Action Observation and Motor Imagery as a Treatment in Patients with Parkinson’s Disease
Abstract
Action observation (AO) and motor imagery (MI) has emerged as promising tool for physiotherapy intervention in Parkinson’s disease (PD). This narrative review summarizes why, how, and when applying AO and MI training in individual with PD. We report the neural underpinning of AO and MI and their effects on motor learning. We examine the characteristics and the current evidence regarding the effectiveness of physiotherapy interventions and we provide suggestions about their implementation with technologies. Neurophysiological data suggest a substantial correct activation of brain networks underlying AO and MI in people with PD, although the occurrence of compensatory mechanisms has been documented. Regarding the efficacy of training, in general evidence indicates that both these techniques improve mobility and functional activities in PD. However, these findings should be interpreted with caution due to variety of the study designs, training characteristics, and the modalities in which AO and MI were applied. Finally, results on long-term effects are still uncertain. Several elements should be considered to optimize the use of AO and MI in clinical setting, such as the selection of the task, the imagery or the video perspectives, the modalities of training. However, a comprehensive individual assessment, including motor and cognitive abilities, is essential to select which between AO and MI suite the best to each PD patients. Much unrealized potential exists for the use AO and MI training to provide personalized intervention aimed at fostering motor learning in both the clinic and home setting.
INTRODUCTION
Parkinson’s disease (PD) is a neurodegenerative disorder characterized by motor and non-motor symptoms [1]. While there is no cure for PD, therapeutic interventions aim to alleviate symptoms through pharmacological therapy, surgical approaches (deep brain stimulation) and rehabilitative interventions [2]. Physiotherapy in PD encompasses several types of training, from aerobic exercises to motor-cognitive combined approaches, with the goal of improving motor function, activities of daily living and quality of life [3–5].
In recent years, there has been growing interest in the use of action observation (AO) and motor imagery (MI) as rehabilitative techniques in PD, owing their potential to engage and enhance, via motor learning mechanisms, motor circuits within the brain [6–9]. Both AO and MI involve the internal simulation of motor programs without physical movement execution and activate a wide network of brain regions, which partially overlap with those involved in actual motor execution [10, 11].
Usually, AO training (AOT) involves observing and imitating movements performed by others. This process is facilitated by specialized neurons in our brain called mirror neurons which fire both when we perform a particular action and when we observe someone else performing the same action. This phenomenon, known as “motor resonance”, facilitates the activation of motor pathways, promoting and potentially improving the acquisition of motor skills [12].
MI training (MIT) involves the mental rehearsal of movements or actions without overt motor output, followed by the physical execution of the imagined movement or action. This includes recalling the sensory consequences (visual or kinesthetic) of the action and envisioning the desired outcome of the imagined movement. By mentally rehearsing a motor skill, individuals can reinforce neural connections and enhance their ability to execute the same skill during physical performance [13].
In this paper we explore emerging knowledge in using AO and MI as physiotherapy techniques to enhance motor performance in individuals with PD. Firstly, we briefly summarize the underlying neural mechanisms of AO and MI in healthy individuals and in people with PD. Then, we revise evidence regarding the effects of AO and MI training on PD-related motor symptoms.
By understanding the current state of research and the implications of these techniques, we hope to contribute valuable insights to the field of physiotherapy for PD and pave the way for further advancements in this area of study.
AO AND MI NEUROPHYSIOLOGY IN HEALTHY PEOPLE
AO and MI are motor-cognitive mechanisms that share substrates with movement execution, relying on the process of movement preparation and involving the premotor cortex (ventral and dorsal), supplementary motor area (SMA), pre-SMA, inferior frontal gyrus (IFG), superior and inferior parietal lobule (SPL and IPL), intraparietal area, and the primary motor cortex (M1) [10, 11, 14, 15]. Recent fMRI studies in humans, have provided supportive evidence for the involvement of cortical-cerebellar and cortico-striatal (e.g., striatum, SNT) networks, like motor execution [16, 17]. This suggests the possible contribution of the cerebellum and basal ganglia to an extended mirror neuron system [11]. Conversely, the involvement of the primary motor cortex (M1) in AO and MI studies has been a subject of controversy, with inconsistent findings across neuroimaging studies. Methodological factors and task instructions may contribute to these discrepancies [10, 11, 18, 19].
However, the neural activation patterns associated with AO and MI differ, reflecting the different sources of information that each process relies on. AO is primarily driven by visual stimuli, such observing others’ behavior and is associated with activation in occipital regions [10]. On the other hand, MI relies on internally generated stimuli involving the reactivation of motor representations stored in memory. During MI, there is a decreased level of activation in sensory cortical regions, somatosensory cortex, SPL [13] and visual areas, which can be attributed to the absence of somatosensory input. The activation of visual cortical regions during MI is task-dependent, with the type of imagery (kinesthetic or visual) influencing the pattern of activation [11]. Furthermore, consistent recruitment of the dorsolateral prefrontal cortex (DLPFC) during MI has been linked to the frontal-executive functions (i.e., working memory), involved in motor preparation [10, 13]. Additionally, recent studies suggest that the retrieval of episodic (perceptual) memories aids image construction during MI [18] suggesting that also posterior (middle) areas of the cingulate, are recruited during imagery processes [20].
Finally, it has been shown that combining AO and MI lead to a greater activation of shared neural areas and enhances corticospinal excitability compared to using AO or MI alone [21, 22]. This may suggest the synergistic effects of integrating AO and MI in motor processes.
AO AND MI NEUROPHYSIOLOGY IN PARKINSON’S DISEASE
The Mirror Neuron System (MNS) is activated in people with PD, but with some differences with respect to healthy controls [23]. A pilot study [24] reported reduced μ-rhythm desynchronization in cortical areas near M1, compared to healthy controls. A previous study on AO in PD patients with freezing of gait (FoG) [25], revealed a reduced activity in the precentral and SMA areas, in comparison with controls. Impaired functionality of fronto-parietal areas during AO of gait has been also reported in individual with PD especially in those with FoG, with respect to control group [26]. During both a “FoG-observation-task” (consisting of watching a patient experiencing FoG during a walking task) and a “gait-observation-task” (consisting of watching a healthy subject performing similar walking tasks without experiencing FoG) PD-FoG patients showed reduced activity of the fronto-parietal MNS relative to controls [27]. In the “FoG-observation-task” relative to the “gait-observation-task”, PD-FoG patients revealed an increased recruitment of the anterior medial prefrontal cortex and a reduced recruitment of the dorsomedial prefrontal cortex and hippocampus relative to controls. Unfortunately, so far evidence on neurophysiological substrate of AO in PD is scarce and limited particularly to patients in a more advanced stage (with FOG). Further research is needed to better understand MNS network function in PD.
