Disease-Modifying Therapies for Multiple System Atrophy: Where Are We in 2022?
Abstract
Multiple system atrophy is a rapidly progressive and fatal neurodegenerative disorder. While numerous preclinical studies suggested efficacy of potentially disease modifying agents, none of those were proven to be effective in large-scale clinical trials. Three major strategies are currently pursued in preclinical and clinical studies attempting to slow down disease progression. These target α-synuclein, neuroinflammation, and restoration of neurotrophic support. This review provides a comprehensive overview on ongoing preclinical and clinical developments of disease modifying therapies. Furthermore, we will focus on potential shortcomings of previous studies that can be avoided to improve data quality in future studies of this rare disease.
INTRODUCTION
Multiple system atrophy (MSA) is a fatal, adult-onset and rapidly progressive neurodegenerative disease characterized by autonomic failure, ataxia, and parkinsonism in any combination [1, 2]. Two motor phenotypes are recognized in MSA: A parkinsonian variant (MSA-P) featuring a poorly levodopa-responsive akinetic-rigid syndrome and a cerebellar variant (MSA-C) presenting with broad based gait, limb ataxia, scanning dysarthria, and cerebellar oculomotor dysfunction. Apart from these motor features, MSA is associated with autonomic failure (urogenital, cardiovascular or both) as well as several other motor and non-motor features including dystonia, pyramidal signs, REM-sleep behavior disorder or stridor [3].
Neuropathologically, misfolded α-synuclein forms insoluble aggregates, termed glial cytoplasmatic inclusions (GCIs), with consecutive microglial activation and release of pro-inflammatory cytokines and oxygen reactive species [4, 5]. Although α-synuclein is primarily an intracellular protein, a variety of α-synuclein species can be found in cerebrospinal fluid (CSF) of patients with α-synucleinopathies [6]. Preclinical studies in mice which developed α-synuclein inclusion pathology after being inoculated with brain homogenates from MSA patients, suggest that abnormally folded α-synuclein may drive the spread of MSA-related pathology from cell-to-cell in a prion-like fashion [7].
Thus far, potential disease-modifying therapies (DMT) have failed in clinical trials, but numerous DMTs are currently in clinical development for MSA and target different key abnormalities of the neurodegenerative cascade in MSA (as illustrated in Fig. 1). Alpha-synuclein is the most obvious therapeutic target and treatment strategies focus on the aggregation, the spreading and the clearance of (misfolded) α-synuclein. Other strategies target neuroinflammation, neurotrophic support, mitochondrial dysfunction, and excitotoxicity. In the present paper, we will review ongoing developments of DMTs in the field. We performed a non-systematic literature review using PubMed and the search terms “MSA”, “multiple system atrophy”, “treatment”, “therapy”, “disease modification”. We selected publications reporting results of disease-modifying trials that involved MSA patients and critical assessed and reviewed these reports. Studies on symptomatic therapies were excluded.
Fig. 1
Therapeutic targets for disease modifying therapies in multiple system atrophy. This figure demonstrates pathological mechanisms underlying Multiple system atrophy and potential disease modifying targets including aggregation, spreading and clearance of α-synuclein (red), neurotrophic support (green) and the cascade of neuroinflammation (violet). Created with BioRender.com.
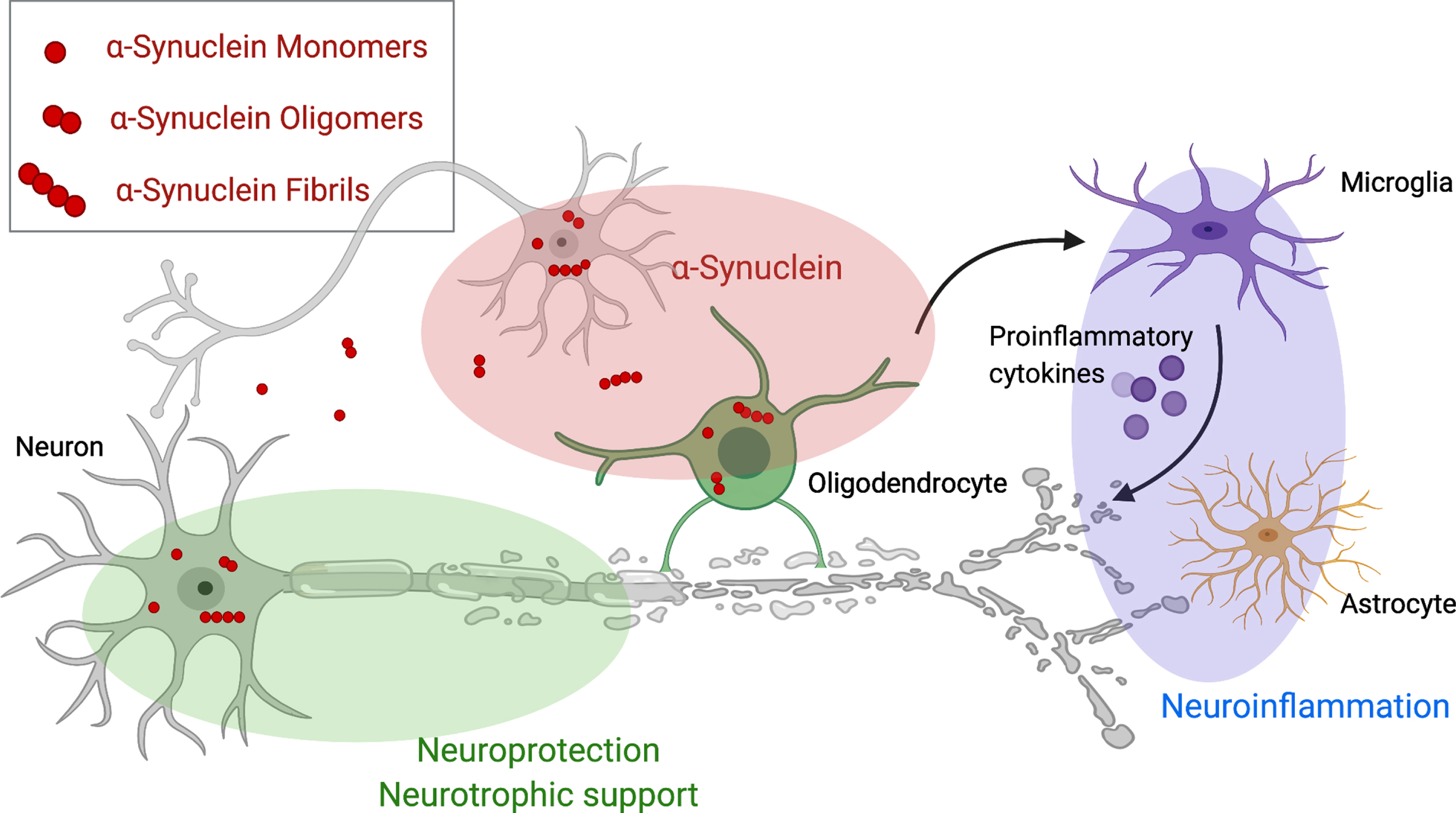
Targeting α-synuclein
GCIs are the hallmark neuropathological finding in MSA and possibly a major contributor to the neurodegenerative cascade in MSA. In contrast to Parkinson’s disease (PD) and Lewy body dementia (DLB), where aggregated α-synuclein predominantly accumulates within astrocytes and neurons, in MSA, it mainly accumulates within oligodendroglia and to a lesser extent in neurons [8, 9]. The pathogenic cascade leading to α-synuclein aggregation and neurodegeneration of this oligodendroglioneuronal proteinopathy are poorly understood [8, 9]. Recent studies suggest an early translocation of α-synuclein to the cell nucleus [10]. Further, myelin-associated oligodendrocyte basic protein and huntingtin interacting protein 1 appear to interact with α-synuclein thriving pathogenic cascade of MSA [11]. Converging evidence suggests a prion-like spreading of misfolded α-synuclein strains as a key pathogenic event [12–21] and some authors even suggested that MSA is a prion disease [7, 22–24]. However, the latter remains a matter of intense debate [25–30].
Clinical development
Therapies targeting α-synuclein in MSA are illustrated in Fig. 2. Table 1 gives an overview on therapeutic strategies targeting α-synuclein.
Fig. 2
Disease modifying therapies targeting α-synuclein assemblies at different stages. Disease modifying therapies target different levels along the α-synuclein aggregation cascade. ASO, antisense oligonucleotides.
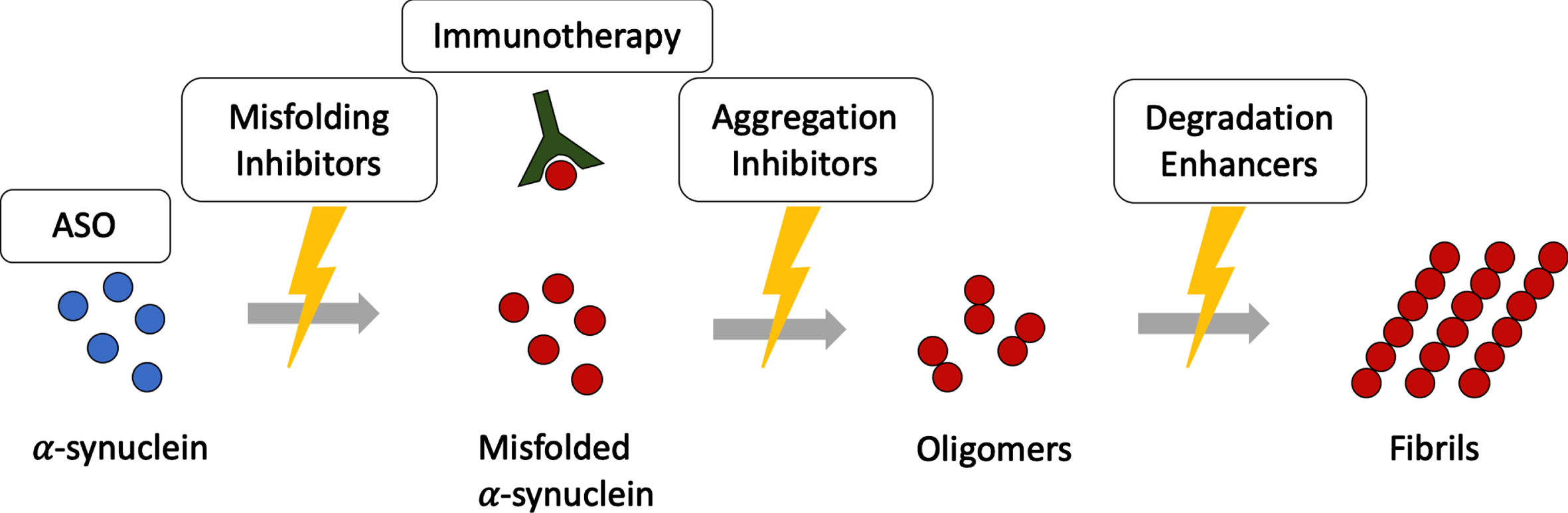
Table 1
Therapeutic strategies targeting α-synuclein in MSA
Mode of action | Substance | Phase | Design | Primary outcome | Results | Comments |
Immunotherapy | PD01A/PD03A | Phase I | RCT | Safety & tolerability | Safe & well-tolerated | PD01A: significant immunoresponse against α-synuclein |
Lu AF82422 | Phase I | RCT | Safety & tolerability | Safe & well-tolerated | In healthy controls and PD patients | |
Antisense oligonucleotides | BIIB101 | Phase I | RCT | Safety & tolerability | – | Ongoing in MSA patients |
Aggregation inhibitors | EGCG | Phase III | RCT | UMSARS part II score | Negative | Exploratory analyses suggested reduced striatal volume loss |
NPT200-11A | Phase I | RCT | Safety & tolerability | Results pending | In healthy volunteers | |
Anle138b | Phase Ib | RCT | Safety & tolerability | – | Ongoing in mild to moderate PD | |
ATH-434 | Phase I | RCT | Safety & tolerability | Safe & well-tolerated | In healthy volunteers | |
CLR01 | Preclinical | Molecular tweezer | ||||
NPT088 | Preclinical | Fusion protein | ||||
Synuclein-D | Preclinical | Small molecule | ||||
IkT-148009 | Preclinical | Small molecule | ||||
Kallikrein-6 | Preclinical | Neurosin | ||||
Degradation enhancers | Rifampicin | Phase III | RCT | UMSARS part I score | Early termination | Futility criteria were met |
Rapamycin | Phase II | RCT | UMSARS total score | Early termination | Futility criteria were met | |
Lithium | Phase II | RCT | Number of SAE & nSAE | Early termination | Severe adverse events |
RCT, randomized-controlled trial; UMSARS, United Multiple System Atrophy Rating Scale; SAE, serious adverse event; nSAE, non-serious adverse event; PD, Parkinson’s disease; MSA, multiple system atrophy.
Active and passive immunization
Although there is no definite evidence that α-synuclein is absolutely essential and the only relevant pathogen for the development of neurodegeneration or clinical parkinsonism [31], the potential contribution of α-synuclein is evidenced by above mentioned preclinical studies and clinical observations that SNCA multiplications cause familiar PD [32]. Furthermore, α-synuclein induced disturbances in cellular pathways (autophagy and lysosomal pathways) [33] and the cell-to-cell transmission likely occurring through secretion via exosome release and reuptake through endocytosis provides a clear rationale for α-synuclein directed therapies warrant further (pre)clinical evaluation [34]. However, it has to be acknowledged that the majority of immunotherapeutic constructs aim to mitigate extracellular pathology and intracellular proteinopathy cannot be tackled directly through these immunotherapeutic attempts. The latter would require the development of intrabodies interfering with intracellular α-synuclein species [35].
