The Use of Image Guided Programming to Improve Deep Brain Stimulation Workflows with Directional Leads in Parkinson’s Disease
Abstract
Background:
Deep brain stimulation (DBS) of the subthalamic nucleus (STN) is a preferred treatment for parkinsonian patients with severe motor fluctuations. Proper targeting of the STN sensorimotor segment appears to be a crucial factor for success of the procedure. The recent introduction of directional leads theoretically increases stimulation specificity in this challenging area but also requires more precise stimulation parameters.
Objective:
We investigated whether commercially available software for image guided programming (IGP) could maximize the benefits of DBS by informing the clinical standard care (CSC) and improving programming workflows.
Methods:
We prospectively analyzed 32 consecutive parkinsonian patients implanted with bilateral directional leads in the STN. Double blind stimulation parameters determined by CSC and IGP were assessed and compared at three months post-surgery. IGP was used to adjust stimulation parameters if further clinical refinement was required. Overall clinical efficacy was evaluated one-year post-surgery.
Results:
We observed 78% concordance between the two electrode levels selected by the blinded IGP prediction and CSC assessments. In 64% of cases requiring refinement, IGP improved clinical efficacy or reduced mild side effects, predominantly by facilitating the use of directional stimulation (93% of refinements).
Conclusions:
The use of image guided programming saves time and assists clinical refinement, which may be beneficial to the clinical standard care for STN-DBS and further improve the outcomes of DBS for PD patients.
INTRODUCTION
Deep brain stimulation (DBS) of the subthalamic nucleus (STN) has efficiently treated motor fluctuations in advanced Parkinson’s disease (PD) for several decades [1–5]. Apart from appropriate patient selection, surgical targeting and post-operative programming remain time consuming components of standard DBS workflows. Recent directional lead technology promises improved spatial specificity of stimulation in DBS and may improve clinical outcomes [6, 7] but also increases the complexity of DBS programming [8]. To address this complexity, innovative IGP software has been recently introduced commercially that enables anatomical mapping, lead location, and visualization of the Volume of Tissue Activated (VTA). IGP offers to serve as a starting point for clinical testing [9, 10]. However, the accuracy of IGP and a practical method for integrating it into clinical practice remains a point of interest for movement disorders physicians. We hypothesize that the use of IGP may reduce programming burden and improve clinical troubleshooting strategies; two major needs in the current clinical practice. In the current study we explored whether IGP will facilitate the use of directional leads by comparing CSC programming from monopolar review (MoR) to IGP. Secondarily, we evaluated the utility of IGP parameters for improving patients suffering mild adverse events or insufficient efficacy after a clinical MoR three months post-surgery. Finally, we investigated the overall clinical efficacy of both strategies at one year for motor and non-motor handicap.
MATERIALS AND METHODS
Patients
Consecutives patients with PD were prospectively enrolled as part of an ancillary study of the PREDISTIM cohort in Lille (Protocol 2013-A00193-42, NCT02360683), approved from the CPP Nord Ouest-IV Ethical Committee. Inclusion criteria were: a diagnosis of PD according to the UJ Parkinson’s Disease Society Brain Bank, disease duration ≥5 years, age between 18 and 75 years, and indication for STN-DBS. Exclusion criteria were: atypical parkinsonism, severe cognitive impairment, psychiatric disorders, levodopa motor response < 30%, and other contraindications to surgery. Clinical assessment included collection of demographic data (age, sex, disease history, ethnicity), treatment history, social status, MDS-UPDRS part I-IV, part III performed in both OFF and ON state during a standard acute levodopa challenge, cognitive and behavioral tests (the Lille Apathy Rating Scale, the Hamilton Anxiety Rating scale, the Hamilton Depression scale, the Montreal Cognitive Assessment, Echelle Comportementale de la Maladie de Parkinson (French Behavioral scale for Parkinson’s disease), Boston naming test), treatments (specific to the disease, unrelated to the disease, psychotropics), and adverse events.
Patients gave written informed consent. The study was conducted according to the good clinical practice and local regulations and data collection was compliant with General Data Protection Regulation rules.
Surgery
All patients were implanted with bilateral directional leads (Cartesia, Boston Scientific, Marlborough, MA) in the STN under general anesthesia by direct targeting using preoperative MRI with Renishaw’s euromata robot (Renishaw, Wotton-under Edge, United Kingdom) and computerized tomography (O-arm™, Medtronic, Dublin, Ireland). These directional leads have four electrode levels, with two annular contacts at the proximal and distal level and two central electrode levels with three segmented contacts each.
