Growth Factor Therapy for Parkinson’s Disease: Alternative Delivery Systems
Abstract
Despite decades of research and billions in global investment, there remains no preventative or curative treatment for any neurodegenerative condition, including Parkinson’s disease (PD). Arguably, the most promising approach for neuroprotection and neurorestoration in PD is using growth factors which can promote the growth and survival of degenerating neurons. However, although neurotrophin therapy may seem like the ideal approach for neurodegenerative disease, the use of growth factors as drugs presents major challenges because of their protein structure which creates serious hurdles related to accessing the brain and specific targeting of affected brain regions. To address these challenges, several different delivery systems have been developed, and two major approaches—direct infusion of the growth factor protein into the target brain region and in vivo gene therapy—have progressed to clinical trials in patients with PD. In addition to these clinically evaluated approaches, a range of other delivery methods are in various degrees of development, each with their own unique potential. This review will give a short overview of some of these alternative delivery systems, with a focus on ex vivo gene therapy and biomaterial-aided protein and gene delivery, and will provide some perspectives on their potential for clinical development and translation.
GROWTH FACTOR THERAPY FOR PARKINSON’S DISEASE: EXTRAORDINARY POTENTIAL, HUGE CHALLENGES
It stands to reason that, if one of the central pathological features of Parkinson’s disease (PD) is the dysfunction, degeneration and death of dopaminergic neurons in the brain [1], then a therapeutic intervention aimed at preventing, slowing or reversing this neuronal pathology would have a transformative impact on current symptomatic therapeutic offerings to patients [2]. One neuroprotective and neurorestorative approach that has extraordinary potential is the use of neurotrophic growth factors, such as glial cell line-derived neurotrophic factor (GDNF), which are inherently capable of promoting the growth and survival of dopaminergic neurons [3]. However, because growth factors are proteins, this presents huge challenges in traversing the blood brain barrier and specifically targeting the diseased brain region(s) [4]. Thus, unless the challenges of growth factor delivery to the brain are surmounted, this promising approach will never reach its full potential for the treatment of PD.
Of the different delivery systems that are under investigation, two frontrunners have emerged, and both have already progressed through preclinical development and evaluation onto clinical testing in patients with PD [4]. These are infusion of the growth factor protein directly into the target brain region via implanted cannulae [5–12], and injection of viral vectors encoding the growth factor gene into the target brain region [13–15]. Although the clinical trials of these approaches have met with mixed success—and this has been the subject of comprehensive analysis elsewhere [4]—the issue of delivery remains one of the major hurdles to clinical translation of growth factor therapy for PD [3]. This article will provide a short overview of some of the other delivery methods that are being developed in this context and, in particular, we will focus on the potential of two broad areas that are making exciting strides forward, namely ex vivo gene delivery, and biomaterial-aided protein or gene delivery (Fig. 1).
Fig. 1
Potential delivery systems for neurotrophic growth factor delivery in PD. To assess the therapeutic potential of neurotrophic factor therapy, direct infusion of the growth factor protein into the target brain region and in vivo gene therapy have progressed to clinical trials in patients with PD. However, one of the major hurdles to the clinical translation of growth factor therapeutics for PD remains issues related to the delivery of these protein drugs. To address this, other delivery systems, including ex vivo gene therapy and biomaterial-aided protein and gene delivery are in preclinical development.