Active immunization was used in MBP-α-synuclein transgenic mice, a model of MSA that expresses α-synuclein in oligodendrocytes, suggesting amelioration of the neurodegenerative pathology in a preclinical model of MSA. In this study, a vaccine-induced production of specific anti-α-synuclein antibodies crossing the blood-brain barrier was observed. Neurodegeneration as well as demyelination in neocortex, striatum, and corpus callosum were markedly reduced in in vivo models of synucleinopathies [36]. A subsequent phase I study in humans reported that AFFITOPE PD01A elicited an antibody response specific to the C-terminal region of α-synuclein and was safe and well-tolerated in patients with early PD [37]. A recent phase I trial in MSA patients revealed that both PD01A and PD03A were able to induce a significant and sustained immune response against α-synuclein with a higher responder rate in the PD01A group [38]. Future studies are required to determine whether PD01A or PD03A-induced antibodies are in fact able to modify the natural course of MSA.
Passive immunization is another promising immunotherapeutic approach. Several novel monoclonal antibodies (mAb) binding to α-synuclein with high affinity are in the therapeutic pipeline.
Lu AF82422 is a mAb proven as safe and well tolerated in a phase I trial in healthy subjects and patients with PD (NCT03611569). A phase II study investigating the safety and efficacy in patients with MSA is planned for the end of 2021 [39].
Other clinical trials investigating passive immunotherapies are currently not being investigated in MSA, but are promising against α-synuclein in PD, and therefore may also be beneficial for MSA treatment. Prasinezumab (also known as PRX002) is a mAb therapy under active development in PD. A phase I study reported that the antibody was safe and well tolerated [40]. A larger, phase II trial failed to slow motor symptom worsening in patients with PD, but showed signals of efficacy on secondary and exploratory outcomes including MDS-UPDRS subscores, MoCA, and striatal dopamine receptor binding (NCT03100149 and NCT04777331) [41].
Another α-synuclein-directed mAb is MEDI1341. This antibody was shown to block cell-to-cell transmission of preformed α-synuclein fibrils in vitro and was able to sequester extracellular α-synuclein in vivo. A phase I study to assess safety and tolerability of single ascending doses of MEDI1341 in healthy volunteers was recently completed, but the results are still pending (NCT03272165). The second phase I trial, assessing multiple ascending doses in patients with PD is ongoing (NCT04449484).
The mAb BIIB054 proved effective in reducing the α-synuclein load and improving behavioural deficits in animal models of PD and DLB [42–44]. A randomized phase I trial in healthy adults and PD patients showed good safety, tolerability, and favourable pharmacokinetic profiles [45]. However, a randomized controlled phase II study in PDs failed to meet its primary outcome and the drug development was discontinued (NCT03318523).
Altogether, active and passive immunotherapy may play a role in disease modification in MSA, but further, large-scale studies are required to confirm the neuroprotective efficacy of immunization strategies. Limitations of accessibility of intraneuronal α-synuclein aggregates not being targeted with immunization need to be overcome. Furthermore, previous immunization studies in Alzheimer’s disease have clearly demonstrated that immunisation in neurodegenerative disease may cut both ways with post-vaccination meningoencephalitis possibly occurring in a substantial number of treated patients with detrimental consequences [46]. Notably, in this trial immunisation resulted in a clearance of amyloid plaques and long-term follow-up demonstrated that patients with Alzheimer’s disease actively immunized against amyloid-β remained virtually amyloid plaque-free for 14 years [47]. However, most patients in this trial had progressed to severe dementia nonetheless [48]. Finally, it remains to be studied whether neuroinflammation contributes to neurodegeneration or protects neurons from toxic alpha-synuclein species in MSA.
Antisense oligonucleotides
Keeping in mind that intracellular aggregation of α-synuclein play a central role in the MSA pathology, the reduction of α-synuclein production itself provides rationale for disease modification. Antisense oligonucleotide (ASO) therapy can inhibit intracellular production of α-synuclein by targeting the pre-mRNA of the SNCA gene [49]. Animal models demonstrated neuroprotective effects and a marked reduction of α-synuclein in CSF and brain tissue [49, 50]. However, there are concerns rendered by preclinical studies suggesting that a complete α-synuclein knock-out may exacerbate neuroinflammation and have detrimental effects [51–53]. Therefore, selecting the appropriate degree of treatment-induced interference with α-synuclein translation is critical.
A phase I randomized controlled trial with intrathecal application of the ASO BIIB101 in MSA patients is ongoing (NCT04165486). Another clinical trial of ASO targeting leucine-rich repeat kinase 2 (LRRK2) is currently underway (NCT03976349).
Inhibition of α-synuclein misfolding
The small molecule Epigallocatechin gallate (EGCG), a green tea extract, binds to unfolded α-synuclein polypeptide chains and inhibits β-sheet formation, thus, preventing aggregation and prion-like spreading [54–56]. Previous studies suggested EGCG as an iron chelator conferring protection against neurotoxicity [57]. Although preclinical models showed evidence of effectiveness [58, 59], a multi-center randomized controlled phase III trial in patients with MSA failed to demonstrate disease modifying effects after 52 weeks of treatment and hepatotoxicity was observed in some patients [60]. However, an exploratory analyses of the MRI sub-study showed lower annual volume loss in striatum and precentral gyrus in EGCG treated MSA patients [61].
Another small molecule for potential MSA therapy is the α-synuclein misfolding inhibitor NPT200-11A. Preclinical studies in mouse models of PD showed beneficial effects on reducing α-synuclein pathology in the cortex and astrogliosis. Normalized striatal dopamine transporter levels and improvement of motor function were observed [62, 63]. A phase I trial in healthy subjects to determine the safety, tolerability and blood levels of NPT200-11A is completed without published results so far (NCT02606682).
Inhibition of α-synuclein aggregation
Anle138b is a small molecule targeting intracellular oligomers of α-synuclein. Preclinical studies reported high oral bioavailability and blood–brain barrier penetration. Anle138b blocks oligomer formation without affecting the monomers of α-synuclein, therefore preserving its physiological function [64]. Behavioral improvements correlating with a 30% reduction of α-synuclein accumulation in substantia nigra pars compacta (SNpc) and striatum as well as a significant reduction of microglial activation were observed in a MSA mouse model [65, 66]. A phase I study in healthy volunteers to determine safety, tolerability, and blood levels of orally administered anle138b has been completed successfully and the results are pending [67]. A Phase 1b study for anle138b in patients with mild to moderate PD is currently recruiting patients (NCT04685265).
Dysregulation of iron metabolism in the SNpc promotes the aggregation of α-synuclein and production of cellular reactive oxygen species causing neuronal death [68]. First experiments with novel quinazolinone inhibitor ATH434 (previously known as PBT434) revealed reduced levels of α-synuclein and markers of oxidative stress accompanied by motor improvement in PD animal models [69]. Similar results were reproduced in transgenic MSA mice [70, 71]. In a phase I study with healthy volunteers, ATH434 was safe and well tolerated (U1111-1211-0052) and achieved CSF concentrations comparable with those associated with efficacy in animal models [72, 73]. Therefore, a phase II study in MSA patients is currently under consideration [74].
Enhancing α-synuclein degradation
There is growing evidence that the autophagy-lysosomal pathway is affected in MSA [75]. One such pathway is the mammalian target of rapamycin complex (mTOR) pathway. Rapamycin, also known as sirolimus, is an immunosuppressant that specifically inhibits actions of mTOR by allosterically modulating access to the catalytic site of mTOR [76]. A recent proof-of-concept study demonstrated partial neuroprotection and reduction in α-synuclein aggregates in PLP-α-synuclein transgenic mice after treatment with rapamycin [77]. An additional preclinical study showed evidence of motor improvement, reduction of 4-hydroxynonenal-protein-adducts, and attenuation of synaptic injury in A53T α-synuclein transgenic mice [78]. However, isolated dysfunctional macroautophagy did not improve clearance of abnormal accumulation of α-synuclein in vitro. A phase II randomized controlled study in patients with MSA assessing efficacy of oral sirolimus on slowing disease progression was recently prematurely terminated because meeting the futility criteria (NCT03589976).
The antibiotic rifampicin inhibits the formation of α-synuclein fibrils and disaggregates fibrils already formed in MSA mouse models [80, 81]. A large phase III placebo controlled trial (NCT01287221) was terminated prematurely after a preplanned interim analysis of the primary endpoint (mean rate of change of UMSARS I score) revealed that futility criteria had been met [82].
Nilotinib is a compound acting as a tyrosine kinase Abelson (Abl) inhibitor approved for the treatment of chronic myeloid leukaemia. Preclinical evidence suggested that this drug can degrade misfolded α-synuclein by enhancing the autophagy-lysosomal pathway [83, 84] and reduce oxidative stress [85, 86]. Unfortunately, Nilotinib failed to show a disease modifying effect in mouse models of MSA [87]. However, findings in PD patients were observed controversial in clinical trials. A small open-label phase I clinical trial in patients with PD dementia and DLB showed positive safety and tolerability profiles [88] and phase II randomized-controlled trial confirmed reasonable drug safety and demonstrated effects on CSF levels of dopamine metabolites, α-synuclein oligomers, and tau in patients with PD [89]. However, another recent phase II trial demonstrated low CSF exposure and no efficacy after a 6-month treatment. These findings will guide trial development in patients with PD and MSA [90].
Lithium reduces α-synuclein aggregation and stimulates autophagy and neuroprotection in preclinical in vivo and in vitro models [91–93]. A phase II trial of lithium in patients with MSA was terminated due to severe adverse events discouraging further attempts of repurposing this drug in MSA [94].
Preclinical developments
Molecular tweezers are nano-chaperones with open cavities able to bind with guest molecules with non-covalent binding or electrostatic effects [95]. Upon treatment with the molecular tweezer CLR01, a reduction of α-synuclein load and dose-dependent reduction in GCI density was reported in a transgenic mouse model of MSA [96, 97]. These encouraging findings suggest a potential for disease modification in MSA and other synucleinopathies; however, the low penetrance through the blood-brain-barrier is a concern that needs to be addressed in future preclinical studies.
NPT088, a fusion protein combining a human immunoglobulin backbone with a general amyloid interaction motif, is currently under active clinical development for Alzheimer’s disease (NCT03008161). The protein’s motif not only recognizes amyloid-beta and phosphorylated tau but also misfolded α-synuclein, markedly decreasing amounts of aggregated α-synuclein in a PD mouse model [98].
The small molecule SynuClean-D was identified by a high-throughput screening assay. First tests in vitro and in PD models showed inhibition of α-synuclein aggregation by binding to α-synuclein fibrils, disruption of amyloid fibrils, and prevention of dopaminergic neurons degeneration [99].
The c-Abl kinase inhibitor IkT-148009 is currently being studied in PD animal models. Plans are also being made to study it in MSA animal models [100]. A phase I clinical trial of IkT-148009 in healthy volunteers and patients with PD is currently underway (NCT04350177).
The neurosin Kallikrein-6 is a serine protease with the ability to cleave α-synuclein in the central nervous system (CNS). When transferred through a lentiviral vector, a reduction of α-synuclein accumulation was shown in DLB/PD transgenic mouse models [101]. In the study by Spencer et al., kallikrein-6 was modified by the R80Q mutation resulting in longer half-life and was fused with the protein apoB for an effective transport through the blood-brain barrier [102]. This neurosin (NR)-R80Q-apoB enrichment resulted in a reduction of α-synuclein accumulation in oligodendrocytes and astrocytes, and in an improvement of the myelin sheath formation in the corpus callosum of MBP-α-synuclein transgenic mice. Additionally, behavioral improvements in cognition and locomotor activity were shown. However, a recent study demonstrated that reduced activity of kallikrein-6 is more likely a compensatory response than the cause of α-synuclein accumulation in MSA [103].
Targeting neuroinflammation
Widespread neuroinflammation and concomitant microglial activation are key histopathological findings in MSA paralleling neurodegeneration in brain areas affected by disease pathology [104]. Increasing evidence suggests that misfolded α-synuclein triggers microglial activation and astrogliosis in MSA and related α-synucleinopathies [4, 105–107]. Neuroimaging studies indicate severe neuroinflammation in MSA patients with a regional pattern consistent with the underlying MSA neuropathology [108]. Further, elevated levels of proinflammatory cytokines were previously reported [109]. However, it remains to be established whether neuroinflammation is a secondary consequence of neurodegeneration or an independent contributor to the pathophysiological cascade in MSA. Studies evaluating the disease-modifying potential of modulators of neuroinflammation were summarized in Table 2.
Table 2
Clinical trials targeting neuroinflammation
Target | Substance | Phase | Design | Primary outcome | Results | Comments |
Inhibition of neuro-inflammation | IVIG | Phase II | OL | Number of AEs | Positive | Motor improvement (small sample size, short treatment period) |
Inhibition of microglial activity | Minocycline | Phase II | RCT | UMSARS part II score | Negative | No motor improvement |
Oxidative stress reduction | Verdiperstat | Phase III | RCT | Modified UMSARS total score | Negative | Failed in terms of primary and key secondary endpoints |
IVIG, intravenous immunoglobulin; RCT, randomized-controlled trial; OL, open label trial; UMSARS, Unified Multiple System Atrophy rating scale.
