A Critical Analysis of Intestinal Enteric Neuron Loss and Constipation in Parkinson’s Disease
Abstract
Constipation afflicts many patients with Parkinson’s disease (PD) and significantly impacts on patient quality of life. PD-related constipation is caused by intestinal dysfunction, but the etiology of this dysfunction in patients is unknown. One possible cause is neuron loss within the enteric nervous system (ENS) of the intestine. This review aims to 1) Critically evaluate the evidence for and against intestinal enteric neuron loss in PD patients, 2) Justify why PD-related constipation must be objectively measured, 3) Explore the potential link between loss of enteric neurons in the intestine and constipation in PD, 4) Provide potential explanations for disparities in the literature, and 5) Outline data and study design considerations to improve future research. Before the connection between intestinal enteric neuron loss and PD-related constipation can be confidently described, future research must use sufficiently large samples representative of the patient population (majority diagnosed with idiopathic PD for at least 5 years), implement a consistent neuronal quantification method and study design, including standardized patient recruitment criteria, objectively quantify intestinal dysfunctions, publish with a high degree of data transparency and account for potential PD heterogeneity. Further investigation into other potential influencers of PD-related constipation is also required, including changes in the function, connectivity, mitochondria and/or α-synuclein proteins of enteric neurons and their extrinsic innervation. The connection between enteric neuron loss and other PD-related gastrointestinal (GI) issues, including gastroparesis and dysphagia, as well as changes in nutrient absorption and the microbiome, should be explored in future research.
INTRODUCTION
Parkinson’s disease (PD) is a progressive and currently incurable neurodegenerative disease [1]. PD is clinically characterized by motor abnormalities, including slowness of voluntary movement (bradykinesia), involuntary movement (resting tremor) and/or increased muscle tone (rigidity) [2, 3], which are commonly accompanied by psychiatric and autonomic dysfunction [3].
The motor dysfunctions in PD primarily manifest due to an impaired ability of the basal ganglia to regulate movement, stemming from dopamine depletion in the striatum [2, 4, 5]. The level of striatal dopamine significantly diminishes once a proportion of substantia nigra pars compacta (SNpc) dopaminergic neurons, and an even greater proportion of their projections, degenerate [6]. The causes and mechanisms underlying this degeneration remain the subject of ongoing research [7, 8], such that it cannot be delayed or prevented by existing clinical interventions [9]. Fortunately, the gold-standard PD medication l-3,4-dihydroxyphenylalanine (levodopa) [10], which is believed to increase the amount of dopamine within the striatum [11], provides substantial and superior relief from PD motor symptoms [9, 12].
In addition to the characteristic movement issues, PD patients commonly suffer from sleep, mood, cognitive and gastrointestinal (GI) dysfunction (collectively termed ‘non-motor symptoms’), none of which are relieved by levodopa or other therapeutics effective at alleviating motor symptoms [13, 14]. These findings, paired with the appearance of hyposmia, depression, constipation and sleep disturbances sometimes years before motor symptom onset, suggest that depletion of striatal dopamine is not what gives rise to non-motor symptoms in PD [13, 15].
In terms of PD-related GI symptoms, which include dysphagia (swallowing difficulty), gastroparesis (slowed stomach emptying), and constipation [16], it has been suggested that dysfunction of the enteric nervous system (ENS) may contribute to their occurrence [17]. The ENS is an extensive neural network embedded within the gut wall, from the esophagus to the internal anal sphincter, and it plays important roles in the control of GI activities [18]. There are connections and bi-directional communication between the central nervous system (CNS) and the ENS, which allow these systems to influence one another and collectively modulate gut function [18]. However, the CNS primarily regulates esophageal movement and stomach contractions [19], while intestinal motility is dominantly controlled by the ENS [20] with input from the vagus nerve, pelvic nerves, and sympathetic prevertebral ganglia [18]. Prolonged colonic transit time (CTT), typically due to decreased colonic motility [21, 22], is thought to be a major contributor to PD-related constipation [23].
Although the prevalence of constipation among PD patients varies between studies [24, 25], constipation is more prevalent in the PD population than in the general population aged 60 years and over [26, 27], can precede the onset of PD motor dysfunction by more than 10 years [28, 29] and significantly impacts on patient quality of life [30]. Current therapies aimed at managing constipation tend to have limited efficacy in PD patients [31, 32]. As loss of SNpc neurons and their striatal projections in the CNS is associated with development of PD motor symptoms, it is hypothesized that neuronal damage and death in the intestinal ENS may underlie the initiation of PD-related constipation.
This review will critically evaluate the evidence for and against intestinal enteric neuron loss in PD patients, justify why PD-related constipation must be objectively measured and explore the potential link between loss of enteric neurons in the intestine and constipation in PD. Importantly, this review will highlight discrepancies in the literature on intestinal enteric neuron loss in PD patients, present potential explanations for these disparities and suggest solutions that could be implemented by future studies.
THE ENTERIC NERVOUS SYSTEM CONTROL OF INTESTINAL CONTENT MOVEMENT
Within the small and large intestine, most enteric neurons are organized into two ganglionated plexuses— the myenteric plexus and the submucosal plexus [18, 33]. Neurons of the myenteric plexus originate between the longitudinal and circular muscle layers of the intestine, while neurons of the submucosal plexus stem from the region between the circular muscle and the intestinal mucosa [18, 33] (Fig. 1).
Fig. 1
Structure of the intestinal ENS. This simplified representation depicts how the myenteric and submucosal plexuses are arranged within the walls of the small intestine, relative to the longitudinal and circular muscle. The ENS within the large intestine exhibits a near-identical organization [20], but the mucosa is not organized into villi [34]. Reproduced by permission from Morgan & Claypool Publishers and Prof Jackie D. Wood from Enteric Nervous System: The Brain-In-The-Gut by Wood © 2011 Morgan & Claypool Life Sciences [33].
![Structure of the intestinal ENS. This simplified representation depicts how the myenteric and submucosal plexuses are arranged within the walls of the small intestine, relative to the longitudinal and circular muscle. The ENS within the large intestine exhibits a near-identical organization [20], but the mucosa is not organized into villi [34]. Reproduced by permission from Morgan & Claypool Publishers and Prof Jackie D. Wood from Enteric Nervous System: The Brain-In-The-Gut by Wood © 2011 Morgan & Claypool Life Sciences [33].](https://content.iospress.com:443/media/jpd/2022/12-6/jpd-12-6-jpd223262/jpd-12-jpd223262-g001.jpg)
Studies of the guinea pig intestine demonstrate that neurons in each plexus interact and influence one another (Fig. 2). For example, submucosal intrinsic sensory neurons (ISNs), which indirectly detect and respond to mechanical stimulation of the intestinal mucosa [35, 36], project to the myenteric plexus [36–38] where the majority of neurons innervating the circular and longitudinal muscle of the intestine reside [18]. Myenteric ISNs, which can directly detect stretching of the intestinal wall [39] or chemical stimulation of the mucosa [40, 41], also provide excitatory input to interneurons and motor neurons in the myenteric plexus [40]. Secretomotor neurons in the submucosal plexus receive excitatory input from certain myenteric neurons [42], as well as from submucosal ISNs [35], and in turn stimulate mucosal epithelial cells to secrete chloride, which facilitates secretion of water and sodium into the intestinal lumen [35].
Fig. 2
Interactions between intestinal enteric neurons. A) Simplified representation of enteric neuronal circuits directly governing intestinal motility, which involve both myenteric and submucosal neurons. B) Simplified representation of the enteric neuronal interactions directly and indirectly facilitating movement of intestinal contents (neuron types colored as in A, with secretomotor neurons additionally depicted in orange). LM, longitudinal muscle; MP, myenteric plexus; CM, circular muscle; SMP, submucosal plexus; MM, muscularis mucosa; MUC, mucosa (of the small intestine — Mucosa of the large intestine is not organized into villi [34]). Adapted by permission from Springer Nature: Springer, The Enteric Nervous System and Gastrointestinal Innervation: Integrated Local and Central Control by Furness et al. © Springer New York 2014 [18] and from Progress in Neurobiology, 72, Furness et al., Intrinsic primary afferent neurons and nerve circuits within the intestine, 143-164 © 2003 Elsevier Ltd [43], with permission from Elsevier Ltd.
![Interactions between intestinal enteric neurons. A) Simplified representation of enteric neuronal circuits directly governing intestinal motility, which involve both myenteric and submucosal neurons. B) Simplified representation of the enteric neuronal interactions directly and indirectly facilitating movement of intestinal contents (neuron types colored as in A, with secretomotor neurons additionally depicted in orange). LM, longitudinal muscle; MP, myenteric plexus; CM, circular muscle; SMP, submucosal plexus; MM, muscularis mucosa; MUC, mucosa (of the small intestine — Mucosa of the large intestine is not organized into villi [34]). Adapted by permission from Springer Nature: Springer, The Enteric Nervous System and Gastrointestinal Innervation: Integrated Local and Central Control by Furness et al. © Springer New York 2014 [18] and from Progress in Neurobiology, 72, Furness et al., Intrinsic primary afferent neurons and nerve circuits within the intestine, 143-164 © 2003 Elsevier Ltd [43], with permission from Elsevier Ltd.](https://content.iospress.com:443/media/jpd/2022/12-6/jpd-12-6-jpd223262/jpd-12-jpd223262-g002.jpg)
Collectively, the complex interplay between various types of myenteric and submucosal neurons underpin secretory and motility reflexes of the intestine [18, 35, 42]. These interrelated reflexes allow intestinal contents to be easily transported (orally to anally) at a speed appropriate for optimal nutrient and water absorption. When intestinal transit is slowed and liquidity of the intestinal contents lowered due to suppression of enteric neuronal activity, increased absorption and inhibited secretion of water and electrolytes results in drier-harder stools and associated constipation [44]. Therefore, if enteric neurons are lost from either plexus in PD patients, this could similarly compromise the timely execution and coordination of reflexes governing movement of intestinal contents, and this may give rise to PD-related constipation. Constipation in patients with severe gut dysmotility correlates with loss of both myenteric and submucosal neurons in the small intestine [45], and neurons in both the myenteric and submucosal plexus are significantly reduced in the colon of patients with slow-transit constipation [46]. Several other conditions involving substantially impaired GI motility are also associated with enteric neuron loss, including Chagas’ disease [47, 48], achalasia and chronic intestinal pseudo-obstruction [31].
INTESTINAL ENTERIC NEURON LOSS IN PARKINSON’S DISEASE
A summary of key results from studies assessing intestinal enteric neuron loss in PD patients is provided in Table 1. This table is intended to provide a general overview of relevant study findings, but the supplementary tables contain the level of detail required to accurately compare and critique these studies.
Table 1
Summary of key results from studies assessing intestinal enteric neuron loss in PD patients
Study reference | Region of the intestine examinedA | Subjects assessedB | PD-related enteric neuron lossC in the intestinal region? | Correlation between intestinal enteric neuron loss and constipationD? |
Lebouvier et al. [49] | Colon (ascending) | 5 PD 3 CC 5 control | SMP: No MP: Not assessed | No correlations performed |
Lebouvier et al. [50] | Colon (ascending, descending) | 29 PD 10 control | SMP: Yes MP: Not assessed | No |
Annerino et al. [51] | Duodenum, colon (transverse) | 13 PD 12 control | SMP: Not assessed MP: No | Constipation not assessed |
Barrenschee et al. [52] | Upper dorsal rectal wall | 12 PD 11* control | SMP: No MP: Not assessed | No relevant correlation performed |
Desmet et al. [53] | Second part of the duodenum | 15 PD 15∧ control | SMP: No MP: Not assessed | Constipation not specifically assessed |
Giancola et al. [54] | Colon (descending) | 29# PD 10 CC 20 control | SMP: No MP: Not assessed | No relevant correlation performed |
PD, PD patients; CC, constipated controls; control, healthy and/or non-constipated controls; SMP, submucosal plexus; MP, myenteric plexus. *10/∧14/#15 included in neuronal quantity analysis. AFor information on tissue collection and tissue processing in each study, refer to Supplementary Table 1. BFor detailed demographics of the PD patients evaluated in each study, refer to Supplementary Table 2. CFor detailed information on how enteric neuron loss was assessed in each study, refer to Supplementary Table 1. Correlations between PD patient demographics and intestinal enteric neuron quantities in each study are presented in Supplementary Table 4. DFor detailed information on how constipation presence and/or severity was assessed in the relevant studies, refer to Supplementary Table 3. Correlations between PD patient demographics and constipation severity score in the relevant studies are presented in Supplementary Table 5. Differences in the descriptive statistics, p-values and individual participant data provided by each study, in addition to the types of statistical tests performed, are detailed in Supplementary Table 6.
