The Role of Microelectrode Recording and Stereotactic Computed Tomography in Verifying Lead Placement During Awake MRI-Guided Subthalamic Nucleus Deep Brain Stimulation for Parkinson’s Disease
Abstract
Background:
Bilateral deep brain stimulation of the subthalamic nucleus (STN-DBS) has become a cornerstone in the advanced treatment of Parkinson’s disease (PD). Despite its well-established clinical benefit, there is a significant variation in the way surgery is performed. Most centers operate with the patient awake to allow for microelectrode recording (MER) and intraoperative clinical testing. However, technical advances in MR imaging and MRI-guided surgery raise the question whether MER and intraoperative clinical testing still have added value in DBS-surgery.
Objective:
To evaluate the added value of MER and intraoperative clinical testing to determine final lead position in awake MRI-guided and stereotactic CT-verified STN-DBS surgery for PD.
Methods:
29 consecutive patients were analyzed retrospectively. Patients underwent awake bilateral STN-DBS with MER and intraoperative clinical testing. The role of MER and clinical testing in determining final lead position was evaluated. Furthermore, interobserver variability in determining the MRI-defined STN along the planned trajectory was investigated. Clinical improvement was evaluated at 12 months follow-up and adverse events were recorded.
Results:
98% of final leads were placed in the central MER-track with an accuracy of 0.88±0.45 mm. Interobserver variability of the MRI-defined STN was 0.84±0.09. Compared to baseline, mean improvement in MDS-UPDRS-III, PDQ-39 and LEDD were 26.7±16.0 points (54%) (p < 0.001), 9.0±20.0 points (19%) (p = 0.025), and 794±434 mg/day (59%) (p < 0.001) respectively. There were 19 adverse events in 11 patients, one of which (lead malposition requiring immediate postoperative revision) was a serious adverse event.
Conclusion:
MER and intraoperative clinical testing had no additional value in determining final lead position. These results changed our daily clinical practice to an asleep MRI-guided and stereotactic CT-verified approach.
INTRODUCTION
Bilateral deep brain stimulation (DBS) of the subthalamic nucleus (STN) has become a cornerstone in the advanced treatment of Parkinson’s disease (PD) [1, 2]. Although the clinical benefit of STN-DBS is well-established, there is a significant variation in the way the surgical procedure is performed across centers [3]. Most centers adopt a more traditional approach under local anesthesia with the patient awake and in the OFF-medication state, to allow for intraoperative microelectrode recording (MER) and clinical testing. The intended target is usually determined based on standard brain atlas coordinates of the STN in relation to anatomical landmarks, such as the anterior and posterior commissure, which were formerly visualized on ventriculography and later on computed tomography (CT) or magnetic resonance imaging (MRI) [4]. Since this method of indirect targeting does not account for intra- and interindividual variability in position, size, and orientation of the STN [5], MER and intraoperative clinical testing are used to further refine the final target.
MER involves insertion of several microelectrodes into the brain and is used as a tool to indirectly identify the neurophysiological location and borders of the STN based on typical neuronal firing patterns. Moreover, it provides the opportunity to apply test stimulation to immediately assess the clinical benefit of stimulation and the threshold for adverse effects. Although it is generally accepted that MER improves the accuracy of lead placement, some reports question the anatomical accuracy of this approach based on post-mortem histopathological evaluation of lead placement [6–8]. Furthermore, the use of MER is associated with a longer operative time, an increase in costs and some studies report an increased risk of hemorrhage [9–11].
With the advancements in MRI-techniques, functional neurosurgeons can now rely on direct visualization of the iron-containing STN on 1.5 Tesla (T), 3.0T and 7.0T MR scanners. Therefore, some centers started to adopt an MRI-guided approach, without the use of MER and intraoperative clinical testing, and with verification of lead placement on an immediate post-operative CT or MRI. These procedures were initially performed with the patient awake, and later under general anesthesia [12, 13]. Long-term clinical outcome has been shown to be correlated with the location of the stimulation site within the MRI-defined STN [14], suggesting that a surgical procedure under general anaesthesia based on ‘direct anatomical targeting’ that allows for accurate and precise lead placement could be a good alternative for the awake and MER-guided procedure. Furthermore, direct targeting has the advantage of being able to optimally correct for inter- and intraindividual variation in location, orientation and size of the STN [15].
These technical advancements raise the question whether MER and intraoperative clinical testing still have an added value if the quality of MR imaging and the accuracy of the operative method are sufficient. In this study, we retrospectively evaluated the added value of MER and intraoperative clinical testing in determining final lead position in an awake MRI-guided and stereotactic CT-verified approach. Despite MER can be performed in both awake and asleep surgery, this study will focus on the added value of MER only.
METHODS
Patient selection
Data from twenty-nine consecutive patients with PD, who underwent awake bilateral STN-DBS between March 2018 and July 2019 at the Radboud University Medical Center, were reviewed retrospectively. Eligible patients had PD, with an unequivocal reduction of at least 30% in OFF-phase symptoms on levodopa, and at least one of the following symptoms despite optimal pharmacologic treatment: cumbersome motor fluctuations, dyskinesia, and/or drug-resistant tremor. Before inclusion, they all underwent an extensive multidisciplinary screening to decide on suitability of bilateral STN-DBS. The screening consisted of a levodopa challenge test to confirm levodopa-responsiveness based on the Movement Disorder Society Unified Parkinson’s Disease Rating Scale (MDS-UPDRS) part III, a battery of questionnaires including all other parts of the MDS-UPDRS and the Parkinson’s Disease Questionnaire-39, both neuropsychological and psychiatric evaluation to exclude patients with significant cognitive impairment and/or psychiatric comorbidity, and a structural MRI to rule out surgical contraindications. The study was approved by the medical ethics committee and all patients gave their written informed consent for their anonymized data to be used for research.