Clinically based post-operative determination of stimulation parameters
Initial programming was delayed to avoid implantation related microlesion effects consistent with timing at other Parkinson DBS expert centers. At three months post-surgery, OFF-Meds patients (overnight withdrawal of all dopaminergic medication for 12 h) were hospitalized (4–48 h) to determine initial DBS stimulation parameters. We observed a wide time frame due to patient’s variable response to stimulation effects. Neurologists and movement disorders specialists (NC, EM, AK, LD, CS, DD, CM) performed a clinical MoR while blinded to lead locations derived from the IGP software. We optimized stimulation settings to improve rigidity, bradykinesia, or tremor while eliciting only manageable or minimal side effects such as dyskinesia, dystonia, tremor, or muscle pulling. Briefly, the pulse width and frequency were standardized at 60μs and 130 Hz. Each electrode level was successively activated with annular stimulation to find the best clinical effect, starting with the more symptomatic side. In the case of an electrode level with directional contacts, each contact was tested individually in a directional mode and in combination as annular mode. Stimulation amplitude was increased in 0.5 mA steps while assessing clinical outcome. We prioritized larger therapeutic window if there was equivalent clinical efficacy, and lastly we prioritized lower clinical efficacy threshold if clinical efficacy and therapeutic window both equivalent.
Imaging guided programming method and timing
All pre- and post-operative images (included standard of care imaging sequences: 3DT1, T2 W, FFE, post-operative CT; see Supplementary Material) were automatically coregistered using Brainlab Elements® rigid Image Fusion (Brainlab AG, Munich, Germany) implemented in Guide™ XT software (Boston Scientific, Marlborough, MA). After the fusions were visually verified the automatic segmentation [11] of the substantia nigra, STN, red nucleus, and internal capsule was performed using Elements Anatomical Mapping (Brainlab AG, Munich, Germany). All segmented structures were again systematically reviewed by a neurosurgeon (GT) and an anatomical expert (ASR) and manually refined to match the expected segmentation based on a priori anatomical knowledge and Schaltenbrand-Wahren atlas cross referencing. Second, both the location and orientation of the implanted DBS leads were automatically detected on post-operative CT using Elements Lead Localization (Brainlab AG, Munich, Germany), which is an essential step to ensure precise stimulation using image guidance. Lastly, a VTA was generated in the software [12] with the standardized parameters of 130 Hz frequency, 60μs pulse width, and 1.5 mA amplitude which was previously determined to be sufficient and clinically relevant [13, 14] to “virtually screen” the lead. We used the anatomical segmentation with the patient specific lead locations to visually choose the two best contact configurations blinded to clinically determined parameters. We chose electrode levels that were adjacent to the dorsal sensorimotor STN [15], utilizing directional stimulation when visual inspection suggested it would achieve more specific activation of the target region. Thus, we chose two contact configurations: each one either a combination of annular stimulation across an entire electrode level or a specific directional contact. This procedure took 35–50 min with 30–40 min dedicated to the 3D anatomical segmentation process and 5–10 min to select the best stimulation parameters with the VTA overlying the appropriate anatomical target (STN and adjacent nuclei).
To assess the accuracy of IGP compared to CSC, we analyzed the concordance between the clinically determined and IGP-predicted two best electrode levels. This allowed us to assess if the significant time savings of using IGP translated into parameters appropriate for routine clinical use. Both the electrode level and the contact configuration (annular stimulation, directional stimulation, or multipolar) identified by clinicians were compared with the contacts suggested by the software determined by the neurosurgeon and anatomical expert.
Clinical refinement strategy
Despite the optimal clinical MoR at three months post-surgery, mild side effects or insufficient clinical efficacy frequently occur after some weeks and may lead to discomfort for the patient. Although directional leads may further optimize therapy, determining optimal directional stimulation parameters takes additional time. To avoid discomfort for the patient with multiple stimulation settings, we tested the usefulness of IGP to improve the clinical refinement workflow by predicting the optimal direction for stimulation. Electrode levels predicted by IGP were compared to clinically determined optimal electrode level and classified as either ‘identical’ when the IGP and CSC coincided, or ‘different’ when they differed.
Assessment of STN-DBS clinical benefit at baseline and one-year post-surgery
Clinical benefit of STN-DBS was assessed one-year post-surgery and compared to baseline. Motor and non-motor handicap were evaluated at the hospital with an acute L-DOPA challenge under both OFF-Meds and ON-Meds conditions. The OFF-Meds condition was done first after overnight withdrawal of L-Dopa and 5 half-lives of dopaminergic agonists. The ON-Meds levodopa dose corresponded to 150% of the usual morning levodopa equivalent dose used by patients to relieve their symptoms. Dopaminergic medication consumption was calculated by the Levodopa-equivalent daily dose (LEDD) which standardizes doses of Levodopa, dopamine agonists and other parkinsonian drugs such as MAO-B inhibitors, COMT inhibitors, and amantadine.