Small intestine
The small intestine has three divisions: the duodenum, the jejunum, and the ileum [34]. In addition to different anatomical positions and subtle structural differences, each division includes a unique combination of glands, lymphoid follicles and neurovasculature [34, 55, 56]. In light of this, enteric neuron loss in each region of the small intestine should be considered separately.
Duodenum
No enteric neuron loss has so far been discovered in the myenteric plexus [51] nor the submucosal plexus [53] of the duodenum in PD patients relative to controls (Fig. 3A; Supplementary Table 1, D and G). However, over 50% of patients recruited into the study by Desmet and colleagues [53], which investigated PD-related submucosal neuron loss in the duodenum, had early-onset PD (onset before age 50 [57–60]) (Supplementary Table 2). Early-onset PD accounts for approximately 10–20% of all PD cases [59] and is commonly associated with mutations in specific genes, including DJ-1 (PARK7), PINK1 (PARK6), and Parkin (PARK2) [61, 62]. Thus, although it is not synonymous with familial PD, early-onset PD is often familial in nature. Therefore, Desmet et al. [53] genetically analyzed the Parkin (PARK2) gene of 5/6 patients with disease onset at or before age 45, but did not discover any mutations. However, this study did not additionally screen for familial PD-linked mutations in DJ-1, PINK1 or other genes— these mutations may have been present in the early-onset patients examined, and perhaps contributed to a profile of symptoms and intestinal neuropathology different from idiopathic PD [53, 59]. Only patients with idiopathic PD were analyzed in the study estimating constipation to precede clinical diagnosis by over 17 years [29], and early-onset cases do not appear to have been analyzed. It is also unclear whether the prior systematic review and meta-analysis of constipation preceding PD motor symptoms [28] is applicable to early-onset and/or familial PD. To our knowledge, the incidence of constipation specifically among patients with familial and/or early-onset PD has not been explored. Therefore, the lack of submucosal neuron loss in patients studied by Desmet et al. [52] may not be applicable to the constipated PD population (Table 1).
Jejunum
Ohlsson and Englund [63] examined samples of jejunum and colon from PD patients and controls. The pilot study reported the presence of atrophic/pyknotic neurons in 15/20 PD patients, either within the submucosal plexus (10/15), myenteric plexus (13/15) or both (9/15), and found no evidence of degenerating neurons in controls [63]. However, it is unclear from this report whether the neuronal degeneration observed in PD patient tissue was identified in samples of jejunum, colon or both. Furthermore, there was no quantitation of intestinal enteric neurons in this study, and thereby no comparison of enteric neuron quantities between PD patients and controls. Consequently, whether or not there is appreciable loss of submucosal or myenteric neurons within the jejunum in PD remains an open question (Supplementary Table 1I).
Large intestine
The large intestine includes the caecum, appendix, colon, rectum and anal canal [34], and the colon has four subdivisions: the ascending colon, the transverse colon, the descending colon and the sigmoid colon [34]. Each colonic region has a distinct amalgam of arterial blood supply, venous blood drainage, innervation and lymphatics, in addition to specific anatomical positions and unique relations to other abdominal structures [64]. Accordingly, it is best to consider enteric neuron loss in each subdivision of the colon separately, where possible.
Colon and rectum
Several colonic regions have been examined for enteric neuron loss in PD patients [49–51, 54] (Fig. 3B–D; Supplementary Table 1, B–D and H). One study found a significant 14% decrease in the mean number of colonic submucosal neurons per ganglion, averaged from one ascending colon sample and one descending colon sample per subject, in PD patients compared to controls [50] (Fig. 3C; Supplementary Table 1C,). A subsequent study found no change in the mean percentage of total colonic neurons that were positive for tyrosine hydroxylase (TH), a marker of catecholaminergic (dopaminergic and noradrenergic) neurons, in the submucosal plexus of PD patients relative to controls [65] (Supplementary Table 1E,). It is important to consider that if catecholaminergic and other neuronal subtypes within the submucosal plexus were lost in equal amounts, the relative proportion of submucosal catecholaminergic neurons to total submucosal neurons would remain the same. Therefore, the results of these two studies [50, 65] together indicate that there may be proportional loss of catecholaminergic neurons and other types of enteric neurons within the human colon, the majority of which are cholinergic [54, 66], from the colonic submucosa in PD.
However, another study compared the mean number of submucosal neurons per ganglion in the descending colon of constipated PD patients, constipated individuals without PD (constipated controls) and control subjects without constipation (healthy controls), and did not find any significant difference between these groups [54] (Fig. 3D; Supplementary Table 1H,). The study by Annerino and colleagues [51] also failed to find myenteric neuron loss in the transverse colon of PD patients relative to controls (Supplementary Table 1D,). Nonetheless, neither of these studies [51, 54] examined enteric neurons within the ascending colon— the study which discovered enteric neuron loss in the colon of PD patients [50] collectively evaluated submucosal neuron quantities across the ascending and descending colon. Additionally, Giancola et al. [54] derived mean submucosal neurons per ganglion from a high proportion of patients with early PD (diagnosed with PD for less than 5 years [67, 68]) relative to Lebouvier et al. [50] (Supplementary Table 2). As it is challenging to distinguish between PD and related conditions within the first 5 years of PD-like symptoms [67, 69, 70], a considerable proportion of patients in the study that did not discover colonic neuron loss [54] may have had a condition other than PD. Indeed, the diagnostic criteria for clinically established PD [3, 68], approximate to earlier definitions of definite PD [71] and probable PD [72], are very difficult for early PD patients to satisfy [68, 71, 72] (Supplementary Table 2). Therefore, it may be the case that patients with clinically established PD experience neuron loss in the colonic submucosal plexus. This loss may be isolated to the ascending and descending subdivisions, although the study which investigated PD-related neuron loss in the transverse colon [51] exclusively examined the myenteric plexus. Interestingly, a pilot study which only included patients diagnosed with PD for over 5 years [49] did not find submucosal neuron loss in the ascending colon of subjectively constipated PD patients relative to both healthy and constipated controls, respectively (Fig. 3B; Supplementary Table 1B,). However, it has been suggested that these results may lack validity due to the very small number of subjects examined [50]. A study of the rectum [52] failed to find submucosal neuron loss in PD patients relative to controls (Fig. 3E; Supplementary Table 1F,).
Fig. 3
Comparisons of mean intestinal enteric neuron quantities between PD and control groups. A) Enteric neurons in the submucosal plexus of the duodenum were detected via immunostaining for HuC/HuD (HuC/D) and neurofilament (NF). Modified from [46] Copyright Desmet et al., eLife article distributed under the terms of the Creative Commons Attribution License (https://creativecommons.org/licenses/by/4.0). B) Enteric neurons in the submucosal plexus of the ascending colon were detected via immunostaining for HuC/D. Adapted by permission from BMJ Publishing Group Limited. Pathological lesions in colonic biopsies during Parkinson’s disease, Lebouvier et al., Gut 57:1741-1743 © 2008 [49]. C) Enteric neurons in the submucosal plexus of the ascending + descending colon were detected via immunostaining for NF. Modified from [50] © 2010 Lebouvier et al., PLoS ONE article distributed under the terms of the Creative Commons Attribution License (https://creativecommons.org/licenses/by/4.0). D) Enteric neurons in the submucosal plexus of the descending colon were detected via immunostaining for HuC/D. Adapted by permission from John Wiley and Sons: Neurogastroenterology & Motility, 29, Giancola et al., Downregulation of neuronal vasoactive intestinal polypeptide in Parkinson’s disease and chronic constipation, e12995 © 2016 John Wiley & Sons Ltd [54]. E) Enteric neurons in the submucosal plexus of the rectum were detected via immunostaining for protein gene product 9.5 (PGP 9.5). Modified from [52] © 2017 Barrenschee et al., Acta Neuropathologica Communications article distributed under the terms of the Creative Commons Attribution 4.0 International License (https://creativecommons.org/licenses/by/4.0). Annerino et al. [51] detected enteric neurons in the myenteric plexus of the duodenum and transverse colon via immunostaining for HuC/D, then compared mean myenteric neuron density (neurons/mm2) between PD patients and controls (not shown). PD, PD patients; CC, constipated control subjects; control, healthy and/or non-constipated control subjects. *p < 0.05 as compared with control.
![Comparisons of mean intestinal enteric neuron quantities between PD and control groups. A) Enteric neurons in the submucosal plexus of the duodenum were detected via immunostaining for HuC/HuD (HuC/D) and neurofilament (NF). Modified from [46] Copyright Desmet et al., eLife article distributed under the terms of the Creative Commons Attribution License (https://creativecommons.org/licenses/by/4.0). B) Enteric neurons in the submucosal plexus of the ascending colon were detected via immunostaining for HuC/D. Adapted by permission from BMJ Publishing Group Limited. Pathological lesions in colonic biopsies during Parkinson’s disease, Lebouvier et al., Gut 57:1741-1743 © 2008 [49]. C) Enteric neurons in the submucosal plexus of the ascending + descending colon were detected via immunostaining for NF. Modified from [50] © 2010 Lebouvier et al., PLoS ONE article distributed under the terms of the Creative Commons Attribution License (https://creativecommons.org/licenses/by/4.0). D) Enteric neurons in the submucosal plexus of the descending colon were detected via immunostaining for HuC/D. Adapted by permission from John Wiley and Sons: Neurogastroenterology & Motility, 29, Giancola et al., Downregulation of neuronal vasoactive intestinal polypeptide in Parkinson’s disease and chronic constipation, e12995 © 2016 John Wiley & Sons Ltd [54]. E) Enteric neurons in the submucosal plexus of the rectum were detected via immunostaining for protein gene product 9.5 (PGP 9.5). Modified from [52] © 2017 Barrenschee et al., Acta Neuropathologica Communications article distributed under the terms of the Creative Commons Attribution 4.0 International License (https://creativecommons.org/licenses/by/4.0). Annerino et al. [51] detected enteric neurons in the myenteric plexus of the duodenum and transverse colon via immunostaining for HuC/D, then compared mean myenteric neuron density (neurons/mm2) between PD patients and controls (not shown). PD, PD patients; CC, constipated control subjects; control, healthy and/or non-constipated control subjects. *p < 0.05 as compared with control.](https://content.iospress.com:443/media/jpd/2022/12-6/jpd-12-6-jpd223262/jpd-12-jpd223262-g003.jpg)
Before continuing, it is worth noting that Singaram et al. [73] examined neurons of the submucosal and myenteric plexuses within the ascending colon of severely constipated PD patients, intractably constipated individuals without PD (constipated controls) and control subjects without constipation (healthy controls), and reported no significant difference between groups in the percentage of neurons positive for vasoactive intestinal peptide (VIP) nor TH in either plexus (Supplementary Table 1A). Although this study evaluated the total number of submucosal and myenteric neurons in the ascending colon of each subject via staining for protein gene product 9.5 (PGP 9.5) [73], whether or not there was a significant difference in the total number of these neurons between any of the groups assessed was not reported. Additionally, as is the case with Corbillé et al. [65], a lack of significant change in the proportion of TH-positive neurons does not necessarily reflect a lack of significant change in the number of TH-positive nor total neurons, as catecholaminergic and other neuronal subtypes (including VIP-positive neurons) may have been lost in proportionate amounts.
Singaram and colleagues [73] also concluded that their severely constipated PD patients had a reduced proportion of myenteric dopaminergic neurons within the ascending colon relative to constipated and healthy controls. This conclusion was based on the findings of immunohistochemical staining for dopamine [73], which is not a validated method for the in situ identification of dopaminergic neurons [51, 65]. The results of TH immunohistochemistry, a technique which is commonly used to identify noradrenergic and dopaminergic neurons in situ, did not indicate a significant reduction in the percentage of myenteric catecholaminergic neurons within the ascending colon of PD patients [73]. Therefore, others have suggested that the reduced proportion of dopaminergic neurons in the myenteric plexus of these patients was artifactual [51, 65]. Artifacts are artificial structures or tissue alterations that result from extraneous factors, including tissue sampling and processing procedures [74], and complicate the histomorphological evaluation of intestinal tissues. As some PD patients in Singaram et al. [73], but no control subjects, had colonic tissue sampled at autopsy (Supplementary Table 1), postmortem artefacts [75] may have been observed exclusively in the PD patient tissue. However, it is also worth noting that the duration and severity of PD in the patients examined [73] far surpassed that of patients in other studies aimed at investigating PD-related intestinal enteric neuron loss (Supplementary Table 2).
Clinically established PD-related neuron loss in the colonic submucosal plexus
Overall, the results of the studies explored above may together be consistent with proportional loss of neuronal subtypes (catecholaminergic, VIPergic, cholinergic and potentially others) in the colonic submucosal plexus of patients with clinically established PD (Supplementary Tables 1 and 2). Whether there is enteric neuron loss within the rectal myenteric plexus, the ileum, caecum, appendix, anal canal, sigmoid colon, myenteric plexus of the descending colon or submucosal plexus of the transverse colon in PD has yet to be investigated.
