Opportunities and Pitfalls of REM Sleep Behavior Disorder and Olfactory Dysfunction as Early Markers in Parkinson’s Disease
Abstract
During its pre-motor stage, Parkinson’s disease (PD) presents itself with a multitude of non-motor symptoms with different degrees of specificity and sensitivity. The most important among them are REM sleep behavior disorder (RBD) and olfactory dysfunction. RBD is a parasomnia characterized by the loss of REM sleep muscle atonia and dream-enacting behaviors. Olfactory dysfunction in individuals with prodromal PD is usually described as hyposmia (reduced sense of smell) or anosmia (complete loss of olfactory function). These symptoms can precede the full expression of motor symptoms by decades. A close comprehension of these symptoms and the underlying mechanisms may enable early screening as well as interventions to improve patients’ quality of life. Therefore, these symptoms have unmatched potential for identifying PD patients in prodromal stages, not only allowing early diagnosis but potentially opening a window for early, possibly disease-modifying intervention. However, they come with certain challenges. This review addresses some of the key opportunities and pitfalls of both RBD and olfactory dysfunction as early markers of PD.
INTRODUCTION
Parkinson’s disease (PD) is characterized by the degeneration of dopaminergic neurons (neurons involved in the control of voluntary movements) in the substantia nigra pars compacta (SNpc) and the loss of their axonal endings in the striatum [1]. The main pathological hallmark of the earliest phases of PD is the intracellular fibrilization of alpha-synuclein, along with Lewy body brainstem pathology [2, 3]. This process is associated with the progressive onset of motor symptoms. Accordingly, PD is currently diagnosed based on the presence of the pathognomonic motor parkinsonian signs, such as bradykinesia, rest tremor, and rigidity [1]. However, these motor symptoms become evident only relatively late in the disease development, when already 50–70% of dopaminergic neurons have been lost [2]. For the development of disease-modifying therapeutic interventions, it is therefore of utmost importance to detect PD well before the development of motor symptoms, in the so-called pre-motor stage.
As a matter of fact, a multitude of non-motor symptoms can be present in the pre-motor stage, such as sleep disorders, autonomic and olfactory dysfunction. Among these, the presence of olfactory loss is a supportive criterion for the clinical diagnosis of PD [1]. Typically, these symptoms antedate motor symptoms by years if not decades, and they become increasingly present over the course of the disease, negatively affecting patient’s quality of life. Sleep disturbances, in particular rapid eye movement (REM) sleep behavior disorder (RBD), and olfactory dysfunction (OD) such as hyposmia (reduced sense of smell) or anosmia (complete loss of olfactory function), are therefore potential prodromal markers of PD, with the former being present in almost half of the patients with a diagnosis of PD, and the latter in almost all of them [4, 5] (Fig. 1).
Fig. 1
Brain areas involved in olfactory dysfunction, REM Behavior Disorder (RBD) and parkinsonism. So far, most of the research on this topic has been conduct on animals. This figure represents the human equivalent of regions in animal models, mainly in rats and cats. For what concerns REM motor control centers, in particular, the sublaterodorsalis nucleus (rats) and the peri-locus coeruleus alpha (cats) are responsible for muscle atonia in REM sleep. These regions correspond to the locus subcoeruleus and the magnocellular reticular formation area in humans.
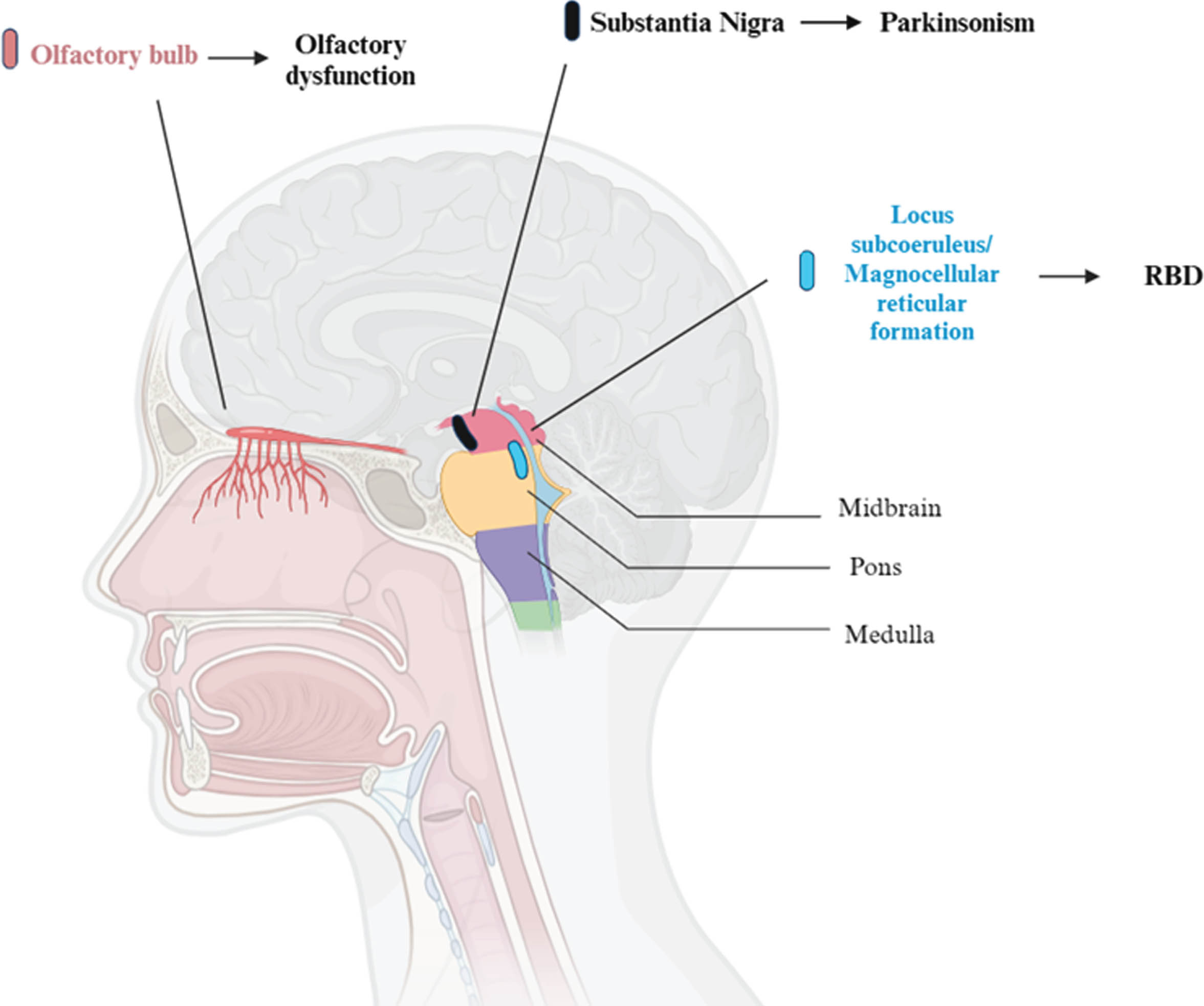
The main advantage in using an early PD marker such as RBD or OD is the opportunity of detecting patients suffering from alpha-synucleinopathy years before the emergence of overt motor and cognitive symptoms. This may allow a timely intervention to be applied in the earlier stages of the neurodegenerative process. Two approaches are interesting in this context. First, disease-modifying therapies may be developed. Second, several risk factors for PD, e.g., lifestyle and food habits, are potentially modifiable [6]. Therefore, the identification of individuals with an increased risk of developing a neurodegenerative disease could lead to a preventive adaptation of these risk factors [7].
While these markers hold promise for early diagnosis and intervention, they also come with certain opportunities and pitfalls. This review aims to unravel some of the main issues that makes RBD and OD such important prodromal features to be studied in PD from the very prodromal stages. A summary of the main opportunities and pitfalls of RBD and OD as early markers of PD is shown in Table 1.