Surgical procedure
All patients were operated under local anesthesia and in the OFF-medication state to allow for MER and intraoperative clinical testing for therapeutic benefit and side-effects. Anti-parkinsonian medication was ceased 12 h prior to surgery. The first nine patients underwent a series of MRI sequences the day prior to surgery on a 3T Siemens Prisma MRI scanner (Siemens, Erlangen, Germany). Sequences included a 3D T2 SPACE to visualize the STN and a 3D T1 after administration of intravenous gadolinium to plan the trajectory. For scanning parameters, see the Supplementary Material. On the day of surgery, a Leksell Vantage stereotactic frame (Elekta Instrument AB, Stockholm, Sweden) was attached to the head under local anesthesia and a total scalp block. The patient was transferred to the intraoperative MRI suite and a stereotactic 3D T1 MPRAGE was acquired on a 3T Siemens Skyra (Siemens, Erlangen, Germany). The frame was subsequently localized by co-registering the preoperative MRI dataset to the intraoperative stereotactic 3D T1 MPRAGE. For the other 20 patients, all imaging was performed intraoperatively. They were briefly put under general anesthesia with short-acting agents that would not interfere with MER. A Leksell Vantage stereotactic frame was subsequently attached to the head and the patient was transferred to the intraoperative MRI suite. MRI sequences were acquired on 3T Siemens Skyra MRI scanner and included a tailored stereotactic 3D T2 SPACE to visualize the STN and localize the frame, and a 3D T1 MPRAGE after administration of intravenous gadolinium to plan the trajectory. See the Supplementary Material for MRI parameters. Target selection, planning of the trajectory, and localization of the frame was done by using Brainlab Elements software (Brainlab, Munich, Germany). The STN was defined as the T2-hypointense structure lateral to the red nucleus. The final target point was defined at the level of the maximal rubral diameter (5–6 mm below the AC-PC plane) and just posterolateral to the target point described by Bejjani et al. [16] (Fig. 1). The entry point was defined at the medial aspect of the middle frontal gyrus, at or just behind the coronal suture. Care was taken that the trajectory would avoid sulci, subcortical vessels, the caudate nucleus, and the ventricular system. General anesthesia was reversed after the MRI scan and the patient was positioned on the operating table, with the frame mounted and fixed to the table.
Fig. 1
Visualization of the STN, MRI-guided targeting and immediate postoperative verification of final electrode position. Axial stereotactic 3D T2-weighted SPACE MRI at 3.0 T through the inferior portion of the STN. This sequence is used for both targeting the STN and localization of the Leksell Vantage frame. Blue and red bullets are indicating the patient-specific intended target at the left and right side respectively, with the corresponding lines indicating the planned trajectories. The orange metal artefacts indicate the position of the final electrodes of the same patient, verified by co-registering an immediate postoperative stereotactic CT to the 3D T2-weighted SPACE MRI.
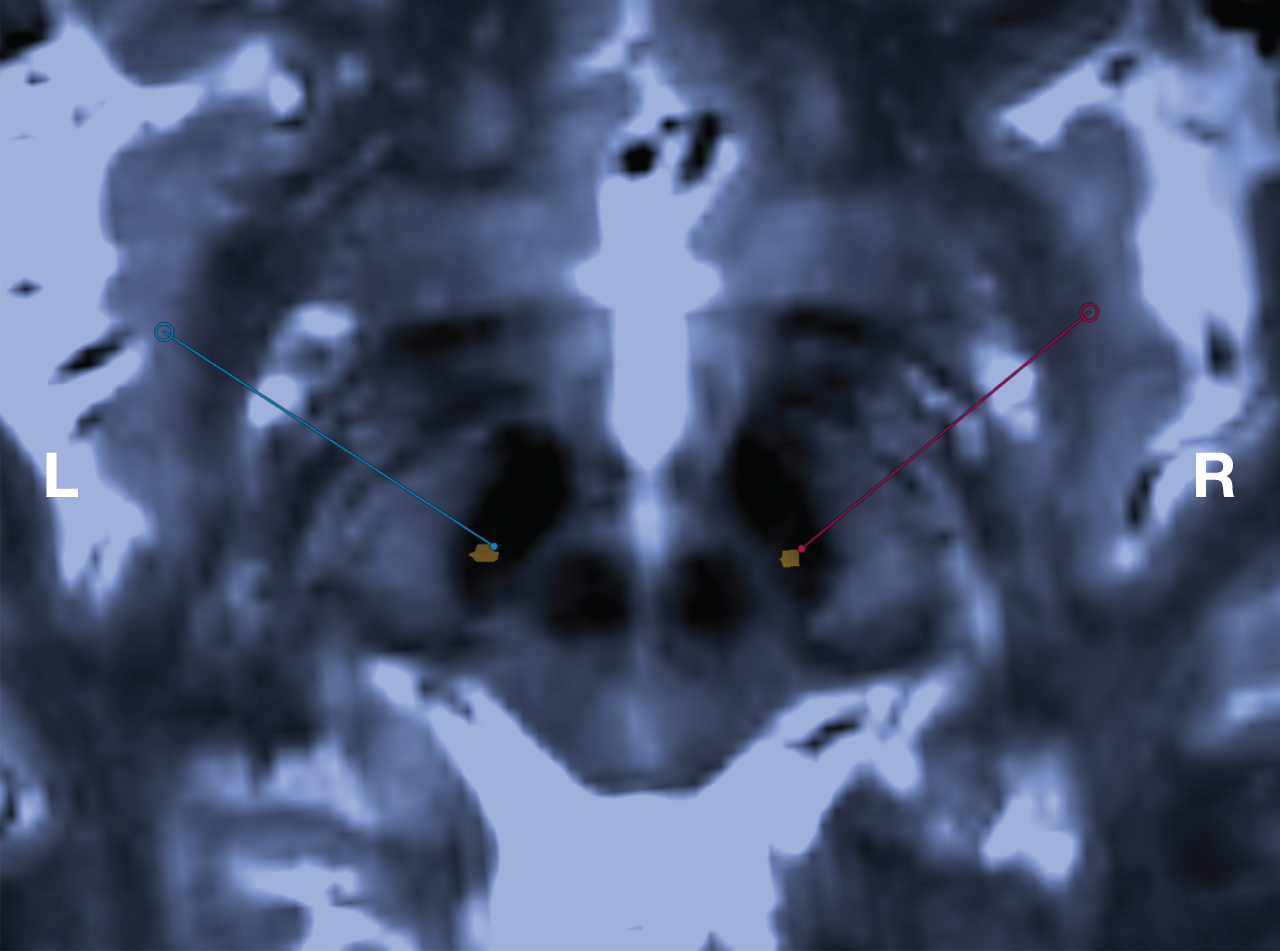
Surgery always commenced on the left side. After administering local anesthetic, a linear incision was made to accommodate a 14 mm frontal burrhole in line with the planned trajectory. A SureTek burrhole device (Boston Scientific, Valencia, CA, USA) was placed to fix the definitive lead later. The dura was opened only locally to facilitate a free pass of the guide tubes used. During insertion of the guide tubes copious irrigation with saline minimized CSF-loss and the associated brain shift. After insertion the burrhole was filled up with fibrin glue. MER was performed in 1 mm steps starting 10 mm above the target and 0.5 mm steps starting 5 mm above the target, until typical nigral activity was seen. The number of microelectrodes used varied between 1 and 4 per side. The recordings were analyzed by a dedicated neurophysiologist. Then, test stimulation was applied with clinical testing by a DBS neurologist. The optimal target was determined by results of MER and intraoperative clinical testing. After determining the optimal target for stimulation, a Vercise Cartesia 8 contact directional DBS-lead (Boston Scientific, Valencia, CA, USA, Model DB-2202) was inserted. The depth of the deepest contact was determined based on the microelectrode recordings. After the insertion of both definitive leads, the patient was placed under general anesthesia and internalization of the extension cables and a right infraclavicular Vercise Gevia rechargeable internal pulse generator (Boston Scientific, Valencia, CA, USA, Model DB-1200-S) was performed. Immediately following surgery, all patients had a stereotactic CT to confirm lead positions. Surgery was only considered to be finished if an acceptable placement of both leads was confirmed. Prophylactic antibiotics (cefazoline 2000 mg) were administered intraoperatively and for three more times in the subsequent 24 h.