Clinical development
Minocycline, a tetracycline antibiotic, was shown to inhibit microglial activation and its downstream events such as secretion of pro-inflammatory cytokines [110, 111]. A phase II randomized controlled trial of minocycline administered to MSA patients for 48 weeks failed to demonstrate motor improvement or neuroprotective effects [111]. However, [11C](R)-PK11195 PET for in vivo evaluation of neuroinflammation demonstrated target engagement with a reduction of subcortical microglial activation in a subgroup of MSA patients [111].
Neuroinflammation and production of toxic cytokines in MSA provide evidence in favor of intravenous immunoglobulin (IVIG) therapy [112–114]. IVIG inhibit autoreactive T-cells, suppress autoantibodies and interfere with the production of cytokines [114]. Novak et al. studied the effects of IVIG infusions in an open-label pilot study in 9 MSA patients showing a decrease of UMSARS scores in majority of patients [114]. No changes on brain MRI and no serious adverse events were observed. Despite these positive signals, a larger, confirmatory study is required to establish the efficacy of IVIG therapy in MSA.
Myeloperoxidase (MPO) plays a key role in the production of reactive oxygen species by phagocytic cells [115–117]. Verdiperstat is a potent inhibitor of MPO suppressing microglial activation and improving motor function in a transgenic MSA mouse model [106]. However these effects failed to influence motor impairments in a mouse model of advanced MSA [118]. Several phase I studies evaluating verdiperstat in healthy subjects reported no safety concerns. A phase II study reported amelioration of microglial activation in patients with PD [119]. In MSA patients, a phase II study showed trends towards clinical efficacy (NCT02388295). A phase III randomized controlled trial has recently being finished and failed to meet its primary and key secondary endpoints including a modified UMSARS score, the Clinical Global Impression of Improvement (CGI-I) score and the MSA quality of life questionnaire (NCT03952806) [120].
Preclinical development
The inflammatory protease caspase-1 promotes the aggregation of α-synuclein [121]. In a proof-of-concept study the caspase-1 inhibitor prodrug VX-765 ameliorated α-synuclein aggregation toxicity in transgenic MSA mice [122].
A combination of α-synuclein directed antibody and anti-inflammatory treatment was recently evaluated in a transgenic MSA mouse model [123]. CD5-D5 is a CNS penetrating single-chain antibody targeting α-synuclein. Lenalidomide, a small thalidomide derivative, marketed as an anticancer drug for multiple myeloma. The combined treatment showed a reduction of astrogliosis, microgliosis, as well as soluble and aggregated α-synuclein levels in transgenic MSA mice.
The immunomodulatory drug Fingolimod, currently marketed for treating multiple sclerosis, shows neuroprotective effects in different animal models by increasing brain-derived neurotrophic factors. The modified derivate FTY720-Mitoxy is known to increase expression of brain-derived neurotrophic factor (BDNF), glial-cell-line derived neurotrophic factor (GDNF), and nerve growth factor [124, 125]. Vidal-Martinez et al. reported a potent protective effect of FTY720-Mitoxy in CNP-α-synuclein transgenic MSA mice by reduction of motor disability and neuroinflammation, restoration of mitochondrial function and an increase of GDNF expression [126].
A synthetic microneurotrophin BNN-20 reduced microglial activation, increased BDNF and restored dopaminergic neurons even in advanced stages of neurodegeneration [127].
Other selected neuroprotective strategies
Potential neuroprotective disease modifying therapies are summarized in Table 3.
Table 3
Clinical trials targeting Neuroprotection and neurotrophic support
Target | Substance | Phase | Design | Primary outcome | Results | Comments |
FAF-1 | KM-819 | Phase I | RCT | Safety & tolerability | Safe & well tolerated | – |
Lipidomic neurotoxicity | YTX-7739 | Phase Ib | – | Safety & tolerability | – | Ongoing |
IGF1 pathway | Intranasal insulin | Phase II | RCT | Verbal fluency total score | – | Motor improvement (only 1 MSA patient) |
IGF1 pathway | Exendin-4 | Phase II | OL | UMSARS part I & II score | – | Ongoing |
Mitochondrial dysfunction | Coenzyme Q10 | Phase II | RCT | UMSARS part II score | – | Ongoing |
Neuronal/glial proliferation | Growth Hormone | Phase II | RCT | Safety & tolerability | Safe & well tolerated | Trend for less worsening of UMSARS [152] |
Immuno-modulation, neuro-protection | MSCs | Phase II | RCT | UMSARS part II score | Positive | Only in MSA-C, imaging not done in all patients [156] |
Mitochondrial dysfunction | Rasagiline | Phase II | RCT | UMSARS part I & II score | Negative | No motor improvement [160] |
Neurotrophic support | Fluoxetine | Phase II | RCT | UMSARS part I & II score | Negative | No motor improvement [163] |
Reduced excitotoxicity | Riluzole | Phase III | RCT | UPDRS part II & III | Negative | No motor improvement or survival rates [137] |
NMDA-modulator | Tllsh2910 | Phase III | RCT | SARA score | – | Ongoing |
IVIG, intravenous immunoglobulin; RCT, randomized-controlled trial; OL, open label trial; UPDRS, Unified Parkinson’s Disease Rating Scale; SARA, Scale for the assessment and rating of ataxia; UMSARS, Unified Multiple System Atrophy Rating Scale; MSA, multiple system atrophy.
Clinical development
KM-819
The protein FAS-associated factor 1 (FAF1) is expressed in cells to induce apoptosis. Studies have shown that these proteins are overexpressed in PD and therefore lead to increased neuronal cell death [128, 129]. KM-819 is an orally active small molecule drug developed as an inhibitor for FAF1. The first study in healthy volunteers showed promising results in safety and tolerability [130]. A phase II trial in PD and MSA patients is being considered [131].
YTX-7739
To screen for α-synuclein associated targets, a lipidomic analysis of its neurotoxicity was performed. This revealed that oleic acid, whose production is triggered by the stearoyl-CoA desaturase (SCD) has a neurotoxic effect on neurons [132, 133]. Therefore, the SCD inhibitor YTX-7739 is currently explored in a phase Ib proof-of-concept study [134].
Riluzole
Riluzole is a glutamate antagonist and the only approved DMT for amyotrophic lateral sclerosis [135]. By blocking sodium and potassium channels, the stimulation of glutamate receptors can be reduced and excitotoxicity induced neuronal death can be prevented. Preclinical studies in rat models of MSA showed a significant reduction of motor deficits and striatal lesion volume, suggesting a potential neuroprotective effect [136]. Seppi et al. carried out a randomized-controlled trial in a small group of 10 MSA patients revealing a lack of motor improvement [137]. A subsequent, large placebo-controlled trial in patients with MSA and PSP reported that riluzole had no effect on disease progression and survival [138].
Tllsh2910
N-methyl-D-aspartic acid (NMDA) receptors in the cerebellum play a role in motor learning and coordination [139]. Tllsh2910, a NMDA modulator, has been found to attenuate ataxic gait in a MSA mouse model. A phase III randomized-controlled single center study is currently recruiting patients with MSA-C (NCT03901638).
Insulin-like growth factor pathway
Insulin plays an important role in many neurodegenerative disorders due to its neuromodulatory, neurotrophic, and neuroprotective effects [140]. There is evidence that insulin-like growth factor-1 (IGF-1) signaling is impaired in PD and Alzheimer’s disease [141]. While a clinical study observed increased plasma insulin and IGF-1 concentrations in patients with MSA patients [142], reduced IGF-1 brain levels were observed in a transgenic mouse model of MSA [143, 144]. A pilot randomized placebo-controlled trial with intranasal insulin in 14 PD and 1 MSA patient showed an improvement of Hoehn & Yahr staging, UMSARS motor scores and verbal fluency without serious adverse events in treated patients [145]. Another promising antidiabetic drug being tested for MSA is the glucagon-like peptide agonist exendin-4 [144]. Bassil et al. evaluated exendin-4 treatment in transgenic MSA mice and observed increased insulin receptor density in the most severely affected brain regions, reduced monomeric α-synuclein load in the striatum and protective effect on survival of nigral dopamine neurons [144]. However, motor signs were not improved in transgenic mice. A phase II open label study on exendin-4 in patients with MSA is currently underway (NCT04431713).
Coenzyme Q10
Even though MSA is largely a sporadic disease, a causal relationship between COQ2 mutations and cerebellar-type MSA was established in Japanese patients [146]. These mutations lead to decreased production of Coenzyme Q10 (CoQ10), which is an electron carrier in the mitochondrial respiratory chain, and a potent antioxidant. In vitro studies with induced pluripotent stem cell-derived dopaminergic neurons from MSA patients reported reduced CoQ10 levels and up-regulation of several CoQ10 biosynthesis enzymes in MSA patients compared to healthy controls [147, 148]. These changes were partially rescued by CoQ10 supplementation [148]. A case report of high-dose ubiquinol treatment in a patient with COQ2 mutation and MSA-C reported no evidence of clinical or imaging benefit after 3 years treatment [149]. Nevertheless, a phase II randomized-controlled trial that already finished recruitment is currently ongoing in Japan (UMIN000031771).
Growth hormone
Growth hormone was shown to stimulate neuronal and glial proliferation and increase myelination and brain size [150]. In contrast, growth hormone deficiency is associated with impaired survival of new neurons and deficits in brain development and function [151, 152]. Along those lines, Holmberg et al. carried out a randomized-controlled trial with recombinant human growth hormone (r-hGH) in patients with MSA. After 12 months of subcutaneous treatment, no difference in the treatment effect between r-hGH-treated and placebo-treated MSA patients was observed, although the small sample size was a significant limitation of this study [152].
Mesenchymal stem cells
Because of their immunomodulatory and neuroprotective effects, mesenchymal stem cells (MSCs) have been the focus of a potential MSA therapy for over a decade now. The first clinical trial was an open-label monocentric study assessing feasibility and safety of intra-arterial MSCs therapy showing promising results [153, 154]. In 2011, Stemberger et al. confirmed potential neuroprotective effects of MSCs in a transgenic mouse model of MSA [155]. A phase II randomized placebo-controlled study reported attenuated UMSARS part II score progression in MSA-C patients receiving autologous bone marrow derived MSCs via intra-arterial or intravenous routes compared to patients receiving placebo [156]. However, procedural related adverse events (small ischemic brain lesions upon intra-arterial infusion) raised safety concerns. This has prompted the conduct of another phase I trial revisiting the safety and tolerability of intra-arterial (carotid arteries) injection of autologous bone marrow-derived mesenchymal stem cells in MSA-C patients which was recently completed in South Korea; the results have not been published so far (NCT03265444).
Singer et al. in 2019 demonstrated slowing of motor progression compared to a historical cohort through intrathecal injections of autologous fat tissue derived MSCs [157]. The adverse event rate increased with higher doses with patients developing low back or posterior leg pain, associated with thickening/MRI enhancement of lumbar nerve roots. Otherwise, this therapy proved to be safe and well tolerated encouraging further clinical development.
Rasagiline
The irreversible monoamine oxidase-B (MAO-B) inhibitor rasagiline shows symptomatic benefits and a possible disease-modifying effect in PD patients by modulation of the mitochondrial metabolism [158]. Preclinical studies in a transgenic mouse model of MSA revealed motor improvement, reduction of GCI load and neuronal protection [159]. However, a multicenter phase II randomized placebo-controlled clinical trial of rasagiline 1 mg/day in MSA-P patients did not show clinical benefits [160].
Selective serotonin-reuptake inhibitors
The neurotrophic factors GDNF and BDNF play an important role in neuroprotection. Selective serotonin-reuptake inhibitors (SSRI), currently used as anti-depressants, are reported to have a positive impact on neurotrophic factor expression. In a transgenic MSA mouse model fluoxetine has been shown to increase GDNF and BDNF levels and to suppress pro-inflammatory cytokines [161, 162]. A phase II randomized placebo-controlled trial of fluoxetine in MSA patients failed to demonstrate fluoxetine superiority over placebo on the total UMSARS score, whereas trends in motor and emotional secondary/exploratory outcomes deserve further investigation [163]. However, a retrospective long-term analysis of over 600 patients with MSA revealed that patients treated with any SSRI did not differ from patients never treated with SSRI in survival, but manifested parkinsonism and falls more frequently [164].
Another study around GDNF is currently recruiting in order to evaluate the safety and potential clinical effects of bilateral imaging-guided infusion of AAV2-GDNF into putamen of patients with MSA in a randomized placebo-controlled phase I trial (NCT04680065).
Preclinical development
Benztropine
The formation and repair of myelin is the main task of oligodendrocytes [165]. Alpha-synuclein accumulation in oligodendrocytes leads to demyelination resulting in axonal dysfunction and neuronal loss. Ettle et al. used the pro-myelinating activity of the muscarinic acetylcholine receptor antagonist benztropine attempting to reverse myelination deficits in different preclinical models of MSA. This experiment showed restoration of the α-synuclein-induced myelination of stem cell-derived oligodendrocytes and prevented neuronal loss in transgenic MSA mice [166]. Although benztropine has been used for decades in clinical neurology, it is debatable whether demyelination is a major target in MSA.