The accessibility of MI in subject with PD is also a topic of ongoing discussion. Brain activation patterns during MI may differ in individual with PD [28–30]. Peterson and co-workers [31] showed that, in the OFF-medication state, individuals with PD were able to imagine walking but with different patterns of brain activation compared to healthy controls. They exhibit reduced activity in globus pallidus and increased activity in the SMA not only during imaged gait but also in complex tasks, such as imaged turning [31] and imaged backward walking [32]. Neuroimaging studies also suggest that PD subjects with freezing of gait (FOG) exhibit greater activity in the mesencephalic locomotor region and decreased activation of SMA and parietal areas during imagined walking compared to those without FOG. Interestingly, this hyperactivity in MLR correlates with severity and duration of FOG [33]. A more recent fMRI study [34], during an imaged turning task, found a higher activation of the superior occipital gyrus, left precentral gyrus, and right postcentral gyrus in freezers compared to non-freezers indicating the compensatory roles of visual information in imagery process. EEG studies have revealed that a 10-minute session of kinesthetic imagery enhances the activity of prefrontal cortex including DLPFC [35].
AO AND MI TRAINING: GENERAL CHARACTERISTICS
Several characteristics should be considered when AOT and MIT are applied:
– First-Person (1stP) vs. Third-Person (3rdP) Perspective: AOT and MIT can be performed from the perspective of the person performing the action (1stP) or from an external observer’s perspective (3rdP). Studies in healthy subjects, results suggest that AO and MI delivered in 1stP perspective elicit greater activation of the MNS [36] and more embodied sensorimotor activity [13] compared to 3rdP perspective. The effectiveness of these perspectives for motor learning is still under investigation.
– Motor Task Complexity: The complexity of the motor task being observed or imagined can influence the effectiveness of AOT and MIT. Observing or imagine more complex motor tasks may take longer and deteriorate imitation abilities after AO or the quality of MI. In some cases, a 3rdP perspective both for AO and MI might be more beneficial for fostering motor learning [6, 37].
– Familiarity of Movement: The familiarity of the movement being observed or imagined plays a role in the effectiveness of AOT and MIT. Individuals are likely to perform better mentally and physically if they have prior experience with the task [38]. The activation of the MNS is also greater when the observed actions are part of the individual’s motor repertoire [39].
– Goal-Directed Transitive Actions: Actions involving interaction with objects and having a clear goal are associated with greater activation of the MNS [40] compared to movements out of context [41]. This is because goal-directed movements are abstractly encoded based on their goal, regardless of the effector used to accomplish them [42].
– Consecutive vs Concurrent modalities. AOT and MIT can be applied consecutively, where the individual observes the action and then performs it, or concurrently [43, 44], where they observe the action while simultaneously performing it [43, 44].
– Duration: It is important to notice that AOT and MIT necessitate distinct durations. To uphold a heightened focus during AOT, it is suggested that each video does not exceed a duration of 1.5 minutes. Moreover, the overall session duration for AOT should ideally range from a minimum of 30 minutes to a maximum of one hour. Conversely, MIT sessions may have a shorter duration than AOT, ranging from a minimum of 15 minutes to 40 minutes. The shorter duration is attributed to the increased cognitive load associated with MIT.
– In consideration of different patient profiles, including executive and attentive abilities, it is plausible to hypothesize a training regimen involving progressively challenging AO and MI exercises.
Taking these different elements into consideration can help optimize the application of AOT and MIT in motor learning and rehabilitation programs for people with PD.
MI ASSESSMENT
To date, various methods are employed for clinically testing MI ability (for review see, Di Rienzo, (2014) [29, 45] and Malouin (2013) [29, 45]. These include:
– Standardized questionnaires: these assess the MI “vividness” and “ease”. Participants provide scores on a 5 or 7-level Likert scale.
– Logbooks: used to collect vividness, ease and accuracy of MI
– Tests (e.g., “congruency” and “mental rotation”): these typically address the accuracy of imagery
– Mental chronometry: involves recording the duration of MI. It allows the measurement of temporal correspondence between imagery and physical execution time. The MI accuracy is often measured as “temporal equivalence” and “isochrony”.
– Oral debriefs: these are used to check adherence to MI instructions, although the interview procedures have been poorly described.
AO AND MI TRAINING IN PARKINSON’S DISEASE
WHY do we apply AOT and MIT in PD?
The use of AO and motor imagery MI training in PD is driven by two main reasons.
First, there is substantial evidence from studies in healthy individuals suggesting that both AO and MI can induce motor learning similar to movement execution. Motor learning involves repeated interactions with the environment to change motor behavior [46, 47], and this process can be accomplished by repeatedly observing an action [48–51] or mentally imagining it [52, 53]. Accordingly, neuroplasticity in the sensorimotor network, a major neurobiological substrate of behavioural learning outcomes [54] can be induced by both action observation [55, 56] and motor imagery training [50, 57].
Secondly, in individuals with PD, MI ability and MNS seem to be relatively preserved. Indeed, in early-stage PD, the vividness and accuracy of MI appear to be maintained in both simple [58] and complex task [59]. Regarding isochrony, it has been reported that, like motor execution, individuals with PD imagine movements at a slower pace than healthy controls [58, 60–63], but this difference can be reduced through external cueing in imagery [61] and potentially, through using AO [64]. A substantially preserved ability for MI, which can also be boosted, makes MIT a promising tool for physiotherapy in PD.
Related to MNS, some studies suggest that the MNS may be preserved in PD. Investigations into subthalamic nucleus activity, which plays a role in modulating basal ganglia output and motor/non-motor information sharing across the cortico-basal ganglia-thalamo-cortical loop, have shown significant modulation in beta activity during deep brain stimulation (DBS) in individuals with PD, particularly in the “on” medication state [65, 66]. Additionally, studies have indicated that voluntary movement imitation and imitative priming do not differ between individuals with PD and controls, further supporting the preservation of the MNS in PD [67, 68].
HOW do we apply AOT and MIT in PD?
In this section, we first summarize available evidence on the effectiveness of AOT and MIT in PD. Then, starting from this body of evidence we revise training modalities used in individual with PD.
Action observation training
Recent research has highlighted the applicability and efficacy of AOT. AOT has been shown to improve motor and functional outcomes [6, 7, 69–71]. Effectiveness on outcome measure was studied both in the short and in the long term (1 to 3 months).
Precisely, AOT has demonstrated effects on static and dynamic balance, with improvement on Berg balance and Tinetti scales scores, [25, 72–75] and gait speed [76]. Moreover, signs of efficacy have been reported on functional mobility, endurance, and ADL, tested by means of Timed Up and Go test (TUG) 6 Minutes Walking Test [74], 10-meter walking test [73], and Functional Independence Measure (FIM) [77]. In addition, AOT have been shown to reduce FOG severity, evaluated with Freezing of Gait Questionnaires [25, 72–74], and episodes, assessed with Freezing of Gait Diary [72].
Regarding upper limb bradykinesia, AOT was demonstrated to increase movement rate during self-paced finger opposition movements [78] and hand dexterity, tested with the nine-hole peg test [75].