Calculation of stereotactic targeting error and postoperative volume of intracranial air
Since depth of the definitive lead was predominantly determined by MER-findings, the error in depth was considered less relevant than the scalar error between the intended target and the trajectory of the definitive lead. The stereotactic targeting error (h) was therefore defined as the shortest (perpendicular) distance between the intended target and the trajectory of the lead. For a more detailed and mathematical description, see Holl et al. [17]. The postoperative air volume was determined by volumetric segmentation, using the Brainlab Elements software, on the immediate postoperative CT-scan.
Determination of the MRI-defined STN
Two observers (RSV and AKS), who were blinded to the MER-results, assessed the T2-weighted MR imaging along the planned lead trajectory from 10 mm above the target to 5 mm past the target, in step sizes equal to those used for MER. Based on imaging alone, they determined whether the planned trajectory at that particular depth was indeed located within the STN or not.
Programming of stimulation
Two weeks after surgery, all patients underwent structured monopolar review of all lead contacts in ring mode and with the patient in the OFF-medication condition. The optimal stimulation contact was defined as the contact with the largest therapeutic window and permanent stimulation was initiated subsequently. Stimulation and medication were further titrated based on clinical response during the follow-up visits.
Follow-up
All patients were assessed 12 months after surgery. They were asked to complete the same battery of questionnaires as preoperatively (including PDQ-39) and an MDS-UPDRS III score was obtained in four conditions (OFF-MED ON-STIM, OFF-MED OFF-STIM, ON-MED OFF-STIM, and ON-MED ON-STIM). The OFF-medication condition was defined as the condition of the patient after withholding antiparkinsonian drugs for 12 h overnight. The ON-medication condition was defined as the condition 1 h after a suprathreshold levodopa dose was administered. The suprathreshold dose was calculated by multiplying the total levodopa equivalent morning dose by 1.2. Clinical assessments in the ON-stimulation condition were done 15 min after switching on the stimulator. Improvement of motor function was defined as the difference between preoperative MDS-UPDRS-III (OFF medication) and 12 months postoperative MDS-UPDRS-III (OFF medication and ON stimulation). Pre and postoperative Levodopa Equivalent Daily Dose (LEDD) were calculated according to the formula described by Tomlinson et al. [18].
Adverse events
Adverse events were recorded for 12 months after surgery. Serious adverse events were defined as any events that lead to permanent disability, death, prolonged hospital stay or new hospital admissions.
Statistical analysis
All data are presented as mean±standard deviation (SD). Ranges [min-max] were provided as well. To quantify interobserver variability, a mean Jaccard’s index of similarity (JI) [19, 20] was used to quantify the overlap between MRI observer 1 and 2 in determining the MRI-defined STN. Furthermore, JI was used to quantify the overlap between the MER-defined STN and the MRI-defined STN for observer 1 and 2 separately. All data were tested for normality using a Shapiro-Wilk test. Differences in mean changes between MDS-UPDRS-III and PDQ-39 scores and LEDD at baseline and at 12 months follow-up were compared using a two-tailed paired sample t-test, since all data was normally distributed. Pearson’s r-coefficient was used to evaluate the correlation between air volume and scalar error. Test results with p-values less than 0.05 were considered statistically significant.
RESULTS
Twenty-nine consecutive patients underwent awake bilateral STN-DBS with MER and intraoperative clinical testing in the period from March 2018 to July 2019. Baseline demographic data and clinical characteristics are shown in Table 1.