Monophosphoryl lipid A
Toll-like receptors (TLR) play an important role for innate immune response. Recently, TLR4 was identified as an important mediator of endogenous α-synuclein clearance by microglia [167]. Deficits of functional TLR4 resulted in increased α-synuclein accumulation, aggravation of the motor disability, and nigral degeneration in double MSA transgenic mice [168]. Preclinical studies in transgenic MSA mice treated with the TLR4 agonist monophosphoryl lipid A revealed an increased microglial α-synuclein uptake, significant motor improvement, rescue of nigral dopaminergic and striatal neurons, and region-specific reduction of the density of GCI in the absence of a marked systemic inflammatory response [169]. This approach offers an interesting option to fortify the endogenous mechanisms of α-synuclein clearance.
Sodium phenylbutyrate
Targeting MSA by its epigenetic roots, Sturm et al. described the hypothesis of a possible interference of α-synuclein with histone acetylation in glial and neuronal cells causing inhibition of acetylation and resulting in neurotoxicity [170, 171]. The pan-histone deacetylase inhibitor sodium phenylbutyrate showed a significant improvement of motor behavior and survival of nigral neurons in PLP–α-synuclein mice [171].
Trial design and outcome measures
Although large efforts were undertaken to conduct disease-modifying trials in MSA, improvements in trial methodology are still required. As highlighted throughout the entire review, we can only speculate on the reasons of failure of previous clinical trials in MSA. It is likely that the trials failed because of lacking efficacy of the studied compound; however, other issues associated with the trial design including too short follow-up periods, insufficient sample sizes, and high drop-out rates may have also had an impact on the outcome of the studies. Therefore, it is important to perform careful sample size estimates in the study planning and introduce measures to improve patient retention and study adherence. Additionally, the novel International Parkinson and Movement Disorder Society (MDS) criteria for the diagnosis of MSA will improve diagnostic accuracy in established as well as early stage disease enabling researchers to recruit more homogenous patient cohorts and earlier stage MSA patients.
Furthermore, there is still an unmet need of reliable surrogate biomarkers defining disease progression in MSA beyond clinical scales. Although much effort is taken here, we need to better define the natural history of this disease and do more research on possible surrogate biomarkers of disease progression including multimodal MRI and biofluid markers. Despite contradictory results in previous studies [172], CSF α-synuclein as well as markers of neurodegeneration (e.g., neurofilament) and glial dysfunction (e.g., GFAP) may be helpful. Recently, a novel PET-tracer has been proved to distinguish MSA from other synucleinopathies demonstrating promising potential as an imaging outcome parameter for future studies [173]. Finally, a clinical outcome assessment instrument capturing patient-cantered milestones will be essential and, recently, efforts attempting to improve and address shortcomings of the current version of the UMSARS were intensified and an expert task force working on a revised UMSARS was established within the MDS.
CONCLUSION AND OUTLOOK
The therapeutic management of patients with MSA remains largely frustrating with a lack of disease-modifying agents and symptomatic therapies that only offer transient and partial benefit to a subgroup of patients. Hence, there is an urgent unmet need for disease modifying therapies in MSA. Over the past two decades preclinical MSA models were developed to thoroughly characterize molecular changes and underlying neuropathophysiological events in MSA. These previous research efforts included neurotoxin-based animal models [174], targeted overexpression of α-synuclein through transgenic modifications [175] or viral vectors [176]. More recent studies performed intracerebral inoculation of MSA brain extracts into transgenic mice in an attempt to study seeding properties of MSA-specific α-synuclein strains. Despite the incomplete recapitulation of human MSA pathology [175, 177] preclinical studies have taught us important lessons on the pathophysiological cascade of MSA and secondary changes caused by aberrant α-synuclein aggregation [178]. These models opened an avenue to develop novel agents with disease-modifying effects and, preclinically, for confirmation of target engagement. However, as highlighted above, several candidate therapies showed preclinical evidence of neuroprotection, but they did not translate into a clinical benefit in large-scale interventional trials raising questions on to what extent preclinical target engagement can predict a clinical benefit.
Although previous therapies targeting α-synuclein failed to slow disease progression [82, 179], there is converging and convincing preclinical evidence that α-synuclein is a key contributor to disease spread and that its toxic effects promote cell death in MSA [8, 22]. Hence, reduction of CNS α-synuclein load exploiting highly effective novel treatment strategies including immunotherapies, genetic modification and enhancing α-synuclein clearance (through disaggregation or enhancing the autophagy-lysosomal pathway) remains a promising approach to disease modification.
Neuroinflammation and its secondary consequences including oxidative stress are other key contributors to neurodegeneration in MSA. Despite evidence of target engagement on PET imaging, minocycline (an antibiotic with anti-inflammatory effects) failed to improve motor function in a small-scale study [111]. More recently, highly specific drugs tackling key enzymes were studied preclinically. Among these, an irreversible inhibitor of myeloperoxidase evolved to the clinical development stage. Based on encouraging results from early phase clinical studies on MPO inhibitors, a large-scale, phase III study has recently been completed but unfortunately, the trial failed at primary and key secondary endpoints.
Stimulation of neuronal and glial proliferation, enhancing myelination through trophic support and cell replacement therapies are areas that are currently being pursued. An early study with recombinant growth hormone was negative; however, there was a numerical trend towards motor improvement in the growth hormone treated group. The small sample size of the study impedes a final conclusion. Although concerns of potential adverse events were raised, mesenchymal stem cells mediated neuroprotective effects in a small-scale study in MSA-C patients and larger, confirmatory studies are currently underway.
In summary, the large number of ongoing preclinical and clinical efforts with promising interventions foster hope for the discovery of a disease-modifying agent.
ACKNOWLEDGMENTS
The authors would also like to thank all trial participants and researchers involved in the ongoing pre-clinical and clinical research for MSA.
CONFLICT OF INTEREST
WP has acted as paid consultant to Affiris, Alterity, Biogen, Lundbeck and Takeda in relation to their drug development programs in MSA.
KS reports fees from Teva, UCB, Lundbeck, AOP Orphan Pharmaceuticals AG, Roche, Gruenenthal, Stada, Lucher Pharma, Biogen, BIAL and Abbvie, honoraria from the International Parkinson and Movement Disorders Society, research grants from FWF Austrian science fund, Michael J Fox Foundation and AOP Orphan Pharmaceuticals AG outside of the submitted work.
GW reports consultancy and lecture fees from Biogen, Biohaven, Inhibikase, Lundbeck, Ono, Takeda, and Theravance, and research grants from the FWF Austrian Science Fund, the Austrian National Bank, the US MSA-Coalition, Parkinson Fonds Austria, and International Parkinson and Movement Disorder Society outside the submitted work
FK reports receiving personal fees from Institut de Recherches Internationales Servier, Clarion Healthcare and the Austrian Society of Neurology; grant support from the MSA Coalition outside of the submitted work
All other authors declare that they have no conflicts of interest.
This research was funded in whole, or in part, by the Austrian Science Fund (FWF) [Grant I 4795-B]. For the purpose of open access, the author has applied a CC BY public copyright license to any Author Accepted Manuscript version arising from this submission.
All financial and material support for this research and work are clearly identified in the manuscript including listing of support specified in the letter of submission that might constitute or give the appearance of influencing the findings, based on the judgment of the Editor-in-Chief in consultation with the Associate Editor handling the review of the manuscript.
REFERENCES
[1] | Fanciulli A , Wenning GK ((2015) ) Multiple-system atrophy. N Engl J Med 372: , 1375–1376. |
[2] | Gilman S , Wenning GK , Low PA , Brooks DJ , Mathias CJ , Trojanowski JQ , Wood NW , Colosimo C , Dürr A , Fowler CJ , Kaufmann H , Klockgether T , Lees A , Poewe W , Quinn N , Revesz T , Robertson D , Sandroni P , Seppi K , Vidailhet M ((2008) ) Second consensus statement on the diagnosis of multiple system atrophy. Neurology 71: , 670–676. |
[3] | Miki Y , Foti SC , Asi YT , Tsushima E , Quinn N , Ling H , Holton JL ((2019) ) Improving diagnostic accuracy of multiple system atrophy: A clinicopathological study. Brain 142: , 2813–2827. |
[4] | Stefanova N , Kaufmann WA , Humpel C , Poewe W , Wenning GK ((2012) ) Systemic proteasome inhibition triggers neurodegeneration in a transgenic mouse model expressing human α-synuclein under oligodendrocyte promoter: Implications for multiple system atrophy. Acta Neuropathol 124: , 51–65. |
[5] | Stefanova N , Reindl M , Neumann M , Haass C , Poewe W , Kahle PJ , Wenning GK ((2005) ) Oxidative stress in transgenic mice with oligodendroglial alpha-synuclein overexpression replicates the characteristic neuropathology of multiple system atrophy. Am J Pathol 166: , 869–876. |
[6] | Cong S , Xiang C , Wang H ((2021) ) Diagnostic utility of fluid biomarkers in multiple system atrophy: A systematic review and meta-analysis. J Neurol 268: , 2703–2712. |
[7] | Woerman AL , Watts JC , Aoyagi A , Giles K , Middleton LT , Prusiner SB ((2018) ) α-synuclein: Multiple system atrophy prions. Cold Spring Harb Perspect Med 8: , a024588. |
[8] | Jellinger KA ((2018) ) Multiple system atrophy: An oligodendroglioneural synucleinopathy. J Alzheimers Dis 62: , 1141–1179. |
[9] | Monzio Compagnoni G , Di Fonzo A ((2019) ) Understanding the pathogenesis of multiple system atrophy: State of the art and future perspectives. Acta Neuropathol Commun 7: , 113. |
[10] | Herrera-Vaquero M , Heras-Garvin A , Krismer F , Deleanu R , Boesch S , Wenning GK , Stefanova N ((2021) ) Signs of early cellular dysfunction in multiple system atrophy. Neuropathol Appl Neurobiol 47: , 268–282. |
[11] | Bettencourt C , Miki Y , Piras IS , de Silva R , Foti SC , Talboom JS , Revesz T , Lashley T , Balazs R , Viré E , Warner TT , Huentelman MJ , Holton JL ((2021) ) MOBP and HIP1 in multiple system atrophy: New α-synuclein partners in glial cytoplasmic inclusions implicated in the disease pathogenesis. Neuropathol Appl Neurobiol 47: , 640–652. |
[12] | Peelaerts W , Bousset L , Baekelandt V , Melki R ((2018) ) α-Synuclein strains and seeding in Parkinson’s disease, incidental Lewy body disease, dementia with Lewy bodies and multiple system atrophy: Similarities and differences. Cell Tissue Res 373: , 195–212. |