Table 1
Summary of the main opportunities and pitfalls of using REM sleep behaviour disorder and olfactory dysfunction (OD) as early biomarker of Parkinson’s Disease
Opportunities | Pitfalls | |
REM sleep behavior disorder | Strong risk factor for developing the overt, motor phase of PD | High heterogeneity in time of phenoconversion and symptoms manifestation |
RBD can be combined with other risk factors for better stratification of patients | Not every patient with PD might have had exhibited RBD prior to diagnosis | |
Clinical and instrumental diagnosis of RBD requires a cost-effective, non-invasive, and widely available tool, polysomnography. | Not every patient with RBD would develop PD (47–64%) | |
Trial-ready cohorts of iRBD patients | ||
Olfactory dysfunction | Can be measured with inexpensive, rapid and sensitive olfactory tests. | OD is not specific to PD. |
It is not yet possible to differentiate a PD-related OD from other forms of OD based on olfactory testing alone. | ||
Olfactory tests can be combined with other risk factors. | ||
Olfactory dysfunction is very sensitive to PD and prodromal forms. |
REM sleep behavior disorder
RBD is a parasomnia characterized by the loss of the physiological muscle atonia during REM sleep, leading to the enactment of dreams [8]. It is referred to as “idiopathic” or “isolated” (iRBD) when not associated to overt neurologic symptoms such as dementia or parkinsonism. According to the 3rd edition of the International classification of sleep disorders (ICSD-3), a clinical diagnosis of RBD is carried out in presence of REM sleep without atonia recorded by an overnight video-polysomnography (Fig. 2), along with the demonstration of repetitive episodes of vocalizations and/or complex motor behaviors occurring during REM sleep [9]. For widespread use, one may pre-screen with screening tools with excellent (above 90%) sensitivity, such as questionnaires (e.g., the Mayo Sleep Questionnaire [10] and the Innsbruck RBD Inventory [11]), e.g., when aiming for narrowing down the pool of potential trial participants [12].
Fig. 2
Polysomnographic epoch of an iRBD patients. Green lines (EOG-L and EOG-R) shows Rapid Eye Movements both for left and right eyes, while EMG records chin muscle activity. This recording shows the presence of REM Sleep Without Atonia (RWA) during REM sleep. EOG, electro-oculogram; EMG, electro-myogram; EKG, electro-cardiogram; Flow, oro-nasal air flow; Thor, thoracic effort; Abd, abdominal effort; FDS, flexor digitorum superficialis; AT, anterior tibialis; L, left; R, right.
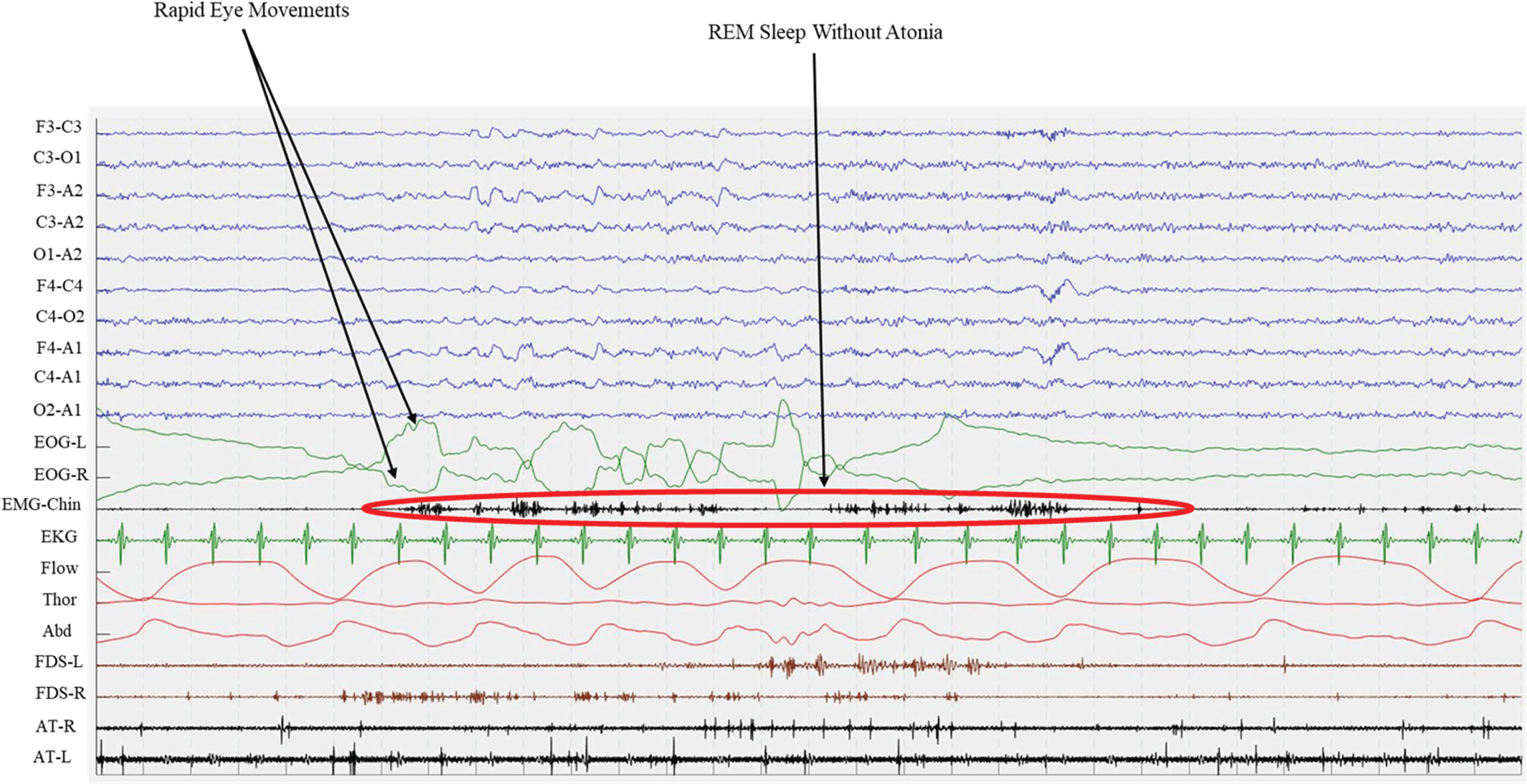
Today iRBD is considered a prodromal stage of alpha-synucleinopathies, namely Parkinson’s disease (PD), dementia with Lewy bodies (DLB), and multiple system atrophy (MSA) [13–15]. In fact, within 12 years from diagnosis, the majority of iRBD patients will phenoconvert to an overt alpha-synucleinopathy, with a phenoconversion rate of almost 7% per year [13–15]. Among these, roughly 57% of patients will develop “parkinsonism first” syndrome (of whom almost 5% being MSA), while up to 43% will develop a “dementia first” syndrome [13]. Additionally, most of iRBD patients already exhibit pathological alpha-synuclein accumulation in the cerebrospinal fluid and/or tissues, including skin [16–20]. In fact, about 90% of patients with iRBD showed a positive Seed Amplification Assay (SAA) for alpha-synuclein in the cerebrospinal fluid [17–19, 21]. Interestingly, the few patients with a negative alpha-synuclein SAA are at very low risk (hazard ratio 0.14) of developing parkinsonism or dementia over time [17]. Similarly, misfolded alpha-synuclein can also be found in the skin of about 80% of iRBD patients, either using SAA or immunofluorescence [22, 23].