Table 1
Baseline demographic data and clinical characteristics
Number of patients | 29 |
Sex –no. (%) | |
Male | 24 (82.8%) |
Female | 5 (17.2%) |
Age at implantation –y [Range] | 62±7.8 [42–77] |
Disease Duration –y [Range] | 9±4.3 [3–21] |
Levodopa Equivalent Daily Dose –mg [Range] | 1354±480 [200–2545.5] |
MDS-UPDRS-III OFF Medication [Range] | 49.5±13.4 [29–90] |
MDS-UPDRS-III ON Medication [Range] | 18.5±10.0 [7–42] |
PDQ-39 [Range] | 46.9±21.5 [12–92] |
MDS-UPDRS, Movement Disorders Society Unified Parkinson’s Disease Rating Scale; PDQ-39, Parkinson’s Disease Questionnaire. The values of age, disease duration, Levodopa Equivalent Daily Dose, MDS-UPDRS, and PDQ-39 are presented as mean±standard deviation of the mean.
Microelectrode recordings
MER was performed in all 29 patients (58 sides) with a total of 170 MER tracks. The mean number of MER tracks used per patient was 2.9±0.6 for the left side and 2.9±0.7 for the right. In 97% of the left-sided trajectories and 100% of the right-sided trajectories, MER identified signals typical for the STN with a mean length of the longest STN trajectory of 5.1±1.0 mm and 4.8±1.0 mm, respectively. In 57 out of 58 (98%) of the sides, the permanent DBS lead was placed in the central track. Only in 1 out of 58 sides (2%), the permanent DBS lead was placed in the lateral track. In one patient there were no MER signals on the left side, due to the interfering effect of dexmedetomidine. Dexmedetomidine was stopped immediately and by the time attention was turned to right side, good quality MER-signals could be acquired.
Motor function, Quality of Life (QoL), and LEDD at 12 months follow-up
A four-condition test at 12 months follow-up was available for all patients. Mean improvement between preoperative MDS-UPDRS-III (OFF medication) and at 12 months follow-up (OFF medication and ON stimulation) was 26.7±16.0 points (54%) (p < 0.001). Completed PDQ-39 questionnaires at baseline and at 12 months follow-up were available for 28 patients. QoL improved with a mean change from baseline to 12 months of 9.0±20.0 points (19%) (p = 0.025). The mean reduction of the LEDD from baseline to 12 months was 794±434 mg/day (59%) (p < 0.001). Results are summarized in Table 2.
Table 2
Clinical Improvement in UPDRS-III, PDQ-39, and LEDD
Baseline Mean±SD [Range] | 12 Months Mean±SD [Range] | Change from baseline Mean±SD (%) | p | |
MDS-UPDRS III | 49.5±13.4 | 22.9±9.2 | 26.7±16.0 | < 0.001 |
OFF medication state | [29–90] | [5–42] | (54%) | |
(OFF meds) | (OFF meds / ON stim) | |||
PDQ-39 | 46.9±21.5 | 39.2±20.8 | 9.0±20.0 | 0.025 |
[12–92] | [10–97] | (19%) | ||
LEDD | 1354±480 | 560±314 | 794±434 | < 0.001 |
mg/day | [200–2545.5] | [0–1275] | (59%) |
MDS-UPDRS, Movement Disorders Society Unified Parkinson’s Disease Rating Scale; PDQ-39, Parkinson’s Disease Questionnaire; LEDD, Levodopa Equivalent Daily Dose.
Stereotactic accuracy and air volume
The mean scalar error of all leads was 0.88±0.45 mm. Mean scalar errors of the left and right leads were 0.92±0.39 mm and 0.82±0.50 mm respectively. This difference was not statistically significant (p = 0.291). The mean volume of air on the immediate postoperative CT was 5.26±9.43 cm3. There was no correlation between air volume and the scalar errors of the left (r = –0.140, p = 0.468) and right (r = 0.154, p = 0.424).
Inter-observer variability in determination of MRI-defined STN, overlap between MER-defined STN and MRI-defined STN and active contacts
The mean JI between observers 1 and 2 in determining the MRI-defined STN along the planned trajectory on both the left and right side for all patients was 0.84±0.09. The mean JI between the MER-defined STN and the MRI-defined STN by observers 1 and 2 were 0.59±0.25 and 0.66±0.25 respectively (Fig. 2). 1 out of 58 (2%) active contacts at 12 months follow-up did not overlap with the MRI-defined STN, whereas 7 out of 58 (12%) did not overlap with the MER-defined STN. The one contact that did not overlap with the MRI-defined STN did not overlap with the MER-defined STN either.
Fig. 2
Evaluation of MER signals and inter-observer variability of the MRI-defined STN along the planned trajectory. Visualization of a one-sided standard evaluation in a particular patient. Depth of the recordings ranges from 7 mm above target (T) to 3 mm below in steps of 0.5 mm starting from 5 mm above target. MER signals of the central track were visualized at each depth and were interpreted by a dedicated neurophysiologist. Characterization of the signals as being STN-specific was indicated in the ‘MER’ column. Two independent observers assessed whether the planned trajectory was located within the MRI-defined STN at every depth. Subsequently, a Jaccard’s Index of Similarity was calculated for MRI Observer 1 and Observer 2. The Jaccard’s Index of Similarity was defined as the number in both sets divided by the number in either set (intersection over union; see formula). The ‘Lead’ column indicates the depth of the contacts of the final lead with the active contact at 12 months follow-up marked in green.