[13] | Vasili E , Dominguez-Meijide A , Outeiro TF ((2019) ) Spreading of α-synuclein and tau: A systematic comparison of the mechanisms involved. Front Mol Neurosci 12: , 107. |
[14] | Tarutani A , Arai T , Murayama S , Hisanaga SI , Hasegawa M ((2018) ) Potent prion-like behaviors of pathogenic α-synuclein and evaluation of inactivation methods. Acta Neuropathol Commun 6: , 29. |
[15] | Dhillon JS , Trejo-Lopez JA , Riffe C , McFarland NR , Hiser WM , Giasson BI , Yachnis AT ((2019) ) Dissecting α-synuclein inclusion pathology diversity in multiple system atrophy: Implications for the prion-like transmission hypothesis. Lab Invest 99: , 982–992. |
[16] | Steiner JA , Quansah E , Brundin P ((2018) ) The concept of alpha-synuclein as a prion-like protein: Ten years after. Cell Tissue Res 373: , 161–173. |
[17] | Karpowicz RJ , Trojanowski JQ , Lee VM ((2019) ) Transmission of α-synuclein seeds in neurodegenerative disease: Recent developments. Lab Invest 99: , 971–981. |
[18] | Brás IC , Outeiro TF ((2021) ) Alpha-synuclein: Mechanisms of release and pathology progression in synucleinopathies. Cells 10: , 375. |
[19] | Jaunmuktane Z , Brandner S ((2020) ) Invited Review: The role of prion-like mechanisms in neurodegenerative diseases. Neuropathol Appl Neurobiol 46: , 522–545. |
[20] | Veys L , Van Houcke J , Aerts J , Van Pottelberge S , Mahieu M , Coens A , Melki R , Moechars D , De Muynck L , De Groef L ((2020) ) Absence of uptake and prion-like spreading of alpha-synuclein and tau after intravitreal injection of preformed fibrils. Front Aging Neurosci 12: , 614587. |
[21] | Woerman AL , Patel S , Kazmi SA , Oehler A , Lee J , Mordes DA , Olson SH , Prusiner SB ((2020) ) Kinetics of α-synuclein prions preceding neuropathological inclusions in multiple system atrophy. PLoS Pathog 16: , e1008222. |
[22] | Prusiner SB , Woerman AL , Mordes DA , Watts JC , Rampersaud R , Berry DB , Patel S , Oehler A , Lowe JK , Kravitz SN , Geschwind DH , Glidden DV , Halliday GM , Middleton LT , Gentleman SM , Grinberg LT , Giles K ((2015) ) Evidence for α-synuclein prions causing multiple system atrophy in humans with parkinsonism. Proc Natl Acad Sci U S A 112: , E5308–5317. |
[23] | Woerman AL , Oehler A , Kazmi SA , Lee J , Halliday GM , Middleton LT , Gentleman SM , Mordes DA , Spina S , Grinberg LT , Olson SH , Prusiner SB ((2019) ) Multiple system atrophy prions retain strain specificity after serial propagation in two different Tg(SNCA*A53T) mouse lines. Acta Neuropathol 137: , 437–454. |
[24] | Watts JC , Giles K , Oehler A , Middleton L , Dexter DT , Gentleman SM , DeArmond SJ , Prusiner SB ((2013) ) Transmission of multiple system atrophy prions to transgenic mice. Proc Natl Acad Sci U S A 110: , 19555–19560. |
[25] | Rey NL , George S , Brundin P ((2016) ) Review: Spreading the word: Precise animal models and validated methods are vital when evaluating prion-like behaviour of alpha-synuclein. Neuropathol Appl Neurobiol 42: , 51–76. |
[26] | Leak RK , Frosch MP , Beach TG , Halliday GM ((2019) ) Alpha-synuclein: Prion or prion-like? Acta Neuropathol 138: , 509–514. |
[27] | Tamgüney G , Korczyn AD ((2018) ) A critical review of the prion hypothesis of human synucleinopathies. Cell Tissue Res 373: , 213–220. |
[28] | Wenning G , Trojanowski JQ , Kaufmann H , Wisniewski T , Rocca WA , Low PA ((2018) ) Is multiple system atrophy an infectious disease? Ann Neurol 83: , 10–12. |
[29] | Scialò C , De Cecco E , Manganotti P , Legname G ((2019) ) Prion and prion-like protein strains: Deciphering the molecular basis of heterogeneity in neurodegeneration. Viruses 11: , 261. |
[30] | Meissner WG , Fernagut PO , Dehay B , Péran P , Traon AP , Foubert-Samier A , Lopez Cuina M , Bezard E , Tison F , Rascol O ((2019) ) Multiple system atrophy: Recent developments and future perspectives. Mov Disord 34: , 1629–1642. |
[31] | Engelender S , Isacson O ((2017) ) The threshold theory for Parkinson’s disease. Trends Neurosci 40: , 4–14. |
[32] | Chartier-Harlin MC , Kachergus J , Roumier C , Mouroux V , Douay X , Lincoln S , Levecque C , Larvor L , Andrieux J , Hulihan M , Waucquier N , Defebvre L , Amouyel P , Farrer M , Destée A ((2004) ) Alpha-synuclein locus duplication as a cause of familial Parkinson’s disease. Lancet 364: , 1167–1169. |
[33] | Dehay B , Bourdenx M , Gorry P , Przedborski S , Vila M , Hunot S , Singleton A , Olanow CW , Merchant KM , Bezard E , Petsko GA , Meissner WG ((2015) ) Targeting α-synuclein for treatment of Parkinson’s disease: Mechanistic and therapeutic considerations. Lancet Neurol 14: , 855–866. |
[34] | Serratos IN , Hernández-Pérez E , Campos C , Aschner M , Santamaría A ((2022) ) An update on the critical role of α-synuclein in Parkinson’s disease and other synucleinopathies: From tissue to cellular and molecular levels. Mol Neurobiol 59: , 620–642. |
[35] | Chatterjee D , Kordower JH ((2019) ) Immunotherapy in Parkinson’s disease: Current status and future directions. Neurobiol Dis 132: , 104587. |
[36] | Mandler M , Valera E , Rockenstein E , Mante M , Weninger H , Patrick C , Adame A , Schmidhuber S , Santic R , Schneeberger A , Schmidt W , Mattner F , Masliah E ((2015) ) Active immunization against alpha-synuclein ameliorates the degenerative pathology and prevents demyelination in a model of multiple system atrophy. Mol Neurodegener 10: , 10. |
[37] | Volc D , Poewe W , Kutzelnigg A , Lührs P , Thun-Hohenstein C , Schneeberger A , Galabova G , Majbour N , Vaikath N , El-Agnaf O , Winter D , Mihailovska E , Mairhofer A , Schwenke C , Staffler G , Medori R ((2020) ) Safety and immunogenicity of the α-synuclein active immunotherapeutic PD01A in patients with Parkinson’s disease: A randomised, single-blinded, phase 1 trial. Lancet Neurol 19: , 591–600. |
[38] | Meissner WG , Traon AP , Foubert-Samier A , Galabova G , Galitzky M , Kutzelnigg A , Laurens B , Lührs P , Medori R , Péran P , Sabatini U , Vergnet S , Volc D , Poewe W , Schneeberger A , Staffler G , Rascol O , Investigators AS ((2020) ) A phase 1 randomized trial of specific active α-synuclein immunotherapies PD01A and PD03A in multiple system atrophy. Mov Disord 35: , 1957–1965. |
[39] | Lundbeck H.A/S (2021) Lundbeck launches a phase II study for potential new treatment of multiple system atro–phy, https://news.cision.com/h–lundbeck-a-s/r/lundbeck-launches-a-phase-ii-study-for-potential-new-treatment-of-multiple-system-atrophy,c3442560 , Last updated November 8, 2021, Accessed on January 10, 2022. |
[40] | Jankovic J , Goodman I , Safirstein B , Marmon TK , Schenk DB , Koller M , Zago W , Ness DK , Griffith SG , Grundman M , Soto J , Ostrowitzki S , Boess FG , Martin-Facklam M , Quinn JF , Isaacson SH , Omidvar O , Ellenbogen A , Kinney GG ((2018) ) Safety and tolerability of multiple ascending doses of PRX002/RG7935, an anti-α-synuclein monoclonal antibody, in patients with Parkinson disease: A randomized clinical trial. JAMA Neurol 75: , 1206–1214. |
[41] | Martins I Prasinezumab Fails to Slow Symptom Worsening in Parkinson’s, But Shows Other Signs of Effectiveness, Trial Finds, https://parkinsonsnewstoday.com/2020/04/24/prasinezumab-fails-to-slow-symptom-worsening-in-parkinsons-trial-finds/, Last updated April 24, 2020, Accessed on January 10, 2022. |
[42] | Weihofen A , Liu Y , Arndt JW , Huy C , Quan C , Smith BA , Baeriswyl JL , Cavegn N , Senn L , Su L , Marsh G , Auluck PK , Montrasio F , Nitsch RM , Hirst WD , Cedarbaum JM , Pepinsky RB , Grimm J , Weinreb PH ((2019) ) Development of an aggregate-selective, human-derived α-synuclein antibody BIIB054 that ameliorates disease phenotypes in Parkinson’s disease models. Neurobiol Dis 124: , 276–288. |
[43] | Masliah E , Rockenstein E , Mante M , Crews L , Spencer B , Adame A , Patrick C , Trejo M , Ubhi K , Rohn TT , Mueller-Steiner S , Seubert P , Barbour R , McConlogue L , Buttini M , Games D , Schenk D ((2011) ) Passive immunization reduces behavioral and neuropathological deficits in an alpha-synuclein transgenic model of Lewy body disease. PLoS One 6: , e19338. |
[44] | Bae EJ , Lee HJ , Rockenstein E , Ho DH , Park EB , Yang NY , Desplats P , Masliah E , Lee SJ ((2012) ) Antibody-aided clearance of extracellular α-synuclein prevents cell-to-cell aggregate transmission. J Neurosci 32: , 13454–13469. |
[45] | Brys M , Fanning L , Hung S , Ellenbogen A , Penner N , Yang M , Welch M , Koenig E , David E , Fox T , Makh S , Aldred J , Goodman I , Pepinsky B , Liu Y , Graham D , Weihofen A , Cedarbaum JM ((2019) ) Randomized phase I clinical trial of anti-α-synuclein antibody BIIB054. Mov Disord 34: , 1154–1163. |
[46] | Orgogozo JM , Gilman S , Dartigues JF , Laurent B , Puel M , Kirby LC , Jouanny P , Dubois B , Eisner L , Flitman S , Michel BF , Boada M , Frank A , Hock C ((2003) ) Subacute meningoencephalitis in a subset of patients with AD after Abeta42 immunization. Neurology 61: , 46–54. |
[47] | Gilman S , Koller M , Black RS , Jenkins L , Griffith SG , Fox NC , Eisner L , Kirby L , Rovira MB , Forette F , Orgogozo JM , Team AQ–S ((2005) ) Clinical effects of Abeta immunization (AN1792) in patients with AD in an interrupted trial. Neurology 64: , 1553–1562. |
[48] | Nicoll JAR , Buckland GR , Harrison CH , Page A , Harris S , Love S , Neal JW , Holmes C , Boche D ((2019) ) Persistent neuropathological effects 14 years following amyloid-β immunization in Alzheimer’s disease. Brain 142: , 2113–2126. |
[49] | Cole TA , Zhao H , Collier TJ , Sandoval I , Sortwell CE , Steece-Collier K , Daley BF , Booms A , Lipton J , Welch M , Berman M , Jandreski L , Graham D , Weihofen A , Celano S , Schulz E , Cole-Strauss A , Luna E , Quach D , Mohan A , Bennett CF , Swayze EE , Kordasiewicz HB , Luk KC , Paumier KL ((2021) ) α-Synuclein antisense oligonucleotides as a disease-modifying therapy for Parkinson’s disease. JCI Insight 6: , e135633. |
[50] | Zhao HT , John N , Delic V , Ikeda-Lee K , Kim A , Weihofen A , Swayze EE , Kordasiewicz HB , West AB , Volpicelli-Daley LA ((2017) ) LRRK2 antisense oligonucleotides ameliorate α-synuclein inclusion formation in a Parkinson’s disease mouse model. Mol Ther Nucleic Acids 8: , 508–519. |
[51] | Benskey MJ , Sellnow RC , Sandoval IM , Sortwell CE , Lipton JW , Manfredsson FP ((2018) ) Silencing alpha synuclein in mature nigral neurons results in rapid neuroinflammation and subsequent toxicity. Front Mol Neurosci 11: , 36. |
[52] | Lewis J , Melrose H , Bumcrot D , Hope A , Zehr C , Lincoln S , Braithwaite A , He Z , Ogholikhan S , Hinkle K , Kent C , Toudjarska I , Charisse K , Braich R , Pandey RK , Heckman M , Maraganore DM , Crook J , Farrer MJ ((2008) ) In vivo silencing of alpha-synuclein using naked siRNA. Mol Neurodegener 3: , 19. |
[53] | Zharikov A , Bai Q , De Miranda BR , Van Laar A , Greenamyre JT , Burton EA ((2019) ) Long-term RNAi knockdown of α-synuclein in the adult rat substantia nigra without neurodegeneration. Neurobiol Dis 125: , 146–153. |
[54] | Rambold AS , Miesbauer M , Olschewski D , Seidel R , Riemer C , Smale L , Brumm L , Levy M , Gazit E , Oesterhelt D , Baier M , Becker CF , Engelhard M , Winklhofer KF , Tatzelt J ((2008) ) Greentea extracts interfere with the stress-protective activity of PrP and the formation of PrP. J Neurochem 107: , 218–229. |
[55] | Ehrnhoefer DE , Bieschke J , Boeddrich A , Herbst M , Masino L , Lurz R , Engemann S , Pastore A , Wanker EE ((2008) ) EGCG redirects amyloidogenic polypeptides into unstructured, off-pathway oligomers. Nat Struct Mol Biol 15: , 558–566. |
[56] | Bieschke J , Russ J , Friedrich RP , Ehrnhoefer DE , Wobst H , Neugebauer K , Wanker EE ((2010) ) EGCG remodels mature alpha-synuclein and amyloid-beta fibrils and reduces cellular toxicity. Proc Natl Acad Sci U S A 107: , 7710–7715. |