CONSTIPATION IN PARKINSON’S DISEASE
Intestinal dysfunction (colonic and/or anorectal dysfunction) gives rise to PD-related constipation
There are three types of constipation commonly encountered in PD patients— slow transit constipation caused by prolonged CTT [16, 23, 76], outlet obstruction, sometimes termed ‘defecatory dysfunction’, due to uncoordinated (dyssynergic) contraction or insufficient relaxation of the puborectalis muscle and anal sphincters during defecation [16, 23, 76] and, most commonly, a combination of both [23]. Objective measures of colonic and anorectal dysfunction are able to distinguish between these subtypes— CTT can be measured via retention of radiopaque markers (ROM) or a wireless motility capsule in the colon [23, 25, 76], while contractility of the anal sphincters, rectal sensory function and recto-anal reflexes can be evaluated using anorectal manometry (ARM) [23, 54, 77]. The state of the puborectalis muscle during defecation attempts can also be discerned via magnetic resonance (MR) imaging (MR defecography) [23, 77]. However, constipation criteria and related questionnaires are more widely utilized by researchers to evaluate PD-related constipation than these objective measures [25]. This is mainly because it has been presumed that less than three bowel movements per week, a common constipation criterion, is reflective of slowed CTT [16, 76] and that anorectal symptoms, such as straining during defecation and sensation of incomplete bowel emptying after defecation (other common constipation criteria), are due to outlet obstruction [16, 76]. For example, the Rome III Criteria for Functional Constipation [78] has frequently been used to diagnose constipation in studies involving PD patients [30, 50, 54, 79–81], and many studies involving PD patients have used the sum of positive responses to constipation items on a related questionnaire, the Rome III Diagnostic Questionnaire for Adult Functional Gastrointestinal Disorders [82], as a semi-quantitative assessment of constipation severity [50, 54, 79–81, 83].
Constipation items on PD questionnaires do not appropriately evaluate intestinal dysfunction
Summing the positive responses to Rome III constipation items is not a validated method of scoring constipation severity [50, 81], nor has the Rome III constipation criteria and related questionnaire themselves been appropriately validated for use in PD research [25]. Nonetheless, it is understandable why several studies involving PD patients utilized either 6 or 7 Rome III constipation items to derive a constipation severity score— all the non-motor symptom questionnaires validated for use in PD patients [84–87] include no more than 1 or 2 items relevant to constipation (Fig. 4), and these do not evaluate colonic and anorectal dysfunction in the same detail as the relevant Rome III items [25]. For example, the Non-Motor Symptoms Scale (NMSS) [84] defines constipation as less than three bowel movements per week (Fig. 4A), which, although thought to reflect prolonged CTT, fails to account for the anorectal symptoms of constipation prominent in PD. Furthermore, definitions of constipation based solely on bowel movement frequency are known to be unreliable [81, 88] and, if reliant on subjects remembering their weekly number of bowel movements, are particularly prone to recall bias [89]. The Non-Motor Symptoms Questionnaire (NMSQuest) [85] and SCOPA-AUT (SCales for Outcomes in PArkinson’s disease— AUTonmic dysfunction) [87] both enquire about the need to strain during defecation, in addition to whether a patient experiences less than three bowel movements per week [85, 87] (Fig. 4B, C). However, only the latter is again utilized as the constipation definition, whereas straining is distinguished from constipation— the reason for this is unclear. Although, the NMSQuest employs a single question to determine if a patient has experienced constipation or straining within the past month [85], which seems to undermine its exclusion of straining from the constipation definition. The NMSQuest additionally appraises sensation of incomplete bowel emptying following defecation (Question 7) [85], but the SCOPA-AUT does not include an equivalent question. Finally, the constipation item on the Unified Parkinson’s Disease Rating Scale (MDS-UPDRS (I), item 11) [86] appears to initially define constipation as bowel movement difficulty, but the descriptions of each constipation severity include inconsistent details pertaining to level of defecation effort, discomfort, activity disturbance, and need for physical help (Fig. 4D)— this may lead to confusion among patients attempting to define their constipation severity. In contrast to constipation items on the SCOPA-AUT, NMSQuest, NMSS, and MDS-UPDRS (I), the Rome III constipation items [78, 90] examine frequency, and thus severity, of the inability to relax the anorectum or the sensation of anorectal blockage, the need for manual maneuvers to facilitate defecation and the occurrence of hard or lumpy stools, in addition to abnormally reduced bowel movements, straining during defecation and sensation of incomplete bowel emptying following defecation [78, 90].
Fig. 4
Constipation assessment items on each PD non-motor symptom questionnaire. A) The question assessing constipation on the Non-Motor Symptoms Scale (NMSS) for PD patients. This figure incorporates the NMSS, which is owned and licensed by the International Parkinson and Movement Disorder Society (MDS). Permission to reproduce the NMSS in this figure was granted by MDS, the copyright holder. Copyright © 2007 International Parkinson and Movement Disorder Society (MDS). All Rights Reserved. B) The question/s assessing constipation on the Non-Motor Symptoms Questionnaire (NMSQuest/NMSQ) for PD patients. This figure incorporates the NMSQ, which is owned and licensed by the International Parkinson and Movement Disorder Society (MDS). Permission to reproduce the NMSQ in this figure was granted by MDS, the copyright holder. Copyright © 2006 International Parkinson and Movement Disorder Society (MDS). All Rights Reserved. C) The question/s assessing constipation on the SCOPA-AUT (SCales for Outcomes in PArkinson’s disease — AUTonmic dysfunction). This figure incorporates the SCOPA-AUT, which is owned and licensed by the International Parkinson and Movement Disorder Society (MDS). Permission to reproduce the SCOPA-AUT in this figure was granted by MDS, the copyright holder. Copyright © 2019 International Parkinson and Movement Disorder Society (MDS). All Rights Reserved. D) The item assessing constipation on the Unified Parkinson’s Disease Rating Scale (MDS-UPDRS). This figure incorporates the MDS-UPDRS, which is owned and licensed by the International Parkinson and Movement Disorder Society (MDS). Permission to reproduce the MDS-UPDRS in this figure was granted by MDS, the copyright holder. Copyright © 2008 International Parkinson and Movement Disorder Society (MDS). All Rights Reserved.
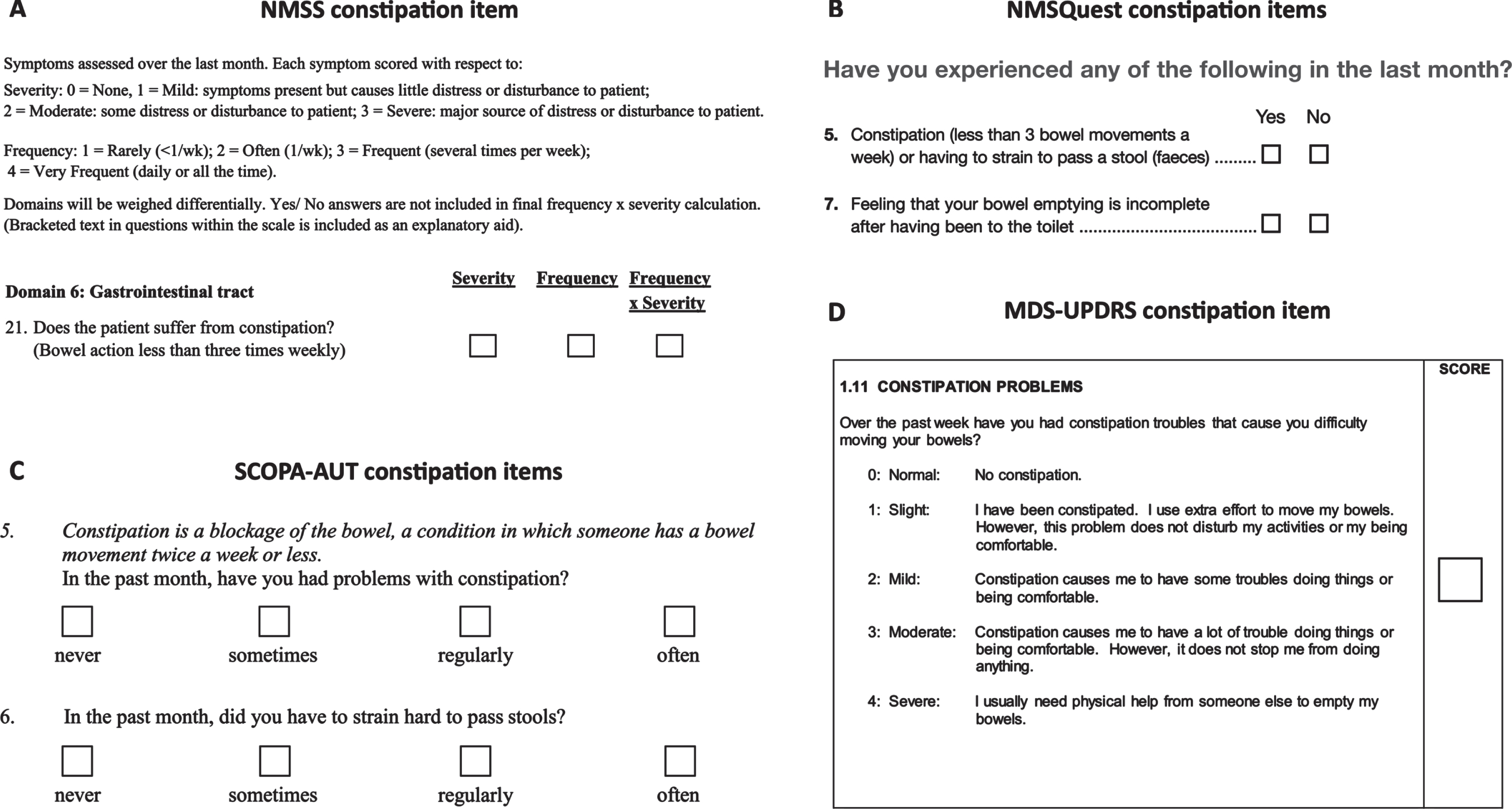
Constipation Scoring System survey results correlate with colonic and anorectal dysfunction in PD
Similar to the Rome III constipation items, the Constipation Scoring System (CSS) questionnaire provides a comprehensive assessment of constipation [88], and has occasionally been utilized in studies involving PD patients [52, 91, 92]. This survey consists of 8 questions (scored on a scale of 0–4, bar one) associated with different aspects of constipation, including bowel movement frequency, painful defecation effort, and sensation of incomplete bowel emptying after defecation [88]. The resulting CSS score has been shown to correlate well with objective measures of colonic and anorectal dysfunction in constipated patients [88], including the results of ARM, CTT, and cinedefectography, a predecessor of MR defecography [93]. Although the CSS score is not validated for use in PD research [81], one study found that objective indicators of PD-related constipation were only completely corroborated by the CSS score, relative to the sum of positive responses to Rome III constipation items and both NMSQuest Question 5 (<3 bowel movements per week or straining during defecation) and 7 (sensation of incomplete bowel emptying following defecation) [81].Specifically, PD patients had a significantly higher total number of retained ROM in the colon, indicative of prolonged CTT, and a significantly larger total colonic volume, predominantly due to transverse colon and rectosigmoid region volume increases (suggestive of co-occurring outlet obstruction) [81]. When the different constipation assessments were compared with these objective measures, all three (NMSQuest Questions 5 and 7, the sum of positive responses to Rome III constipation items and the CSS score) correlated with total colonic volume, but only the CSS score correlated significantly with total colonic ROM (CTT) [81].
Correlations between any constipation items/criteria and intestinal dysfunction are weak in PD
However, the significant correlation between CSS score and total colonic ROM was weak (R2 = 0.19), as was the significant correlation between total colonic volume and both the CSS score (R2 = 0.34) and the sum of positive responses to Rome III constipation items (R2 = 0.33) [81]. Although the significant correlations between Questions 5 and 7 of the NMSQuest and total colonic volume were moderate (r = 0.504 and r = 0.538, respectively) [81], the strength of these correlations appear to be due to an outlier (SupplementaryFigure 1). This lack of strong correlations between quantitative indicators of PD-related constipation and constipation as defined by various questionnaires, validated for use in PD research or otherwise, reflects an overarching issue: objectively evaluated colonic abnormalities and criteria-defined constipation are weakly related. The prevalence of constipation among PD patients is known to vary substantially between studies [24, 25], but this was thought to be due mainly to variable definitions of constipation being utilized across studies [25, 94]. However, the comparative results of two recent studies demonstrate that, in PD patients with the same prevalence of prolonged CTT (79% vs. 78%, the prevalence of constipation diagnosed by identical criteria still varies greatly (32% vs. 100% [54, 81].