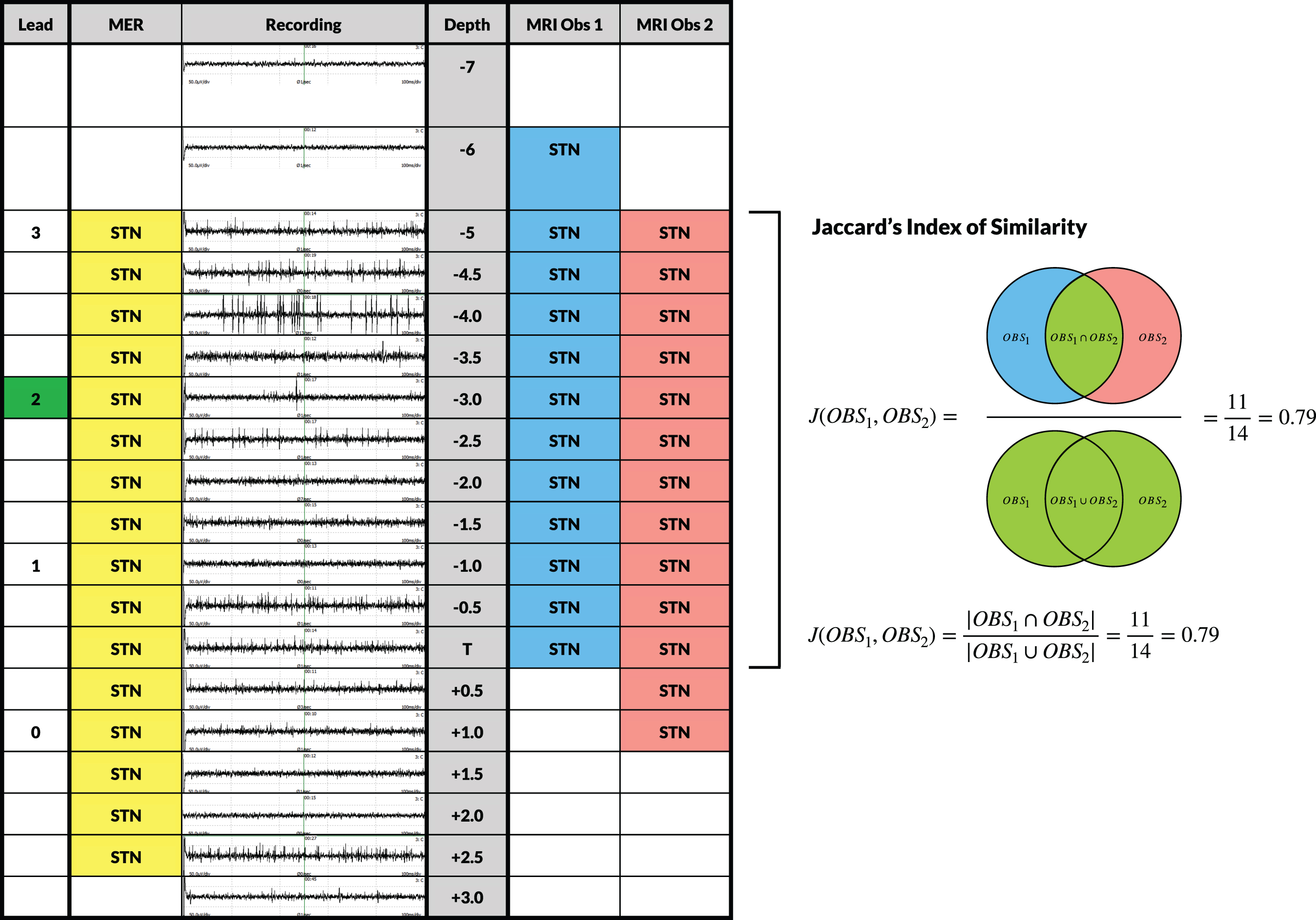
Adverse events
Adverse events were recorded at 12 months follow-up for all patients. One patient was re-operated after a displacement in depth of the right lead was noted on the immediate post-operative CT. The displacement appeared to be caused by an erroneous fixation of the lead in the burrhole device. The retaining clip was not properly locked, allowing for the lead to migrate. In the following patients, the retaining clip was locked meticulously and double checked to prevent more cases of lead migration from happening. There were no infections, intracerebral hemorrhages, epilepsy or hardware failures. All other adverse events are listed in Table 3. In total, there were 19 adverse events in 11 patients.
Table 3
Adverse Events
Mild No. of events | Moderate No. of events | Severe No. of events | Total No. of events (%) | |
Lead malposition requiring revision | – | – | 1 | 1 (3%) |
Traction on extension cable | 1 | 0 | 0 | 1 (3%) |
Dysarthria | 4 | 0 | 0 | 4 (14%) |
Gait disorder | 1 | 1 | 0 | 2 (7%) |
Balance disorder | 2 | 0 | 0 | 2 (7%) |
Dyskinesia | 1 | 0 | 0 | 1 (3%) |
Cognitive decline | 2 | 1 | 0 | 3 (10%) |
Depression | 1 | 0 | 0 | 1 (3%) |
Impulse control disorder | 1 | 0 | 0 | 1 (3%) |
Apathy | 1 | 0 | 0 | 1 (3%) |
Dysphagia | 1 | 0 | 0 | 1 (3%) |
Hypersalivation | 1 | 0 | 0 | 1 (3%) |
DISCUSSION
This study evaluates whether MER with intraoperative clinical testing has an added value in awake bilateral STN-DBS surgery when an MRI-guided and stereotactic CT-verified approach has been used. Baseline patient demographics in the present study were similar to those in other comparable studies in terms of disease duration and severity [1, 21, 22].
Clinical improvement
The mean overall improvement from baseline to 12 months for the MDS-UPDRS-III motor score, PDQ-39 summary index score and the mean reduction in LEDD was consistent with other studies [1, 21, 22].