[57] | Lorenzen N , Nielsen SB , Yoshimura Y , Vad BS , Andersen CB , Betzer C , Kaspersen JD , Christiansen G , Pedersen JS , Jensen PH , Mulder FA , Otzen DE ((2014) ) How epigallocatechin gallate can inhibit α-synuclein oligomer toxicity in vitro. J Biol Chem 289: , 21299–21310. |
[58] | Chen M , Wang T , Yue F , Li X , Wang P , Li Y , Chan P , Yu S ((2015) ) Tea polyphenols alleviate motor impairments, dopaminergic neuronal injury, and cerebral α-synuclein aggregation in MPTP-intoxicated parkinsonian monkeys. Neuroscience 286: , 383–392. |
[59] | Xu Q , Langley M , Kanthasamy AG , Reddy MB ((2017) ) Epigallocatechin gallate has a neurorescue effect in a mouse model of Parkinson disease. J Nutr 147: , 1926–1931. |
[60] | Levin J , Maaß S , Schuberth M , Giese A , Oertel WH , Poewe W , Trenkwalder C , Wenning GK , Mansmann U , Südmeyer M , Eggert K , Mollenhauer B , Lipp A , Löhle M , Classen J , Münchau A , Kassubek J , Gandor F , Berg D , Egert-Schwender S , Eberhardt C , Paul F , Bötzel K , Ertl-Wagner B , Huppertz HJ , Ricard I , Höglinger GU , Group PS ((2019) ) Safety and efficacy of epigallocatechin gallate in multiple system atrophy (PROMESA): A randomised, double-blind, placebo-controlled trial. Lancet Neurol 18: , 724–735. |
[61] | Levin JM , Schuberth S , Respondek M , Paul G , Mansmann F , Oertel U , Lorenzl WH , Krismer S , Seppi F , Poewe K , Wenning W , Giese G , Bötzel A , Höglinger K , G. PROMESA study group ((2016) ) The PROMESA-protocol: Progression rate of multiple system atrophy under EGCG supplementation as anti-aggregation-approach. J Neural Transm (Vienna) 123: , 439–445. |
[62] | Wrasidlo W , Tsigelny IF , Price DL , Dutta G , Rockenstein E , Schwarz TC , Ledolter K , Bonhaus D , Paulino A , Eleuteri S , Skjevik Å , Kouznetsova VL , Spencer B , Desplats P , Gonzalez-Ruelas T , Trejo-Morales M , Overk CR , Winter S , Zhu C , Chesselet MF , Meier D , Moessler H , Konrat R , Masliah E ((2016) ) A de novo compound targeting α-synuclein improves deficits in models of Parkinson’s disease. Brain 139: , 3217–3236. |
[63] | Price DL , Koike MA , Khan A , Wrasidlo W , Rockenstein E , Masliah E , Bonhaus D ((2018) ) The small molecule alpha-synuclein misfolding inhibitor, NPT200-11, produces multiple benefits in an animal model of Parkinson’s disease. Sci Rep 8: , 16165. |
[64] | Wagner J , Ryazanov S , Leonov A , Levin J , Shi S , Schmidt F , Prix C , Pan-Montojo F , Bertsch U , Mitteregger-Kretzschmar G , Geissen M , Eiden M , Leidel F , Hirschberger T , Deeg AA , Krauth JJ , Zinth W , Tavan P , Pilger J , Zweckstetter M , Frank T , Bähr M , Weishaupt JH , Uhr M , Urlaub H , Teichmann U , Samwer M , Bötzel K , Groschup M , Kretzschmar H , Griesinger C , Giese A ((2013) ) Anle138b: A novel oligomer modulator for disease-modifying therapy of neurodegenerative diseases such as prion and Parkinson’s disease. Acta Neuropathol 125: , 795–813. |
[65] | Heras-Garvin A , Weckbecker D , Ryazanov S , Leonov A , Griesinger C , Giese A , Wenning GK , Stefanova N ((2019) ) Anle138b modulates α-synuclein oligomerization and prevents motor decline and neurodegeneration in a mouse model of multiple system atrophy. Mov Disord 34: , 255–263. |
[66] | Lemos M , Venezia S , Refolo V , Heras-Garvin A , Schmidhuber S , Giese A , Leonov A , Ryazanov S , Griesinger C , Galabova G , Staffler G , Wenning GK , Stefanova N ((2020) ) Targeting α-synuclein by PD03 AFFITOPE® and Anle138b rescues neurodegenerative pathology in a model of multiple system atrophy: Clinical relevance. Transl Neurodegener 9: , 38. |
[67] | Matthias T , MODAG Successfully Completes Phase 1 Study of their Lead Candidate Anle138b and Receives Additional USD 1.4 Million from Michael J. Fox Foun- dation, MODAG, https://www.modag.net/images/202000805MODAGanle138bTrialCompletionMJFFundingEnglish.pdf, Last updated August 05, 2020, Accessed on January 10, 2022. |
[68] | Follmer C , Coelho-Cerqueira E , Yatabe-Franco DY , Araujo GD , Pinheiro AS , Domont GB , Eliezer D ((2015) ) Oligomerization and membrane-binding properties of covalent adducts formed by the interaction of α-synuclein with the toxic dopamine metabolite 3,4-dihydroxyphenylacetaldehyde (DOPAL). J Biol Chem 290: , 27660–27679. |
[69] | Finkelstein DI , Billings JL , Adlard PA , Ayton S , Sedjahtera A , Masters CL , Wilkins S , Shackleford DM , Charman SA , Bal W , Zawisza IA , Kurowska E , Gundlach AL , Ma S , Bush AI , Hare DJ , Doble PA , Crawford S , Gautier EC , Parsons J , Huggins P , Barnham KJ , Cherny RA ((2017) ) The novel compound PBT434 prevents iron mediated neurodegeneration and alpha-synuclein toxicity in multiple models of Parkinson’s disease. Acta Neuropathol Commun 5: , 53. |
[70] | Finkelstein D , Stefanova N , Adlard P , Bradbury M , Stamler D ((2019) ) PBT434 prevents α-synuclein aggregation, neuron loss, motor dysfunction and reduces glial cell inclusions in a transgenic mouse model of multiple system atrophy. Neurology 92: (15 Suppl), P5.8–006. |
[71] | Heras-Garvin A , Refolo V , Schmidt C , Malfertheiner K , Wenning GK , Bradbury M , Stamler D , Stefanova N ((2021) ) ATH434 reduces α-synuclein-related neurodegeneration in a murine model of multiple system atrophy. Mov Disord 36: , 2605–2614. |
[72] | Stamler D , Bradbury M , Wong C , Offman E ((2019) ) A first in human study of PBT434, a novel small molecule inhibitor of α-synuclein aggregation. Neurology 92: (Suppl 15), S4.001. |
[73] | Stamler D , Bradbury M , Wong CW , Offman E ((2020) ) A phase 1 study of PBT434, a novel small molecule inhibitor of α-synuclein aggregation, in adult and older adult volunteers. Neurology 94: (15 Suppl), 4871. |
[74] | Alerity Therapeutics A , European Commission approves Orphan Designation for Alterity’s lead drug candidate, https://alteritytherapeutics.com/investor-centre/news/2020/01/14/european-commission-approves-orphandesignation-for-alteritys-lead-drug-candidate/, Last 1250 updated January 14, 2020, Accessed on January 10, 2022. |
[75] | Ndayisaba A , Wenning GK ((2020) ) Inhibition of the mammalian target or rapamycin (mTOR): A potential therapeutic strategy for multiple system atrophy. Clin Auton Res 30: , 7–8. |
[76] | Cappoli N , Mezzogori D , Tabolacci E , Coletta I , Navarra P , Pani G , Dello Russo C ((2019) ) The mTOR kinase inhibitor rapamycin enhances the expression and release of pro-inflammatory cytokine interleukin 6 modulating the activation of human microglial cells. EXCLI J 18: , 779–798. |
[77] | Lopez-Cuina M , Guerin P , Bezard E , Meissner W , Fernagut P ((2018) ) Rapamycin for treating MSA: A preclinical proof of concept study. Mov Disord 33: (Suppl 2)https://www.mdsabstracts.org/abstract/rapamycin-for-treating-msa-a-preclinical-proof-of-concept-study/. |
[78] | Bai X , Wey MC , Fernandez E , Hart MJ , Gelfond J , Bokov AF , Rani S , Strong R ((2015) ) Rapamycin improves motor function, reduces 4-hydroxynonenal adducted protein in brain, and attenuates synaptic injury in a mouse model of synucleinopathy. Pathobiol Aging Age Relat Dis 5: , 28743. |
[79] | Fellner L , Buchinger E , Brueck D , Irschick R , Wenning GK , Stefanova N ((2018) ) Limited effects of dysfunctional macroautophagy on the accumulation of extracellularly derived α-synuclein in oligodendroglia: Implications for MSA pathogenesis. BMC Neurosci 19: , 32. |
[80] | Li J , Zhu M , Rajamani S , Uversky VN , Fink AL ((2004) ) Rifampicin inhibits alpha-synuclein fibrillation and disaggregates fibrils. Chem Biol 11: , 1513–1521. |
[81] | Ubhi K , Rockenstein E , Mante M , Patrick C , Adame A , Thukral M , Shults C , Masliah E ((2008) ) Rifampicin reduces alpha-synuclein in a transgenic mouse model of multiple system atrophy. Neuroreport 19: , 1271–1276. |
[82] | Low PA , Robertson D , Gilman S , Kaufmann H , Singer W , Biaggioni I , Freeman R , Perlman S , Hauser RA , Cheshire W , Lessig S , Vernino S , Mandrekar J , Dupont WD , Chelimsky T , Galpern WR ((2014) ) Efficacy and safety of rifampicin for multiple system atrophy: A randomised, double-blind, placebo-controlled trial. Lancet Neurol 13: , 268–275. |
[83] | Hebron ML , Lonskaya I , Moussa CE ((2013) ) Nilotinib reverses loss of dopamine neurons and improves motor behavior via autophagic degradation of α-synuclein in Parkinson’s disease models. Hum Mol Genet 22: , 3315–3328. |
[84] | Hebron ML , Lonskaya I , Olopade P , Selby ST , Pagan F , Moussa CE ((2014) ) Tyrosine kinase inhibition regulates early systemic immune changes and modulates the neuroimmune response in α-synucleinopathy. J Clin Cell Immunol 5: , 259. |
[85] | Karuppagounder SS , Brahmachari S , Lee Y , Dawson VL , Dawson TM , Ko HS ((2014) ) The c-Abl inhibitor, nilotinib, protects dopaminergic neurons in a preclinical animal model of Parkinson’s disease. Sci Rep 4: , 4874. |
[86] | Mahul-Mellier AL , Fauvet B , Gysbers A , Dikiy I , Oueslati A , Georgeon S , Lamontanara AJ , Bisquertt A , Eliezer D , Masliah E , Halliday G , Hantschel O , Lashuel HA ((2014) ) c-Abl phosphorylates α-synuclein and regulates its degradation: Implication for α-synuclein clearance and contribution to the pathogenesis of Parkinson’s disease. Hum Mol Genet 23: , 2858–2879. |
[87] | Lopez-Cuina M , Guerin PA , Canron MH , Delamarre A , Dehay B , Bezard E , Meissner WG , Fernagut PO ((2020) ) Nilotinib fails to prevent synucleinopathy and cell loss in a mouse model of multiple system atrophy. Mov Disord 35: , 1163–1172. |
[88] | Pagan F , Hebron M , Valadez EH , Torres-Yaghi Y , Huang X , Mills RR , Wilmarth BM , Howard H , Dunn C , Carlson A , Lawler A , Rogers SL , Falconer RA , Ahn J , Li Z , Moussa C ((2016) ) Nilotinib effects in Parkinson’s disease and dementia with Lewy bodies. J Parkinsons Dis 6: , 503–517. |
[89] | Pagan FL , Hebron ML , Wilmarth B , Torres-Yaghi Y , Lawler A , Mundel EE , Yusuf N , Starr NJ , Anjum M , Arellano J , Howard HH , Shi W , Mulki S , Kurd-Misto T , Matar S , Liu X , Ahn J , Moussa C ((2020) ) Nilotinib effects on safety, tolerability, and potential biomarkers in Parkinson disease: A phase 2 randomized clinical trial. JAMA Neurol 77: , 309–317. |
[90] | Simuni T , Fiske B , Merchant K , Coffey CS , Klingner E , Caspell-Garcia C , Lafontant DE , Matthews H , Wyse RK , Brundin P , Simon DK , Schwarzschild M , Weiner D , Adams J , Venuto C , Dawson TM , Baker L , Kostrzebski M , Ward T , Rafaloff G , Parkinson Study Group NILO-PD Investigators and Collaborators ((2021) ) Efficacy of nilotinib in patients with moderately advanced Parkinson disease: A randomized clinical trial. JAMA Neurol 78: , 312–320. |
[91] | Hou L , Xiong N , Liu L , Huang J , Han C , Zhang G , Li J , Xu X , Lin Z , Wang T ((2015) ) Lithium protects dopaminergic cells from rotenone toxicity via autophagy enhancement. BMC Neurosci 16: , 82. |
[92] | Li XZ , Chen XP , Zhao K , Bai LM , Zhang H , Zhou XP ((2013) ) Therapeutic effects of valproate combined with lithium carbonate on MPTP-induced parkinsonism in mice: Possible mediation through enhanced autophagy. Int J Neurosci 123: , 73–79. |
[93] | Heras-Garvin A , Stefanova N ((2020) ) MSA: From mechanisms to experimental therapeutics. Parkinsonism Relat Disord 73: , 94–104. |
[94] | Saccà F , Marsili A , Quarantelli M , Brescia Morra V , Brunetti A , Carbone R , Pane C , Puorro G , Russo CV , Salvatore E , Tucci T , De Michele G , Filla A ((2013) ) A randomized clinical trial of lithium in multiple system atrophy. J Neurol 260: , 458–461. |
[95] | Hardouin-Lerouge M , Hudhomme P , Sallé M ((2011) ) Molecular clips and tweezers hosting neutral guests. Chem Soc Rev 40: , 30–43. |