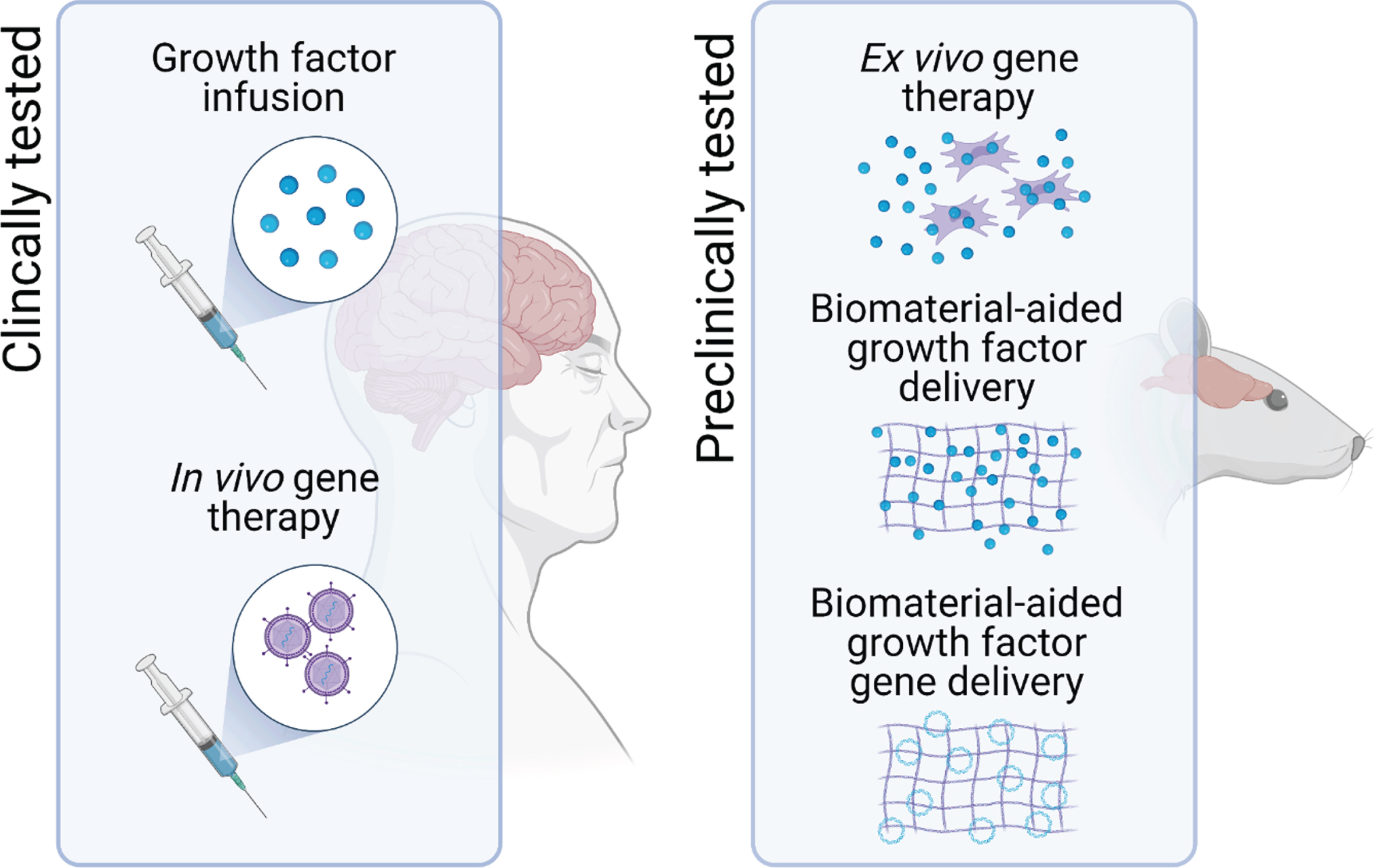
ALTERNATIVE DELIVERY SYSTEMS: EX VIVO GENE DELIVERY
Ex vivo gene therapy is the process by which cells are genetically engineered to produce therapeutic factors, such as neurotrophic growth factors, prior to their use as a therapy for patients [16]. In this approach, the cells themselves are then used as the delivery vehicles for the growth factors. Proponents of this approach suggest that its main advantage over the in vivo gene therapy approaches that have already reached clinical trials for PD are that, because the genetic engineering occurs ex vivo, the gene vector is not injected directly into the patient’s brain and the engineered cells can be subjected to rigorous preclinical evaluation prior to administration to the patient. Notwithstanding this suggestion, it must be emphasised that the safety of in vivo gene therapy for PD has been shown in several clinical trials (reviewed in [17]).
As with all emerging therapeutic approaches, there are a plethora of considerations that need to be explored before ex vivo growth factor gene delivery can be tested in patients with PD [16]. These are summarised in Table 1 and include the choice of gene vector, the nature of the cell donor, the type of cell and the route of administration.