Intestinal dysfunction occurs in the majority of PD patients and must be objectively evaluated
In contrast to the apparent inconsistency of criteria-based constipation assessments, CTT is significantly prolonged in PD patients relative to controls [81, 95–98] or normal values [99] in most cases. Anorectal dysfunction results among PD patients appear more variable [95, 98, 100, 101], but delayed colonic transit plus impaired anorectal function seems to be the most common finding in PD patients who match constipation criteria or believe they do (60–65%, relative to anorectal dysfunction only (13–26.7%, isolated slow transit (6.7–13% or no transit and anorectal impairment (6.7–9% [54, 77]. More broadly, intestinal dysfunction seems to occur in the majority of PD patients, both when the majority match constipation criteria or believe they do [54, 77, 98, 99] and when the majority do not [81, 97]. Therefore, it is clear that constipation criteria and related questionnaire items do not reliably assess characteristics of slow transit constipation nor outlet obstruction, and thus cannot reliably identify the types of constipation encountered in PD patients.
RELATIONSHIP BETWEEN COLONIC ENTERIC NEURON LOSS AND PD-RELATED CONSTIPATION
Each of the studies that specifically investigated submucosal neuron loss in the colon or rectum of PD patients [49, 50, 52, 54] also assessed which participants exhibited constipation [50, 52], or exclusively recruited patients who were constipated to compare with constipated and healthy (non-constipated) controls [49, 54] (Supplementary Table 3).
Both Lebouvier et al. [50] and Giancola et al. [54] used the Rome III Criteria for Functional Constipation [78] to investigate constipation in their studies— the former study diagnosed constipation in 23/29 PD patients and 1/10 control subjects according to these criteria [50], while the latter utilized fulfilment of the criteria to guide recruitment of constipated individuals with and without PD, as well as non-constipated controls [54]. Lebouvier and colleagues [50] additionally employed the number of positive responses to 6 constipation items from the Rome III Diagnostic Questionnaire for Adult Functional Gastrointestinal Disorders [82] as a semi-quantitative assessment of constipation severity. Giancola and colleagues [54] included a correlation involving the severity of Rome III-criteria defined constipation in PD (on a scale of 0–6) in their supporting results (Supplementary Figure 2). However, as explored earlier, summing the positive responses to constipation items on the relevant Rome III questionnaire has not been validated as a means of scoring constipation severity [50, 81]. More importantly, a constipation diagnosis based on subjective criteria or related questionnaire items does not reliably correspond with prolonged CTT nor anorectal abnormalities, which objectively define the types of constipation encountered in PD patients [16, 23, 76]. The results of both studies [50, 54] must be considered in light of these limitations.
Lebouvier et al. [50] found no correlation between the number of neurons per ganglion in the colon and constipation severity [50] (Supplementary Table 3B,). However, this does not exclude the possibility that the colonic submucosal neuron loss discovered by this study contributed to colonic dysfunction in the PD patients examined, as colonic function was not objectively evaluated. Giancola et al. [54] did not report a relationship between the mean number of submucosal neurons per ganglion within the descending colon and the presence of Rome III criteria-defined constipation, likely because there was no significant difference in this mean between their PD patients, constipated controls and healthy controls [54] (Supplementary Table 3C,). However, this result does not rule out a connection between the mean number of submucosal neurons per ganglion in the descending colon and colonic function. Fortunately, Giancola et al. [54] objectively evaluated colonic function in PD patients via CTT assessment, in addition to examining their anal and rectal function using ARM [54]. In the subset of PD patients who underwent both ARM and CTT assessment, 78% were found to experience colonic dysfunction (prolonged CTT) and 65% additionally experienced anorectal dysfunction (ARM abnormalities) [54]. However, this study did not seek to correlate the mean number of submucosal neurons per ganglion in the descending colon with the mean number of residual intracolonic ROM within the PD group, likely because these means were derived from different, though partially overlapping, subsets of PD patients [54, 102].
Giancola and colleagues [54] also reported no significant difference in the mean number of residual intracolonic ROM between the 23 PD patients and the 9 constipated controls who completed both CTT evaluation and ARM [54]. However, 55% of the patients with known disease duration included in this analysis (12/22) had been diagnosed with PD for less than 5 years (Supplementary Table 2). Therefore, the appreciable misdiagnosis rate among early PD patients [67, 69, 70] may have contributed to this finding, such that it may not be applicable to the PD population. Assuming the patients with early PD were accurately diagnosed, the lack of significant difference in mean CTT between PD patients and constipated controls [54] still may not be generalizable to patients with clinically established PD [3, 68] (similar to probable PD [72] or definite PD [71] in earlier diagnostic criteria), who constitute the majority of patients examined in other studies of PD-related intestinal enteric neuron loss (Supplementary Table 2). Indeed, the amount of residual intracolonic ROM was positively correlated with PD duration (spanning 1–10 years) in patients who had their CTT evaluated by Giancola et al. [54]— this could reflect an increase in the severity of PD-related constipation with years since PD diagnosis, or with increasing diagnostic accuracy, but this correlation should be interpreted with caution (Supplementary Figure 2).
The study that examined the ascending colon of subjectively constipated PD patients, all of whom had been diagnosed with PD for over 5 years, did not report a relationship between the mean number of submucosal neurons per ganglion and the presence of constipation [49] (Supplementary Table 3A,). However, the PD patients assessed in this study were not formally diagnosed with constipation [49], and the intractably constipated controls may have been constipated due to isolated outlet obstruction or other issues not directly related to colonic enteric neuropathy. Others have also suggested that the very small number of participants examined in this pilot study limit the validity of its findings [50].
One study examined both rectal submucosal neuron density and constipation severity of PD patients and control subjects [52]. Although no correlation between these variables was reported, neither mean neuron density within the submucosal plexus of the rectum nor mean constipation severity score (CSS score) differed significantly between the PD and control groups [52] (Supplementary Table 3D,). However, it is unclear whether any participant was ‘constipated’ according to their CSS score, and constipation was not objectively evaluated in any PD patient nor control subject [52]. Furthermore, the control group may have consisted of both constipated and non-constipated individuals, as rectal tissue from some control subjects was obtained during colonoscopy for GI symptoms [52], whereas the presence of existing GI issues in the PD patients examined was not described. Regardless, constipation in any participant assessed may have been related to enteric neuropathy within the colon, rather than enteric neuron loss in the rectum.
LIMITATIONS, DISPARITIES AND SUGGESTIONS FOR FUTURE STUDIES
The main limitations of the reviewed studies and relevant recommendations for future research are summarized in Table 2.
Table 2
Main limitations of the reviewed studies and suggestions to improve future research
Limitations of reviewed studies | Suggestions for future research |
Small sample sizes impede applicability of intestinal enteric neuron loss results to the PD population | Sufficiently large patient and control samples should be utilized. |
Recruitment/over-representation of early and early-onset PD patients may have confounded studies’ results | Recruitment criteria should ensure patient samples are representative of the PD population (majority diagnosed with idiopathic PD for at least 5 years) |
Differences in intestinal enteric neuron loss results between studies may be influenced by PD heterogeneity | The factors currently believed to contribute to PD heterogeneity, including particular genetic variants, RNA expression patterns, pesticide exposures and sleep disturbances, should be additionally assessed. |
Incomplete reporting and different outcome definitions limit ability to perform a meta-analysis/compare study results and draw accurate conclusions | Individual participant results and/or relevant descriptive statistics, p-values and details of statistical tests should be provided. Study outcomes must be defined and analysed in the same manner across studies. Specifically: |
1) A consistent neuronal quantification method and study design (including standardized statistical analyses) should be implemented. | |
2) Constipation should be assessed via objective measures of colonic transit and anorectal function. | |
Furthermore, complete data and methodological transparency would enhance the validity of any future retrospective analysis. |
Small sample sizes impede applicability of intestinal enteric neuron loss results to the PD population
The majority of studies that compared intestinal enteric neuron quantities between PD patients and controls derived these quantities from 15 or fewer participants per group (Supplementary Table 1), which is typically considered too few for findings to be generalizable [103, 104]. The small sample sizes utilized by the reviewed studies therefore limited their ability to detect any real PD-related change in intestinal enteric neuron quantities, and any relationship between constipation and intestinal enteric neuron loss [103]. This also limited the power of any correlation analyzes performed (Supplementary Tables 1 and 3), including correlations run between PD patient demographics and intestinal enteric neuron quantities (Supplementary Table 4) or constipation severity scores (Supplementary Table 5). Although the only study to report a significant PD-related reduction in total intestinal enteric neurons evaluated 29 patients [50], their results were compared against only 10 controls [103, 104]. Future studies must therefore utilize larger sample sizes if their PD-related intestinal enteric neuron loss results are to be confidently generalized to the patient population. Although the exact sample sizes required should be estimated via a priori power analyzes [104], PD and control groups of at least n = 30 could be an ideal place to start.
Incomplete reporting and different outcome definitions limit ability to perform a meta-analysis
When several studies with small samples seek to answer the same question, it is not unusual for researchers to perform a meta-analysis on the quantitative findings from each study to derive conclusions with higher power [105–107]. Unfortunately, individual participant results and/or relevant descriptive statistics, p-values and details of statistical tests were not consistently reported across the studies that sought to assess intestinal enteric neuron loss in PD (Supplementary Table 6), limiting the ability to perform a meta-analysis [108]. It is also difficult to perform a meta-analysis when the same outcome is treated or analyzed differently across studies [108], as well as examining different regions of the intestine (Table 1), mean neurons per ganglion was quantified differently in each reviewed study and neuronal density was instead quantified in some studies (Supplementary Table 1). Additionally, the constipation assessments utilized were not consistent across relevant studies and thus the same outcome (constipation) was defined differently (Supplementary Table 3). Future studies should implement a consistent neuronal quantification method and study design (including standardized statistical analyzes, where possible) and diagnose constipation via objective measures of colonic transit and anorectal function [16, 23, 76] rather than using constipation criteria or related questionnaire items.
Recruitment/over-representation of early and early-onset PD patients may have confounded studies’ results
The studies that sought to evaluate intestinal enteric neuron loss in PD utilized different recruitment criteria for both patients and control subjects (Supplementary Tables 1 and 3). Furthermore, the proportion of recruited patients with early PD (diagnosed with PD for less than 5 years [67, 68]), early-onset PD (onset before age 50 [57–60]), and severe PD (Hoehn and Yahr Stage 4 or greater [109, 110], and perhaps also indicated by a dopa-responsiveness ≤30% [3, 50], UPDRS-III score ≥45 [111]/MDS-UPDRS (III) score ≥59 [86, 112] or a UPDRS-III axial score ≥21 [50, 111]) varied between studies (Supplementary Table 2), and over 50% of patients in some studies had early PD [54] or early-onset PD [53] (Supplementary Table 2). PD onset age, duration and severity may independently or jointly influence intestinal dysfunction and neuropathology [113–116]. Furthermore, given early-onset PD accounts for only 10–20% of PD cases [59] and might not be associated with constipation, while patients with early PD have appreciable rates of misdiagnosis [67, 69, 70], these patient groups should not be over-represented. Recruitment criteria utilized in future investigations should ensure patient samples are representative of the PD population (majority diagnosed with idiopathic PD for at least 5 years).
Discrepancies between colonic enteric neuron loss results may instead be due to PD heterogeneity
Only one of the three studies that specifically investigated enteric neuron quantities in the colon discovered PD-related submucosal neuron loss [50] (Supplementary Table 1, B, C and H). The validity of preliminary ascending colon results from Lebouvier et al. [49] has been questioned in their follow-up study [50], while the lack of descending colon submucosal neuron loss in Giancola et al. [54] may be explained by the disproportionate inclusion of potentially misdiagnosed (early PD) patients [67, 69, 70]. However, assuming the majority of early PD patients evaluated by Giancola and colleagues [54] were not misdiagnosed, and recognizing that constipation can predate PD diagnosis by over a decade [28, 29], it could be argued that significant enteric neuron loss should be present in the colon of these patients, if such loss were a main contributor to PD-related constipation. In this case, the absence of PD-related submucosal neuron loss found [54] would contradict the significant submucosal neuron loss in PD patients observed by Lebouvier and colleagues [50], if the latter were due to substantial neuron reductions in the descending (or both ascending and descending) colon (Table 1). Rather than being an outright inconsistency, however, this potential disparity in the literature may be attributable to PD heterogeneity.