So far, disease modification clinical trials have been unsuccessful in PD [24, 25]. However, a recent proof-of-concept study suggested that a neuroprotection trial is feasible in iRBD patients [26], putting forward that using disease-modifying therapies on prodromal stage of synucleinopathies may be more effective. Therefore, iRBD patients may be the ideal, and the readiest target population for testing disease-modifying treatments. However, to design such trials, we need biomarkers that can more precisely predict short-term phenoconversion to the overt stage of synucleinopathies.
Opportunities of RBD as early marker in PD
One clear advantage of RBD as a PD early marker is that both the clinical and instrumental diagnosis of RBD is straightforward and internationally accepted and based on polysomnography, a cost-effective, non-invasive, and widely available tool.
In fact, the presence of RBD is by far the strongest risk factor for developing the overt, motor phase of PD [27]. Indeed, a new biological definition of PD suggests that there should no longer be distinction between “prodromal” and “defined” disease stages, but rather the identification of clinical symptoms and signs of PD that have occurred as a consequence of the alpha-synucleinopathy neurodegenerative process [28, 29]. These include the cardinal motor signs of parkinsonism, but also polysomnography proven RBD. However, to identify iRBD patients at high risk of short term phenoconversion, several other prodromal risk factors, such as age, migro-striatal dopaminergic impairment, mild cognitive impairment (MCI), and OD, have been extensively studied in iRBD cohorts worldwide.
In iRBD patients, the most obvious risk factor for phenoconversion is age. Indeed, older patients—especially older than 70 years of age—are at high risk of phenoconversion on the short term [13–15]. These patients may, however, not be the ideal target for testing disease-modifying therapies.
Another very strong risk factors for short term phenoconversion in iRBD patients is nigro-striatal dopaminergic impairment, as measured by dopamine transporter (DAT) SPECT with [123I]FP-CIT, with an adjusted Hazard Ratio (aHR) >4 [14, 15]. This has been shown in single center studies [30, 31] and later confirmed in multicenter initiatives [13, 14]. Interestingly, DAT-SPECT metrics worsen faster over time in iRBD patients than in healthy controls [32], reflecting progressive nigro-striatal dopaminergic dysfunction. Therefore, DAT-SPECT may be considered a staging biomarker, able to identify iRBD patients with high risk of short-term phenoconversion. Moreover, DAT-SPECT may be also able to monitor neurodegeneration progression, and it might be considered as a surrogate/additional efficacy endpoint for disease-modifying treatment trials [26].
Next, MCI can be observed in about 30% of iRBD patients [13, 33], and is an additional strong risk factor for short-term phenoconversion (aHR > 2.3), especially for developing the dementia-first phenotype (aHR > 2.7) [13, 33]. Thus, the presence of MCI may be used to stratify those patients that are likely to develop the dementia-first phenotype. To note, MCI diagnostic criteria and the neuropsychological tests used in the literature are highly heterogeneous; therefore, a consensus on the neuropsychological assessment for iRBD patients is needed [34].
Moreover, OD is one of the earliest non-motor symptoms to occur in PD (see section below), and has emerged as an intriguing and potentially important feature associated with RBD, manifesting even more than 20 years before phenoconversion [35, 36]. It is worth nothing that OD has a good predictive value for short-term phenoconversion in iRBD patients (aHR > 2.6) [13]. Other non-motor biomarkers (e.g., urinary dysfunctions, orthostatic symptoms, depression/ anxiety) have shown only moderate ability in predicting phenoconversion in iRBD patients [13]. Similarly, they did not distinguish between iRBD patients who converted to “dementia first” versus those who converted to “parkinsonism first” [13].
Other biomarkers studied in iRBD such as fluorodeoxyglucose positron emission tomography, magnetic resonance imaging as well as neurophysiological metrics are extensively described in details elsewhere [37].
Most literature analyzed individual biomarkers with univariate approaches. Still, a recent multicentric study showed, using a multivariate approach, that the combination of age older than 70, constipation and abnormal DAT-SPECT at putamen level best identifies iRBD at risk of short term phenoconversion [14]. Future studies should investigate the combined weight of the most promising phenoconversion biomarkers in iRBD, such as DAT-SPECT, alpha-synuclein assays, MCI, autonomic symptoms, and OD. Finally, one crucial advantages in using RBD as an early PD marker, in the light of future disease-modifying trials, is that large trial ready cohorts of iRBD patients already exist in North America [38] and in Europe [39, 40].
Pitfalls of RBD as early marker in PD
Despite the potential of using RBD as an early marker of PD, there are some issues that must be considered. One of the biggest challenges of early biomarkers is the high heterogeneity in time of phenoconversion and symptoms manifestation in prodromal patients. In fact, the mean interval between RBD diagnosis and phenoconversion is 2–4 years, with a median time of 2–8 years [13–15]. However, few patients may have a longstanding prodromal phase that last more than 10 years [41]. Therefore, it is crucial to stratify the patients according to their risk of developing the overt PD stage.
Further, it is important to highlight that not every patient had exhibited RBD prior to diagnosis. In fact, the pooled prevalence of RBD in PD patients is 46% [42]. Therefore, using RBD as an early marker for PD might represent a risk of identifying only one specific PD phenotype. This “PD with RBD” phenotype is characterized by more severe motor and non-motor symptoms, poorer quality of life and worse prognosis [42–44]. Indeed, in up to 42% of patients PD is associated with both RBD and MCI [45], representing a malignant PD phenotype, as opposed to a more benign one, with predominant motor symptoms [46]. Such a distinction may have clinical implications, as patients with the malignant phenotype are less responsive to dopaminergic therapies and exhibit early impairment of daily life activities [33].
Dream enactment behaviors might also be of non-neurodegenerative origin, such as narcolepsy, limbic encephalitis, brainstem lesions, post-traumatic stress disorder and traumatic brain injury [47, 48]. However, most such cases do not fall in the definition of idiopathic/isolated RBD because of the presence of other neurological or psychiatric signs and symptoms.
Finally, not every patient with iRBD will develop PD. In fact, 47–64% of iRBD patients phenoconvert to PD, 35–46% to DLB, and 2–7% to MSA [13–15]. While MSA is rare, with a specific pathophysiological process involving oligodendroglia alpha-synucleinopathy, PD and DLB share a common pathophysiological process, that is the presence of neuronal alpha-synuclein aggregates (Lewy bodies). Indeed, even if PD and DLB have several clinical, imaging and neuropathologic differences [49], they may be considered part of the same Lewy body disorders spectrum including PD, PD dementia, and DLB. To note, the presence of RBD seems to characterize a specific (more severe) group of patients along this continuum. Indeed, RBD is present in most DLB patients, and identifies those PD patients at high risk of developing cognitive impairment and dementia, as compared to mainly motor PD patients [44, 50].
Olfactory dysfunction in Parkinson’s disease
OD is common, affecting up to 20% of the general population [51]. It can be quantitative, i.e., complete (anosmia) or partial (hyposmia), or qualitative, i.e., distorted odor perception (parosmia) or perception of odors in the absence of an odor source (phantosmia) [52]. OD considerably impacts quality of life, as it can result in reduced awareness of danger cues (smoke, spoiled food), abnormal eating behavior (increased salt/sugar intake, anorexia), anxiety and social isolation (due to lacking perception of the own body odor) [53]. There are many underlying causes of OD, including sinunasal diseases, viral infection of the upper respiratory tract, traumatic brain injury, congenital anosmia, exposure to toxic substances, nasal surgery, or neurodegenerative diseases [54].
In fact, OD is a common non-motor symptom of PD [55, 56] as more than 90% of PD patients exhibit OD [57, 58]. Most reports on OD in PD focus on quantitative aspects; there is little to no systematic literature available on qualitative OD [59]. OD occurs early during disease progression, as much as 10 to 20 years before the onset of motor disorders and hence diagnosis [27]. While the exact mechanisms responsible for OD in PD are not completely understood, the olfactory bulb—the first relay station of the olfactory pathway that receives neuronal input from the olfactory receptor neurons in the olfactory epithelium of the nasal cavity—is one of the first brain areas to show accumulation of Lewy bodies [60, 61]. In fact, PD pathology spreads throughout the brain in a specific fashion, impacting the olfactory bulb and the anterior olfactory nucleus in the early stages [60, 62]. One fascinating albeit controversial hypothesis that could explain this pattern is that the olfactory epithelium may be one of two potential entry points for the hitherto unknown pathogen causing PD (the other being the gut) [63, 64].