MER tracks and stereotactic accuracy
The definite position of the permanent DBS leads in this study was solely determined by MER and the results of intraoperative clinical testing. Based on these results, 98% of all leads were placed in the central track. Only one lead (2%) was placed in the lateral track. During this particular procedure, we noted that the stimulation over the central micro-macroelectrode did not work properly, despite good MER signals prior to the stimulation. Impedances were checked and revealed an impedance of 0.0 kΩ of the central micro-macroelectrode. The electrode was replaced with a new micro-macroelectrode, but the impedance remained 0.0 kΩ. Finally, the central MER-cable was switched with one of the unused channels. Subsequently, impedance was checked and revealed a normal impedance. Stimulation and clinical testing were then performed in the usual fashion. Unfortunately, only right after the procedure we found out that there was a short circuit between the central and lateral channel. So, instead of stimulating the central electrode, we were in fact stimulating the lateral electrode. Therefore, the definitive lead was placed in the lateral track based on incorrect assumptions. This means that, even in experienced hands, the technical complexity of MER can induce errors. Several studies have reported the percentage of definitive leads that were placed in the central track [23–28]. These percentages vary from 32% to 73.5% and are evidently lower than the percentage reported in this study. This difference may have several different explanations. The MRI-sequences we used are tailored to accommodate a realistic visualization of the STN and are corrected for MRI distortion to localize the stereotactic frame as accurately as possible. Performing the scans under general anesthesia, for the last 19 patients, evidently reduced movement artefacts and therefore improved the quality of the images. For these 19 patients targeting and localization of the frame was performed on the 3D T2 SPACE only, making CT-MRI or MRI-MRI co-registration unnecessary. Furthermore, care was taken to minimize the amount of CSF loss during the procedure, with a mean air volume of 5.26±9.43 cm3 on the immediate postoperative CT. All the items mentioned above contribute to a reduction of targeting error [29, 30]. Another explanation could be the difference in mindset, regarding targeting, between MER-guided and MRI-guided surgeons. An MER-guided surgeon would rely on the MER and results of clinical testing to determine and confirm the final position of the definitive lead, whereas and MRI-guided surgeon would rely on immediate postoperative imaging to verify the correct position of the definitive lead. Therefore, meticulous determination of the intended target on the preoperative MRI is likely to be more essential for MRI-guided surgeons since correction of the final position based on MER and results of clinical testing is not possible.
Long term clinical outcomes of DBS have shown to be highly dependent on accuracy of lead placement. Rolston et al. reported revision or removal of leads in 15.2–34.0% of the patients. Up to 48.5% of these revisions were likely due to improper targeting despite the use of MER and intraoperative clinical testing [31]. Furthermore, Okun et al. reported that up to 46% of all treatment failures appeared to be related to misplaced leads [32]. The accuracy of lead placement in the present study was similar to those published in other studies using an MRI-guided technique [13, 17]. This accuracy was well within the 2 mm interspacing of the different MER tracks.
MER vs. MRI-defined STN in the planned trajectory and correlation with active contact localization
In defining the STN along the planned trajectory, there appeared to be relatively high overlap between the MRI-defined STN of observer 1 and observer 2. This means that the STN could be defined quite reliably based on MRI alone. The similarity of both observers on the one hand and MER-results on the other are lower, indicating a discrepancy between the electrophysiologically-defined STN and the MRI-defined STN. Most likely, this is because both the STN and the substantia nigra have a similar hypointense aspect on the targeting MRI. Therefore, distinction between these two structures can only be done by indirect anatomical landmarks in an MRI-guided approach. However, this difference did not seem to be clinically relevant, since more active contacts did not overlap with the MER-defined STN than with the MRI-defined STN.
Added value of MER in an MRI-guided approach
Ninety-eight percent of the final leads in this study were placed in the central track, despite MER and intraoperative clinical testing. Accuracy of lead placement in this study was well within the 2 mm interspacing of the different MER tracks. Considering our clinical outcome, medication reduction and incidence of adverse effects at 12 months follow-up are consistent with other studies, this suggests that MER would not be of additional value if a solely MRI-guided and stereotactic CT-verified approach is used. However, this approach requires that the target can be determined reliably on tailored MRI sequences and that the operative technique is accurate enough to get the definitive lead at the intended target. The key factor remains how the optimal target should be determined. MER has shown to increase the operative time by 3 h and more than doubles the cost of STN-DBS-surgery, without improving clinical efficacy [11, 33]. These higher costs are due to personnel, operation time, equipment and anesthesia costs to perform the procedure awake with MER and intraoperative clinical testing. Furthermore, some studies suggest that MER is associated with an increased risk of hemorrhagic complications [10]. Although DBS surgeons who are used to MER might not feel comfortable without having the intraoperative feedback of MER and intraoperative clinical testing, omitting MER may have several advantages. It will likely result in a reduction of hemorrhagic complications and a significant reduction in operation time and costs, especially since omitting MER and intraoperative clinical testing allows for an asleep MRI-guided and CT or MRI-verified approach. Moreover, patients do not have to experience the burden of coming off their anti-parkinsonian medication 12–24 h prior to surgery and undergoing awake surgery. The latter consideration should, however, not be generalized, since some patients may prefer to undergo an awake procedure [34]. Based on the results of this study and the experience of our team, we changed our practice to an asleep MRI-guided and stereotactic CT-verified approach, which created the opportunity to operate two instead of one patients a day.
Limitations of this study
Although this study evaluates the added value of MER in MRI-guided STN-DBS in a thorough manner, several limitations should be considered. This study is limited by its relatively small study population and the retrospective study design. Furthermore, the results of our study are dependent on the quality of the MR imaging, experience of the team with an MRI-guided approach and the accuracy of the operative technique. Therefore, generalizing the outcome of this study should be done with caution.
CONCLUSION
Since our team has experience in both awake MER-guided and asleep MRI-guided STN-DBS, we got the unique opportunity to evaluate the value of MER in MRI-guided STN-DBS. In this study we demonstrated that MER and intraoperative clinical testing are not of additional value in determination of the final lead position, if an adequate MRI-guided and stereotactic CT-verified technique is used. These results changed our daily clinical practice to an asleep MRI-guided and stereotactic CT-verified approach. However, further research should be directed towards a properly designed comparative trial that evaluates the added value of MER and intraoperative clinical testing and towards giving more insight into optimal and patient-specific target localization.
CONFLICT OF INTEREST
RSV acts as an independent consultant for Boston Scientific. The other authors have nothing to disclose.