Table 1
The key considerations facing ex vivo growth factor delivery for PD
Key considerations | Questions to explore | |
Choice of Vector | Type | –Viral vs. non-viral vectors |
Safety | –Risk of insertional mutagenesis | |
–Regulatability/controllability of gene expression | ||
Efficacy | –Engineering dividing vs. non-dividing cells | |
–Stability of transduction/transfection | ||
–Genetic packaging capacity | ||
Nature of Cell Donor | Donors | –Allogeneic vs. autologous |
–Adult vs. embryonic or fetal | ||
–Ethical implications | ||
Cell Type | Cells | –Availability |
–Expandability | ||
–Transducability/transfectability | ||
Safety | –Tumorigenicity | |
–Need for immune suppression | ||
Efficacy | –Ability to survive in the brain | |
–Ability to provide sustained delivery | ||
Route of Administration | Systemic | –Ability to access brain |
–Potential for off-target effects | ||
Intracerebral (to ventricles) | –Ability to diffuse to target site | |
–Potential for off-target effects | ||
–Potential for damage to brain parenchyma | ||
Intracerebral (to target site) | –Potential for damage to brain parenchyma |
References: For more detail on the considerations facing the development, evaluation and clinical translation of ex vivo gene therapy for PD, please see this excellent review by Svendsen and colleagues [16].
Vectors
A range of gene vectors can be used for ex vivo gene therapy including viral vectors, plasmid vectors, and more recently developed gene editing technologies, and each of these has its associated advantages and drawbacks. Regarding potential for clinical translation, the vector will need to be safe, particularly in terms of the risk of insertional mutagenesis/oncogenesis as well as offering control over gene expression in instances where the patient might experience unwanted consequences of excessive/uncontrolled growth factor production. The selected vector will also need to be efficacious in terms of its ability to engineer the selected cell type and to drive long term expression of the growth factor gene.
Donors
The cell donor is also an important consideration, particularly in terms of using cells from the patients themselves (autologous) versus using cells from different donors (allogenic), but also in terms of the ethical acceptability of the cell source with adult cells being more ethically acceptable than those derived from embryonic or fetal sources.
Cells
The type of cell used for ex vivo gene therapy is of critical importance for clinical translation. The cells should be readily available, and amenable to in vitro expansion and genetic engineering. From a safety perspective, there should be no risk of tumorigenicity from the implanted cells, and ideally from a safety perspective, they should be autologous so that immunosuppression is not required. With regard to efficacy, the cells used should be able to survive for many years in the brain in order to provide sustained growth factor delivery to the site of degeneration.
Route
The route of administration for the cells is also an important consideration. Systemic delivery may seem ideal but this has consequences for reaching the target brain region and has increased potential for off-target effects. The obvious alternative is intracerebral, either into the ventricles (less damaging, but more remote) or into the target site (more direct, but potentially more damaging).
Taking these considerations into account, several innovative approaches to ex vivo growth factor gene delivery are emerging. Studies by Clive Svendsen and colleagues have used human neural progenitor cells for successful ex vivo delivery of GDNF in rodent and nonhuman primate models of PD [18, 19], and a recent study by the same group [20] used a doxycycline-regulated plasmid vector to drive controllable GDNF expression in induced pluripotent stem cell (iPSC)-derived neural progenitors in the mouse brain. The vector construct was capable of stable integration into the progenitor genome and could regulate GDNF expression in the brain over multiple doxycycline cycles. Moreover, the use of iPSCs as the source of cells opens the possibility of an ethically-sound, autologous approach to ex vivo growth factor gene therapy, and their differentiation into neural progenitors improves both their safety in terms of tumorigenesis as well as their efficacy in terms of long-term ability to survive in the brain. Using iPSC-derived neural progenitors does require direct transplantation into the target site but this seems like a small cost to pay for all of the potential benefits of this innovative approach.
Another intriguing approach that may allow for systemic delivery of the engineered cells harnesses the inherent ability of circulating macrophages to home to, and gain entry to, sites of damage, neuroinflammation and degeneration in the brain [21]. Several studies have now shown that macrophages or hematopoietic stem cells (HSCs) with a macrophage specific promotor can be engineered to overexpress growth factors (including GDNF and neurturin) [22–28]. When administered intravascularly or intrathecally, these cells accumulate at sites of degeneration in the brain, produce GDNF and ameliorate parkinsonism in several rodent models of PD.
ALTERNATIVE DELIVERY SYSTEMS: BIOMATERIAL-AIDED GROWTH FACTOR DELIVERY
Another alternative delivery system for growth factor delivery in PD that has seen exponential research and development over the past 15 years is in the use of biomaterial-based systems to aid protein or gene delivery [29–31]. Biomaterials are materials that are engineered to interact with biological systems primarily for therapeutic purposes. Their potential application to PD therapeutics is vast and has been reviewed extensively elsewhere [31]. For growth factor delivery specifically, biomaterials have potential to aid in the delivery and maintenance of growth factor proteins or genes in the brain.