Finally, some studies also reveal a global beneficial effect of AOT with significant improvements on quality of life [25, 72], and subjective-reported mobility (tested with PDQ-39, mobility dimension score) [76].
Noteworthy long term additional benefits on disease severity (UPDRS-II and UPDRS-III), biomechanics of ecological task (Center of Pressure COP, and moving timings) and quality of life (PDQ-39) were found when the intervention was associated with ecological auditory cues [74].
Motor imagery training
The number of trials that specifically evaluate the effects of motor training based on MI in clinical practice is limited with marked differences in research protocol, training regimen, outcome measures and a small sample size. Plus, results are contradictory.
Positive studies showed that training based on motor imagery improve mobility, as evidenced by a reduction in the mean TUG score [79], balance Tinetti [80], push and release test, turning 360° [79], and daily activities, measured with Impact on Participation and Autonomy Scale Questionnaire [79]. Moreover, sign of efficacy was seen after MIT training on disease severity [79–81] and bradykinesia measured with a Standing up and lying down test [81]. Only a case-report study showed improvement of pain (assessed by Visual analogic scale-VAS) [80] and only one RCT tested and revealed an improvement in participants’ MI ability (KVIQ) after training [79]. Two RCT studies reported an improvement in participant’s body schema [82] and in visuospatial working memory [79]. Effectiveness on outcome measure was studied both in the short (immediately after the training [79–81] and in the long term (12-week follow-up) [80].
Conversely, two studies suggested that MIT may not confer benefits. The first study by Braun et al. [83] found no difference between MIT and a relaxation protocol on mobility (TUG) and walking (10 M Walk test) parameters, following kinesthetic and visual MIT, despite observing a larger effects of MI training in sub-groups of less impaired patients. Similarly, the second study, a randomized controlled trial by Santiago et al. [84], reported no benefits of MIT added to physical practice in terms of mobility (evaluated by means of TUG) and walking, assessed by means of Functional Gait assessment (FGA). Moreover, kinematic parameters of stride length, stance and swing time, hip range of motion were collected. However, it’s worth noting that these results refer to a single session of a multi-level protocol of MIT [84].
Training modalities used in individual with PD
Applying AO or MI in a physiotherapy protocol in PD involves specific considerations to optimize its effectiveness.
Action observation training
– Perspective: While the 1st person perspective has shown potential benefits for balance and mobility in PD [75], most studies have utilized the 3rd person perspective as it allows capturing all elements of a motor task more easily [25, 72–74, 76–78].
– Familiarity of movements and tasks complexity: The selection of observed acts in AOT is crucial, and they should be linked to the target symptom and relevant to the individual’s motor repertoire. Different types of movements have been used for specific goals. The observation of strategies to circumvent FOG episodes, such as shifting weight, wide turning, stepping over obstacles have been applied in FOG-focused AOT [25, 72–74]. For gait improvement, videos of usual and dual task walking or different functional daily actions have been proposed [75–77]. For improving upper limb function, videos of repetitive finger movements (intransitive movements) [78] or task-oriented actions (transitive movements) have been used [75]. Videos performed by healthy actors were commonly used, except for one study [76] that used gait videos of both healthy and individuals with PD.
– Finally, for increasing participants attention multisensory stimuli, such as action-related “sonified” sounds or auditory cues, were incorporated in the video-clip [25, 74].
– Consecutive vs Concomitant: In general, the observation of the videos is proposed with an “attentional” modality (i.e., active watching) compared to “passive” modality (i.e., watching without further instructions) [72–74, 78, 85]. Regarding consecutive or concomitant modalities, all the studies used the consecutive modality, except for one [74] when AO was combined with movement execution.
– Time, Dosage, Setting: Common session frequency is 2 to 3 times a week for 4 to 8 weeks. Videos last between 40 seconds to 6 minutes, and training session 45–60 minutes, with approximately 25 minutes dedicated to AO [25, 72, 73, 75–78]. Except for one study that administered AOT in a group setting with multiple participants [73], most of the studies used a one-to-one (physiotherapist-patient) training modality [70]. 7 studies were performed in clinical setting or research laboratories, while only 1 was accomplished in a home-based setting [76].
Motor imagery training
– Perspective: Four studies required to imagine both 1stP and 3rdP perspectives [79, 81–83]. Others used only 1stP perspective [80, 84]. Abraham and colleagues [79, 82] employed the Dynamic Neuro-Cognitive Imagery (DNI) method, that incorporate different MI categories (e.g., emotional, metaphorical) and mental-imagery-related assistive tools (i.e., self-talk and self-touch).
– Familiarity of movements and motor Tasks: To enhance MI performance, the familiarity of the movement should be considered. Functional tasks such as gait initiation, sitting down, standing up, walking, and turning in various contexts have been proposed [79–84].
– Consecutive vs Concomitant: The current MIT approach focuses on alternated single mental and physical repetitions of the proposed tasks to exploit the priming effects of MI on physical performance [86] and reduce the number of physical repetitions [87]. Only in one study a series of mental repetitions was required before executing the imagined actions [84]. Regarding consecutive or concomitant modalities, all the studies used the consecutive modality, except for two [79, 82] when MI was combined with movement execution.
– Time, Dosage, Setting: Studies showed effectiveness of MIT vary in training duration ranging from 2 weeks to 12 weeks with session frequency from 1 time to 5 times a week Training duration was about from 20 minutes to 2 hour [79–82]. The modality of MIT training also differs among studies. Three of the studies used a one-to-one modality [80, 83, 84], whereas the other three studies used a group setting, where multiple participants underwent the MIT intervention together [79, 81, 82].
Finally, it is noteworthy that before implementing AO or MI in a physiotherapy protocol for PD, a comprehensive individual assessment should be conducted. This assessment should include evaluating the patient’s motor impairments, disease stage, cognitive abilities, and medication states. Understanding the specific needs and abilities of each patient will help tailor the intervention accordingly.
WHEN do we apply AOT and MIT in PD?
In this section, we summarize from the available evidence the characteristics of individual with PD which may benefit from AOT and MIT.
Action observation training
Common characteristics of the patients included in AOT studies can be extracted [6, 7, 69, 71].
These studies typically recruited patients with a Hoehn and Yahr (H&Y) stage ranging from 1 to 3, indicating a moderate level of motor impairment. The disease duration was at least 5 years. All participants were on a stable dopaminergic medication regimen for a minimum of 4 weeks prior the intervention. AOT was proposed during their ON phase, and no patients with DBS were enrolled in any study. Moreover, studies investigating the effectiveness of AOT on FOG, recruited participants who experienced freezing episodes at least once a week, lasting for at least 2 seconds [25, 72, 73]
In addition to motor impairments, another essential inclusion criterion common to all the studies was the absence of severe cognitive deficits (MMSE > 25). This ensured that participants could effectively engage in the AOT interventions.