SUPPLEMENTARY MATERIAL
[1] The supplementary material is available in the electronic version of this article: https://dx.doi.org/10.3233/JPD-223149.
REFERENCES
[1] | Deuschl G , Schade-Brittinger C , Krack P , Volkmann J , Schafer H , Botzel K , Daniels C , Deutschlander A , Dillmann U , Eisner W , Gruber D , Hamel W , Herzog J , Hilker R , Klebe S , Kloss M , Koy J , Krause M , Kupsch A , Lorenz D , Lorenzl S , Mehdorn HM , Moringlane JR , Oertel W , Pinsker MO , Reichmann H , Reuss A , Schneider GH , Schnitzler A , Steude U , Sturm V , Timmermann L , Tronnier V , Trottenberg T , Wojtecki L , Wolf E , Poewe W , Voges J , German Parkinson Study Group, Neurostimulation Section ((2006) ) A randomized trial of deep-brain stimulation for Parkinson’s disease. N Engl J Med 355: , 896–908. |
[2] | Schuepbach WM , Rau J , Knudsen K , Volkmann J , Krack P , Timmermann L , Halbig TD , Hesekamp H , Navarro SM , Meier N , Falk D , Mehdorn M , Paschen S , Maarouf M , Barbe MT , Fink GR , Kupsch A , Gruber D , Schneider GH , Seigneuret E , Kistner A , Chaynes P , Ory-Magne F , Brefel Courbon C , Vesper J , Schnitzler A , Wojtecki L , Houeto JL , Bataille B , Maltete D , Damier P , Raoul S , Sixel-Doering F , Hellwig D , Gharabaghi A , Kruger R , Pinsker MO , Amtage F , Regis JM , Witjas T , Thobois S , Mertens P , Kloss M , Hartmann A , Oertel WH , Post B , Speelman H , Agid Y , Schade-Brittinger C , Deuschl G , EARLYSTIM Study Group ((2013) ) Neurostimulation for Parkinson’s disease with early motor complications. N Engl J Med 368: , 610–622. |
[3] | Abosch A , Timmermann L , Bartley S , Rietkerk HG , Whiting D , Connolly PJ , Lanctin D , Hariz MI ((2013) ) An international survey of deep brain stimulation procedural steps. Stereotact Funct Neurosurg 91: , 1–11. |
[4] | Benabid AL , Krack PP , Benazzouz A , Limousin P , Koudsie A , Pollak P ((2000) ) Deep brain stimulation of the subthalamic nucleus for Parkinson’s disease: Methodologic aspects and clinical criteria. Neurology 55: , S40–44. |
[5] | Ashkan K , Blomstedt P , Zrinzo L , Tisch S , Yousry T , Limousin-Dowsey P , Hariz MI ((2007) ) Variability of the subthalamic nucleus: The case for direct MRI guided targeting. Br J Neurosurg 21: , 197–200. |
[6] | Counelis GJ , Simuni T , Forman MS , Jaggi JL , Trojanowski JQ , Baltuch GH ((2003) ) Bilateral subthalamic nucleus deep brain stimulation for advanced PD: Correlation of intraoperative MER and postoperative MRI with neuropathological findings. Mov Disord 18: , 1062–1065. |
[7] | Hariz M , Blomstedt P , Limousin P ((2004) ) The myth of microelectrode recording in ensuring a precise location of the DBS electrode within the sensorimotor part of the subthalamic nucleus. Mov Disord 19: , 863–864. |
[8] | McClelland S , 3rd, Vonsattel JP , Garcia RE , Amaya MD , Winfield LM , Pullman SL , Yu Q , Fahn S , Ford B , Goodman RR ((2007) ) Relationship of clinical efficacy to postmortem-determined anatomic subthalamic stimulation in Parkinson syndrome. Clin Neuropathol 26: , 267–275. |
[9] | Gorgulho A , De Salles AA , Frighetto L , Behnke E ((2005) ) Incidence of hemorrhage associated with electrophysiological studies performed using macroelectrodes and microelectrodes in functional neurosurgery. J Neurosurg 102: , 888–896. |
[10] | Zrinzo L , Foltynie T , Limousin P , Hariz MI ((2012) ) Reducing hemorrhagic complications in functional neurosurgery: A large case series and systematic literature review. J Neurosurg 116: , 84–94. |
[11] | McClelland S , 3rd ((2011) ) A cost analysis of intraoperative microelectrode recording during subthalamic stimulation for Parkinson’s disease. Mov Disord 26: , 1422–1427. |
[12] | Nakajima T , Zrinzo L , Foltynie T , Olmos IA , Taylor C , Hariz MI , Limousin P ((2011) ) MRI-guided subthalamic nucleus deep brain stimulation without microelectrode recording: Can we dispense with surgery under local anaesthesia? Stereotact Funct Neurosurg 89: , 318–325. |
[13] | Chen T , Mirzadeh Z , Chapple KM , Lambert M , Shill HA , Moguel-Cobos G , Troster AI , Dhall R , Ponce FA ((2018) ) Clinical outcomes following awake and asleep deep brain stimulation for Parkinson disease. J Neurosurg 130: , 109–120. |
[14] | Wodarg F , Herzog J , Reese R , Falk D , Pinsker MO , Steigerwald F , Jansen O , Deuschl G , Mehdorn HM , Volkmann J ((2012) ) Stimulation site within the MRI-defined STN predicts postoperative motor outcome. Mov Disord 27: , 874–879. |
[15] | Patel NK , Khan S , Gill SS ((2008) ) Comparison of atlas- and magnetic-resonance-imaging-based stereotactic targeting of the subthalamic nucleus in the surgical treatment of Parkinson’s disease. Stereotact Funct Neurosurg 86: , 153–161. |
[16] | Bejjani BP , Dormont D , Pidoux B , Yelnik J , Damier P , Arnulf I , Bonnet AM , Marsault C , Agid Y , Philippon J , Cornu P ((2000) ) Bilateral subthalamic stimulation for Parkinson’s disease by using three-dimensional stereotactic magnetic resonance imaging and electrophysiological guidance. J Neurosurg 92: , 615–625. |