Statistical analysis
Data are presented as means±SEM or percentages. Statistical analysis was performed using Prism v7.0a (GraphPad Software, San Diego, CA). An unpaired Mann-Whitney nonparametric test was performed for each individual clinical data comparison between the baseline (V0) and one year after surgery (V1).
RESULTS
Patients
Thirty-two consecutive parkinsonian patients were included (22 males, 10 females; 35–68 years old, mean 58.4 ±8.6; mean disease duration of 11.2±0.8 years; Table 1). All patients underwent a bilateral STN-DBS surgery with directional leads. No patients were excluded from analysis. No hemorrhage, infection or other severe adverse events were observed in this cohort.
Table 1
Clinical data on Predistim advanced parkinsonian’ patients from Lille at baseline and one-year post-surgery
Baseline (V0) | One-year post-surgery (V1) | p | |||
Mean±SEM | Range | Mean±SEM | Range | ||
Age (y) | 58.4±1.5 | 35–68 | |||
Gender | |||||
Men | 22 | ||||
Women | 10 | ||||
Disease duration (years) | 11.2±0.8 | 3–23 | |||
LEDD (mg) | 1528±127.5 | 500–3051 | 755.7±81.9 | 100–1496 | < 0.0001*** |
LEED Reduction with DBS (%) | 44.3±3.1 | 15.4–72.9 | |||
MDS-UPDRS part III | |||||
Worst OFF | 46.4±2.8 | 19–85 | 50.2±3.3 | 25–88 | 0.311 |
Best ON | 12.8±1.5 | 2–32 | 14.0±1.5 | 2–31 | 0.297 |
ON-Stim / OFF-Meds | 28.1±2.8 | 7–66 | < 0.0001*** | ||
OFF-Stim / ON-Meds | 25.0±2.7 | 2–54 | |||
MDS-UPDRS part IV | 8.1±0.8 | 0–16 | 5.2±0.8 | 0–14 | 0.021* |
MDS-UPDRS total | |||||
Worst OFF | 76.7±4.2 | 28–116 | 50.5±3.4 | 25–88 | < 0.0001*** |
Best ON | 42.9±4 | 18–89 | 43.3±3.2 | 24–79 | 0.613 |
Hoehn &Yahr | |||||
OFF-Meds | 2.8±0.2 | 2–5 | 2.8±0.2 | 1.5–5 | 0.928 |
ON-Meds | 1.2±0.1 | 0–3 | 1.7±0.1 | 0–3 | 0.005** |
Schwab &England | |||||
OFF-Meds | 67.5±4.2 | 20–100 | 71.3±4.4 | 20–100 | 0.464 |
ON-Meds | 97.2±1.3 | 70–100 | 94±1.6 | 70–100 | 0.034* |
MoCA | 25.8±0.4 | 22–30 | 25.2±0.4 | 20–29 | 0.376 |
PDQ39 | 53.2±4.5 | 5–101 | 52.4±4.8 | 15–102 | 0.398 |
Values represent mean±SEM or number (%). p < 0.05 is significant. LEDD, Levodopa Equivalent Daily Dose; MoCA, Montreal Cognitive Assessment; PDQ-39, Parkinson’s Disease Questionnaire 39 items; UPDRS, Unified Parkinson’s Disease Rating Scale.
Contact prediction concordance
Among the 64 directional leads in the study, we observed a 78% concordance between the clinically determined electrode level and the two best IGP predicted levels (Fig. 1A). If IGP was predicting one directional contact on an electrode level and CSC was predicting the same electrode level but as annular stimulation, we considered this as a contact concordance. Because the VTA visualization allows fractionalized stimulation of the STN that could utilize two adjacent electrode levels, we considered the more relevant comparison to be whether the two IGP determined electrode levels included the clinical level.
Fig. 1
Implementation of combined MoR and IGP in clinical practice saves time and assists clinical refinement. A) We observed 78% (50/64 directional leads) concordance of the electrode level identified by monopolar review and IGP software (A1). Among the 22 patients experiencing insufficient efficacy or mild side effects, 64% (14/22) saw clinical improvements by utilizing either directional stimulation or selecting the contact predicted by IGP. MoR, Monopolar review; IGP, Image Guided Programming. B) Representative VTA model in one patient with improvement using directional stimulation. 3D VTA visualization of the activated contacts are represented for ring mode stimulation (upper row) and directional stimulation (lower row). Basal ganglia nuclei near the STN are segmented on a T2-weighted MRI axial view. We observed a the VTA was more compressed in the case of directional mode (white arrows) than ring mode. Thal, Thalamus (turquoise); STN, Subthalamic Nucleus (green); RN, Red Nucleus (red); SN, Substantia Nigra (purple); A, anterior; P, posterior; R, right; L, left.