Motor imagery training
MI is an explicit learning requiring cognitive load (i.e., working memory) and may induce mental fatigue [13, 19]. PD patients may exhibit cognitive decline in attention, executive function, short-term working memory, and visuospatial memory, which varies depending on the stage and time of disease onset [88], potentially reducing MIT effectiveness [29, 89]. Therefore, a screening for cognitive impairment and MI ability should be mandatory prior to start MIT training [45].
In studies applied MIT, most of the participants had no severe cognitive deficits (MMSE score range 26–30; MoCA 23–29) [79–84] and normal visuo-spatial memory and attention [79, 81]. The H&Y stage range between 2 and 3 and a disease duration ranging from 4 to 12 years [79–82, 84]. Only in one study [83] enrolled patients were in H&Y stage from 1 to 4 with a disease duration from 4 to 6 years. MIT was always proposed during ON condition, and no patients with DBS or FOG were enrolled in any study.
Two studies [79, 84] assessed MI ability by means of the VMIQ-2; KVIQ-20 and MIQ-RS score, and one study [66] encountered MIQ-R –Kinesthetic score. In only two studies mood severity was assessed and patients reported a mild depressive status [79, 82].
Combined AO and MI
Recent physiotherapy protocols have explored the combined use of AO and MI techniques, where participants observe an action and simultaneously imagine executing it, based on promising neuro physiological findings associated with their simultaneous application [21, 22]. A pilot study combining AO-MI, followed by physical execution, demonstrated preliminary evidence of effectiveness in improving dexterity and timed action performance in participants with PD [90]. Furthermore, the AO-MI technique combined with dual-task gait and balance training resulted in specific functional brain reorganization in motor control and executive-attentive areas, leading to lasting improvements in dual-task, mobility and balance [85]. However, in a different study by Bezerra et al. [91], no significant improvement in balance or FOG was observed when AO-MI was combined with gait training.
Despite these mixed results, there is still potential for combining AO and MI with gait training to improve motor function in PD, but further research is needed to confirm and better understand its effectiveness.
FUTURE PERSPECTIVE
Both AO and MI techniques have the potential to be administered to patients at home or through telemedicine, making them easily accessible and feasible for remote interventions.
In addition to their standalone application, these techniques could be combined with noninvasive neuromodulation techniques to foster neuroplasticity processes and improve their effectiveness in rehabilitation programs. Transcranial direct current stimulation (tDCS), has been explored in combination with MI. A pilot study [92] combining tDCS with MI in healthy subjects showed short-term effects on postural control, suggesting its potential use in physiotherapy programs. Moreover, a recent study [93] using high-definition tDCS (HD-tDCS) targeting the inferior parietal lobe (IPL) and inferior frontal gyrus (IFG), combined with AO, demonstrated improved reaching time in healthy subjects compared to sham stimulation.
Take home messages |
∘ AO and MI training are promising tools for physiotherapy in individuals with PD. |
∘ Neurophysiological evidence supports that brain networks involved in AO and MI processes are relatively preserved in PD. |
∘ Both AO and MI have been shown to improve motor performance and activities of daily living. |
∘ Cognitive abilities should be assessed to ensure that the individuals are suitable candidates for MI training. |
∘ New technologies can be incorporated to boost the effectiveness of AO and MI training. |
∘ Future research is needed to establish consensus on optimal treatment parameters and training modalities. |
Fig. 1
A) Depiction of AO and MI networks in human brain. AO task predominantly activates premotor and motor regions (Premotor cortex (PMC), supplementary motor area (SMA), pre-SMA and M1 (green)), parietal areas, (parietal lobule (PL) and intraparietal areas (purple)) and visual areas (red). MI task activates regions including PMC, SMA, pre-SMA and M1 (green), PL and intraparietal area (purple). Visual imagery shows activity in visual areas (red) and kinesthetic MI in the primary somatosensory area (orange). Additionally, the dorsolateral prefrontal cortex (DLPFC) is consistently recruited during Motor Imagery task (yellow). Both cerebellum (light blue) and basal ganglia (blue) are activated during AO and MI. B) Cartoon of consecutive and concurrent training modalities.
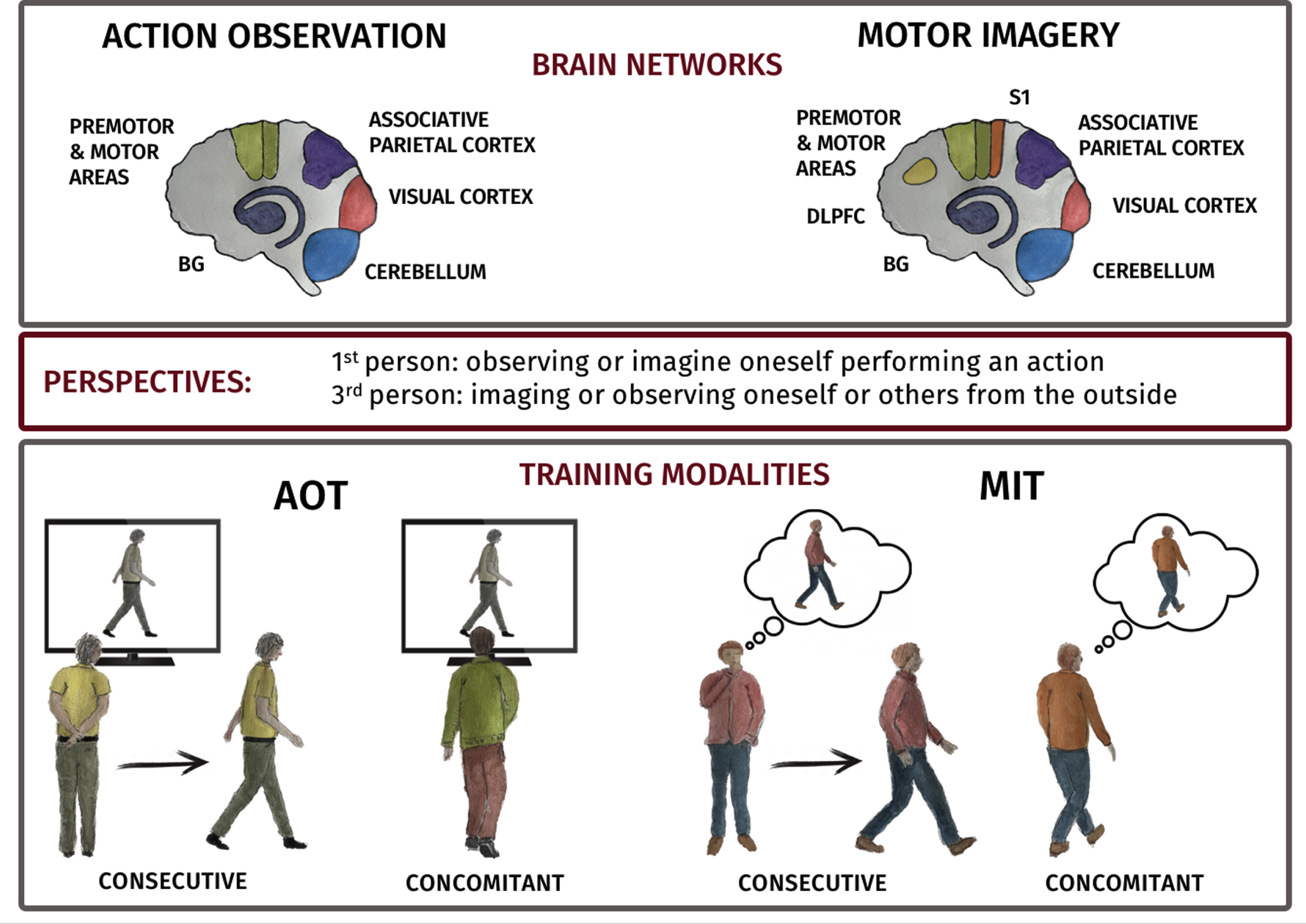
Another promising approach is the use of neuro feedback, a technique that provides real-time feedback from EEG signals to allow individuals to regulate their own brain activity. An fMRI study [94] employing neuro feedback-guided motor imagery in individual with PD showed an improved self-perceived physical activities level after MI training. However more recent trials [95, 96] did not find additional benefit from adding neuro feedback to MIT in individual with PD in the mid-stage of the disease. Potential barriers to successful neuro feedback regulation may include mental fatigue, distraction, and frustrated efforts during motor imagery [95]. These challenges should be carefully considered when incorporating neuro feedback into rehabilitation programs.