For several years, it has been recognized that PD is highly diverse in terms of onset, symptoms and impairments, disease progression and response to medication [14, 117–122]. The combination of clinical symptoms experienced and the biological processes underpinning these manifestations are potentially unique to each PD patient, such that PD may be resistant to useful subtyping [123]. Individual patient variation is thought to be due to the complex interplay between a variety of factors [123], including numerous genetic variants [124–128] and altered RNA expression [129]. Medical co-morbidities such as diabetes, anemia and cancer can additionally influence the pathogenesis and progression of PD [130]. Heterogeneity in the sleep disturbances experienced by PD patients, which include REM sleep behavior disorder (RBD) and insomnia, may be related to the heterogeneity in genetics, RNA expression and motor symptoms [131], and may exacerbate motor symptoms and cognitive issues to variable degrees [132]. Other factors such as pesticide exposures [133–136], age and sex [137] likely contribute to the individual-level heterogeneity, with PD sex differences themselves seemingly influenced by the population of origin (Western vs. Asian) and the age at disease onset [138]. Concussions have been shown to significantly increase the risk of PD development [139], while cerebral microbleeds have been posited to contribute to heterogeneous PD pathogenesis [140].
In light of the heterogeneity prevalent in PD, intestinal enteric neuron loss might occur in some patients but not in others, or in distinct amounts (and perhaps involving different neuronal subtypes) in each patient. Such heterogeneous loss could contribute to the diversity of intestinal dysfunctions underlying PD-related constipation [54, 77]. Future studies in this area should account for potential heterogeneity by including assessment of the phenomena currently believed to interact and contribute to individual patient variation.
Data and methodological transparency would enhance the validity of any future retrospective analysis
Each future study of intestinal enteric neuron loss and/or constipation in PD should make all methodological considerations and data acquired available online along with the published research article, including:
• Enteric neuron quantities in exact amounts/numbers, rather than in proportions/percentages, and all parameters (neuronal, ganglionic, tissue or otherwise) relevant to the neuronal quantification method implemented. The total number of neurons and neurons per ganglion/neuronal density, for example, in each plexus and in each intestinal region examined should be presented separately. How an enteric ganglion was defined, how many ganglia were examined in each sample and/or how intestinal tissue stretch was accounted for should also be specified.
• Clarification/explanation regarding which participants underwent objective assessments of intestinal function (CTT evaluation and ARM, for example) and which patients and controls were included in neuronal quantification and correlation analyzes. A scatterplot with regression line, if applicable, and correlation co-efficient for each correlation performed should also be presented.
• Detailed, de-identified information on every individual examined, including age, sex, ethnicity and other demographics, specific medications, co-morbidities and history of particular medical conditions, cerebral injuries or pesticide exposures, the presence of particular genetic variants, RNA expression patterns or sleep disturbances, and the disease duration, severity and age at onset for each PD patient.
• Ensuring the information listed above is freely accessible would allow for precise and complete comparisons between relevant future studies, and enable incorporation of future study data into large-scale retrospective analyzes capable of determining the variables that may influence intestinal enteric neuron loss and/or constipation in PD patients.
OTHER CONSIDERATIONS
Challenges in obtaining objective constipation measures and myenteric plexus samples from PD patients
It can be difficult to consistently evaluate intestinal dysfunction among PD patients. For example, while a few patients refuse CTT evaluation and/or ARM in some studies [54], only a small subgroup of patients consent to combined assessment of colonic transit and anorectal function in other studies [77]. Patients may also refuse the endoscopy required for intestinal biopsy collection [54], without which numbers and properties of intestinal enteric neurons cannot be evaluated, but endoscopy refusal does not occur in most studies [50, 53, 65]. Even when objective assessments of both colonic transit and anorectal function are performed in patients from whom intestinal biopsies are also obtained, these biopsies often only contain the mucosa and submucosa— sampling the myenteric plexus via routine endoscopic biopsies presents a bleeding risk, and so cannot be done safely [53]. Full-thickness intestinal wall samples include the myenteric plexus [16], but these can only be obtained from living PD patients if part of their colon is resected [73]. Moreover, the need for colectomy entails that the patient likely suffers from an intestinal dysfunction unrepresentative of most PD patients. For example, two large-scale (though CSS score-based) studies suggest that severe constipation, one reason for colonic resection [73], is not common among PD patients [91, 92]. Other potential reasons for colectomy such as cancer [73] or a bowel disease, in addition to the resection itself, could contribute to any dysfunction recorded and thus confound results. Samples of intestinal myenteric plexus are easier to obtain from PD patients postmortem [51, 63] but, for obvious reasons, any myenteric neuron loss found in these samples cannot be subsequently compared with functional colonic and anorectal assessments.
The link between enteric neuron loss and intestinal dysfunction in preclinical studies is unclear
Due to the challenges associated with intestinal myenteric plexus sampling from live PD patients, combined with the difficulties in obtaining measures of intestinal function that are not influenced by issues other than PD, some researchers have resorted to PD animal model studies to elucidate the connection between intestinal myenteric neurons and PD-related constipation. Unfortunately, when myenteric neuronal parameters within the intestine and intestinal function of several PD mouse models were compared in the same study, the onset and severity of both myenteric neuropathy and intestinal dysfunction were heavily influenced by mouse age, sex and species, as well as neurotoxin doses, duration of neurotoxin treatment and timing of post-treatment assessments [141]. Because of these limitations, the extent to which intestinal dysfunction is correlated with myenteric degeneration in various mouse models of PD remains uncertain.
Intestinal tissue artifacts could impact on neuronal quantification
Post-mortem artifacts can occur in intestinal tissue sampled at autopsy [75] and operation-associated artifacts may be present in surgical specimens [74, 142]. Such artifacts impact on histomorphological evaluation of intestinal tissues, including quantification of enteric neurons. None of the studies that quantified intestinal enteric neuron loss compared PD and control tissue sampled via different methods (Supplementary Table 1) and future studies should continue to only compare intestinal tissues collected via the same method (at autopsy, via biopsy or via resection, for example) in an attempt to partially control for sampling artifacts.
Enteric neuron dysfunction and extrinsic innervation may contribute to PD-related constipation
Aside from enteric neuron loss, changes in the function, connectivity, mitochondria and/or α-synuclein proteins of enteric neurons, especially VIPergic and cholinergic subpopulations [54, 66], may underlie or contribute to intestinal dysfunctions in PD patients. However, to our knowledge, only one study has evaluated the function of intestinal enteric neurons and their mitochondria in PD patients, and discovered no neuronal nor mitochondrial dysfunction relative to controls [53]. The potential causative role of altered enteric neurotransmission in PD-related constipation, whether due to loss of neuronal subtypes or neurotransmitter expression, is contentious [50, 51, 54, 65, 143] and studies of intestinal α-synuclein alterations in PD patients have produced diverse results deserving of a discussion beyond the scope of this review. Since constipation precedes the onset of PD motor dysfunction and diagnosis by 10–20 years in some cases [28, 29], a large-scale prospective study of constipated individuals, wherein their intestinal function and enteric neuron function, connectivity, mitochondria and/or α-synuclein protein parameters are periodically assessed over the long-term, could be an ideal way to elucidate the role of enteric neuronal changes in PD development and symptoms. A large multicenter study of a similar style provided valuable information on the conversion rate of RBD to parkinsonisms and dementias, and determined the predictive value of various prodromal markers [144].
Extrinsic innervation to the ENS is also important for gastrointestinal function and thus extrinsic fibers, including vagal nerves, may play a role in PD-related constipation. Further research is required to better understand the role of both sympathetic and parasympathetic extrinsic visceral sensory afferent and visceral motor efferent fibers in the intestinal dysfunctions and disease pathology of PD patients.
The potential impact of enteric neuron loss on other GI functions requires investigation in PD patients
Although patients suffer from a range of GI issues, including gastroparesis and dysphagia [16], constipation is the only GI dysfunction that has been specifically evaluated and discussed in studies of PD-related enteric neuron loss. Additionally, the potential impact of enteric neuron loss on small intestinal functions, such as nutrient absorption, and the microbiome [145–147] is yet to be investigated in PD patients. Future clinical studies should explore the connection between loss of enteric neurons and microbiome alterations, malabsorption and other PD-related GI dysfunctions.
CONCLUSIONS
The studies that sought to investigate PD-related intestinal enteric neuron loss were limited by small sample sizes and over-representation of minority PD patients (early-onset PD patients) or patients potentially misdiagnosed with PD (early PD patients). However, when these studies are considered relative to one another in terms of sample size and the proportion of early PD patients included, results suggest neuron loss may occur in the colonic submucosal plexus of patients with clinically established PD. The studies which specifically investigated PD-related submucosal neuron loss in the colon or rectum additionally explored constipation, but the criteria-based assessments these studies utilized do not reliably evaluate the intestinal dysfunctions of PD patients. One study did objectively measure intestinal function, but over 50% of the examined patients had early PD. In preclinical investigations, enteric neuropathy and intestinal function findings are heavily influenced by animal, treatment, and assessment parameters, such that the extent to which intestinal dysfunction is correlated with enteric degeneration in PD mouse models remains uncertain.
Before the connection between intestinal enteric neuron loss and PD-related constipation can be confidently described, future research must objectively quantify intestinal dysfunctions in PD patients and use sufficiently large samples representative of the patient population (majority diagnosed with idiopathic PD for at least 5 years). Future investigations should also implement a consistent neuronal quantification method and study design, including standardized patient recruitment criteria, publish with a high degree of data transparency and account for potential PD heterogeneity.
Further investigation into other potential influencers of PD-related constipation is required, including changes in the function, connectivity, mitochondria and/or α-synuclein proteins of enteric neurons and their extrinsic innervation. The connection between enteric neuron loss and other PD-related GI issues, including gastroparesis and dysphagia, as well as changes in nutrient absorption and the microbiome, should be explored in future research.
ACKNOWLEDGMENTS
We thank Mr. Stephen Yoannidis for his technical expertise in formatting figures and tables. We thank Prof. Roberto De Giorgio and Prof. Fiorella Giancola (Department of Translational Medicine, University of Ferrara) for kindly providing us with unpublished results and additional clarifications that greatly aided our attempted re-analysis of PD-related intestinal enteric neuron loss and constipation data. Finally, we thank Dr. Sara Vogrin (Medical Statistician, St Vincent’s Hospital) and Mr. Cameron Patrick (Statistical Consultant, Melbourne Statistical Consulting Platform) for their expert advice and guidance during our trial re-analysis of relevant PD datasets.
This review was funded by National Health and Medical Research Council (NHMRC) Ideas Grant APP1183420 (Protecting the Gut: A Novel Therapeutic Avenue for Parkinson’s Disease).
CONFLICT OF INTEREST
The authors have no conflict of interest to report. The funder had no role in the design nor writing of this review.
DATA AVAILABILITY
Most data utilized and analyzed during the writing of this review are available within published articles. Unpublished datasets (supplied by Prof. Roberto De Giorgio and Prof. Fiorella Giancola) were also analyzed and can be obtained from these authors upon reasonable request.
SUPPLEMENTARY MATERIAL
[1] The supplementary material is available in the electronic version of this article: https://dx.doi.org/10.3233/JPD-223262.