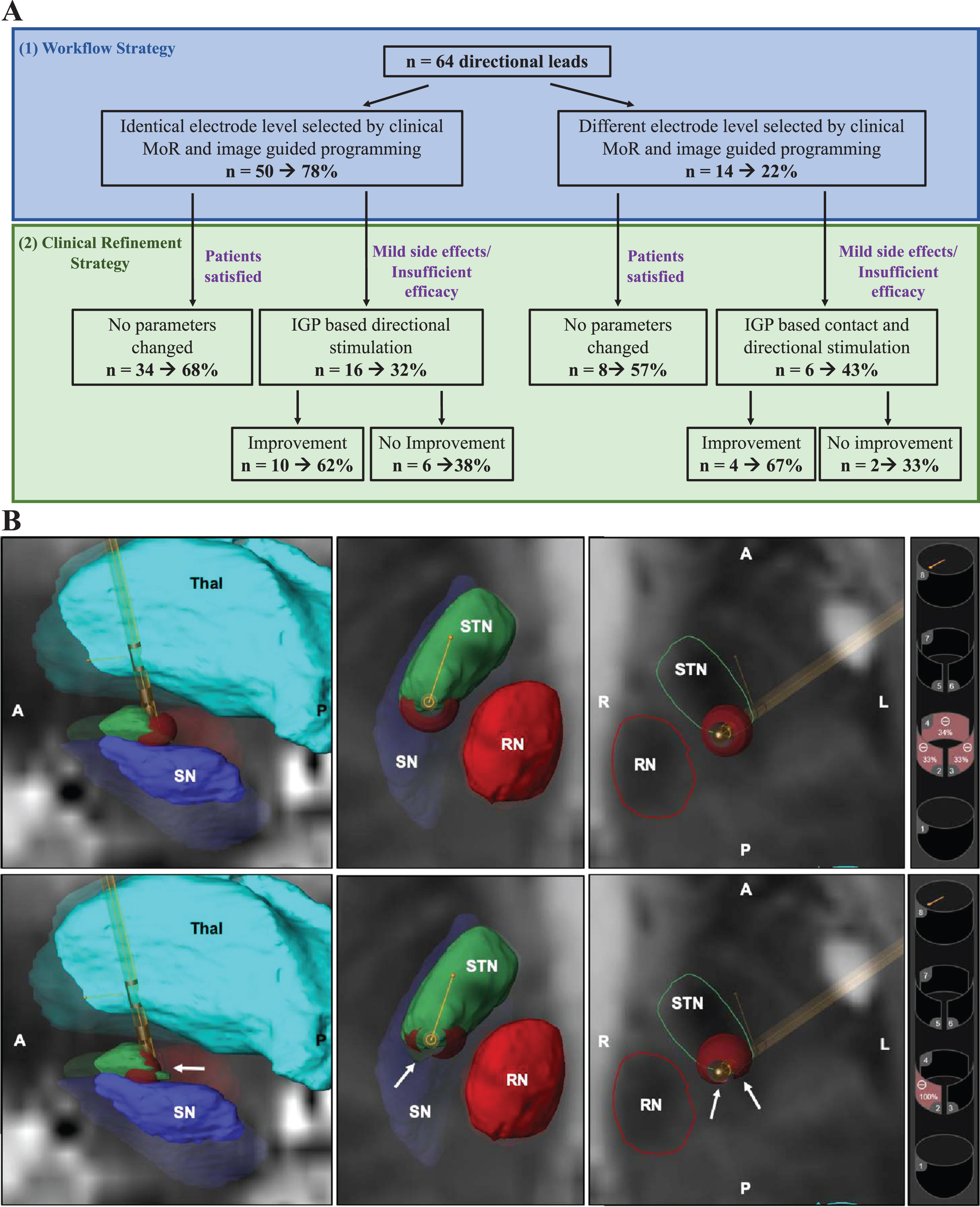
Directional stimulation settings were suggested in 95.3% (N = 61/64) of cases with IGP while directional stimulation was used in only 26.6% (N = 17/64) of cases after MoR.
In 22% of cases, the electrode level differed between clinical and IGP evaluation (Fig. 1A) occurring in the less symptomatic side of patients in 58.3% of cases, which is frequently more difficult to assess clinically.
Clinical refinement outcomes
Mild side effects (dystonia, gait disorder, or freezing) were observed for 37% of patients (N = 12/32) after three months with clinically based programming. Seven of 12 patients had the same electrode level selected by clinically based and IGP. However, when directional stimulation was explored, 71.4% of patients (N = 5/7) improved (Table 2, Fig. 1B). For the 5 remaining patients, clinically determined contacts differed from the two electrode levels selected with IGP. Switching the active contact to the contact level suggested by the IGP resulted in 60% of patients (N = 3/5) improving (Table 2).