Finally, mild cognitive decline should not be perceived as a constraint to treatment but as a viable target for intervention, as suggested by recent evidence [97, 98]. Future studies should consider testing AOT or MIT in PD-MCI patients to assess its impact on cognitive dysfunctions.
CONCLUSION
Based on the available evidence, both AO and MI training shown promising results in improving motor and functional outcomes in individuals with PD. Evidence are strongest for the effectiveness of AOT, whereas due to the exiguous number of studies and conflicting results the effectiveness of MI is more uncertain. To optimize the effectiveness of AOT and MIT in PD, several features (e.g., perspective, tasks complexity, training modalities) should be taken into account. Tailoring the interventions to the individual’s needs and abilities can enhance their benefits. However, more research is needed to establish consensus on optimal treatment parameters and training regimens. Additionally, larger sample sizes and careful consideration of disease stage, cognitive abilities, and medication states are essential for future studies to provide a more comprehensive understanding of the mechanisms underlying AO and MI in PD.
ACKNOWLEDGMENTS
The authors have no acknowledgments to report.
FUNDING
This work was carried out within the framework of the project “RAISE –Robotics and AI for Socio-economic Empowerment” and has been supported by European Union –Next Generation EU.
CONFLICT OF INTEREST
The authors have no conflict of interest to report.
DATA AVAILABILITY
Data sharing is not applicable to this article as no datasets were generated or analyzed during this study.
REFERENCES
[1] | Bloem BR , Okun MS , Klein C ((2021) ) Parkinson’s disease. Lancet 397: , 2284–2303. |
[2] | Church FC ((2021) ) Review treatment options for motor and non-motor symptoms of Parkinson’s disease. Biomolecules 11: , 612. |
[3] | Cosentino C , Baccini M , Putzolu M , Ristori D , Avanzino L , Pelosin E ((2020) ) Effectiveness of physiotherapy on freezing of gait in parkinson’s disease: A systematic review and meta-analyses. Mov Disord 35: , 523–536. |
[4] | Johansson H , Folkerts AK , Hammarström I , Kalbe E , Leavy B ((2023) ) Effects of motor-cognitive training on dual-task performance in people with Parkinson’s disease: A systematic review and meta-analysis. J Neurol 270: , 2890–2907. |
[5] | Ernst M , Folkerts AK , Gollan R , Lieker E , Caro-Valenzuela J , Adams A , Cryns N , Monsef I , Dresen A , Roheger M , Eggers C , Skoetz N , Kalbe E ((2023) ) Physical exercise for people with Parkinson’s disease: A systematic review and network meta-analysis. Cochrane Database Syst Rev 1: , CD013856. |
[6] | Giannakopoulos I , Karanika P , Papaxanthis C , Tsaklis P ((2022) ) The effects of action observation therapy as a rehabilitation tool in Parkinson’s disease patients: A systematic review. Int J Environ Res Public Health Res Public Health 19: , 3311. |
[7] | Lahuerta-Martín S , Llamas-Ramos R , Llamas-Ramos I ((2022) ) Effectiveness of therapies based on mirror neuron system to treat gait in patients with Parkinson’s disease—a systematic review. J Clin Med 11: , 4236. |
[8] | Abraham A , Duncan RP , Earhart GM ((2021) ) The role of mental imagery in Parkinson’s disease rehabilitation. Brain Sci 11: , 185. |
[9] | Abbruzzese G , Avanzino L , Marchese R , Pelosin E ((2015) ) Action observation and motor imagery: Innovative cognitive tools in the rehabilitation of Parkinson’s disease. Parkinsons Dis 2015: , 124214. |
[10] | Hardwick RM , Caspers S , Eickhoff SB , Swinnen SP ((2018) ) Neural correlates of action: Comparing meta-analyses of imagery, observation, and execution. Neurosci Biobehav Rev 94: , 31–44. |
[11] | Henschke JU , Pakan JMP ((2023) ) Engaging distributed cortical and cerebellar networks through motor execution, observation, and imagery. Front Syst Neurosci 17: , 1165307. |
[12] | Uithol S , van Rooij I , Bekkering H , Haselager P ((2011) ) Understanding motor resonance. Soc Neurosci 6: , 388–397. |
[13] | Ladda AM , Lebon F , Lotze M ((2021) ) Using motor imagery practice for improving motor performance –a review. Brain Cogn 150: , 105705. |
[14] | Ge S , Wang P , Liu H , Lin P , Gao J , Wang R , Iramina K , Zhang Q , Zheng W ((2019) ) Neural activity and decoding of action observation using combined EEG and fNIRS measurement. Front Hum Neurosci 13: , 357. |
[15] | Su WC , Dashtestani H , Miguel HO , Condy E , Buckley A , Park S , Perreault JB , Nguyen T , Zeytinoglu S , Millerhagen J , Fox N , Gandjbakhche A ((2023) ) Simultaneous multimodal fNIRS-EEG recordings reveal new insights in neural activity during motor execution, observation, and imagery. Sci Rep 13: , 5151. |
[16] | Errante A , Fogassi L ((2020) ) Activation of cerebellum and basal ganglia during the observation and execution of manipulative actions. Sci Rep 10: , 12008. |
[17] | Errante A , Gerbella M , Mingolla GP , Fogassi L ((2023) ) Activation of cerebellum, basal ganglia and thalamus during observation and execution of mouth, hand, and foot actions. Brain Topogr 36: , 476–499. |
[18] | Hetu S , Gregoire M , Saimpont A , Coll MP , Eugène F , Michon PE , Jackson PL ((2013) ) The neural network of motor imagery: An ALE meta-analysis. Neurosci Biobehav Rev 37: , 930–949. |
[19] | Cuomo G , Maglianella V , Ghanbari Ghooshchy S , Zoccolotti P , Martelli M , Paolucci S , Morone G , Iosa M ((2022) ) Motor imagery and gait control in Parkinson’s disease: Techniques and new perspectives in neurorehabilitation. Expert Rev Neurother 22: , 43–51. |
[20] | Jessey TB , Lin B , Subramanium SV , Kraeutner SN ((2023) ) Disrupting somatosensory processing impairs motor execution but not motor imagery. Hum Mov Sci 90: , 103101. |