REFERENCES
[1] | Kouli A , Torsney KM , Kuan W-L ((2018) ) Parkinson–s disease: Etiology, neuropathology, and pathogenesis. In Parkinson25; s Disease: Pathogenesis and Clinical Aspects, Stoker TB, Greenland JC, eds. Codon Publications, Brisbane, pp. 3–26. |
[2] | Lanciego JL , Luquin N , Obeso JA ((2012) ) Functional neuroanatomy of the basal ganglia. Cold Spring Harb Perspect Med 2: , a009621. |
[3] | Postuma RB , Berg D , Stern M , Poewe W , Olanow W , Oertel W , Obeso J , Marek K , Litvan I , Lang AE , Halliday G , Goetz CG , Gasser T , Dubois B , Chan P , Bloem BR , Adler CH , Deuschl G ((2015) ) MDS clinical diagnostic criteria for Parkinson’s disease. Mov Disord 30: , 1591–1599. |
[4] | Albin RL , Young AB , Penney JB ((1989) ) The functional anatomy of basal ganglia disorders. Trends Neurosci 12: , 366–375. |
[5] | Giguère N , Burke Nanni S , Trudeau L-E ((2018) ) On cell loss and selective vulnerability of neuronal populations in Parkinson’s disease. Front Neurol 9: , 455. |
[6] | Cheng H-C , Ulane CM , Burke RE ((2010) ) Clinical progression in Parkinson disease and the neurobiology of axons. Ann Neurol 67: , 715–725. |
[7] | Barzilai A , Melamed E ((2003) ) Molecular mechanisms of selective dopaminergic neuronal death in Parkinson’s disease. Trends Mol Med 9: , 126–132. |
[8] | Michel PP , Hirsch EC , Hunot S ((2016) ) Understanding dopaminergic cell death pathways in Parkinson disease. Neuron 90: , 675–691. |
[9] | Fox SH , Katzenschlager R , Lim S-Y , Barton B , de Bie RMA , Seppi K , Coelho M , Sampaio C ((2018) ) International Parkinson and Movement Disorder Society evidence-based medicine review: Update on treatments for the motor symptoms of Parkinson’s disease. Mov Disord 33: , 1248–1266. |
[10] | Mercuri NB , Bernardi G ((2005) ) The ‘magic’ of L-dopa: Why is it the gold standard Parkinson’s disease therapy? . Trends Pharmacol Sci 26: , 341–344. |
[11] | Chagraoui A , Boulain M , Juvin L , Anouar Y , Barrière G , De Deurwaerdère P ((2019) ) L-DOPA in Parkinson’s disease: Looking at the “false” neurotransmitters and their meaning. Int J Mol Sci 21: , 294. |
[12] | Miyasaki JM , Martin W , Suchowersky O , Weiner WJ , Lang AE ((2002) ) Practice parameter: Initiation of treatment for Parkinson’s disease: An evidence-based review: Report of the Quality Standards Subcommittee of the American Academy of Neurology. Neurology 58: , 11–17. |
[13] | Chaudhuri KR , Healy DG , Schapira AHV ((2006) ) Non-motor symptoms of Parkinson’s disease: Diagnosis and management. Lancet Neurol 5: , 235–245. |
[14] | Halli-Tierney AD , Luker J , Carroll DG ((2020) ) Parkinson disease. Am Fam Physician 102: , 679–691. |
[15] | Goldman JG , Postuma R ((2014) ) Premotor and nonmotor features of Parkinson’s disease. Curr Opin Neurol 27: , 434–441. |
[16] | Pfeiffer RF ((2018) ) Gastrointestinal dysfunction in Parkinson’s disease. Curr Treat Options Neurol 20: , 54. |
[17] | Chalazonitis A , Rao M ((2018) ) Enteric nervous system manifestations of neurodegenerative disease. Brain Res 1693: , 207–213. |
[18] | Furness JB , Callaghan BP , Rivera LR , Cho H-J ((2014) ) The enteric nervous system and gastrointestinal innerva tion: Integrated local and central control. In Microbial Endocrinology: The Microbiota-Gut-Brain Axis in Health and Disease, Lyte M, Cryan JF, eds. Springer, New York, pp. 39–71. |
[19] | Furness JB ((2012) ) The enteric nervous system and neurogastroenterology. Nat Rev Gastroenterol Hepatol 9: , 286–294. |
[20] | Furness JB (2006) The Enteric Nervous System, Blackwell, Oxford. |
[21] | Lin HC , Prather C , Fisher RS , Meyer JH , Summers RW , Pimentel M , McCallum RW , Akkermans LMA , Loening-Baucke V ((2005) ) Measurement of gastrointestinal transit. Dig Dis Sci 50: , 989–1004. |
[22] | Snape Jr WJ ((1997) ) Role of colonic motility in guiding therapy in patients with constipation. Dig Dis 15: , 104–111. |
[23] | Su A , Gandhy R , Barlow C , Triadafilopoulos G ((2017) ) A practical review of gastrointestinal manifestations in Parkinson’s disease. Parkinsonism Relat Disord 39: , 17–26. |
[24] | Fasano A , Visanji NP , Liu LWC , Lang AE , Pfeiffer RF ((2015) ) Gastrointestinal dysfunction in Parkinson’s disease. Lancet Neurol 14: , 625–639. |
[25] | Knudsen K , Krogh K , Østergaard K , Borghammer P ((2017) ) Constipation in Parkinson’s disease: Subjective symptoms, objective markers, and new perspectives. Mov Disord 32: , 94–105. |
[26] | Chen H , Zhao EJ , Zhang W , Lu Y , Liu R , Huang X , Ciesielski-Jones AJ , Justice MA , Cousins DS , Peddada S ((2015) ) Meta-analyses on prevalence of selected Parkinson’s nonmotor symptoms before and after diagnosis. Transl Neurodegener 4: , 1. |
[27] | Suares NC , Ford AC ((2011) ) Prevalence of, and risk factors for, chronic idiopathic constipation in the community: Systematic review and meta-analysis. Am J Gastroenterol 106: , 1582–1591. |
[28] | Adams-Carr KL , Bestwick JP , Shribman S , Lees A , Schrag A , Noyce AJ ((2016) ) Constipation preceding Parkinson’s disease: A systematic review and meta-analysis. J Neurol Neurosurg Psychiatry 87: , 710–716. |
[29] | Scott GD , Lim MM , Drake MG , Woltjer R , Quinn JF ((2021) ) Onset of skin, gut, and genitourinary prodromal Parkinson’s disease: A study of 1.5 million veterans. Mov Disord 36: , 2094–2103. |
[30] | Yu Q-J , Yu S-Y , Zuo L-J , Lian T-H , Hu Y , Wang R-D , Piao Y-S , Guo P , Liu L , Jin Z , Li L-X , Chan P , Chen S-D , Wang X-M , Zhang W ((2018) ) Parkinson disease with constipation: Clinical features and relevant factors. Sci Rep 8: , 567. |
[31] | Di Nardo G , Blandizzi C , Volta U , Colucci R , Stanghellini V , Barbara G , Del Tacca M , Tonini M , Corinaldesi R , De Giorgio R ((2008) ) Review article: Molecular, pathological and therapeutic features of human enteric neuropathies. Aliment Pharmacol Ther 28: , 25–42. |
[32] | Han MN , Finkelstein DI , McQuade RM , Diwakarla S ((2022) ) Gastrointestinal dysfunction in Parkinson’s disease: Current and potential therapeutics. J Pers Med 12: , 144. |
[33] | Wood JD Enteric nervous system: The brainin-the-gut. In Colloquium Series on Integrated Systems Physiology: From Molecule to Function to Disease. Morgan & Claypool, San Rafael. |
[34] | Ross MH , Pawlina W ((2006) ) Histology: A Text and Atlas: With correlated cell and molecular biology. 5th ed., Lippincott Williams & Wilkins, Philadelphia. |
[35] | Cooke HJ ((1998) ) “Enteric tears”: Chloride secretion and its neural regulation. News Physiol Sci 13: , 269–274. |
[36] | Kirchgessner AL , Tamir H , Gershon MD ((1992) ) Identification and stimulation by serotonin of intrinsic sensory neurons of the submucosal plexus of the guinea pig gut: Activity-induced expression of Fos immunoreactivity. J Neurosci 12: , 235–248. |
[37] | Kirchgessner AL , Gershon MD ((1988) ) Projections of submucosal neurons to the myenteric plexus of the guinea pig intestine: tracing of microcircuits by retrograde and anterograde transport. }. J Comp Neurol 277: , 487–498. |
[38] | Song Z-M , Costa M , Brookes SJH ((1998) ) Projections of submucous neurons to the myenteric plexus in the guinea pig small intestine. J Comp Neurol 399: , 255–268. |
[39] | Kunze WAA , Furness JB , Bertrand PP , Bornstein JC ((1998) ) Intracellular recording from myenteric neurons of the guinea-pig ileum that respond to stretch. J Physiol 506: , 827–842. |
[40] | Bertrand PP , Kunze WAA , Bornstein JC , Furness JB , Smith ML ((1997) ) Analysis of the responses of myenteric neurons in the small intestine to chemical stimulation of the mucosa. Am J Physiol Gastrointest Liver Physiol 273: , G422–G435. |
[41] | Kunze WAA , Bornstein JC , Furness JB ((1995) ) Identification of sensory nerve cells in a peripheral organ (the intestine) of a mammal. Neuroscience 66: , 1–4. |
[42] | Moore BA , Vanner S ((2000) ) Properties of synaptic inputs from myenteric neurons innervating submucosal S neurons in guinea pig ileum. Am J Physiol Gastrointest Liver Physiol 278: , G273–G280. |
[43] | Furness JB , Jones C , Nurgali K , Clerc N ((2004) ) Intrinsic primary afferent neurons and nerve circuits within the intestine. Prog Neurobiol 72: , 143–164. |
[44] | Wood JD ((2016) ) Enteric nervous system: Neuropathic gastrointestinal motility. Dig Dis Sci 61: , 1803–1816. |
[45] | Boschetti E , Malagelada C , Accarino A , Malagelada JR , Cogliandro RF , Gori A , Bonora E , Giancola F , Bianco F , Tugnoli V , Clavenzani P , Azpiroz F , Stanghellini V , Sternini C , De Giorgio R ((2019) ) Enteric neuron density correlates with clinical features of severe gut dysmotility. Am J Physiol Gastrointest Liver Physiol 317: , G793–G801801. |
[46] | Bassotti G , Villanacci V ((2006) ) Slow transit constipation: A functional disorder becomes an enteric neuropathy. World J Gastroenterol 12: , 4609–4613. |
[47] | Adad SJ , Cancado CG , Etchebehere RM , Teixeira VPA , Gomes UA , Chapadeiro E , Lopes ER ((2001) ) Neuron count reevaluation in the myenteric plexus of chagasic megacolon after morphometric neuron analysis. Virchows Archiv 438: , 254–258. |
[48] | Iantorno G , Bassotti G , Kogan Z , Lumi CM , Cabanne AM , Fisogni S , Varrica LM , Bilder CR , Munoz JP , Liserre B , Morelli A , Villanacci V ((2007) ) The enteric nervous system in chagasic and idiopathic megacolon. Am J Surg Pathol 31: , 460–468. |
[49] | Lebouvier T , Chaumette T , Damier P , Coron E , Touchefeu Y , Vrignaud S , Naveilhan P , Galmiche J-P , Brunley des Varannes S , Derkinderen P , Neunlist M ((2008) ) Pathological lesions in colonic biopsies during Parkinson’s disease. Gut 57: , 1741–1743. |
[50] | Lebouvier T , Neunlist M , Bruley des Varannes S , Coron E , Drouard A , N’Guyen J-M , Chaumette T , Tasselli M , Paillusson S , Flamand M , Galmiche J-P , Damier P , Derkinderen P ((2010) ) Colonic biopsies to assess the neuropathology of Parkinson’s disease and its relationship with symptoms.. PLoS One 5: , e12728. |
[51] | Annerino DM , Arshad S , Taylor GM , Adler CH , Beach TG , Greene JG ((2012) ) Parkinson’s disease is not associated with gastrointestinal myenteric ganglion neuron loss. Acta Neuropathol 124: , 665–680. |
[52] | Barrenschee M , Zorenkov D , Böttner M , Lange C , Cossais F , Scharf AB , Deuschl G , Schneider SA , Ellrichmann M , Fritscher-Ravens A , Wedel T ((2017) ) Distinct pattern of enteric phospho-alpha-synuclein aggregates and gene expression profiles in patients with Parkinson’s disease. Acta Neuropathol Commun 5: , 1. |
[53] | Desmet A-S , Cirillo C , Tack J , Vandenberghe W , Vanden Berghe P ((2017) ) Live calcium and mitochondrial imaging inthe enteric nervous system of Parkinson patients and controls. eLife 6: , e26850. |
[54] | Giancola F , Torresan F , Repossi R , Bianco F , Latorre R , Ioannou A , Guarino M , Volta U , Clavenzani P , Mazzoni M , Chiocchetti R , Bazzoli F , Travagli RA , Sternini C , De Giorgio R ((2017) ) Downregulation of neuronal vasoactiveintestinal polypeptide in Parkinson’s disease and chronic constipation. Neurogastroenterol Motil 29: , e12995. |