[96] | Stefanova N , Kallab M , Ochoa J , Biggs K , Bouquio D , Schrader T , Klärner FG , Poewe W , Wenning GK , Bitan G (2017) argeting alpha-synuclein pathology with the molec1ular tweezer CLR01 in multiple system atrophy. The 13th International Congress of Alzheimer’s and Parkinson’s Diseases, March 29–April 2, 2017; Symposium 07 - Treatment strategies for PD, DLB and MSA. |
[97] | Herrera-Vaquero M , Bouquio D , Kallab M , Biggs K , Nair G , Ochoa J , Heras-Garvin A , Heid C , Hadrovic I , Poewe W , Wenning GK , Klärner FG , Schrader T , Bitan G , Stefanova N ((2019) ) The molecular tweezer CLR01 reduces aggregated, pathologic, and seeding-competent α-synuclein in experimental multiple system atrophy. Biochim Biophys Acta Mol Basis Dis 1865: , 165513. |
[98] | Krishnan R , Tsubery H , Proschitsky MY , Asp E , Lulu M , Gilead S , Gartner M , Waltho JP , Davis PJ , Hounslow AM , Kirschner DA , Inouye H , Myszka DG , Wright J , Solomon B , Fisher RA ((2014) ) A bacteriophage capsid protein provides a general amyloid interaction motif (GAIM) that binds and remodels misfolded protein assemblies. J Mol Biol 426: , 2500–2519. |
[99] | Pujols J , Peña-Díaz S , Lázaro DF , Peccati F , Pinheiro F , González D , Carija A , Navarro S , Conde-Giménez M , García J , Guardiola S , Giralt E , Salvatella X , Sancho J , Sodupe M , Outeiro TF , Dalfó E , Ventura S ((2018) ) Small molecule inhibits α-synuclein aggregation, disrupts amyloid fibrils, and prevents degeneration of dopaminergic neurons.10481-. Proc Natl Acad Sci U S A 115: , 10486. |
[100] | Biospace (2021) Inhibikase Therapeutics Receives Grant from U.S. National Institutes of Health to Evaluate IkT-148009 for the Treatment of Multiple System Atrophy, https://www.biospace.com/article/releases/inhibikasetherapeutics-receives-grant-from-u-s-national-institutesof-health-to-evaluate-ikt-148009-for-the-treatment-ofmultiple-system-atrophy/, Last updated September 23, 2021, Accessed on January 10, 2022. |
[101] | Spencer B , Michael S , Shen J , Kosberg K , Rockenstein E , Patrick C , Adame A , Masliah E ((2013) ) Lentivirus mediated delivery of neurosin promotes clearance of wild-type α-synuclein and reduces the pathology in an α-synuclein model of LBD. Mol Ther 21: , 31–41. |
[102] | Spencer B , Valera E , Rockenstein E , Trejo-Morales M , Adame A , Masliah E ((2015) ) A brain-targeted, modified neurosin (kallikrein-6) reduces α-synuclein accumulation in a mouse model of multiple system atrophy. Mol Neurodegener 10: , 48. |
[103] | Kiely AP , Miners JS , Courtney R , Strand C , Love S , Holton JL ((2019) ) Exploring the putative role of kallikrein-6, calpain-1 and cathepsin-D in the proteolytic degradation of α-synuclein in multiple system atrophy. Neuropathol Appl Neurobiol 45: , 347–360. |
[104] | Vieira BD , Radford RA , Chung RS , Guillemin GJ , Pountney DL ((2015) ) Neuroinflammation in multiple system atrophy: Response to and cause of α-synuclein aggregation. Front Cell Neurosci 9: , 437. |
[105] | Sanchez-Guajardo V , Tentillier N , Romero-Ramos M ((2015) ) The relation between α-synuclein and microglia in Parkinson’s disease: Recent developments. Neuroscience 302: , 47–58. |
[106] | Stefanova N , Georgievska B , Eriksson H , Poewe W , Wenning GK ((2012) ) Myeloperoxidase inhibition ameliorates multiple system atrophy-like degeneration in a transgenic mouse model. Neurotox Res 21: , 393–404. |
[107] | Lim S , Chun Y , Lee JS , Lee SJ ((2016) ) Neuroinflammation in synucleinopathies. Brain Pathol 26: , 404–409. |
[108] | Jucaite A , Cselényi Z , Kreisl WC , Rabiner EA , Varrone A , Carson RE , Rinne JO , Savage A , Schou M , Johnström P , Svenningsson P , Rascol O , Meissner WG , Barone P , Seppi K , Kaufmann H , Wenning GK , Poewe W , Farde L ((2022) ) Glia imaging differentiates multiple system atrophy from Parkinson’s disease: A positron emission tomography study with [11 C]PBR28 and machine learning analysis. Mov Disord 37: , 119–129. |
[109] | Yamasaki R , Yamaguchi H , Matsushita T , Fujii T , Hiwatashi A , Kira JI ((2017) ) Early strong intrathecal inflammation in cerebellar type multiple system atrophy by cerebrospinal fluid cytokine/chemokine profiles: A case control study. J Neuroinflammation 14: , 89. |
[110] | Ryu JK , Franciosi S , Sattayaprasert P , Kim SU , McLarnon JG ((2004) ) Minocycline inhibits neuronal death and glial activation induced by beta-amyloid peptide in rat hippocampus. Glia 48: , 85–90. |
[111] | Dodel R , Spottke A , Gerhard A , Reuss A , Reinecker S , Schimke N , Trenkwalder C , Sixel-Döring F , Herting B , Kamm C , Gasser T , Sawires M , Geser F , Köllensperger M , Seppi K , Kloss M , Krause M , Daniels C , Deuschl G , Böttger S , Naumann M , Lipp A , Gruber D , Kupsch A , Du Y , Turkheimer F , Brooks DJ , Klockgether T , Poewe W , Wenning G , Schade-Brittinger C , Oertel WH , Eggert K ((2010) ) Minocycline 1-year therapy in multiple-system-atrophy: Effect on clinical symptoms and [(11)C] (R)-PK11195 PET (MEMSA-trial). Mov Disord 25: , 97–107. |
[112] | Ishizawa K , Komori T , Sasaki S , Arai N , Mizutani T , Hirose T ((2004) ) Microglial activation parallels system degeneration in multiple system atrophy. J Neuropathol Exp Neurol 63: , 43–52. |
[113] | Langerveld AJ , Mihalko D , DeLong C , Walburn J , Ide CF ((2007) ) Gene expression changes in postmortem tissue from the rostral pons of multiple system atrophy patients. Mov Disord 22: , 766–777. |
[114] | Novak P , Williams A , Ravin P , Zurkiya O , Abduljalil A , Novak V ((2012) ) Treatment of multiple system atrophy using intravenous immunoglobulin. BMC Neurol 12: , 131. |
[115] | Choi DK , Pennathur S , Perier C , Tieu K , Teismann P , Wu DC , Jackson-Lewis V , Vila M , Vonsattel JP , Heinecke JW , Przedborski S ((2005) ) Ablation of the inflammatory enzyme myeloperoxidase mitigates features of Parkinson’s disease in mice. J Neurosci 25: , 6594–6600. |
[116] | Green PS , Mendez AJ , Jacob JS , Crowley JR , Growdon W , Hyman BT , Heinecke JW ((2004) ) Neuronal expression of myeloperoxidase is increased in Alzheimer’s disease. J Neurochem 90: , 724–733. |
[117] | Gray E , Thomas TL , Betmouni S , Scolding N , Love S ((2008) ) Elevated activity and microglial expression of myeloperoxidase in demyelinated cerebral cortex in multiple sclerosis. Brain Pathol 18: , 86–95. |
[118] | Kaindlstorfer C , Sommer P , Georgievska B , Mather RJ , Kugler AR , Poewe W , Wenning GK , Stefanova N ((2015) ) Failure of neuroprotection despite microglial suppression by delayed-start myeloperoxidase inhibition in a model of advanced multiple system atrophy: Clinical implications. Neurotox Res 28: , 185–194. |
[119] | Jucaite A , Svenningsson P , Rinne JO , Cselényi Z , Varnäs K , Johnström P , Amini N , Kirjavainen A , Helin S , Minkwitz M , Kugler AR , Posener JA , Budd S , Halldin C , Varrone A , Farde L ((2015) ) Effect of the myeloperoxidase inhibitor AZD3241 on microglia: A PET study in Parkinson’s disease. Brain 138: , 2687–2700. |
[120] | Biohaven pharmaceuticals (2021) Biohaven provides update on phase 3 trial and multiple system atrophy (MSA) program, https://www.biohavenpharma.com/investors/news-events/press-releases/09-27-2021, Last updated September 27, 2021, Accessed on January 10, 2022. |
[121] | Wang W , Nguyen LT , Burlak C , Chegini F , Guo F , Chataway T , Ju S , Fisher OS , Miller DW , Datta D , Wu F , Wu CX , Landeru A , Wells JA , Cookson MR , Boxer MB , Thomas CJ , Gai WP , Ringe D , Petsko GA , Hoang QQ ((2016) ) Caspase-1 causes truncation and aggregation of the Parkinson’s disease-associated protein α-synuclein. Proc Natl Acad Sci U S A 113: , 9587–9592. |
[122] | Bassil F , Fernagut PO , Bezard E , Pruvost A , Leste-Lasserre T , Hoang QQ , Ringe D , Petsko GA , Meissner WG ((2016) ) Reducing C-terminal truncation mitigates synucleinopathy and neurodegeneration in a transgenic model of multiple system atrophy. Proc Natl Acad Sci U S A 113: , 9593–9598. |
[123] | Valera E , Spencer B , Fields JA , Trinh I , Adame A , Mante M , Rockenstein E , Desplats P , Masliah E ((2017) ) Combination of alpha-synuclein immunotherapy with anti-inflammatory treatment in a transgenic mouse model of multiple system atrophy. Acta Neuropathol Commun 5: , 2. |
[124] | Vargas-Medrano J , Segura-Ulate I , Yang B , Chinnasamy R , Arterburn JB , Perez RG ((2019) ) FTY720-Mitoxy reduces toxicity associated with MSA-like α-synuclein and oxidative stress by increasing trophic factor expression and myelin protein in OLN-93 oligodendroglia cell cultures. Neuropharmacology 158: , 107701. |
[125] | Segura-Ulate I , Yang B , Vargas-Medrano J , Perez RG ((2017) ) FTY720 (Fingolimod) reverses α-synuclein-induced downregulation of brain-derived neurotrophic factor mRNA in OLN-93 oligodendroglial cells. Neuropharmacology 117: , 149–157. |
[126] | Vidal-Martinez G , Segura-Ulate I , Yang B , Diaz-Pacheco V , Barragan JA , De-Leon Esquivel J , Chaparro SA , Vargas-Medrano J , Perez RG ((2020) ) FTY720-Mitoxy reduces synucleinopathy and neuroinflammation, restores behavior and mitochondria function, and increases GDNF expression in multiple system atrophy mouse models. Exp Neurol 325: , 113120. |
[127] | Panagiotakopoulou V , Botsakis K , Delis F , Mourtzi T , Tzatzarakis MN , Dimopoulou A , Poulia N , Antoniou K , Stathopoulos GT , Matsokis N , Charalampopoulos I , Gravanis A , Angelatou F ((2020) ) Anti-neuroinflammatory, protective effects of the synthetic microneurotrophin BNN-20 in the advanced dopaminergic neurodegeneration of “weaver” mice. Neuropharmacology 165: , 107919. |
[128] | Betarbet R , Anderson LR , Gearing M , Hodges TR , Fritz JJ , Lah JJ , Levey AI ((2008) ) Fas-associated factor 1 and Parkinson’s disease. Neurobiol Dis 31: , 309–315. |
[129] | Sul JW , Park MY , Shin J , Kim YR , Yoo SE , Kong YY , Kwon KS , Lee YH , Kim E ((2013) ) Accumulation of the parkin substrate, FAF1, plays a key role in the dopaminergic neurodegeneration. Hum Mol Genet 22: , 1558–1573. |
[130] | Shin W , Lim KS , Kim MK , Kim HS , Hong J , Jhee S , Kim J , Yoo S , Chung YT , Lee JM , Cho DY ((2019) ) A first-in-human study to investigate the safety, tolerability, pharmacokinetics, and pharmacodynamics of KM-819 (FAS-associated factor 1 inhibitor), a drug for Parkinson’s disease, in healthy volunteers. Drug Des Devel Ther 13: , 1011–1022. |
[131] | Park HS , Song YS , Moon BS , Yoo SE , Lee JM , Chung YT , Kim E , Lee BC , Kim SE ((2020) ) Neurorestorative effects of a novel Fas-associated factor 1 inhibitor in the MPTP model: An [18 F]FE-PE2I positron emission tomography analysis study. Front Pharmacol 11: , 953. |
[132] | Sharon R , Bar-Joseph I , Frosch MP , Walsh DM , Hamilton JA , Selkoe DJ ((2003) ) The formation of highly soluble oligomers of alpha-synuclein is regulated by fatty acids and enhanced in Parkinson’s disease. Neuron 37: , 583–595. |
[133] | Fanning S , Haque A , Imberdis T , Baru V , Barrasa MI , Nuber S , Termine D , Ramalingam N , Ho GPH , Noble T , Sandoe J , Lou Y , Landgraf D , Freyzon Y , Newby G , Soldner F , Terry-Kantor E , Kim TE , Hofbauer HF , Becuwe M , Jaenisch R , Pincus D , Clish CB , Walther TC , Farese RV , Srinivasan S , Welte MA , Kohlwein SD , Dettmer U , Lindquist S , Selkoe D ((2019) ) Lipidomic analysis of α-Synuclein neurotoxicity identifies stearoyl CoA desaturase as a target for Parkinson treatment. Mol Cell 73: , 1001–1014.e1008. |
[134] | Vincent BM , Tardiff DF , Piotrowski JS , Aron R , Lucas MC , Chung CY , Bacherman H , Chen Y , Pires M , Subramaniam R , Doshi DB , Sadlish H , Raja WK , Solís EJ , Khurana V , Le Bourdonnec B , Scannevin RH , Rhodes KJ ((2018) ) Inhibiting stearoyl-CoA desaturase ameliorates α-synuclein cytotoxicity. Cell Rep 25: , 2742–2754.e2731. |
[135] | Cheah BC , Vucic S , Krishnan AV , Kiernan MC ((2010) ) Riluzole, neuroprotection and amyotrophic lateral sclerosis. Curr Med Chem 17: , 1942–1199. |