Table 2
Improvement of side effects or clinical efficacy with image guided programming
Patient | Clinical Presentation | Initial Outcome | Modification based on IGP | Final Outcome |
1 | Hypokinetic gait | Insufficient efficacy | Directional stimulation and adjacent contact | Partial improvement |
2 | Left Side Dystonia | Side effect | Directional stimulation and adjacent contact | Resolved |
3 | Bilateral freezing | Insufficient efficacy | Adjacent contact | Partial improvement |
4 | Hypokinetic gait and dystonia | Insufficient efficacy and side effect | Directional stimulation | Gait improvement and partial improvement of dystonia |
5 | Bilateral dystonia | Side effect | Directional stimulation | Resolved |
6 | Left foot dystonia | Side effect | Directional stimulation | Resolved |
7 | Slight dopa-responsive freezing of gait | Insufficient efficacy | Directional stimulation and adjacent contact | Partial improvement |
8 | Left foot dystonia, dysarthria | Side effect | Directional stimulation and adjacent contact | Not resolved |
9 | Tremor | Insufficient efficacy | Directional stimulation | No improvement |
10 | Freezing | Insufficient efficacy | Directional stimulation | No Improvement |
11 | Tremor | Insufficient efficacy | Directional stimulation | Partial improvement |
12 | Dyskinesia | Too much efficacy | Directional stimulation and adjacent contact | No improvement |
Side effects correspond to motor symptoms induced by STN-DBS, insufficient efficacy correspond to motor symptoms insufficiently relieved by STN-DBS. Partial improvement correspond to clinical refinement not fully abrogated but satisfactory for the patient.
When no improvements were observed with the IGP settings, we typically observed a similar outcome to clinically determined parameters.
Clinical efficacy assessment of STN-DBS one-year post-surgery
The overall efficacy of STN-DBS with directional leads and the respective efficacy of the two programming strategies combining clinical based and IGP analysis was assessed at one-year post-surgery. Compared to baseline, we observed a 39.4% % reduction of MDS-UPDRS part III OFF-Meds (46.4±2.8 at baseline WOFF vs. 28.1±2.8 at one year ON-stim/OFF-Meds, p < 0.0001), a 66% reduction of the MDS-UPDRS total score under OFF-Meds conditions (76.7±4.2 at baseline vs. 50.5±3.4 at one year, p < 0.0001), a 64% significant reduction of MDS-UPDRS part IV score (8.1±0.8 at baseline vs. 5.2±0.8 at one year, p < 0.05), and a 49% decrease of dopaminergic treatment reflected by lower LEDD (1528±127.5 at baseline vs. 755.7±81.9 at one year, p < 0.0001) (Table 1).
DISCUSSION
To take full advantage of directional lead technology, we explored the potential benefit of combining clinical and image guided programming (IGP) for contact prediction in routine clinical use. Our data suggest that the use of IGP in standard clinical DBS programming is beneficial for both neurologist and patient by reducing programming time and helping avoid clinical issues of insufficient efficacy and mild side effects (Fig. 2). Indeed, we observed a good concordance between contacts predicted by the software and the MoR. The differences observed in the use of directional settings by clinically determined and IGP suggested settings is not surprising considering the significant time burden of a MoR, compounded by patient discomfort in the OFF-Meds state and requirement to allow the effects of a previously tested setting to washout. This necessarily limits the number of contacts that can be tested during the MoR as suggested by Waldthaler and collaborators [9]. In line with other pilot studies [10, 16, 17], we propose that clinicians could benefit by beginning the clinical evaluation with the two IGP predicted electrode levels along with their contacts parameters, perhaps allowing the programmer to prioritize directional stimulation. This may assist in optimizing the therapeutic window, finally choosing the best configuration in terms of clinical benefit for the patient. Furthermore, despite clinical evaluations and good therapeutic windows, mild side effect or insufficient efficacy are frequently observed. We show here that visualization of the VTA alongside anatomical structures enabled by IGP may help clinicians to abrogate side effects.
Fig. 2
Image guided programming to save time and to assist clinical refinement. min, minutes; h, hours; STN, subthalamic nucleus.