OD can play a role in the clinical diagnosis of PD, particularly in its early phase, as the presence of OD is a supportive criterion for diagnosis [1, 65]. However, this is complicated by the fact that, as many individuals with OD, patients with PD are often not aware of OD [66]. Further, although OD is a sensitive early marker for PD, its low specificity limits the clinical application for screening.
Opportunities of OD as early marker in PD
Olfactory testing has the potential to serve as an early, non-invasive, and inexpensive screening method for PD. Different methods allow for measuring olfactory function; however, only few are both well-validated and commercially available. While the UPSIT is a pure odor identification test [67], the Sniffin’ Sticks test allows for the combined assessment of odor identification, odor discrimination and odor detection thresholds [68]. As PD affects all olfactory tasks in an uniform manner compared to, e.g., Alzheimer’s disease [69, 70], both the UPSIT and the Sniffin’ Sticks test are effective in detecting a PD-related OD [55]. However, they both are relatively time-consuming. Shorter versions of these tests also allow for the differentiation of PD patients and healthy controls [71, 72].
Further, olfactory testing also allows to differentiate PD from other neurological disorders. More specifically, odor identification tests distinguish PD patients from individuals with other neurological disorders such as MSA, supranuclear palsy, essential tremor, drug-induced parkinsonism, restless leg syndrome, pure autonomic failure, vascular parkinsonism, corticobasal disease, and postural instability with rest tremor [55].
To differentiate PD-related OD and non-Parkinsonian OD, routine olfactory tests should therefore be administered together with complementary (olfactory) tests. One possibility may be retro-nasal testing, i.e., the perception of olfactory stimuli in the oral cavity perceived via the nasopharynx. This main contributor to flavor perception appears to be distinctly affected in PD [73]. Another method is to combine olfactory testing with other chemosensory tests. In this context, the trigeminal system is of particular interest. This third chemosensory system next to smell and taste that allows the perception of sensations such as the freshness, warmth, and pungency of odorants, via the activation of nasal chemoreceptors on the trigeminal nerve, i.e., cranial nerve V [74]. Typically, OD is associated with a loss of trigeminal sensitivity, but this is not the case in PD [75]. In other words, trigeminal sensitivity distinguishes PD-related OD from a non-Parkinsonian OD [75]. This PD specific chemosensory impairment pattern can be observed with behavioral, electrophysiological and neuroimaging techniques on different processing levels [76]. The study of this interaction is therefore an interesting avenue for the early detection of PD.
Pitfalls of OD as early marker in PD
One of the current challenges in using olfactory tests for the early detection of PD is their lack of specificity. In fact, one in three elderly individuals have olfactory loss due to a variety of other etiologies. Many forms of OD are caused by pathologies unlikely to be related to PD, such as sinunasal disease or viral infections of the upper respiratory tract. However, idiopathic OD, which represents approximately one sixth of OD [52], is a prime candidate for an early marker for PD, being associated with an increased risk of developing PD [77–79]. However, given the low specificity of OD, important parameters such as median phenoconversion time and percentage of converters are still unknown. In this context it is important to point out that repeating the number of olfactory assessments may improve the specificity of odor identification tests for the early detection of PD-related OD [80]. Machine learning may combine olfactory tests with other non-motor data (e.g., handwriting patterns, movement, neuroimaging, cerebrospinal fluid, voice) to increase diagnostic specificity [81].
While olfactory testing allows to differentiate PD from a series of neurological diseases [55], it remains challenging to distinguish PD-related OD from OD caused by other neurodegenerative conditions such as Alzheimer’s disease [82]. Nevertheless, PD patients exhibit greater impairment in detection threshold to Alzheimer’s disease patients, while the latter group appears to be more affected in terms of identification [69]. When aiming to distinguish PD-related OD from OD caused by other neurodegenerative diseases, individuals with iRBD are of particular interest.
In fact, olfactory testing enables the identification of iRBD patients at short-term risk of developing DLB or PD [66]. However, one cannot yet distinguish the phenoconversion trajectory with smell tests alone, even if, after phenoconversion, DLB patients and PD patients seem to be distinguishable using olfactory and trigeminal threshold test [73, 74]. The combined study of the olfactory and trigeminal systems appears to be a promising avenue, albeit this remains speculative. Importantly, both the presence and the predictive value of a PD-specific impairment pattern has yet to be shown in prodromal PD [72, 73].
Conclusions
The search for early biomarkers of PD is a critical area of research with the potential to revolutionize the diagnosis and management of this condition. As research in these areas continues to evolve, it is essential to consider the broader implications for clinical practice. RBD and OD represent two promising candidates in this pursuit. Their association raises intriguing questions about shared pathophysiology and their potential role as early markers for neurodegenerative diseases. Indeed, olfactory assessment, when combined with DAT-SPECT, increases its specificity and sensitivity in the prediction of phenoconversion of iRBD patients [83] pointing out the usefulness of using combined biomarkers, instead of single ones [14, 37]. RBD’s strong association with synucleinopathies, including PD, and its potential to serve as a prodromal marker make it a valuable area of investigation. Similarly, OD’s high prevalence in PD, its ability to precede motor symptoms, and its potential as a predictive marker when combined with nigrostriatal dopaminergic imaging underscore its significance in the early detection of the disease. To note, a recent study showed that almost all iRBD patient with OD had a positive alpha-synuclein assay [18], suggesting that the combined use of these two biomarkers may be the best choice for selecting the patients to be enrolled in disease-modifying trials.
In summary, both RBD and OD show promise as early markers for PD, offering opportunities for early detection, monitoring, and research. However, they should be used in conjunction with other biomarkers, so to increase specificity and sensitivity, but also to identify those patients at higher risk of developing the motor symptoms of PD. While much progress has been made, further research is needed to refine the use of these biomarkers in clinical practice and improve the accuracy of early PD diagnosis. Additionally, ongoing research into the underlying mechanisms linking these biomarkers to PD will deepen our understanding of the disease and may lead to the design of successful disease-modifying clinical trials.
ACKNOWLEDGMENTS
The authors have no acknowledgments to report.
FUNDING
BO has received a research grant from GE Healthcare. SB is supported by CIUSSS NIM and UQTR. JF is supported by CIHR (AFF_173514), NSERC (RGPIN-2022-04813), and FRQS/Parkinson Québec (Chercheur-boursier 283144). This work was partially supported by a grant from the Italian Ministry of Health to IRCCS Ospedale Policlinico San Martino (Fondi per la Ricerca Corrente, and Italian Neuroscience network (RIN)), by #NEXTGENERATIONEU (NGEU) and partially funded by the Ministry of University and Research (MUR), National Recovery and Resilience Plan (NRRP), project MNESYS (PE0000006) – A Multiscale integrated approach to the study of the nervous system in health and disease (DN. 1553 11.10.2022). This work was developed within the framework of the DINOGMI Department of Excellence of MIUR 2018-2022 (legge 232 del 2016).
CONFLICT OF INTEREST
JF received speaker fees from AromaInfo, Forst and Sabes, and royalties from StyriaBooks. DA received lecture fees from Idorsia, Bioprojet, Italfarmaco, Bruno, Valeas and Lundbeck.