Biomaterial-aided growth factor protein delivery
One approach that has been the subject of intense research is using polymeric biomaterials as intracerebrally-injectable microcarriers to protect encapsulated growth factor proteins and release them slowly over time within the diseased region of the brain [32]. Although numerous studies have shown that intracerebral injection of microencapsulated growth factors including GDNF [33–39] and vascular endothelial growth factor (VEGF) [34, 35, 40] can improve parkinsonism in both rodent [34–42] and primate [33] models of PD, the main limitation of this approach is that the growth factor release in the brain is rapid and not sustained, a major issue if biomaterial-aided growth factor therapy is to provide meaningful benefit in the decades-long pathogenesis of PD in patients. One potential solution to this could be the use of highly charged microscale depot systems that provide strong electrostatic attraction between the therapeutic protein(s) and the microcarrier [43]. Not only does this result in slower release of the therapeutic protein, but it also has the potential to be refillable or reloadable, although this too is limited as each refill would require intracerebral injection of the growth factor.
Whilst intracerebral infusion of growth factors represents the most direct way to bypass the blood-brain barrier, intranasal delivery represents an exciting alternative delivery route to the midbrain, via the olfactory system and trigeminal nerve [44–46]. This is another delivery method where biomaterial systems can aid with delivery of the growth factor to the brain. In particular, using cationic lipid-based nanoparticles can protect the encapsulated growth factor(s) and increase electrostatic interactions at the olfactory epithelium to increase uptake to the brain [44–46]. Although several studies have shown that intranasal administration of the GDNF protein itself can ameliorate parkinsonism in animal models [47, 48], encapsulation of the protein within lipid nanoparticles [48–50], especially those functionalized with the cell-penetrating peptide TAT [50], significantly improves the outcome. Although ease of administration is a clear plus with intranasal delivery, this route of administration has been shown to increase GDNF expression throughout the whole brain [51], a potential limitation to clinical translation in terms of off-target effects.
Biomaterial-aided growth factor gene delivery
In general, despite some potential advantages in safety and production, non-viral gene vectors have lagged behind their viral counterparts, and non-viral gene delivery to the brain, whether via intracerebral or systemic routes, has proven particularly challenging [52]. Systemic administration would represent a significant clinical advantage over intracerebral administration required for the in vivo gene therapy approach used for viruses. Although there has been some success in using Trojan horse liposomes [53] for systemic GDNF gene delivery in PD models [54, 55], in general, brain uptake is typically low and without regional targeting [29].
An exciting recent advancement which could serve to overcome these drawbacks, has been to harness the potential of focused ultrasound to disrupt the blood brain barrier thereby improving brain access and targeting of circulating therapeutics [56–58]. Indeed, liposomes [59–63] and other biomaterial particles [64–66] have been shown to protect and improve brain penetrability of both growth factor protein [60, 63, 65] and gene therapies [59, 61, 62, 64, 66] after focused ultrasound in animal models of PD. The first clinical trial of focused ultrasound for blood brain barrier opening in PD is currently running (NCT03608553) and time will tell if this approach is both safe and efficacious.
CONCLUSION
Despite many decades of intensive research, there remains no disease-modifying neuroprotective or neurorestorative therapy for PD. Many consider that the most promise lies with growth factor therapy, but significant challenges have to be surmounted in order to translate these protein drugs to an effective and safe therapeutic option for patients. Direct infusion of the growth factor proteins to, and in vivo gene therapy within, the affected brain region(s) are still the only approaches that have reached clinical trial, and they remain the most promising solution. However, recent developments in ex vivo gene therapy (particularly in engineering controllable gene vectors and in harnessing patient-derived, neurally reprogrammable adult cells) and in biomaterial-aided protein and gene delivery (especially related to intranasal delivery and delivery through the ultrasound disrupted blood brain barrier) bring alternative growth factor delivery systems ever closer to clinical trial.
ACKNOWLEDGMENTS
Our research in this field is funded by the Michael J Foundation for Parkinson’s Research (Grant Number: 17244), Science Foundation Ireland (Grant Number: 19/FFP/6554) and the European Union Horizon 2020 Programme (H2020-MSCA-ITN-2015) under the Marie Sklodowska-Curie Innovative Training Networks (Grant Number: 676408).