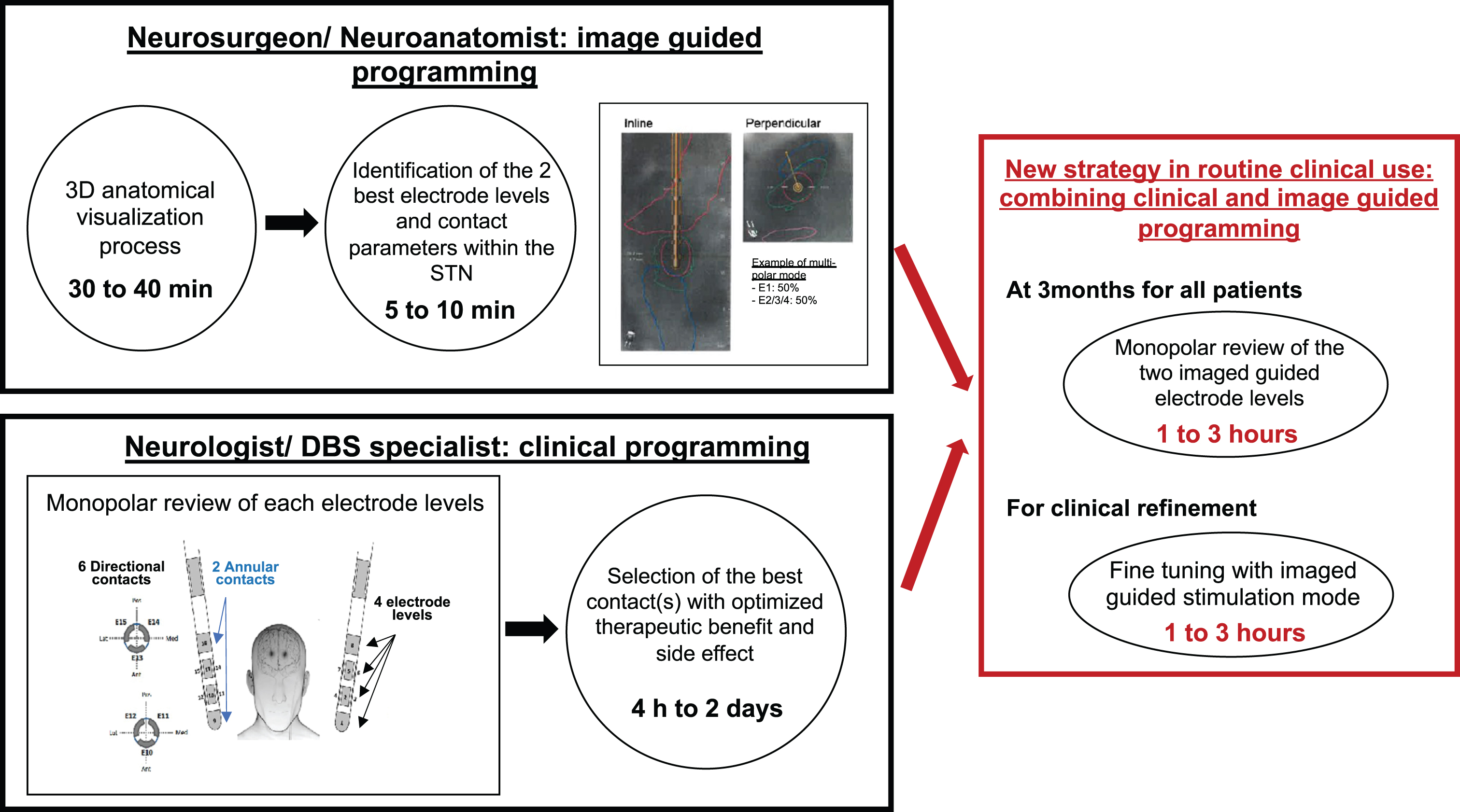
In few cases no improvement with the IGP settings were observed. One reason could be inaccurate localization or orientation detection of the implanted DBS leads on post-operative CT, which may occur for various reasons. We quantified the air volume in the CT to reasonably rule out pneumocephalus. However, there is also a well-described tendency of DBS leads to rotate in the day following surgery [18]. Additionally, brain shift caused by cerebrospinal fluid leakage does not stabilize until one month after surgery [19], which could also contribute to inaccurate localization. To be cautious, we advise replication of this analysis on a larger cohort of patients requiring clinical refinement and add additional post-operative imaging obtained after leads have settled.
The clinical efficacy of STN-DBS at one-year post-surgery and the satisfaction of the patients were in the range of expected results, confirming normal patients selection, targeting, and programming. Moreover, this is in line with significant observed improvement on the UPDRS part III scores and reduction of the LEDD after reprogramming guided by the fused images of MRI and CT at 6 months post-surgery on 54 patients [20]. The lack of improvement in quality of life we observed may be explained by its classical variability and our relatively small sample of patients.
In sum, our study contributes to growing evidence that paves the way for the use of IGP in regular DBS clinical practice and the possible high achievement of combining the two types of programming to maximize the benefit of using directional leads. Nevertheless, the results of this study should be confirmed in a larger multicentric cohort with a longer term of clinical evaluation.
ACKNOWLEDGMENTS
We thank all participants and their families for their cooperation. The authors are grateful for financial support from the French Ministry of Health (National PHRC 2012), France Parkinson charity association, Boston Scientific and support from the French clinical research network NS-Park/F-CRIN.