REFERENCES
[1] | Postuma RB , Berg D , Stern M , Poewe W , Olanow CW , Oertel W , Obeso J , Marek K , Litvan I , Lang AE , Halliday G , Goetz CG , Gasser T , Dubois B , Chan P , Bloem BR , Adler CH , Deuschl G ((2015) ) MDS clinical diagnostic criteria for Parkinson’s disease. Mov Disord 30: , 1591–1601. |
[2] | Dickson DW ((2018) ) Neuropathology of Parkinson disease. Parkinsonism Relat Disord 46: , S30–S33. |
[3] | Pagano G , Boess FG , Taylor KI , Ricci B , Mollenhauer B , Poewe W , Boulay A , Anzures-Cabrera J , Vogt A , Marchesi M , Post A , Nikolcheva T , Kinney GG , Zago WM , Ness DK , Svoboda H , Britschgi M , Ostrowitzki S , Simuni T , Marek K , Koller M , Sevigny J , Doody R , Fontoura P , Umbricht D , Bonni A , PASADENA Investigatorsm Prasinezumab study Group ((2021) ) A phase II study to evaluate the safety and efficacy of prasinezumab in early Parkinson’s disease (PASADENA): Rationale, design, and baseline data. Front Neurol 12: , 705407. |
[4] | Lyu Z , Zheng S , Zhang X , Mai Y , Pan J , Hummel T , Hähner A , Zou L ((2021) ) Olfactory impairment as an early marker of Parkinson’s disease in REM sleep behaviour disorder: A systematic review and meta-analysis. J Neurol Neurosurg Psychiatry 92: , 271–281. |
[5] | Daalen JMJ , Tosserams A , Mahlknecht P , Seppi K , Bloem BR , Darweesh SKL ((2021) ) Towards subgroup-specific risk estimates: A meta-analysis of longitudinal studies on olfactory dysfunction and risk of Parkinson’s disease. Parkinsonism Relat Disord 84: , 155–163. |
[6] | Belvisi D , Pellicciari R , Fabbrini G , Tinazzi M , Berardelli A , Defazio G ((2020) ) Modifiable risk and protective factors in disease development, progression and clinical subtypes of Parkinson’s disease: What do prospective studies suggest? Neurobiol Dis 134: , 104671. |
[7] | Arnaldi D , Antelmi E , Louis EKS , Postuma RB , Arnulf I ((2017) ) Idiopathic REM sleep behavior disorder and neurodegenerative risk: To tell or not to tell to the patient? How to minimize the risk? Sleep Med Rev 36: , 82–95. |
[8] | Arnulf I ((2012) ) REM sleep behavior disorder: Motor manifestations and pathophysiology. Mov Disord 27: , 677–689. |
[9] | American Academy of Sleep Medicine ((2014) ) International classification of sleep disorders, American Academy of Sleep Medicine. |
[10] | Boeve BF , Molano JR , Ferman TJ , Lin S-C , Bieniek K , Tippmann-Peikert M , Boot B , St. Louis EK , Knopman DS , Petersen RC , Silber MH ((2013) ) Validation of the Mayo Sleep Questionnaire to screen for REM sleep behavior disorder in a community-based sample. J Clin Sleep Med 9: , 475–480. |
[11] | Frauscher B , Ehrmann L , Zamarian L , Auer F , Mitterling T , Gabelia D , Brandauer E , Delazer M , Poewe W , Högl B ((2012) ) Validation of the Innsbruck REM sleep behavior disorder inventory. Mov Disord 27: , 1673–1678. |
[12] | Videnovic A , Ju Y-ES , Arnulf I , Cochen-De Cock V , Högl B , Kunz D , Provini F , Ratti P-L , Schiess MC , Schenck CH , Trenkwalder C , on behalf of the Treatment and Trials Working Group of the International RBD Study Group ((2020) ) Clinical trials in REM sleep behavioural disorder: Challenges and opportunities. J Neurol Neurosurg Psychiatry 91: , 740–749. |
[13] | Postuma RB , Iranzo A , Hu M , Högl B , Boeve BF , Manni R , Oertel WH , Arnulf I , Ferini-Strambi L , Puligheddu M , Antelmi E , Cochen De Cock V , Arnaldi D , Mollenhauer B , Videnovic A , Sonka K , Jung KY , Kunz D , Dauvilliers Y , Provini F , Lewis SJ , Buskova J , Pavlova M , Heidbreder A , Montplaisir JY , Santamaria J , Barber TR , Stefani A , Louis SEK , Terzaghi M , Janzen A , Leu-Semenescu S , Plazzi G , Nobili F , Sixel-Doering F , Dusek P , Bes F , Cortelli P , Ehgoetz Martens K , Gagnon JF , Gaig C , Zucconi M , Trenkwalder C , Gan-Or Z , Lo C , Rolinski M , Mahlknecht P , Holzknecht E , Boeve AR , Teigen LN , Toscano G , Mayer G , Morbelli S , Dawson B , Pelletier A ((2019) ) Risk and predictors of dementia and parkinsonism in idiopathic REM sleep behaviour disorder: A multicentre study. Brain 142: , 744–759. |
[14] | Arnaldi D , Chincarini A , Hu MT , Sonka K , Boeve B , Miyamoto T , Puligheddu M , De Cock VC , Terzaghi M , Plazzi G , Tachibana N , Morbelli S , Rolinski M , Dusek P , Lowe V , Miyamoto M , Figorilli M , Verbizier D De , Bossert I , Antelmi E , Meli R , Barber TR , Trnka J , Miyagawa T , Serra A , Pizza F , Bauckneht M , Bradley KM , Zogala D , McGowan DR , Jordan L , Manni R , Nobili F ((2021) ) Dopaminergic imaging and clinical predictors for phenoconversion of REM sleep behaviour disorder. Brain 144: , 278–287. |
[15] | Zhang H , Iranzo A , Högl B , Arnulf I , Ferini-Strambi L , Manni R , Miyamoto T , Oertel WH , Dauvilliers Y , Ju YE , Puligheddu M , Sonka K , Pelletier A , Montplaisir JY , Stefani A , Ibrahim A , Frauscher B , Leu-Semenescu S , Zucconi M , Terzaghi M , Miyamoto M , Janzen A , Figorilli M , Fantini ML , Postuma RB ((2022) ) Risk factors for phenoconversion in rapid eye movement sleep behavior disorder. Ann Neurol 91: , 404–416. |
[16] | Antelmi E , Pizza F , Donadio V , Filardi M , Sosero YL , Incensi A , Vandi S , Moresco M , Ferri R , Marelli S , Ferini-Strambi L , Liguori R , Plazzi G ((2019) ) Biomarkers for REM sleep behavior disorder in idiopathic and narcoleptic patients. Ann Clin Transl Neurol 6: , 1872–1876. |
[17] | Iranzo A , Fairfoul G , Ayudhaya ACN , Serradell M , Gelpi E , Vilaseca I , Sanchez-Valle R , Gaig C , Santamaria J , Tolosa E , Riha RL , Green AJE ((2021) ) Detection of α-synuclein in CSF by RT-QuIC in patients with isolated rapid-eye-movement sleep behaviour disorder: A longitudinal observational study. Lancet Neurol 20: , 203–212. |
[18] | Siderowf A , Concha-Marambio L , Lafontant D-E , Farris CM , Ma Y , Urenia PA , Nguyen H , Alcalay RN , Chahine LM , Foroud T , Galasko D , Kieburtz K , Merchant K , Mollenhauer B , Poston KL , Seibyl J , Simuni T , Tanner CM , Weiub D , Videnovic A , Choi SH , Kurth R , Caspell-Garcia C , Coffey CS , Frasier M , Oliveira LM , Hutten SJ , Sherer T , Marek K , Soto C , on behalf of the Parkinson’s Progression Markers Initiative ((2023) ) Assessment of heterogeneity among participants in the Parkinson’s Progression Markers Initiative cohort using α-synuclein seed amplification: A cross-sectional study. Lancet Neurol 22: , 407–417. |