[136] | Scherfler C , Sather T , Diguet E , Stefanova N , Puschban Z , Tison F , Poewe W , Wenning GK ((2005) ) Riluzole improves motor deficits and attenuates loss of striatal neurons in a sequential double lesion rat model of striatonigral degeneration (Parkinson variant of multiple system atrophy). J Neural Transm (Vienna) 112: , 1025–1033. |
[137] | Seppi K , Peralta C , Diem-Zangerl A , Puschban Z , Mueller J , Poewe W , Wenning GK ((2006) ) Placebo-controlled trial of riluzole in multiple system atrophy. Eur J Neurol 13: , 1146–1148. |
[138] | Bensimon G , Ludolph A , Agid Y , Vidailhet M , Payan C , Leigh PN , Group NS ((2009) ) Riluzole treatment, survival and diagnostic criteria in Parkinson plus disorders: The NNIPPS study. Brain 132: , 156–171. |
[139] | Sanchez-Perez A , Llansola M , Cauli O , Felipo V ((2005) ) Modulation of NMDA receptors in the cerebellum. II. Signaling pathways and physiological modulators regulating NMDA receptor function. Cerebellum 4: , 162–170. |
[140] | Shemesh E , Rudich A , Harman-Boehm I , Cukierman-Yaffe T ((2012) ) Effect of intranasal insulin on cognitive function: A systematic review. J Clin Endocrinol Metab 97: , 366–376. |
[141] | Bassil F , Fernagut PO , Bezard E , Meissner WG ((2014) ) Insulin, IGF-1 and GLP-1 signaling in neurodegenerative disorders: Targets for disease modification? Prog Neurobiol 118: , 1–18. |
[142] | Pellecchia MT , Pivonello R , Longo K , Manfredi M , Tessitore A , Amboni M , Pivonello C , Rocco M , Cozzolino A , Colao A , Barone P ((2010) ) Multiple system atrophy is associated with changes in peripheral insulin-like growth factor system. Mov Disord 25: , 2621–2626. |
[143] | Numao A , Suzuki K , Miyamoto M , Miyamoto T , Hirata K ((2014) ) Clinical correlates of serum insulin-like growth factor-1 in patients with Parkinson’s disease, multiple system atrophy and progressive supranuclear palsy. Parkinsonism Relat Disord 20: , 212–216. |
[144] | Bassil F , Canron MH , Vital A , Bezard E , Li Y , Greig NH , Gulyani S , Kapogiannis D , Fernagut PO , Meissner WG ((2017) ) Insulin resistance and exendin-4 treatment for multiple system atrophy. Brain 140: , 1420–1436. |
[145] | Novak P , Pimentel Maldonado DA , Novak V ((2019) ) Safety and preliminary efficacy of intranasal insulin for cognitive impairment in Parkinson disease and multiple system atrophy: A double-blinded placebo-controlled pilot study. PLoS One 14: , e0214364. |
[146] | Collaboration M-SAR ((2013) ) Mutations in COQ2 in familial and sporadic multiple-system atrophy. N Engl J Med 369: , 233–244. |
[147] | Monzio Compagnoni G , Kleiner G , Samarani M , Aureli M , Faustini G , Bellucci A , Ronchi D , Bordoni A , Garbellini M , Salani S , Fortunato F , Frattini E , Abati E , Bergamini C , Fato R , Tabano S , Miozzo M , Serratto G , Passafaro M , Deleidi M , Silipigni R , Nizzardo M , Bresolin N , Comi GP , Corti S , Quinzii CM , Di Fonzo A ((2018) ) Mitochondrial dysregulation and impaired autophagy in iPSC-derived dopaminergic neurons of multiple system atrophy. Stem Cell Rep 11: , 1185–1198. |
[148] | Nakamoto FK , Okamoto S , Mitsui J , Sone T , Ishikawa M , Yamamoto Y , Kanegae Y , Nakatake Y , Imaizumi K , Ishiura H , Tsuji S , Okano H ((2018) ) The pathogenesis linked to coenzyme Q10 insufficiency in iPSC-derived neurons from patients with multiple-system atrophy. Sci Rep 8: , 14215. |
[149] | Mitsui J , Koguchi K , Momose T , Takahashi M , Matsukawa T , Yasuda T , Tokushige SI , Ishiura H , Goto J , Nakazaki S , Kondo T , Ito H , Yamamoto Y , Tsuji S ((2017) ) Three-year follow-up of high-dose ubiquinol supplementation in a case of familial multiple system atrophy with compound heterozygous COQ2 mutations. Cerebellum 16: , 664–672. |
[150] | Ajo R , Cacicedo L , Navarro C , Sánchez-Franco F ((2003) ) Growth hormone action on proliferation and differentiation of cerebral cortical cells from fetal rat. Endocrinology 144: , 1086–1097. |
[151] | Lichtenwalner RJ , Forbes ME , Sonntag WE , Riddle DR ((2006) ) Adult-onset deficiency in growth hormone and insulin-like growth factor-I decreases survival of dentate granule neurons: Insights into the regulation of adult hippocampal neurogenesis. J Neurosci Res 83: , 199–210. |
[152] | Holmberg B , Johansson JO , Poewe W , Wenning G , Quinn NP , Mathias C , Tolosa E , Cardozo A , Dizdar N , Rascol O , Slaoui T , Growth-Hormone MSA Study Group; European MSA Study Group ((2007) ) Safety and tolerability of growth hormone therapy in multiple system atrophy: A double-blind, placebo-controlled study. Mov Disord 22: , 1138–1144. |
[153] | Lee PH , Kim JW , Bang OY , Ahn YH , Joo IS , Huh K ((2008) ) Autologous mesenchymal stem cell therapy delays the progression of neurological deficits in patients with multiple system atrophy. Clin Pharmacol Ther 83: , 723–730. |
[154] | Quinn N , Barker RA , Wenning GK ((2008) ) Are trials of intravascular infusions of autologous mesenchymal stem cells in patients with multiple system atrophy currently justified, and are they effective? Clin Pharmacol Ther 83: , 663–665. |
[155] | Stemberger S , Jamnig A , Stefanova N , Lepperdinger G , Reindl M , Wenning GK ((2011) ) Mesenchymal stem cells in a transgenic mouse model of multiple system atrophy: Immunomodulation and neuroprotection. PLoS One 6: , e19808. |
[156] | Lee PH , Lee JE , Kim HS , Song SK , Lee HS , Nam HS , Cheong JW , Jeong Y , Park HJ , Kim DJ , Nam CM , Lee JD , Kim HO , Sohn YH ((2012) ) A randomized trial of mesenchymal stem cells in multiple system atrophy. Ann Neurol 72: , 32–40. |
[157] | Singer W , Dietz AB , Zeller AD , Gehrking TL , Schmelzer JD , Schmeichel AM , Gehrking JA , Suarez MD , Sletten DM , Minota Pacheco KV , Coon EA , Sandroni P , Benarroch EE , Fealey RD , Matsumoto JY , Bower JH , Hassan A , McKeon A , Windebank AJ , Mandrekar JN , Low PA ((2019) ) Intrathecal administration of autologous mesenchymal stem cells in multiple system atrophy.e77-e. Neurology 93: , e77–e87. |
[158] | Olanow CW , Rascol O , Hauser R , Feigin PD , Jankovic J , Lang A , Langston W , Melamed E , Poewe W , Stocchi F , Tolosa E , ADAGIO Study Investigators ((2009) ) A double-blind, delayed-start trial of rasagiline in Parkinson’s disease. N Engl J Med 361: , 1268–1278. |
[159] | Stefanova N , Poewe W , Wenning GK ((2008) ) Rasagiline is neuroprotective in a transgenic model of multiple system atrophy. Exp Neurol 210: , 421–427. |
[160] | Poewe W , Seppi K , Fitzer-Attas CJ , Wenning GK , Gilman S , Low PA , Giladi N , Barone P , Sampaio C , Eyal E , Rascol O , Rasagiline-for-MSA investigators ((2015) ) Efficacy of rasagiline in patients with the parkinsonian variant of multiple system atrophy: A randomised, placebo-controlled trial. Lancet Neurol 14: , 145–152. |
[161] | Ubhi K , Inglis C , Mante M , Patrick C , Adame A , Spencer B , Rockenstein E , May V , Winkler J , Masliah E ((2012) ) Fluoxetine ameliorates behavioral and neuropathological deficits in a transgenic model mouse of α-synucleinopathy. Exp Neurol 234: , 405–416. |
[162] | Zhang F , Zhou H , Wilson BC , Shi JS , Hong JS , Gao HM ((2012) ) Fluoxetine protects neurons against microglial activation-mediated neurotoxicity. Parkinsonism Relat Disord 18: Suppl 1, S213–217. |
[163] | Rascol O , Cochen de Cock V , Pavy-Le Traon A , Foubert-Samier A , Thalamas C , Sommet A , Rousseau V , Perez-Lloret S , Fabbri M , Azulay JP , Corvol JC , Couratier P , Damier P , Defebvre L , Durif F , Geny C , Houeto JL , Remy P , Tranchant C , Verin M , Tison F , Meissner WG , MSA-FLUO Study Group ((2021) ) Fluoxetine for the symptomatic treatment of multiple system atrophy: The MSA-FLUO Trial. Mov Disord 36: , 1704–1711. |
[164] | Coon EA , Ahlskog JE , Silber MH , Fealey RD , Benarroch EE , Sandroni P , Mandrekar JN , Low PA , Singer W ((2018) ) Do selective serotonin reuptake inhibitors improve survival in multiple system atrophy? Parkinsonism Relat Disord 48: , 51–53. |
[165] | Simons M , Nave KA ((2015) ) Oligodendrocytes: Myelination and axonal support. Cold Spring Harb Perspect Biol 8: , a020479. |
[166] | Ettle B , Kerman BE , Valera E , Gillmann C , Schlachetzki JC , Reiprich S , Büttner C , Ekici AB , Reis A , Wegner M , Bäuerle T , Riemenschneider MJ , Masliah E , Gage FH , Winkler J ((2016) ) α-Synuclein-induced myelination deficit defines a novel interventional target for multiple system atrophy. Acta Neuropathol 132: , 59–75. |
[167] | Fellner L , Irschick R , Schanda K , Reindl M , Klimaschewski L , Poewe W , Wenning GK , Stefanova N ((2013) ) Toll-like receptor 4 is required for α-synuclein dependent activation of microglia and astroglia. Glia 61: , 349–360. |
[168] | Stefanova N , Fellner L , Reindl M , Masliah E , Poewe W , Wenning GK ((2011) ) Toll-like receptor 4 promotes α-synuclein clearance and survival of nigral dopaminergic neurons. Am J Pathol 179: , 954–963. |
[169] | Venezia S , Refolo V , Polissidis A , Stefanis L , Wenning GK , Stefanova N ((2017) ) Toll-like receptor 4 stimulation with monophosphoryl lipid A ameliorates motor deficits and nigral neurodegeneration triggered by extraneuronal α-synucleinopathy. Mol Neurodegener 12: , 52. |
[170] | Kontopoulos E , Parvin JD , Feany MB ((2006) ) Alpha-synuclein acts in the nucleus to inhibit histone acetylation and promote neurotoxicity. Hum Mol Genet 15: , 3012–3023. |
[171] | Sturm E , Fellner L , Krismer F , Poewe W , Wenning GK , Stefanova N ((2016) ) Neuroprotection by epigenetic modulation in a transgenic model of multiple system atrophy. Neurotherapeutics 13: , 871–879. |
[172] | Laurens B , Constantinescu R , Freeman R , Gerhard A , Jellinger K , Jeromin A , Krismer F , Mollenhauer B , Schlossmacher MG , Shaw LM , Verbeek MM , Wenning GK , Winge K , Zhang J , Meissner WG ((2015) ) Fluid biomarkers in multiple system atrophy: A review of the MSA Biomarker Initiative. Neurobiol Dis 80: , 29–41. |
[173] | Capotosti F (2022); Discovery of [18F] ACI-12589, a novel and promising PET-tracer for alpha-synuclein. Oral presentation; ADPD 2022 International Conference; Barcelona, Spain; March 18, 2022. |
[174] | Stefanova N , Wenning GK ((2016) ) Review: Multiple system atrophy: Emerging targets for interventional therapies. Neuropathol Appl Neurobiol 42: , 20–32. |
[175] | Stefanova N , Wenning GK ((2015) ) Animal models of multiple system atrophy. Clin Auton Res 25: , 9–17. |
[176] | Marmion DJ , Rutkowski AA , Chatterjee D , Hiller BM , Werner MH , Bezard E , Kirik D , McCown T , Gray SJ , Kordower JH ((2021) ) Viral-based rodent and nonhuman primate models of multiple system atrophy: Fidelity to the human disease. Neurobiol Dis 148: , 105184. |
[177] | Fellner L , Wenning GK , Stefanova N ((2015) ) Models of multiple system atrophy. Curr Top Behav Neurosci 22: , 369–393. |
[178] | Wenning GK , Jellinger KA ((2005) ) The role of alpha-synuclein in the pathogenesis of multiple system atrophy. Acta Neuropathol 109: , 129–140. |
[179] | Levin J , Maass S , Schuberth M , Giese A , Wolfgang O , Werner P , Trenkwalder C , Gregor W , Mansmann U , Suedmeyer M , Eggert K , Mollenhauer B , Lipp A , Loehle M , Classen J , Muenchau A , Kassubeck J , Ebersbach G , Berg D , Egert S , Eberhardt C , Paul F , Boetzel K , Ertl-Wagner B , Huppertz H , Ricard I , Hoeglinger G ((2018) ) PROMESA: A randomised, double-blind, placebo-controlled trial to evaluate the progression rate of MSA under EGCG sup-plementation as anti-aggregation-approach. Mov Disord 33: (Suppl 2), https://www.mdsabstracts.org/abstract/promesa-a-randomised-double-blind-placebo-controlled-trial-to-evaluate-the-progression-rate-of-msa-under-egcg-supplementation-as-anti-aggregation-approach/ |