FUNDING
The study has been supported by the French Ministry of Health (National PHRC 2012), France Parkinson charity association and Boston Scientific.
CONFLICT OF INTEREST
A.S. Rolland, G Touzet, N Carriere, E Mutez, A Kreisler, C Simonin, G Kuchcinski, N Chalhoub, J.P. Pruvo declare no disclosures relevant to the manuscript; L Defebvre reported Advisory for Abbvie, lectures with Abbvie, UCB and Orkyn; N Reyns declares no disclosures relevant to the manuscript; D Devos received PHRC grants from the French Ministry of Health and research funding from the ARLSA charity, the France Parkinson Charity and the Credit Agricole Foundation; served on several Scientific Advisory Boards for Orkyn, Air Liquide, Abbvie, Lundbeck, Ever Pharma, Medtronic and Boston Scientific and has equity stake in InBrain Pharma; C Moreau received PHRC grants from the French Ministry of Health and research funding from the ARLSA charity, the France Parkinson Charity and the Credit Agricole Foundation, served on several Scientific Advisory Boards for Abbvie, Ever Pharma, Medtronic and Boston Scientific and has equity stake in InBrain Pharma.
Dr. Moreau is an Editorial Board member of this journal but was not involved in the peer-review process nor had access to any information regarding its peer review.
DATA AVAILABILITY
All data of this study are available from the corresponding author, upon reasonable request.
SUPPLEMENTARY MATERIAL
[1] The supplementary material is available in the electronic version of this article: https://dx.doi.org/10.3233/JPD-225126.
REFERENCES
[1] | Deuschl G , Schade-Brittinger C , Krack P , Volkmann J , Schäfer H , Bötzel K , Daniels C , Deutschnländer A , Dillmann U , Eisner W , Gruver D , Hamel W , Herzog J , Hilker R , Klebe S , Kloss M , Koy J , Krause M , Kupsch A , Lorenz D , Lorenzl S , Medhoren HM , Moringlane JR , Oertel W , Pinsker MO , Reichmann H , Reuss A , Schenider GH , Schnitzler A , Steude U , Sturm V , Timmermann L , Tronnier V , Trottenberg T , Wojtecki L , Wolf E , Poewe W , Voges J ((2006) ) A randomized trial of deep-brain stimulation for Parkinson’s disease. N Engl J Med 355: , 896–908. |
[2] | Okun MS , Gallo BV , Mandybur G , Jagid J , Foote KD , Revilla FJ , Alterman R , Jankovic J , Simpson R , Junn F , Verhagen L , Arle JE , Ford B , Goodman RR , Stewart RM , Horn S , Baltuch GH , Kopell BH , Marshall F , Peichel D , Pahwa R , Lyons KE , Tröster AI , Vitek JL , Tagliati M ; SJM DBS Study Group ((2012) ) Subthalamic deep brain stimulation with a constant-current device in Parkinson’s disease: An open-label randomised controlled trial. Lancet Neurol 11: , 140–149. |
[3] | Schuepbach WMM , Rau J , Knudsen K , Volkmann J , Krack P , Timmermann L , Hälbig TD , Hesekamp H , Navarro SM , Meier N , Falk D , Mehdorn M , Paschen S , Maarouf M , Barbe MT , Fink GR , Kupsch A , Gruber D , Schneider GH , Seigneuret E , Kistner A , Chaynes P , Ory-Magne F , Brefel Courbon C , Vesper J , Schnitzer A , Wojtecki L , Houeto JL , Bataille B , Maltete D , Damier P , Raoul Sn Sixel-Doering F , Hellwig D , Gharabaghi A , Krüger R , Pinsker MO , Amtage F , Régis JM , Witjas T , Thobois S , Mertens P , Kloss M , Hartmann A , Oertel WH , Post B , Speelman H , Agid Y , Schade-Brittinger C , Deuschl G , EARLYSTIM Study Group ((2013) ) Neurostimulation for Parkinson’s disease with early motor complications. N Engl J Med 368: , 610–622. |
[4] | Weaver FM , Follett K , Stern M , Hur K , Harris C , Marks WJ Jr , Rothlind J , Sagher O , Reda D , Moy CS , Pahwa R , Birchiel K , Hogarth P , Lai EC , Duda JE , Holloway K , Samii A , Horn S , Bronstein J , Stoner G , Heemskerk J , Hunag GD , CSP 468 Study Group ((2009) ) Bilateral deep brain stimulation vs best medical therapy for patients with advanced Parkinson disease. JAMA 301: , 63–73. |