[19] | Poggiolini I , Gupta V , Lawton M , Lee S , El-Turabi A , Querejeta-Coma A , Trenkwalder C , Sixel-Döring F , Foubert-Samier A , Pavy-Le Traon A , Plazzi G , Biscarini F , Montplaisir J , Gagnon JF , Postuma R , Antelmi E , Meissner WG , Mollenhauer B , Ben-Shlomo Y , Hu MT , Parkkinen L ((2022) ) Diagnostic value of cerebrospinal fluid alpha-synuclein seed quantification in synucleinopathies. Brain 145: , 584–595. |
[20] | Doppler K ((2021) ) Detection of dermal alpha-synuclein deposits as a biomarker for Parkinson’s disease. J Parkinsons Dis 11: , 937–947. |
[21] | Concha-Marambio L , Weber S , Farris CM , Dakna M , Lang E , Wicke T , Ma Y , Starke M , Ebentheuer J , Sixel-Döring F , Muntean ML , Schade S , Trenkwalder C , Soto C , Mollenhauer B ((2023) ) Accurate detection of α-synuclein seeds in cerebrospinal fluid from isolated rapid eye movement sleep behavior disorder and patients with Parkinson’s disease in the DeNovo Parkinson (DeNoPa) Cohort. Mov Disord 38: , 567–578. |
[22] | Liguori R , Donadio V , Wang Z , Incensi A , Rizzo G , Antelmi E , Biscarini F , Pizza F , Zou W , Plazzi G ((2023) ) A comparative blind study between skin biopsy and seed amplification assay to disclose pathological α-synuclein in RBD. NPJ Parkinsons Dis 9: , 34. |
[23] | Iranzo A , Mammana A , Muñoz-Lopetegi A , Dellavalle S , Mayà G , Rossi M , Serradell M , Baiardi S , Arqueros A , Quadalti C , Perssinotti A , Ruggeri E , Cano JS , Gaig C , Parchi P ((2023) ) Misfolded α-synuclein assessment in the skin and CSF by RT-QuIC in isolated REM sleep behavior disorder. Neurology 100: , e1944–e1954. |
[24] | Lungu C , Cedarbaum JM , Dawson TM , Dorsey ER , Faraco C , Federoff HJ , Fiske B , Fox R , Goldfine AM , Kieburtz K , Macklin EA , Matthews H , Rafaloff G , Saunders-Pullman R , Schor NF , Schwarzschild MA , Sieber BA , Simuni T , Surmeier DJ , Tamiz A , Werner MH , Wright CB , Wyse R ((2021) ) Seeking progress in disease modification in Parkinson disease. Parkinsonism Relat Disord 90: , 134–141. |
[25] | Mari Z , Mestre TA ((2022) ) The disease modification conundrum in Parkinson’s disease: Failures and hopes. Front Aging Neurosci 14: , 810860. |
[26] | Arnaldi D , Famà F , Girtler N , Brugnolo A , Pardini M , Mattioli P , Meli R , Massa F , Orso B , Sormani MP , Donegani MI , Bauckneht M , Morbelli S , Nobili F ((2021) ) Rapid eye movement sleep behavior disorder: A proof-of-concept neuroprotection study for prodromal synucleinopathies. Eur J Neurol 28: , 1210–1217. |
[27] | Berg D , Postuma RB , Adler CH , Bloem BR , Chan P , Dubois B , Gasser T , Goetz CG , Halliday G , Joseph L , Lang AE , Liepelt-Scarfone I , Litvan I , Marek K , Obeso J , Oertel W , olanow w , Poewe W , Stern M , Deuschl G ((2015) ) MDS research criteria for prodromal Parkinson’s disease. Mov Disord 30: , 1600–1611. |
[28] | Höglinger GU , Adler CH , Berg D , Klein C , Outeiro TF , Poewe W , Postuma R , Stoessl AJ , Lang AE ((2024) ) A biological classification of Parkinson’s disease: The SynNeurGe research diagnostic criteria. Lancet Neurol 23: , 191–204. |
[29] | Simuni T , Chahine LM , Poston K , Brumm M , Buracchio T , Campbell M , Chowdhury S , Coffey C , Concha-Marambio L , Dam T , DiBiaso P , Foroud T , Frasier M , Gochanour C , Jennings D , Kieburtz K , Kopil CM , Merchant K , Mollenhauer B , Montine T , Nudelman K , Pagano G , Seibyl J , Sherer T , Singleton A , Stephenson D , Stern M , Soto C , Tanner CM , Tolosa E , Weintraub D , Xiao Y , Siderowf A , Dunn B , Marek K ((2024) ) A biological definition of neuronal α-synuclein disease: Towards an integrated staging system for research. Lancet Neurol 23: , 178–190. |
[30] | Iranzo A , Santamaría J , Valldeoriola F , Serradell M , Salamero M , Gaig C , Niñerola-Baizán A , Sánchez-Valle R , Lladó A , De Marzi R , Stefani A , Seppi K , Pavia J , Hogl B , Poewe W , Tolosa E , Lomena F ((2017) ) Dopamine transporter imaging deficit predicts early transition to synucleinopathy in idiopathic rapid eye movement sleep behavior disorder. Ann Neurol 82: , 419–428. |
[31] | Li Y , Kang W , Yang Q , Zhang L , Zhang L , Dong F , Chen S , Liu J ((2017) ) Predictive markers for early conversion of iRBD to neurodegenerative synucleinopathy diseases. Neurology 88: , 1493–1500. |
[32] | Iranzo A , Valldeoriola F , Lomeña F , Molinuevo JL , Serradell M , Salamero M , Cot A , Ros D , Pavía J , Santamaria J , Tolosa E ((2011) ) Serial dopamine transporter imaging of nigrostriatal function in patients with idiopathic rapid-eye-movement sleep behaviour disorder: A prospective study. Lancet Neurol 10: , 797–805. |
[33] | Joza S , Hu MT , Jung K , Kunz D , Arnaldi D , Lee J , Ferini-Strambi L , Antelmi E , Sixel-Döring F , De Cock VC , Montplaisir JY , Welch J , Kim HJ , Bes F , Mattioli P , Woo KA , Marelli S , Plazzi G , Mollenhauer B , Pelletier A , Razzaque J , Sunwoo JS , Girtler N , Trankwalder C , Gagnon JF , Postuma R , for the International REM Sleep Behavior Disorder Study Group ((2023) ) Prodromal dementia with Lewy bodies in REM sleep behavior disorder: A multicenter study. Alzheimers Dement 20: , 91–102. |
[34] | Fiamingo G , Capittini C , De Silvestri A , Rebuffi C , Cerami C , Arnaldi D , Terzaghi M ((2023) ) Neuropsychological evaluation of phenoconversion risk in REM sleep behaviour disorder: A scoping review. J Sleep Res 32: , e13873. |
[35] | Dušek P , Ibarburu VL y L , Bezdicek O , Dall’antonia I , Dostalova S , Kovalska P , Krupička R , Nepožitek J , Nikolai T , Novotný M , Perinova P , Rusz J , Serranova T , Tykalova TUlmanova O , Meckova Z , Ptacnik V , Trnka J , Zogala D , Ruzicka E , Sonka K ((2019) ) Relations of non-motor symptoms and dopamine transporter binding in REM sleep behavior disorder. Sci Rep 9: , 15463. |
[36] | Fereshtehnejad S-M , Yao C , Pelletier A , Montplaisir JY , Gagnon J-F , Postuma RB ((2019) ) Evolution of prodromal Parkinson’s disease and dementia with Lewy bodies: A prospective study. Brain 142: , 2051–2067. |