[55] | Campbell F , GI-Duodenum, , Small Bowel https://pathologia.ed.ac.uk/topic/gi-duodenum-and-small-bowel-normal-histology, Accessed August 8, 2021. |
[56] | Jones O , The small intestine, https://teachmeanatomy.info/abdomen/gi-tract/small-intestine, Accessed August 8, 2021. |
[57] | Elkouzi A , Young onset Parkinson’s, https://www.parkinson.org/Understanding-Parkinsons/What-is-Parkinsons/Young-Onset-Parkinsons, Accessed October 6, 2021. |
[58] | John Hopkins Medicine.Young-onset Parkinson’s disease, https://www.hopkinsmedicine.org/health/conditions-anddiseases/parkinsons-disease/youngonset-parkinsons-disease, Accessed October 6, 2021. |
[59] | The Michael J. Fox Foundation. Young-onset Parkinson’s disease, https://www.michaeljfox.org/news/young-onsetparkinsons-disease Accessed October 6, 2021. |
[60] | Parkinson’s Australia. Young onset Parkinson’s, https://www.parkinsons.org.au/young-onset-parkinson-s,Accessed October 6, 2021. |
[61] | Day JO , Mullin S ((2021) ) The genetics of Parkinson’s disease and implications for clinical practice. Genes 12: , 1006. |
[62] | Scott L , Dawson VL , Dawson TM ((2017) ) Trumping neurodegeneration: Targeting common pathways regulated by autosomal recessive Parkinson’s disease genes. Exp Neurol 298: , 191–201. |
[63] | Ohlsson B , Englund E ((2019) ) Atrophic myenteric and submucosal neurons are observed in Parkinson’s disease. Parkinsons Dis 2019: , 7935820. |
[64] | Aubrey-Jones D , The colon, https://teachmeanatomy.info/abdomen/gi-tract/colon/, Accessed August 8, 2021. |
[65] | Corbillé A-G , Coron E , Neunlist M , Derkinderen P , Lebouvier T ((2014) ) Appraisal of the dopaminergic andnoradrenergic innervation of the submucosal plexus in PD. J Parkinsons Dis 4: , 571–576. |
[66] | Anlauf M , Schäfer MK-H , Eiden L , Weihe E ((2003) ) Chemical coding of the human gastrointestinal nervous system: Cholinergic, VIPergic, and catecholaminergic phenotypes. J Comp Neurol 459: , 90–111. |
[67] | Adler CH , Beach TG , Hentz JG , Shill HA , Caviness JN , Driver-Dunckley E , Sabbagh MN , Sue LI , Jacobson SA , BeldenCM , Dugger BN ((2014) ) Low clinical diagnostic accuracy of early vs advanced Parkinson disease: Clinicopathologicstudy. Neurology 83: , 406–412. |
[68] | Postuma RB , Poewe W , Litvan I , Lewis S , Lang AE , Halliday G , Goetz CG , Chan P , Slow E , Seppi K , Schaffer E , Rios-Romenets S , Mi T , Maetzler C , Li Y , Heim B , Bledsoe IO , Berg D ((2018) ) Validation of the MDS Clinical Diagnostic Criteria for Parkinson’s disease. Mov Disord 33: , 1601–1608. |
[69] | Beach TG , Adler CH ((2018) ) Importance of low diagnostic accuracy for early Parkinson’s disease. Mov Disord 33: , 1551–1554. |
[70] | Rizzo G , Copetti M , Arcuti S , Martino D , Fontana A , Logroscino G ((2016) ) Accuracy of clinical diagnosis of Parkinson disease: A systematic review and meta-analysis. Neurology 86: , 566–576. |
[71] | Hughes AJ , Daniel SE , Kilford L , Lees AJ ((1992) ) Accuracy of clinical diagnosis of idiopathic Parkinson’s disease: A clinico-pathological study of 100 cases. J Neurol Neurosurg Psychiatry 55: , 181–184. |
[72] | Gelb DJ , Oliver E , Gilman S ((1999) ) Diagnostic criteria for Parkinson disease. Arch Neurol 56: , 33–39. |
[73] | Singaram C , Ashraf W , Gaumnitz EA , Torbey C , Sengupta A , Pfeiffer R , Quigley EM ((1995) ) Dopaminergic defect ofenteric nervous system in Parkinson’s disease patients with chronic constipation. Lancet 346: , 861–864. |
[74] | Bernstein ML ((1978) ) Biopsy technique: The pathological considerations. J Am Dent Assoc 96: , 438–443. |
[75] | Kaushal A ((2019) ) Post-mortem artefacts. J Evol Med Dent Sci 8: , 2929. |
[76] | Skjærbæk C , Knudsen K , Horsager J , Borghammer P ((2021) ) Gastrointestinal dysfunction in Parkinson’sdisease. J Clin Med 10: , 493. |
[77] | De Pablo-Fernández E , Passananti V , Zárate-López N , Emmanuel A , Warner T ((2019) ) Colonic transit,high-resolution anorectal manometry and MRI defecography study of constipation in Parkinson’s disease. Parkinsonism Relat Disord 66: , 195–201. |
[78] | Longstreth GF , Thompson WG , Chey WD , Houghton LA , Mearin F , Spiller RC ((2006) ) Functional bowel disorders. Gastroenterology 130: , 1480–1491. |
[79] | Gjerløff T , Fedorova T , Knudsen K , Munk OL , Nahimi A , Jacobsen S , Danielsen EH , Terkelsen AJ , Hansen J , Pavese N , Brooks DJ , Borghammer P ((2015) ) Imaging acetylcholinesterase density in peripheral organs in Parkinson’sdisease with 11C-donepezil PET. Brain 138: , 653–663. |
[80] | Horsager J , Andersen KB , Knudsen K , Skjærbæk C , Fedorova TD , Okkels N , Schaeffer E , Bonkat SK , Geday J , Otto M , Sommerauer M , Danielsen EH , Bech E , Kraft J , Munk OL , Hansen SD , Pavese N , Göder R , Brooks DJ , BergD , Borghammer P ((2020) ) Brain-first versus body-first Parkinson’s disease: A multimodal imaging case-controlstudy. Brain 143: , 3077–3088. |
[81] | Knudsen K , Fedorova TD , Bekker AC , Iversen P , Østergaard K , Krogh K , Borghammer P ((2017) ) Objective colonic dysfunction is far more prevalent than subjective constipation in Parkinson’s disease: A colon transit and volume study. J Parkinsons Dis 7: , 359–367. |
[82] | Mostafa R ((2008) ) Rome III: The functional gastrointestinal disorders, third edition, 2006. World J Gastroenterol 14: , 2124–2125. |
[83] | Fedorova TD , Seidelin LB , Knudsen K , Schacht AC , Geday J , Pavese N , Brooks DJ , Borghammer P ((2017) ) Decreased intestinal acetylcholinesterase in early Parkinson disease: An 11C-donepezil PET study. Neurology 88: , 775–781. |
[84] | Chaudhuri KR , Martinez-Martin P , Brown RG , Sethi K , Stocchi F , Odin P , Ondo W , Abe K , MacPhee G , MacMahon D , Barone P , Rabey M , Forbes A , Breen K , Tluk S , Naidu Y , Olanow W , Williams AJ , Thomas S , Rye D , Tsuboi Y , Hand A , Schapira AHV ((2007) ) The metric properties of a novel non-motor symptoms scale for Parkinson’s disease: Results from an international pilot study. Mov Disord 22: , 1901–1911. |
[85] | Chaudhuri KR , Martinez-Martin P , Schapira AHV , Stocchi F , Sethi K , Odin P , Brown RG , Koller W , Barone P , MacPhee G , Kelly L , Rabey M , MacMahon D , Thomas S , Ondo W , Rye D , Forbes A , Tluk S , Dhawan V , Bowron A , Williams AJ , Olanow CW ((2006) ) International multicenter pilot study of the first comprehensive self-completed nonmotor symptoms questionnaire for Parkinson’s disease: The NMSQuest Study. Mov Disord 21: , 916–923. |
[86] | Goetz CG , Tilley BC , Shaftman SR , Stebbins GT , Fahn S , Martinez-Martin P , Poewe W , Sampaio C , Stern MB , Dodel R , Dubois B , Holloway R , Jankovic J , Kulisevsky J , Lang AE , Lees A , Leurgans S , LeWitt PA , Nyenhuis D , Olanow CW , Rascol O , Schrag A , Teresi JA , van Hilten JJ , LaPelle N ((2008) ) Movement Disorder Society-Sponsored Revision of the Unified Parkinson’s Disease Rating Scale (MDS-UPDRS): Scale presentation and clinimetric testing results. Mov Disord 23: , 2129–2170. |
[87] | Visser M , Marinus J , Stiggelbout AM , van Hilten JJ ((2004) ) Assessment of autonomic dysfunction in Parkinson’s disease: The SCOPA-AUT. Mov Disord 19: , 1306–1312. |
[88] | Agachan F , Chen T , Pfeifer J , Reissman P , Wexner SD ((1996) ) A constipation scoring system to simplify evaluation and management of constipated patients. Dis Colon Rectum 39: , 681–685. |
[89] | Ashraf W , Park F , Lof J , Quigley EMM ((1996) ) An examination of the reliability of reported stool frequency in the diagnosis of idiopathic constipation. Am J Gastroenterol 91: , 26–32. |
[90] | Rhondali W , Nguyen L , Palmer L , Kang D-H , Hui D , Bruera E ((2013) ) Self-reported constipation in patients with advanced cancer: A preliminary report. J Pain Symptom Manage 45: , 23–32. |
[91] | Frazzitta G , Ferrazzoli D , Folini A , Palamara G , Maestri R ((2019) ) Severe constipation in Parkinson’s disease and in parkinsonisms: Prevalence and affecting factors. Front Neurol 10: , 621. |
[92] | Krogh K , Østergaard K , Sabroe S , Laurberg S ((2008) ) Clinical aspects of bowel symptoms in Parkinson’s disease. Acta Neurol Scand 117: , 60–64. |
[93] | Alame AM , Bahna H ((2012) ) Evaluation of constipation. Clin Colon Rectal Surg 25: , 5–11. |
[94] | Borghammer P , Knudsen K , Brooks DJ ((2016) ) Imaging systemic dysfunction in Parkinson’s disease. Curr Neurol Neurosci Rep 16: , 51. |
[95] | Edwards LL , Quigley EMM , Harned RK , Hofman R , Pfeiffer RF ((1994) ) Characterization of swallowing and defecation in Parkinson’s disease. Am J Gastroenterol 89: , 15–25. |
[96] | Jost WH , Schimrigk K ((1993) ) Cisapride treatment of constipation in Parkinson’s disease. Mov Disord 8: , 339–343. |
[97] | Knudsen K , Haase A-M , Fedorova TD , Bekker AC , Østergaard K , Krogh K , Borghammer P ((2017) ) Gastrointestinal transit time in Parkinson’s disease using a magnetic tracking system. J Parkinsons Dis 7: , 471–479. |
[98] | Sakakibara R , Odaka T , Uchiyama T , Asahina M , Yamaguchi K , Yamaguchi T , Yamanishi T , Hattori T ((2003) ) Colonic transit time and rectoanal videomanometry in Parkinson’s disease. J Neurol Neurosurg Psychiatry 74: , 268–272. |
[99] | Wang C-P , Sung W-H , Wang C-C , Tsai P-Y ((2013) ) Early recognition of pelvic floor dyssynergia and colorectal assessment in Parkinson’s disease associated with bowel dysfunction. , e130-e. Colorectal Dis 15: , 137. |
[100] | Bassotti G , Maggio D , Battaglia E , Giulietti O , Spinozzi F , Reboldi G , Serra AM , Emanuelli G , Chiarioni G ((2000) ) Manometric investigation of anorectal function in early and late stage Parkinson’s disease. J Neurol Neurosurg Psychiatry 68: , 768–770. |
[101] | Stocchi F , Badiali D , Vacca L , D’Alba L , Bracci F , Ruggieri S , Torti M , Berardelli A , Corazziari E ((2000) ) Anorectal function in multiple system atrophy and Parkinson’s disease. Mov Disord 15: , 71–76. |
[102] | De Giorgio R , Giancola F (2021) personal communications . |
[103] | Button KS , Ioannidis JPA , Mokrysz C , Nosek BA , Flint J , Robinson ESJ , Munafò MR ((2013) ) Power failure: Why small sample size undermines the reliability of neuroscience. Nat Rev Neurosci 14: , 365–376. |
[104] | Faber J , Fonseca LM ((2014) ) How sample size influences research outcomes. Dental Press J Orthod 19: , 27–29. |
[105] | Ioannidis JPA ((2005) ) Why most published research findings are false. , e. PLoS Med 2: , 124. |
[106] | Ioannidis JPA ((2007) ) Why most published research findings are false: Author’s Reply to Goodman and Greenland. PLoS Med 4: , e215. |
[107] | Turner RM , Bird SM , Higgins JPT ((2013) ) The impact of study size on meta-analyses: Examination of underpoweredstudies in Cochrane reviews. PLoS One 8: , e59202. |
[108] | McKenzie JE , Brennan SE ((2019) ) Chapter 12: Synthesizing and presenting findings using other methods. In Cochrane Handbook for Systematic Reviews of Interventions. 2nd ed., Higgins JPT, Thomas J, Chandler J, Cumpston M, Li T, Page MJ, Welch VA, eds. John Wiley & Sons, Chichester, pp. 321–347. |
