextual memory by S 5 in aged mice: Comparison with memantinePsychopharmacology (Berl)196: 555564 |
[83] | Beracochea D, Liscia P, Tronche C, Chauveau F, Jouanin JC, Pierard C(2008) Stress modulation of the memory retrograde-enhancing effects of the awakening drug modafinil in micePsychopharmacology (Berl)196: 113 |
[84] | Celerier A, Pierard C, Rachbauer D, Sarrieau A, Beracochea D(2004) Contextual and serial discriminations: A new learning paradigm to assess simultaneously the effects of acute stress on retrieval of flexible or stable information in miceLearn Mem11: 196204 |
[85] | Chauveau F, Pierard C, Corio M, Celerier A, Christophe T, Vouimba RM, Guillou JL, Beracochea D(2009) Mediodorsal thalamic lesions block the stress-induced inversion of serial memory retrieval pattern in miceBehav Brain Res203: 270278 |
[86] | Pierard C, Tronche C, Liscia P, Chauveau F, Beracochea D(2009) Combined effects of acute stress and amphetamine on serial memory retrieval pattern in micePsychopharmacology (Berl)203: 463473 |
[87] | Bonhomme D, Pallet V, Dominguez G, Servant L, Henkous N, Lafenetre P, Higueret P, Beracochea D, Touyarot K(2014) Retinoic acid modulates intrahippocampal levels of corticosterone in middle-aged mice: Consequences on hippocampal plasticity and contextual memoryFront Aging Neurosci6: 6 |
[88] | Beracochea D, Tronche C, Coutan M, Dorey R, Chauveau F, Pierard C(2011) Interaction between Diazepam and Hippocampal Corticosterone after Acute Stress: Impact on Memory in Middle-Aged MiceFront Behav Neurosci5: 14 |
Figures and Tables
Fig.1
The retinoid cascade. Vitamin A is present in the diet as retinyl esters (animal food) and as provitamin A carotenoids (vegetables and fruits). Intestinal enzymes are required for several processes leading to re-esterification of retinol in enterocytes prior to incorporation into chylomicrons for lymphatic transport. Vitamin A is stored in the liver as retinyl esters which are hydrolysed and released as retinol, in response to the body’s needs, to maintain a constant concentration of retinol in the plasma, bound to retinol-binding protein (RBP) and to transthyretin (TTR). In target tissues as brain, retinol is locally converted to RA through sequential oxidative steps involving retinol (ADH) and retinaldehyde (RALDH) dehydrogenases. RA may then enter the nucleus to bind to RA receptors in order to modulate gene transcription on the hormone responsive element (HRE).
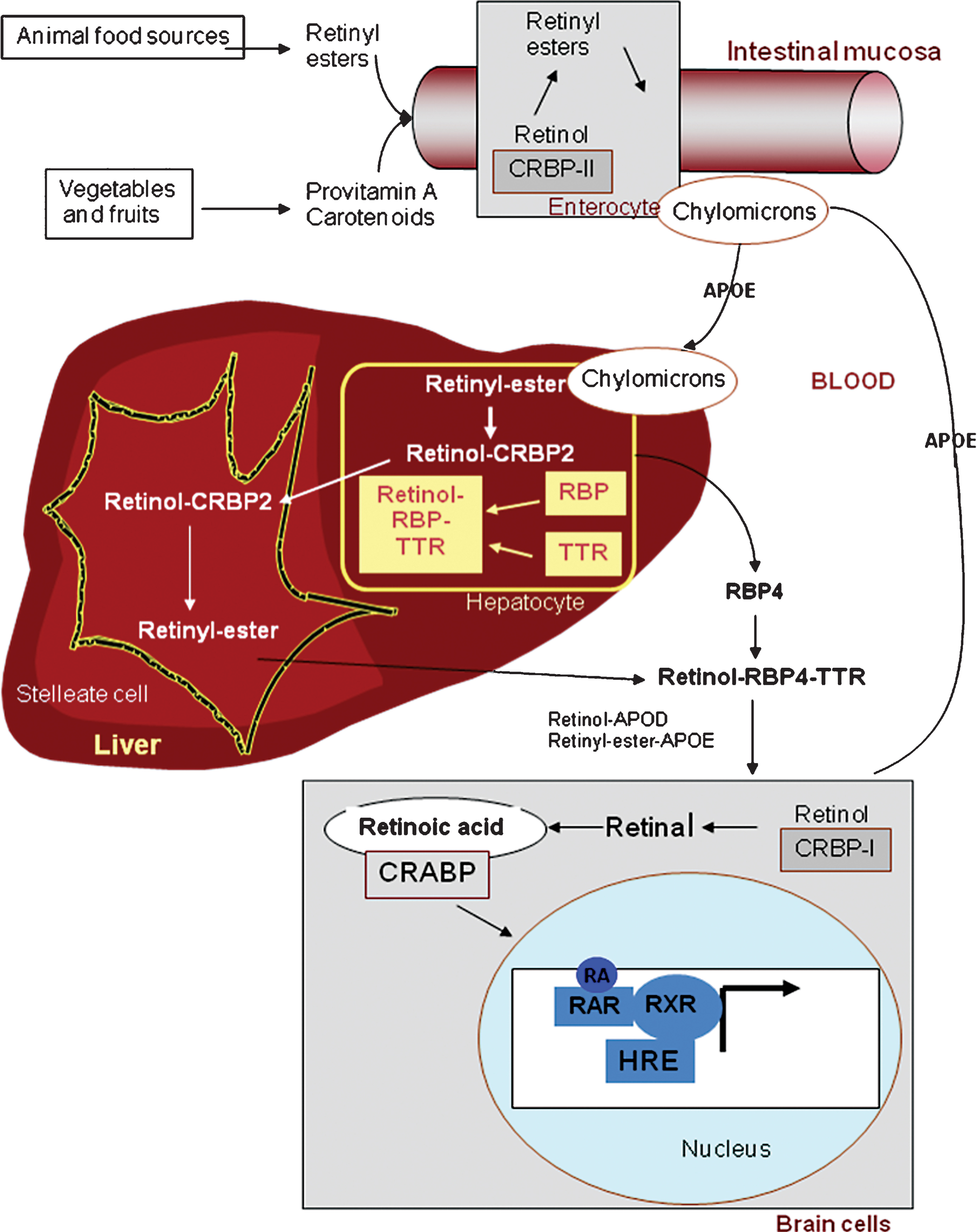