[37] | Miglis MG , Adler CH , Antelmi E , Arnaldi D , Baldelli L , Boeve BF , Cesari M , Dall’Antonia I , Diederich NJ , Doppler K , Dušek P , Ferri R , Gagnon JF , Gan-or Z , Hermann W , Högl B , Hu MT , Iranzo A , Janzen A , Kuzkina A , Lee JY , Leenders KL , Lewis SJGG , Liguori C , Liu J , Lo C , Ehgoetz Martens KA , Nepozitek J , Plazzi G , Provini F , Puligheddu M , Rolinski M , Rusz J , Stefani A , Summers RLSS , Yoo D , Zitser J , Oertel WH , Antonia ID , Diederich NJ , Doppler K , Dušek P , Ferri R , Gagnon JF , Gan-or Z , Hermann W , Högl B , Hu MT , Iranzo A , Janzen A , Kuzkina A , Lee JY , Leenders KL , Lewis SJGG , Liguori C , Liu J , Lo C , Martens KAE , Nepozitek J , Plazzi G , Provini F , Puligheddu M , Rolinski M , Rusz J , Stefani A , Summers RLSS , Yoo D , Zitser J ((2021) ) Biomarkers of conversion to α-synucleinopathy in isolated rapid-eye-movement sleep behaviour disorder. Lancet Neurol 20: , 671–684. |
[38] | Elliott JE , Lim MM , Keil AT , Postuma RB , Pelletier A , Gagnon J , St. Louis EK , Forsberg LK , Fields JA , Huddleston DE , Bliwise DL , Avidan AY , Howell MJ , Schenck CH , McLeland J , Criswell SR , Videnovic A , During EH , Miglis MG , Scprecher DR , Lee-Iannotti JK , Boeve BF , Ju YES , the North American Prodromal Synucleinopathy (NAPS) Consortium ((2023) ) Baseline characteristics of the North American prodromal Synucleinopathy cohort. Ann Clin Transl Neurol 10: , 520–535. |
[39] | Arnaldi D , Mattioli P , Pardini M , Morbelli S , Capriglia E , Rubino A , Rustioni V , Terzaghi M , Casaglia E , Serra A , Figorilli M , Liguori C , Fernandes M , Placidi F , Baldelli L , Provini F , Ferini-Strambi L , Marelli S , Plazzi G , Antelmi E , Brunetti V , Bonanni E , Puligheddu M , for the FARPRESTO Consortium ((2023) ) Clinical and dopaminergic imaging characteristics of the FARPRESTO cohort of trial-ready idiopathic rapid eye movement sleep behavior patients. Eur J Neurol 30: , 3703–3710. |
[40] | Puligheddu M , Figorilli M , Antelmi E , Arnaldi D , Casaglia E , d’Aloja E , Ferini-Strambi L , Ferri R , Gigli GL , Ingravallo F , Maestri M , Terzaghi M , Plazzi G , and the FARPRESTO Consortium ((2022) ) Predictive risk factors of phenoconversion in idiopathic REM sleep behavior disorder: The Italian study “FARPRESTO.”. Neurol Sci 43: , 6919–6928. |
[41] | Iranzo A , Stefani A , Serradell M , Martí MJ , Lomeña F , Mahlknecht P , Stockner H , Gaig C , Fernández-Arcos A , Poewe W , Tolosa E , Hogl B , Santamaria J , For the SINBAR (Sleep Innsbruck Barcelona) group ((2017) ) Characterization of patients with longstanding idiopathic REM sleep behavior disorder. Neurology 89: , 242–248. |
[42] | Maggi G , Vitale C , Cerciello F , Santangelo G ((2023) ) Sleep and wakefulness disturbances in Parkinson’s disease: A meta-analysis on prevalence and clinical aspects of REM sleep behavior disorder, excessive daytime sleepiness and insomnia. Sleep Med Rev 68: , 101759. |
[43] | Arnaldi D , Morbelli S , Brugnolo A , Girtler N , Picco A , Ferrara M , Accardo J , Buschiazzo A , de Carli F , Pagani M , Nobili F ((2016) ) Functional neuroimaging and clinical features of drug naive patients with de novo Parkinson’s disease and probable RBD. Parkinsonism Relat Disord 29: , 47–53. |
[44] | Arnaldi D , De Carli F , Famà F , Brugnolo A , Girtler N , Picco A , Pardini M , Accardo J , Proietti L , Massa F , Bauckneht M , Morbelli S , Sambuceti G , Nobili F ((2017) ) Prediction of cognitive worsening in de novo Parkinson’s disease: Clinical use of biomarkers. Mov Disord 32: , 1738–1747. |
[45] | Vendette M , Gagnon J-F , Decary A , Massicotte-Marquez J , Postuma RB , Doyon J , Panisset M , Montplaisir J ((2007) ) REM sleep behavior disorder predicts cognitive impairment in Parkinson disease without dementia. Neurology 69: , 1843–1849. |
[46] | Arnaldi D , Nobili F ((2018) ) The clinical relevance of cognitive impairment in REM sleep behavior disorder. Neurology 90: , 909–910. |
[47] | Jones MB , Jeevan S , Wang J , Li R , Agrawal R , Sharafkaneh A , Marsh L , Jorge RE ((2020) ) Clinical correlates of dream enactment behaviors in previously deployed OEF/OIF/OND veterans: An exploratory analysis. J Neuropsychiatry Clin Neurosci 32: , 147–153. |
[48] | Barone DA ((2020) ) Dream enactment behavior—a real nightmare: A review of post-traumatic stress disorder, REM sleep behavior disorder, and trauma-associated sleep disorder. J Clin Sleep Med 16: , 1943–1948. |
[49] | Lewis SJG ((2023) ) Same, same ... but different!. Brain 146: , 3568–3570. |
[50] | Fereshtehnejad S-M , Zeighami Y , Dagher A , Postuma RB ((2017) ) Clinical criteria for subtyping Parkinson’s disease: Biomarkers and longitudinal progression. Brain 140: , 1959–1976. |
[51] | Desiato VM , Levy DA , Byun YJ , Nguyen SA , Soler ZM , Schlosser RJ ((2021) ) The prevalence of olfactory dysfunction in the general population: A systematic review and meta-analysis. Am J Rhinol Allergy 35: , 195–205. |
[52] | Hummel T , Whitcroft KL , Andrews P , Altundag A , Cinghi C , Costanzo RM , Damm M , Frasnelli J , Gudziol H , Gupta N , Haehne A , Holbrook E , Hong SC , Hornung D , Huttenbrik KB , Kamel R , Kobayashi M , Konstamtimidis I , Landis BN , Leopold DA , Macchi A , Miwa T , Moesges R , Mullol J , Mueller CA , Ottaviano G , Passali GC , Philpott C , Pinto JM , Ramakrishnan VJ , Rombaux P , Roth Y , Schlosser RA , Shu B , Soler G , Stjarne P , Stuck BA , Vodicka J , Welge-Luessen A ((2017) ) Position paper on olfactory dysfunction. Rhinology 54: , 1–30. |
[53] | Croy I , Nordin S , Hummel T ((2014) ) Olfactory disorders and quality of life—an updated review. Chem Senses 39: , 185–194. |
[54] | Whitcroft KL , Altundag A , Balungwe P , Boscolo-Rizzo P , Douglas R , Enecilla MLB , Fjaeldstad AW , Fornazieri MA , Frasnelli J , Gane S , Gudziol H , Gupta N , Haehner A , Hernandez AK , Holbrook EH , Hopkins C , Hsieh JW , Huart C , Husain S , Kamel R , Kim JK , Kobayashi M , Konstantinidis I , Landis BN , Lechner M , Macchi A , Mazal PP , Miri I , Miwa T , Mori E , Mullol J , Mueller CA , Ottaviano G , Patel ZM , PhilpottPinto JM , Ramakrishnan VR , Roth Y , Schlossr RJ , Stjarne P , Van gerven L , Vodicka J , Welge-Luessen A , Wormald PJ , Hummel T ((2023) ) Position paper on olfactory dysfunction: 2023. Rhinology. |
[55] | Alonso CCG , Silva FG , Costa LOP , Freitas SMSF ((2021) ) Smell tests to distinguish Parkinson’s disease from other neurological disorders: A systematic review and meta-analysis. Expert Rev Neurother 21: , 365–379. |