[109] | Goetz CG , Poewe W , Rascol O , Sampaio C , Stebbins GT , Counsell C , Giladi N , Holloway RG , Moore CG , Wenning GK , Yahr MD , Seidl L ((2004) ) Movement disorder society task force report on the hoehn and yahr staging scale: Status and recommendations. Mov Disord 19: , 1020–1028. |
[110] | Hoehn MM , Yahr MD ((1967) ) Parkinsonism: Onset, progression, and mortality. Neurology 17: , 427–442. |
[111] | Fahn S , Elton R , Members of the UPDRS Development Committee (1987) The Unified Parkinson’s Disease Rating Scale. In Recent Developments inParkinson’s Disease, Vol 2., Fahn S, Marsden CD, Calne DB, Goldstein M, eds. Macmillan Healthcare Information, Florham Park, NJ, pp. 153-163, 293-304. |
[112] | Martínez-Martín P , Rodríguez-Blázquez C , Alvarez M , Arakaki T , Arillo VC , Chaná P , Fernández W , Garretto N , Martínez-Castrillo JC , Rodríguez-Violante M , Serrano-Dueñas M , Ballesteros D , Rojo-Abuin JM , Chaudhuri KR , Merello M ((2015) ) Parkinson’s disease severity levels and MDS-Unified Parkinson’s Disease Rating Scale. Parkinsonism Relat Disord 21: , 50–54. |
[113] | Bhatia D , Grozdanov V , Ruf WP , Kassubek J , Ludolph AC , Weishaupt JH , Danzer KM ((2021) ) T-cell dysregulation is associated with disease severity in Parkinson’s disease. J Neuroinflammation 18: , 250. |
[114] | Cilia R , Cereda E , Klersy C , Canesi M , Zecchinelli AL , Mariani CB , Tesei S , Sacilotto G , Meucci N , Zini M , Ruffmann C , Isaias IU , Goldwurm S , Pezzoli G ((2015) ) Parkinson’s disease beyond 20 years. J Neurol Neurosurg Psychiatry 86: , 849–855. |
[115] | Maki K , Gruber-Baldini A , Barr E , von Coelln FR , Reich S , Shulman L ((2016) ) Comparing effects of age of onset and disease duration in Parkinson disease (P5.379). , P5. Neurology 86: , 379. |
[116] | Pagano G , Ferrara N , Brooks DJ , Pavese N ((2016) ) Age at onset and Parkinson disease phenotype. Neurology 86: , 1400–1407. |
[117] | Jankovic J , McDermott M , Carter J , Gauthier S , Goetz C , Golbe L , Huber S , Koller W , Olanow C , Shoulson I , Stern M , Tanner C , Weiner W , the Parkinson Study Group ((1990) ) Variable expression of Parkinson’s disease: A base-line analysis of the DATATOP cohort. Neurology 40: , 1529–1534. |
[118] | Kehagia AA , Barker RA , Robbins TW ((2010) ) Neuropsychological and clinical heterogeneity of cognitive impairment and dementia in patients with Parkinson’s disease. Lancet Neurol 9: , 1200–1213. |
[119] | Lewis SJG , Barker RA ((2009) ) Understanding the dopaminergic deficits in Parkinson’s disease: Insights into disease heterogeneity. J Clin Neurosci 16: , 620–625. |
[120] | Merchant H , Luciana M , Hooper C , Majestic S , Tuite P ((2008) ) Interval timing and Parkinson’s disease: Heterogeneity in temporal performance. Exp Brain Res 184: , 233–248. |
[121] | Pettit L , McCarthy M , Davenport R , Abrahams S ((2013) ) Heterogeneity of letter fluency impairment and executive dysfunction in Parkinson’s disease. J Int Neuropsychol Soc 19: , 986–994. |
[122] | Ryden LE , Lewis SJG ((2019) ) Parkinson’s disease in the era of personalised medicine: One size does not fit all. Drugs Aging 36: , 103–113. |
[123] | Mestre TA , Fereshtehnejad S-M , Berg D , Bohnen NI , Dujardin K , Erro R , Espay AJ , Halliday G , van Hilten JJ , Hu MT , Jeon B , Klein C , Leentjens AFG , Marinus J , Mollenhauer B , Postuma R , Rajalingam R , Rodríguez-Violante M , Simuni T , Surmeier DJ , Weintraub D , McDermott MP , Lawton M , Marras C ((2021) ) Parkinson’s disease subtypes: Critical appraisal and recommendations. J Parkinsons Dis 11: , 395–404. |
[124] | Iwaki H , Blauwendraat C , Leonard HL , Kim JJ , Liu G , Maple-Grødem J , Corvol J-C , Pihlstrøm L , van Nimwegen M , Hutten SJ , Nguyen K-DH , Rick J , Eberly S , Faghri F , Auinger P , Scott KM , Wijeyekoon R , Van Deerlin VM , Hernandez DG , Gibbs JR , International Parkinson’s Disease Genomics Consortium, Chitrala KN , Day-Williams AG , Brice A , Alves G , Noyce AJ , Tysnes O-B , Evans JR , Breen DP , Estrada K , Wegel CE , Danjou F , Simon DK , Andreassen O , Ravina B , Toft M , Heutink P , Bloem BR , Weintraub D , Barker RA , Williams-Gray CH , van de Warrenburg BP , Van Hilten JJ , Scherzer CR , Singleton AB , Nalls MA ((2019) ) Genomewide association study of Parkinson’s disease clinical biomarkers in 12 longitudinal patients’ cohorts. Mov Disord 34: , 1839–1850. |
[125] | Kumar S , Yadav N , Pandey S , Muthane UB , Govindappa ST , Abbas MM , Behari M , Thelma BK ((2020) ) Novel and reported variants in Parkinson’s disease genes confer high disease burden among Indians. Parkinsonism Relat Disord 78: , 46–52. |
[126] | Li YI , Wong G , Humphrey J , Raj T ((2019) ) Prioritizing Parkinson’s disease genes using population-scale transcriptomic data. Nat Commun 10: , 994. |
[127] | Pihlstrøm L , Morset KR , Grimstad E , Vitelli V , Toft M ((2016) ) A cumulative genetic risk score predicts progression in Parkinson’s disease. Mov Disord 31: , 487–490. |
[128] | Sharma M , Ioannidis JPA , Aasly JO , Annesi G , Brice A , Broeckhoven CV , Bertram L , Bozi M , Crosiers D , Clarke C , Facheris M , Farrer M , Garraux G , Gispert S , Auburger G , Vilariño-Güell C , Hadjigeorgiou GM , Hicks AA , Hattori N , Jeon B , Lesage S , Lill CM , Lin J-J , Lynch T , Lichtner P , Lang AE , Mok V , Jasinska-Myga B , Mellick GD , Morrison KE , Opala G , Pramstaller PP , Pichler I , Park SS , Quattrone A , Rogaeva E , Ross OA , Stefanis L , Stockton JD , Satake W , Silburn PA , Theuns J , Tan E-K , Toda T , Tomiyama H , Uitti RJ , Wirdefeldt K , Wszolek Z , Xiromerisiou G , Yueh K-C , Zhao Y , Gasser T , Maraganore D , Krüger R ((2012) ) Large-scale replication and heterogeneity in Parkinson disease genetic loci. Neurology 79: , 659–667. |
[129] | Keo A , Mahfouz A , Ingrassia AMT , Meneboo J-P , Villenet C , Mutez E , Comptdaer T , Lelieveldt BPF , Figeac M , Chartier-Harlin M-C , van de Berg WDJ , van Hilten JJ , Reinders MJT ((2020) ) Transcriptomic signatures of brain regional vulnerability to Parkinson’s disease. Commun Biol 3: , 101. |
[130] | Santiago JA , Bottero V , Potashkin JA ((2017) ) Biological and clinical implications of comorbidities in Parkinson’s disease. Front Aging Neurosci 9: , 394. |
[131] | Stefani A , Högl B ((2020) ) Sleep in Parkinson’s disease. Neuropsychopharmacol 45: , 121–128. |
[132] | Nedergaard M , Goldman SA ((2020) ) Glymphatic failure as a final common pathway to dementia. Science 370: , 50–56. |
[133] | Breckenridge CB , Berry C , Chang ET , Sielken RL Jr , Mandel JS ((2016) ) Association between Parkinson’s disease and cigarette smoking, rural living, well-water consumption, farming and pesticide use: Systematic review and meta-analysis. , e. PLoS One 11: , 0151841. |
[134] | Moisan F , Spinosi J , Delabre L , Gourlet V , Mazurie J-L , Bénatru I , Goldberg M , Weisskopf MG , Imbernon E , Tzourio C , Elbaz A ((2015) ) Association of Parkinson’s disease and its subtypes with agricultural pesticideexposures in men: A case-control study in France. Environ Health Perspect 123: , 1123–1129. |
[135] | Shrestha S , Parks CG , Umbach DM , Richards-Barber M , Hofmann JN , Chen H , Blair A , Freeman LEB , Sandler DP ((2020) ) Pesticide use and incident Parkinson’s disease in a cohort of farmers and their spouses. Environ Res 191: , 110186. |
[136] | van der Mark M , Brouwer M , Kromhout H , Nijssen P , Huss A , Vermeulen R ((2012) ) Is pesticide use related to Parkinson disease? Some clues to heterogeneity in study results. Environ Health Perspect 120: , 340–347. |
[137] | Szewczyk-Krolikowski K , Tomlinson P , Nithi K , Wade-Martins R , Talbot K , Ben-Shlomo Y , Hu MTM ((2014) ) The influence of age and gender on motor and non-motor features of early Parkinson’s disease: Initial findings from the Oxford Parkinson Disease Center (OPDC) discovery cohort. Parkinsonism Relat Disord 20: , 99–105. |
[138] | Taylor KSM , Cook JA , Counsell CE ((2007) ) Heterogeneity in male to female risk for Parkinson’s disease. J Neurol Neurosurg Psychiatry 78: , 905–906. |
[139] | Morissette MP , Prior HJ , Tate RB , Wade J , Leiter JRS ((2020) ) Associations between concussion and risk of diagnosis of psychological and neurological disorders: A retrospective population-based cohort study. Fam Med Comm Health 8: , e000390. |
[140] | Yang H-J ((2018) ) Cerebral microbleeds and the heterogeneity of Parkinson’s disease. J Korean Med Sci 33: , e293. |
[141] | McQuade RM , Singleton LM , Wu H , Lee S , Constable R , Di Natale M , Ringuet MT , Berger JP , Kauhausen J , Parish CL , Finkelstein DI , Furness JB , Diwakarla S ((2021) ) The association of enteric neuropathy with gut phenotypes in acute and progressive models of Parkinson’s disease. Sci Rep 11: , 7934. |
[142] | Taqi SA , Sami SA , Sami LB , Zaki SA ((2018) ) A review of artifacts in histopathology. J Oral Maxillofac Pathol 22: , 279. |
[143] | Derkinderen P , Cossais F , de Guilhem de Lataillade A , Leclair-Visonneau L , Neunlist M , Paillusson S , De Giorgio R (2021) Gastrointestinal mucosal biopsies in Parkinson’s disease: Beyond alpha-synuclein detection. J Neural Transm (Vienna). doi: 10.1007/s00702-021-02445-6. |
[144] | Postuma RB , Iranzo A , Hu M , Högl B , Boeve BF , Manni R , Oertel WH , Arnulf I , Ferini-Strambi L , Puligheddu M , Antelmi E , Cochen De Cock V , Arnaldi D , Mollenhauer B , Videnovic A , Sonka K , Jung K-Y , Kunz D , Dauvilliers Y , Provini F , Lewis SJ , Buskova J , Pavlova M , Heidbreder A , Montplaisir JY , Santamaria J , Barber TR , Stefani A , St Louis EK , Terzaghi M , Janzen A , Leu-Semenescu S , Plazzi G , Nobili F , Sixel-Doering F , Dusek P , Bes F , Cortelli P , Ehgoetz Martens K , Gagnon J-F , Gaig C , Zucconi M , Trenkwalder C , Gan-Or Z , Lo C , Rolinski M , Mahlknecht P , Holzknecht E , Boeve AR , Teigen LN , Toscano G , Mayer G , Morbelli S , Dawson B , Pelletier A ((2019) ) Risk and predictors of dementia and parkinsonism in idiopathic REM sleep behaviour disorder: A multicentre study. Brain 142: , 744–759. |
[145] | Petrov VA , Saltykova IV , Zhukova IA , Alifirova VM , Zhukova NG , Dorofeeva YB , Tyakht AV , Kovarsky BA , Alekseev DG , Kostryukova ES , Mironova YS , Izhboldina OP , Nikitina MA , Perevozchikova TV , Fait EA , Babenko VV , Vakhitova MT , Govorun VM , Sazonov AE ((2017) ) Analysis of gut microbiota in patients with Parkinson’s disease. Bull Exp Biol Med 162: , 734–737. |
[146] | Romano S , Savva GM , Bedarf JR , Charles IG , Hildebrand F , Narbad A ((2021) ) Meta-analysis of the Parkinson’s disease gut microbiome suggests alterations linked to intestinal inflammation. NPJ Parkinsons Dis 7: , 27. |
[147] | Shen T , Yue Y , He T , Huang C , Qu B , Lv W , Lai H-Y ((2021) ) The association between the gut microbiota and Parkinson’s disease, a meta-analysis. Front Aging Neurosci 13: , 636545. |