[21] | Sakamoto M , Muraoka T , Mizuguchi N , Kanosue K ((2009) ) Combining observation and imagery of an action enhances human corticospinal excitability. Neurosci Res 65: , 23–27. |
[22] | Kaneko N , Masugi Y , Yokoyama H , Nakazawa K ((2018) ) Difference in phase modulation of corticospinal excitability during the observation of the action of walking, with and without motor imagery. Neuroreport 29: , 169–173. |
[23] | Farina E , Borgnis F , Pozzo T ((2020) ) Mirror neurons and their relationship with neurodegenerative disorders. J Neurosci Res 98: , 1070–1094. |
[24] | Heida T , Poppe NR , de Vos CC , van Putten MJ , van Vugt JP ((2014) ) Event-related mu-rhythm desynchronization during movement observation is impaired in Parkinson’s disease. Clin Neurophysiol 125: , 1819–1825. |
[25] | Agosta F , Gatti R , Sarasso E , Volonte MA , Canu E , Meani A , Sarro L , Copetti M , Cattrysse E , Kerckhofs E , Comi G , Falini A , Filippi M ((2017) ) Brain plasticity in Parkinson’s disease with freezing of gait induced by action observation training. J Neurol 264: , 88–101. |
[26] | Bommarito G , Putzolu M , Avanzino L , Cosentino C , Botta A , Marchese R , Inglese M , Pelosin E ((2020) ) Functional correlates of action observation of gait in patients with Parkinson’s disease. Neural Plast 2020: , 8869201. |
[27] | Sarasso E , Agosta F , Piramide N , Canu E , Volontè MA , Filippi M ((2021) ) Brain activity of the emotional circuit in Parkinson’s disease patients with freezing of gait. Neuroimage Clin 30: , 102649. |
[28] | Cunnington R , Egan GF , O’Sullivan JD , Hughes AJ , Bradshaw JL , Colebatch JG ((2001) ) Motor imagery in Parkinson’s disease: A PET study. Mov Disord 16: , 849–857. |
[29] | Di Rienzo F , Collet C , Hoyek N , Guillot A ((2014) ) Impact of neurologic deficits on motor imagery: A systematic review of clinical evaluations. Neuropsychol Rev 24: , 116–147. |
[30] | Helmich RC , de Lange FP , Bloem BR , Toni I ((2007) ) Cerebral compensation during motor imagery in Parkinson’s disease. Neuropsychologia 45: , 2201–2215. |
[31] | Peterson DS , Pickett KA , Duncan RP , Perlmutter JS , Earhart GM ((2014) ) Brain activity during complex imagined gait tasks in Parkinson disease. Clin Neurophysiol 125: , 995–1005. |
[32] | Myers PS , McNeely ME , Pickett KA , Duncan RP , Earhart GM ((2018) ) Effects of exercise on gait and motor imagery in people with Parkinson disease and freezing of gait. Parkinsonism Relat Disord 53: , 89–95. |
[33] | Snijders AH , Leunissen I , Bakker M , Overeem S , Helmich RC , Bloem BR , Toni I ((2011) ) Gait-related cerebral alterations in patients with Parkinson’s disease with freezing of gait. Brain 134: , 59–72. |
[34] | Huang HC , Chen CM , Lu MK , Liu BL , Li CI , Chen JC , Wang GJ , Lin HC , Duann JR , Tsai CH ((2021) ) Gait-related brain activation during motor imagery of complex and simple ambulation in Parkinson’s disease with freezing of gait. Front Aging Neurosci 13: , 731332. |
[35] | Lim VK , Polych MA , Holländer A , Byblow WD , Kirk IJ , Hamm JP ((2006) ) Kinesthetic but not visual imagery assists in normalizing the CNV in Parkinson’s disease. Clin Neurophysiol 117: , 2308–2314. |
[36] | Ge S , Liu H , Lin P , Gao J , Xiao C , Li Z ((2018) ) Neural basis of action observation and understanding from first- and third-person perspectives: An fMRI study. Front Behav Neurosci 12: , 283. |
[37] | Gäumann S , Gerber RS , Suica Z , Wandel J , Schuster-Amft C ((2021) ) A different point of view: The evaluation of motor imagery perspectives in patients with sensorimotor impairments in a longitudinal study. BMC Neurol 21: , 297. |
[38] | Guillot A , Collet C ((2005) ) Duration of mentally simulated movement: A review. J Mot Behav 37: , 10–20. |
[39] | Calvo-Merino B , Grèzes J , Glaser DE , Passingham RE , Haggard P ((2006) ) Seeing or doing? Influence of visual and motor familiarity in action observation.. Curr Biol 16: , 1905–1910. |
[40] | Pfenninger C , Grosprêtre S , Remontet A , Lapole T ((2021) ) Comparison of the on-line effects of different motor simulationconditions on corticospinal excitability in healthy participants. Sci Rep 11: , 13176. |
[41] | Enticott PG , Kennedy HA , Bradshaw JL , Rinehart NJ , Fitzgerald PB ((2010) ) Understanding mirror neurons: Evidence for enhanced corticospinal excitability during the observation of transitive but not intransitive hand gestures. Neuropsychologia 48: , 2675–2680. |
[42] | Betti S , Deceuninck M , Sartori L , Castiello U ((2019) ) Action observation and effector independency. Front Hum Neurosci 13: , 416. |
[43] | Abraham A , Gose R , Schindler R , Nelson BH , Hackney ME ((2019) ) Dynamic Neuro-Cognitive Imagery (DNITM) improves developpe performance, kinematics, and mental imagery ability in university-level dance students.. Front Psychol 10: , 382. |
[44] | Cardellicchio P , Dolfini E , Hilt PM , Fadiga L , D’Ausilio A ((2020) ) Motor cortical inhibition during concurrent action execution and action observation. Neuroimage 208: , 116445. |
[45] | Malouin F , Jackson PL , Richards CL ((2013) ) Towards the integration of mental practice in rehabilitation programs. A critical review. Front Hum Neurosci 7: , 576. |
[46] | Krakauer JW , Hadjiosif AM , Xu J , Wong AL , Haith AM ((2019) ) Motor learning. Compr Physiol 9: , 613–663. |
[47] | Wolpert DM , Ghahramani Z , Flanagan JR ((2001) ) Perspectives and problems in motor learning. Trends Cogn Sci 5: , 487–494. |
[48] | Ashford D , Bennett SJ , Davids K ((2006) ) Observational modeling effects for movement dynamics and movement outcome measures across differing task constraints: A meta-analysis. J Mot Behav 38: , 185–205. |
[49] | Vogt S , Thomaschke R ((2007) ) From visuo-motor interactions to imitation learning: Behavioural and brain imaging studies. J Sports Sci 25: , 497–517. |