[17] | Holl EM , Petersen EA , Foltynie T , Martinez-Torres I , Limousin P , Hariz MI , Zrinzo L ((2010) ) Improving targeting in image-guided frame-based deep brain stimulation. Neurosurgery 67: , 437–447. |
[18] | Tomlinson CL , Stowe R , Patel S , Rick C , Gray R , Clarke CE ((2010) ) Systematic review of levodopa dose equivalency reporting in Parkinson’s disease. Mov Disord 25: , 2649–2653. |
[19] | Real R , Vargas JM ((1996) ) The probabilistic basis of Jaccard’s Index of Similarity. Syst Biol 45: , 380–385. |
[20] | Jaccard P ((1908) ) Nouvelles recherches sur la distribution florale. Bull Soc Vaud Sci Nat 44: , 223–270. |
[21] | Weaver FM , Follett K , Stern M , Hur K , Harris C , Marks WJ Jr., Rothlind J , Sagher O , Reda D , Moy CS , Pahwa R , Burchiel K , Hogarth P , Lai EC , Duda JE , Holloway K , Samii A , Horn S , Bronstein J , Stoner G , Heemskerk J , Huang GD , CSP 468 Study Group ((2009) ) Bilateral deep brain stimulation vs best medical therapy for patients with advanced Parkinson disease: A randomized controlled trial. JAMA 301: , 63–73. |
[22] | Odekerken VJ , van Laar T , Staal MJ , Mosch A , Hoffmann CF , Nijssen PC , Beute GN , van Vugt JP , Lenders MW , Contarino MF , Mink MS , Bour LJ , van den Munckhof P , Schmand BA , de Haan RJ , Schuurman PR , de Bie RM ((2013) ) Subthalamic nucleus versus globus pallidus bilateral deep brain stimulation for advanced Parkinson’s disease (NSTAPS study): A randomised controlled trial. Lancet Neurol 12: , 37–44. |
[23] | Bour LJ , Contarino MF , Foncke EM , de Bie RM , van den Munckhof P , Speelman JD , Schuurman PR ((2010) ) Long-term experience with intraoperative microrecording during DBS neurosurgery in STN and GPi. Acta Neurochir (Wien) 152: , 2069–2077. |
[24] | Temel Y , Wilbrink P , Duits A , Boon P , Tromp S , Ackermans L , van Kranen-Mastenbroek V , Weber W , Visser-Vandewalle V ((2007) ) Single electrode and multiple electrode guided electrical stimulation of the subthalamic nucleus in advanced Parkinson’s disease. Neurosurgery 61: , 346–355; discussion; 355-347. |
[25] | Hamel W , Fietzek U , Morsnowski A , Schrader B , Herzog J , Weinert D , Pfister G , Muller D , Volkmann J , Deuschl G , Mehdorn HM ((2003) ) Deep brain stimulation of the subthalamic nucleus in Parkinson’s disease: Evaluation of active electrode contacts. J Neurol Neurosurg Psychiatry 74: , 1036–1046. |
[26] | Amirnovin R , Williams ZM , Cosgrove GR , Eskandar EN ((2006) ) Experience with microelectrode guided subthalamic nucleus deep brain stimulation. Neurosurgery 58: , ONS96–102; discussion ONS196-102. |
[27] | Rola R , Tutaj M , Koziara H , Koziorowski D , Brodacki B , Karliński M , Tykocki T , Krolicki B , Mandat T ((2016) ) Optimizing final electrode placement Based on intraoperative neurophysiological evaluation during subthalamic deep brain stimulation for Parkinson’s disease. J Neurol Neurophysiol 7: , 4. |
[28] | Frequin HL , Bot M , Dilai J , Scholten MN , Postma M , Bour LJ , Contarino MF , de Bie RMA , Schuurman PR , van den Munckhof P ((2020) ) Relative contribution of magnetic resonance imaging, microelectrode recordings, and awake test stimulation in final lead placement during deep brain stimulation surgery of the subthalamic nucleus in Parkinson’s disease. Stereotact Funct Neurosurg 98: , 118–128. |
[29] | Azmi H , Machado A , Deogaonkar M , Rezai A ((2011) ) Intracranial air correlates with preoperative cerebral atrophy and stereotactic error during bilateral STN DBS surgery for Parkinson’s disease. Stereotact Funct Neurosurg 89: , 246–252. |
[30] | Geevarghese R , O’Gorman Tuura R , Lumsden DE , Samuel M , Ashkan K ((2016) ) Registration accuracy of CT/MRI fusion for localisation of deep brain stimulation electrode position: An imaging study and systematic review. Stereotact Funct Neurosurg 94: , 159–163. |
[31] | Rolston JD , Englot DJ , Starr PA , Larson PS ((2016) ) An unexpectedly high rate of revisions and removals in deep brain stimulation surgery: Analysis of multiple databases. Parkinsonism Relat Disord 33: , 72–77. |
[32] | Okun MS , Tagliati M , Pourfar M , Fernandez HH , Rodriguez RL , Alterman RL , Foote KD ((2005) ) Management of referred deep brain stimulation failures: A retrospective analysis from 2 movement disorders centers. Arch Neurol 62: , 1250–1255. |
[33] | Maiti TK , Konar S , Bir S , Kalakoti P , Nanda A ((2016) ) Intra-operative micro-electrode recording in functional neurosurgery: Past, present, future. J Clin Neurosci 32: , 166–172. |
[34] | LaHue SC , Ostrem JL , Galifianakis NB , San Luciano M , Ziman N , Wang S , Racine CA , Starr PA , Larson PS , Katz M ((2017) ) Parkinson’s disease patient preference and experience with various methods of DBS lead placement. Parkinsonism Relat Disord 41: , 25–30. |