[56] | Fullard ME , Morley JF , Duda JE ((2017) ) Olfactory dysfunction as an early biomarker in Parkinson’s disease. Neurosci Bull 33: , 515–525. |
[57] | Doty RL ((2012) ) Olfaction in Parkinson’s disease and related disorders. Neurobiol Dis 46: , 527–552. |
[58] | Haehner A , Boesveldt S , Berendse HW , Mackay-Sim A , Fleischmann J , Silburn PA , Johnston AN , Mellick GD , Herting B , Reichmann H , Hummel T ((2009) ) Prevalence of smell loss in Parkinson’s disease-a multicenter study. Parkinsonism Relat Disord 15: , 490–494. |
[59] | Landis BN , Burkhard PR ((2008) ) Phantosmias and Parkinson disease. Arch Neurol 65: , 1237–1239. |
[60] | Beach TG , Adler CH , Lue L , Sue LI , Bachalakuri J , Henry-Watson J , Sasse J , Boyer S , Shirohi S , Brooks R , Eschbacher J , White CL , Akiyama H , Caviness J , Shill HA , Connor DJ , Sabbagh MN , Walker DG , & the Arizona Parkinson’s Disease Consortium ((2009) ) Unified staging system for Lewy body disorders: Correlation with nigrostriatal degeneration, cognitive impairment and motor dysfunction. Acta Neuropathol 117: , 613–634. |
[61] | Braak H , Del Tredici K , Rüb U , De Vos RAI , Steur ENHJ , Braak E ((2003) ) Staging of brain pathology related to sporadic Parkinson’s disease. Neurobiol Aging 24: , 197–211. |
[62] | Braak H , Ghebremedhin E , Rüb U , Bratzke H , Del Tredici K ((2004) ) Stages in the development of Parkinson’s disease-related pathology. Cell Tissue Res 318: , 121–134. |
[63] | Arshamian A , Iravani B , Lundström JN ((2022) ) Is congenital anosmia protective for Parkinson’s disease triggered by pathogenic entrance through the nose? NPJ Parkinsons Dis 8: , 152. |
[64] | Chen H , Wang K , Scheperjans F , Killinger B ((2022) ) Environmental triggers of Parkinson’s disease-Implications of the Braak and dual-hit hypotheses. Neurobiol Dis 163: , 105601. |
[65] | Berg D , Adler CH , Bloem BR , Chan P , Gasser T , Goetz CG , Halliday G , Lang AE , Lewis S , Li Y , Liepelt-Scarfone I , Litvan I , Marek K , Maetzler C , Mi T , Obeso J , Oertel W , Olanow CW , Poewe W , Rios-Romenets S , Schaffer e , Seppi K , Heim B , Slow E , Stern M , Bledsoe IO , Deuschl G , Postuma RB ((2018) ) Movement disorder society criteria for clinically established early Parkinson’s disease. Mov Disord 33: , 1643–1646. |
[66] | Schmidt N , Paschen L , Witt K ((2020) ) Invalid self-assessment of olfactory functioning in Parkinson’s disease patients may mislead the neurologist. Parkinsons Dis 2020: , 7548394. |
[67] | Doty RL , Shaman P , Applebaum SL , Giberson R , Siksorski L , Rosenberg L ((1984) ) Smell identification ability: Changes with age. Science 226: , 1441–1443. |
[68] | Hummel T , Sekinger B , Wolf SR , Pauli E , Kobal G ((1997) ) “Sniffin” sticks’. Olfactory performance assessed by the combined testing of odor identification, odor discrimination and olfactory threshold. Chem Senses 22: , 39–52. |
[69] | Rahayel S , Frasnelli J , Joubert S ((2012) ) The effect of Alzheimer’s disease and Parkinson’s disease on olfaction: A meta-analysis. Behav Brain Res 231: , 60–74. |
[70] | Trentin S , Fraiman de Oliveira BS , Ferreira Felloni Borges Y , de Mello Rieder CR ((2022) ) Systematic review and meta-analysis of Sniffin Sticks Test performance in Parkinson’s disease patients in different countries. Eur Arch Oto-Rhino-Laryngology 279: , 1123–1145. |
[71] | Landolfi A , Picillo M , Pellecchia MT , Troisi J , Amboni M , Barone P , Erro R ((2023) ) Screening performances of an 8-item UPSIT Italian version in the diagnosis of Parkinson’s disease. Neurol Sci 44: , 889–895. |
[72] | Lo C , Arora S , Ben-Shlomo Y , Barber TR , Lawton M , Klein JC , Kanavou S , Janzen A , Sittig E , Oertel WH , grosset DG , Hu MT ((2021) ) Olfactory testing in Parkinson disease and REM behavior disorder: A machine learning approach. Neurology 96: , e2016–e2027. |
[73] | Aubry-Lafontaine E , Tremblay C , Durand-Martel P , Dupré N , Frasnelli J ((2020) ) Orthol, but not retronasal olfaction is specifically impaired in Parkinson’s disease. Chem Senses 45: , 401–406. |
[74] | Terrier L-M , Hadjikhani N , Destrieux C ((2022) ) The trigeminal pathways. J Neurol 269: , 3443–3460. |
[75] | Tremblay C , Frasnelli J ((2021) ) Olfactory-trigeminal interactions in patients with Parkinson’s disease. Chem Senses 46: , bjab018. |
[76] | Tremblay C , Martel PD , Frasnelli J ((2017) ) Trigeminal system in Parkinson’s disease: A potential avenue to detect Parkinson-specific olfactory dysfunction. Parkinsonism Relat Disord 44: , 85–90. |
[77] | Haehner A , Hummel T , Hummel C , Sommer U , Junghanns S , Reichmann H ((2007) ) Olfactory loss may be a first sign of idiopathic Parkinson’s disease. Mov Disord 22: , 839–842. |
[78] | Haehner A , Masala C , Walter S , Reichmann H , Hummel T ((2019) ) Incidence of Parkinson’s disease in a large patient cohort with idiopathic smell and taste loss. J Neurol 266: , 339–345. |
[79] | Sommer U , Hummel T , Cormann K , Mueller A , Frasnelli J , Kropp J , Reichmann H ((2004) ) Detection of presymptomatic Parkinson’s disease: Combining smell tests, transcranial sonography, and SPECT. Mov Disord 19: , 1196–1202. |
[80] | Vaswani PA , Morley JF , Jennings D , Siderowf A , Marek K , Seibyl J , Stern M , Russell D , Sethi K , Frank S , Simuni T , Hauser R , Ravina B , Richards I , Liang G , Adler C , Saunders-Pullman R , Evatt ML , Lai E , Subramanian I , Hogarth P , Chung K ((2022) ) Serial olfactory testing for the diagnosis of prodromal Parkinson’s disease in the PARS study. Parkinsonism Relat Disord 104: , 15–20. |
[81] | Mei J , Desrosiers C , Frasnelli J ((2021) ) Machine learning for the diagnosis of Parkinson’s disease: A review of literature. Front Aging Neurosci 13: , 633752. |
[82] | Goodwin GR , Bestwick JP , Noyce AJ ((2022) ) The potential utility of smell testing to screen for neurodegenerative disorders. Expert Rev Mol Diagn 22: , 139–148. |
[83] | Jennings D , Siderowf A , Stern M , Seibyl J , Eberly S , Oakes D , Marek K , for the PARS Investigators ((2017) ) Conversion to Parkinson disease in the PARS hyposmic and dopamine transporter-deficit prodromal cohort. JAMA Neurol 74: , 933–940. |