[50] | Avanzino L , Gueugneau N , Bisio A , Ruggeri P , Papaxanthis C , Bove M ((2015) ) Motor cortical plasticity induced by motor learning through mental practice. Front Behav Neurosci 9: , 105. |
[51] | Hodges NJ ((2017) ) Observations on action-observation research: An autobiographical retrospective across the past two decades. Kinesiol Rev 6: , 240–260. |
[52] | Gentili R , Han CE , Schweighofer N , Papaxanthis C ((2010) ) Motor learning without doing:Trial-by-trial improvement in motor performance during mental training. J Neurophysiol 104: , 774–783. |
[53] | Bonassi G , Lagravinese G , Bisio A , Ruggeri P , Pelosin E , Bove M , Avanzino L ((2020) ) Consolidation and retention of motor skill after motor imagery training. Neuropsychologia 143: , 107472. |
[54] | Dayan E , Cohen LG ((2011) ) Neuroplasticity subserving motor skill learning. Neuron 72: , 443–454. |
[55] | Lepage JF , Morin-Moncet O , Beaule V , de Beaumont L , Champoux F , Theoret H ((2012) ) Occlusion of LTP-like plasticity in human primary motor cortex by action observation. PLoS One 7: , e38754. |
[56] | McGregor HR , Cashaback JGA , Gribble PL ((2018) ) Functional plasticity in somatosensory cortex supports motor learning by observing. Curr Biol 28: , 3892. |
[57] | Ruffino C , Papaxanthis C , Lebon F ((2017) ) Neural plasticity during motor learning with motor imagery practice: Review and perspectives. Neuroscience 341: , 61–78. |
[58] | Heremans E , Feys P , Nieuwboer A , Vercruysse S , Vandenberghe W , Sharma N , Helsen W ((2011) ) Motor imagery ability in patients with early- and mid-stage Parkinson disease. Neurorehabil Neural Repair 25: , 168–177. |
[59] | Pickett KA , Peterson DS , Earhart GM ((2012) ) Motor imagery of gait tasks in individuals with Parkinson disease. J Parkinsons Dis 2: , 19. |
[60] | Cohen RG , Chao A , Nutt JG , Horak FB ((2011) ) Freezing of gait is associated with a mismatch between motor imagery and motor execution in narrow doorways, not with failure to judge doorway passability. Neuropsychologia 49: , 3981. |
[61] | Heremans E , Nieuwboer A , Feys P , Vercruysse S , Vandenberghe W , Sharma N , Helsen WF ((2012) ) external cueing improves motor imagery quality in patients with Parkinson disease. Neurorehabil Neural Repair 26: , 27–35. |
[62] | Avanzino L , Pelosin E , Martino D , Abbruzzese G ((2013) ) Motor timing deficits in sequential movements in Parkinson disease are related to action planning: A motor imagery study. PLoS One 8: , e75454. |
[63] | Scarpina F , Magnani FG , Tagini S , Priano L , Mauro A , Sedda A ((2019) ) Mental representation of the body in action in Parkinson’s disease. Exp Brain Res 237: , 2505–2521. |
[64] | Nonnekes J , Ružička E , Nieuwboer A , Hallett M , Fasano A , Bloem BR ((2019) ) Compensation strategies for gait impairments inParkinson disease: A review. JAMA Neurol 76: , 718–725. |
[65] | Marceglia S , Fiorio M , Foffani G , Mrakic-Sposta S , Tiriticco M , Locatelli M , Caputo E , Tinazzi M , Priori A ((2009) ) Modulation of beta oscillations in the subthalamic area during action observation in Parkinson’s disease. Neuroscience 161: , 1027–1036. |
[66] | Alegre M , Rodriguez-Oroz MC , Valencia M , Perez-Alcazar M , Guridi J , Iriarte J , Obeso JA , Artieda J ((2010) ) Changes in subthalamic activity during movement observation in Parkinson’s disease: Is the mirror system mirrored in the basal ganglia? . Clin Neurophysiol 121: , 414–425. |
[67] | Bonivento C , Rumiati RI , Biasutti E , Humphreys GW ((2013) ) The role of the basal ganglia in action imitation: Neuropsychological evidence from Parkinson’s disease patients. Exp Brain Res 224: , 211–220. |
[68] | Bek J , Gowen E , Vogt S , Crawford T , Poliakoff E ((2018) ) Action observation produces motor resonance in Parkinson’s disease. J Neuropsychol 12: , 298–311. |
[69] | Temporiti F , Adamo P , Cavalli E , Gatti R ((2020) ) Efficacy and characteristics of the stimuli of action observation therapy in subjects with Parkinson’s disease: A systematic review. Front Neurol 11: , 808. |
[70] | Caligiore D , Mustile M , Spalletta G , Baldassarre G ((2017) ) Action observation and motor imagery for rehabilitation in Parkinson’s disease: A systematic review and an integrative hypothesis. Neurosci Biobehav Rev 72: , 210–222. |
[71] | Ryan D , Fullen B , Rio E , Segurado R , Stokes D , O’Sullivan C ((2021) ) Effect of action observation therapy in the rehabilitation of neurologic and musculoskeletal conditions: A systematic review. Arch Rehabil Res Clin Transl 3: , 100106. |
[72] | Pelosin E , Avanzino L , Bove M , Stramesi P , Nieuwboer A , Abbruzzese G ((2010) ) Action observation improves freezing of gait in patients with Parkinson’s disease. Neurorehabil Neural Repair 24: , 746–752. |
[73] | Pelosin E , Barella R , Bet C , Magioncalda E , Putzolu M , Di Biasio F , Cerulli C , Casaleggio M , Abbruzzese G , Avanzino L ((2018) ) Effect of group-based rehabilitation combining action observation with physiotherapy on freezing of gait in Parkinson’s disease. Neural Plast 2018: , 4897276. |
[74] | Mezzarobba S , Grassi M , Pellegrini L , Catalan M , Kruger B , Furlanis G , Manganotti P , Bernardis P ((2018) ) Action observation plus sonification. A novel therapeutic protocol for Parkinson’s patient with freezing of gait. Front Neurol 8: , 723. |
[75] | Giorgi G , Ferrarello F , Merlo F , Fumagalli S , Marchionni N , Di Bari M ((2018) ) First-person perspective action observation training in individuals with Parkinson’s disease: A consideration-of-concept controlled pilot trial. J Geriatr Phys Ther 41: , 134–142. |
[76] | Jaywant A , Ellis TD , Roy S , Lin CC , Neargarder S , Cronin-Golomb A ((2016) ) Randomized controlled trial of a home-based action observation intervention to improve walking in Parkinson disease. Arch Phys Med Rehabil 97: , 665–673. |