[5] | Williams A , Gill S , Varma T , Jenkinson C , Quinn N , Mitchell R , Scott R , Ives N , Rick N , Daniels J , Patel S , Wheatley K , PD SURG Collaborative Group ((2010) ) Deep brain stimulation plus best medical therapy versus best medical therapy alone for advanced Parkinson’s disease (PD SURG trial): A randomised, open-label trial. Lancet Neurol 9: , 581–591. |
[6] | Lehto LJ , Slopsema JP , Johnson MD , Shatillo A , Teplitzky BA , Utecht L , Adriany G , Mangia S , Sierra A , Low WC , Gröhn O , Michaelu S ((2017) ) Orientation selective deep brain stimulation. J Neural Eng 14: , 016016. |
[7] | Schupbach WMM , Chabardes S , Matthies C , Pollo C , Steigerwald F , Timmermann L , Visser Vandewalle V , Volkmann J , Schuurman PR ((2017) ) Directional leads for deep brain stimulation: Opportunities and challenges. Mov Disord 32: , 1371–1375. |
[8] | Ten Brinke TR , Odekerben VJJ , Dijk JM , van den Munchkof P , Schuurman PR , de Bie RMA ((2018) ) Directional deep brain stimulation: First experiences in centers across the globe. Brain Stimul 11: , 949–950. |
[9] | Waldthaler J , Bopp M , Kühn N , Bacara B , Keuler M , Gjorgjevski M , Carl B , Timmermann L , Nimsky C , Pedrosa DJ ((2012) ) Imaging-based programming of subthalamic nucleus deep brain stimulation in Parkinson’s disease. Brain Stimul 14: , 1109–1117. |
[10] | Lange F , Steigerwald F , Malzaker T , Brandt GA , Ordofer TM , Roothans J , Reich MM , Fricke P , Volkmann J , Matthies C , Capetian PD ((2021) ) Reduced programming time and strong symptom control even in chronic course through imaging-based DBS programming. Front Neurol 12: , 785529. |
[11] | Reinacher PC , Varkuti B , Krüger MT , Piroth T , Egger K , Roelz R , Coenen VA ((2019) ) Automatic segmentation of the subthalamic nucleus: A viable option to support planning and visualization of patient-specific targeting in deep brain stimulation. Oper Neurosurg 17: , 497–502. |
[12] | Butson CR , Cooper SE , Henderson JM , McIntyre CC ((2007) ) Patient-specific analysis of the volume of tissue activated during deep brain stimulation. Neuroimage 34: , 661–670. |
[13] | Pollo C , Kaelin-Lang A , Oertel MF , Stieglitz L , Taub E , Fuhr P , Lozano AM , Raabe A , Schüpach M ((2014) ) Directional deep brain stimulation: An intraoperative double-blind pilot study. Brain 137: , 2015–2026. |
[14] | Dembek TA , Hoevels M , Hellerbach A , Horn A , Petry-Schmelzer JN , Borggrefe J , Witrhs J , Dafsari HS , Barbe MT , Visser-Vandewalle V , Treuer H ((2019) ) Directional DBS leads show large deviations from their intended implantation orientation. Parkinsonism Relat Disord 67: , 117–121. |
[15] | Coenen VA , Prescher A , Schmidt T , Picozzi P , Gielen FLH ((2008) ) What is dorso-lateral in the subthalamic nucleus (STN)? - a topographic and anatomical consideration on the ambiguous description of today’s primary target for deep brain stimulation (DBS) surgery. Acta Neurochir 150: , 1163–1165. |
[16] | Pavese N , Tai YF , Yousif N , Nandi D , Bain PG ((2020) ) Traditional trial and error versus neuroanatomic 3-dimensional image software-assisted deep brain stimulation programming in patients with Parkinson Disease. World Neurosurg 134: , e98–e102. |
[17] | Vilkhu G , Goas C , Miller JA , Kelly SM , McDonald KJ , Tsai AJ , Dviwedi A , Dalm B D , Merola A ((2023) ) Clinician vs. imaging-based subthalamic nucleus deep brain stimulation programming. Parkinsonism Relat Disord 106: , 105241. |
[18] | Krüger MT , Naseri Y , Cavalloni F , Reinacher PC , Kägi G , Weber J , Brogle D , Bozinov O , Hägele-Link S , Brugger F ((2021) ) Do directional deep brain stimulation leads rotate after implantation? Acta Neurochir 163: , 197–203. |
[19] | Kim YH , Kim HJ , Kim C , Jeon BS , Paek SH ((2010) ) Comparison of electrode location between immediate postoperative day and 6 months after bilateral subthalamic nucleus deep brain stimulation. Acta Neurochir 113: , 639–647. |
[20] | Lee J-Y , Jeon BS , Paek SH , Lim YH , Kim M-R , Kim C ((2010) ) Reprogramming guided by the fused images of MRI and CT in subthalamic nucleus stimulation in Parkinson disease. Clin Neurol Neurosurg 112: , 47–53. |