[77] | Buccino G , Gatti R , Giusti MC , Negrotti A ((2011) ) Action observation treatment improves autonomy in daily activities in Parkinson’s disease patients: Results from a pilot study. Mov Disord 26: , 1963–1964. |
[78] | Pelosin E , Bove M , Ruggeri P , Avanzino L , Abbruzzese G ((2013) ) Reduction of bradykinesia of finger movements by a single session of action observation in Parkinson disease. Neurorehabil Neural Repair 27: , 552–560. |
[79] | Abraham A , Hart A , Andrade I , Hackney ME ((2018) ) Dynamic neuro-cognitive imagery improves mental imagery ability, disease severity, and motor and cognitive functions in people with Parkinson’s disease. Neural Plast 2018: , 6168507. |
[80] | Zangrando F , Piccinini G , Pelliccioni A , Saraceni VM , Paolucci T ((2015) ) Neurocognitive rehabilitation in Parkinson’s disease with motor imagery: A rehabilitative experience in a case report. Case Rep Med 2015: , 670385. |
[81] | Tamir R , Dickstein R , Huberman M ((2007) ) Integration of motor imagery and physical practice in group treatment applied to subjects with Parkinson’s disease. Neurorehabil Neural Repair 21: , 68–75. |
[82] | Abraham A , Hart A , Dickstein R , Hackney ME ((2019) ) “Will you draw mea pelvis?” Dynamic neuro-cognitive imagery improves pelvic schemaand graphic-metric representation in people with Parkinson’sdisease: A randomized controlled trial. Complement Ther Med 43: , 28–35. |
[83] | Braun S , Beurskens A , Kleynen M , Schols J , Wade D ((2011) ) Rehabilitation with mental practice has similar effects on mobility as rehabilitation with relaxation in people with Parkinson’s disease: A multicentre randomised trial. J Physiother 57: , 27–34. |
[84] | De Melo Santiago LM , De Oliveira DA , De Macêdo Ferreira LGL , DeBrito Pinto HY , Spaniol AP , De Lucena Trigueiro LC , Ribeiro TS , DeSousa AVC , Piemonte MEP , Lindquist ARR ((2015) ) Immediate effects ofadding mental practice to physical practice on the gait ofindividuals with Parkinson’s disease: Randomized clinical trial. NeuroRehabilitation 37: , 263–271. |
[85] | Sarasso E , Agosta F , Piramide N , Gardoni A , Canu E , Leocadi M , Castelnovo V , Basaia S , Tettamanti A , Volontè MA , Filippi M ((2021) ) Action observation and motor imagery improve dual task in Parkinson’s disease: A clinical/fMRI study. Mov Disord 36: , 2569–2582. |
[86] | Pascual-Leone A , Nguyet D , Cohen LG , Brasil-Neto JP , Cammarota A , Hallett M ((1995) ) Modulation of muscle responses evoked by transcranial magnetic stimulation during the acquisition of new fine motor skills. J Neurophysiol 74: , 1037–1045. |
[87] | Reiser M , Büsch D , Munzert J ((2011) ) Strength gains by motor imagery with different ratios of physical to mental practice. Front Psychol 2: , 194. |
[88] | How D , Wagner H , Brach M ((2022) ) Using motor imagery to access alternative attentional strategies when navigating environmental boundaries to prevent freezing of gait –a perspective. Front Hum Neurosci 16: , 750612. |
[89] | Yaguez L , Canavan AGM , Lange H , Homberg V ((1999) ) Motor learning by imagery is differentially affected in Parkinson’s and Huntington’s diseases. Behav Brain Res 102: , 115–127. |
[90] | Bek J , Holmes PS , Craig CE , Franklin ZC , Sullivan M , Webb J , Crawford TJ , Vogt S , Gowen E , Poliakoff E ((2021) ) Action Imagery and Observation in Neurorehabilitation for Parkinson’s Disease (ACTION-PD): Development of a user-informed home training intervention to improve functional hand movements. Parkinsons Dis 2021: , 4559519. |
[91] | Bezerra PT , Santiago LM , Silva IA , Souza AA , Pegado CL , Damascena CM , Ribeiro TS , Lindquist AR ((2022) ) Action observation and motor imagery have no effect on balance and freezing of gait in Parkinson’s disease: A randomized controlled trial. Eur J Phys Rehabil Med 58: , 715. |
[92] | Saruco E , Rienzo F DI , Nunez-Nagy S , Rubio-Gonzalez MA , Jackson PL , Collet C , Saimpont A , Guillot A ((2017) ) Anodal tDCS over the primary motor cortex improves motor imagery benefits on postural control: A pilot study. Sci Rep 7: , 480. |
[93] | Schwell G , Kozol Z , Tarshansky D , Einat M , Frenkel-Toledo S ((2023) ) The effect of action observation combined with high-definition transcranial direct current stimulation on motor performance in healthy adults: A randomized controlled trial. Front Hum Neurosci 17: , 1126510. |
[94] | Tinaz S , Para K , Vives-Rodriguez A , Martinez-Kaigi V , Nalamada K , Sezgin M , Scheinost D , Hampson M , Louis ED , Constable RT ((2018) ) Insula as the interface between body awareness and movement: A neurofeedback-guided kinesthetic motor imagery study in Parkinson’s disease. Front Hum Neurosci 12: , 496. |
[95] | Tinaz S , Kamel S , Aravala SS , Elfil M , Bayoumi A , Patel A , Scheinost D , Sinha R , Hampson M ((2022) ) Neurofeedback-guided kinesthetic motor imagery training in Parkinson’s disease: Randomized trial. Neuroimage Clin 34: , 102980. |
[96] | Cherry J , Kamel S , Elfil M , Aravala SS , Bayoumi A , Patel A , Sinha R , Tinaz S ((2023) ) Mental imagery content is associated with disease severity and specific brain functional connectivity changes in patients with Parkinson’s disease. Brain Imaging Behav 17: , 161–171. |
[97] | Estrada-Barranco C , Martinez-Javaloyes MLÁ , Rodriguez-Costa I , Sanz-Esteban I , Bermejo-Franco A , Aranda-Ruiperez A , Gallegos-Martínez MLÁ ((2023) ) Effectiveness of a programbased on Action-Observation Training (AOT) on motor, functional andcognitive aspects in patients with cognitive impairment: Anon-randomized controlled trial. Healthcare (Basel) 11: , 1030. |
[98] | Rojasavastera R , Bovonsunthonchai S , Hiengkaew V , Senanarong V ((2020) ) Action observation combined with gait training to improve gait and cognition in elderly with mild cognitive impairment a randomized controlled trial. Dement Neuropsychol 14: , 118–127. |