CONFLICT OF INTEREST
The authors have no conflict of interest to report.
REFERENCES
[1] | Simon DK , Tanner CM , Brundin P ((2020) ) Parkinson disease epidemiology, pathology, genetics, and pathophysiology. Clin Geriatr Med 36: , 1–12. |
[2] | Stoker TB , Barker RA ((2020) ) Recent developments in the treatment of Parkinson’s disease. F1000Res 9: , F1000 Faculty Rev-862. |
[3] | Sidorova YA , Saarma M ((2020) ) Can growth factors cure Parkinson’s disease? Trends Pharmacol Sci 41: , 909–922. |
[4] | Barker RA , Bjorklund A , Gash DM , Whone A , Van Laar A , Kordower JH , Bankiewicz K , Kieburtz K , Saarma M , Booms S , Huttunen HJ , Kells AP , Fiandaca MS , Stoessl AJ , Eidelberg D , Federoff H , Voutilainen MH , Dexter DT , Eberling J , Brundin P , Isaacs L , Mursaleen L , Bresolin E , Carroll C , Coles A , Fiske B , Matthews H , Lungu C , Wyse RK , Stott S , Lang AE ((2020) ) GDNF and Parkinson’s disease: Where next? A summary from a recent workshop. J Parkinsons Dis 10: , 875–891. |
[5] | Whone A , Luz M , Boca M , Woolley M , Mooney L , Dharia S , Broadfoot J , Cronin D , Schroers C , Barua NU , Longpre L , Barclay CL , Boiko C , Johnson GA , Fibiger HC , Harrison R , Lewis O , Pritchard G , Howell M , Irving C , Johnson D , Kinch S , Marshall C , Lawrence AD , Blinder S , Sossi V , Stoessl AJ , Skinner P , Mohr E , Gill SS ((2019) ) Randomized trial of intermittent intraputamenal glial cell line-derived neurotrophic factor in Parkinson’s disease. Brain 142: , 512–525. |
[6] | Kordower JH , Palfi S , Chen EY , Ma SY , Sendera T , Cochran EJ , Cochran EJ , Mufson EJ , Penn R , Goetz CG , Comella CD ((1999) ) Clinicopathological findings following intraventricular glial-derived neurotrophic factor treatment in a patient with Parkinson’s disease. Ann Neurol 46: , 419–424. |
[7] | Gill SS , Patel NK , Hotton GR , O’Sullivan K , McCarter R , Bunnage M , Brooks DJ , Svendsen CN , Heywood P ((2003) ) Direct brain infusion of glial cell line-derived neurotrophic factor in Parkinson disease. Nat Med 9: , 589–595. |
[8] | Slevin JT , Gerhardt GA , Smith CD , Gash DM , Kryscio R , Young B ((2005) ) Improvement of bilateral motor functions in patients with Parkinson disease through the unilateral intraputaminal infusion of glial cell line-derived neurotrophic factor. J Neurosurg 102: , 216–222. |
[9] | Love S , Plaha P , Patel NK , Hotton GR , Brooks DJ , Gill SS ((2005) ) Glial cell line-derived neurotrophic factor induces neuronal sprouting in human brain. Nat Med 11: , 703–704. |
[10] | Patel NK , Pavese N , Javed S , Hotton GR , Brooks DJ , Gill SS ((2013) ) Benefits of putaminal GDNF infusion in Parkinson disease are maintained after GDNF cessation. Neurology 81: , 1176–1178. |
[11] | Lang AE , Gill S , Patel NK , Lozano A , Nutt JG , Penn R , Brooks DJ , Hotton G , Moro E , Heywood P , Brodsky MA , Burchiel K , Kelly P , Dalvi A , Scott B , Stacy M , Turner D , Wooten VG , Elias WJ , Laws ER , Dhawan V , Stoessl AJ , Matcham J , Coffey RJ , Traub M ((2006) ) Randomized controlled trial of intraputamenal glial cell line-derived neurotrophic factor infusion in Parkinson disease. Ann Neurol 59: , 459–466. |
[12] | Nutt JG , Burchiel KJ , Comella CL , Jankovic J , Lang AE , Laws ER Jr. , Lozano AM , Penn RD , Simpson RK Jr. , Stacy M , Wooten GF , ICV GDNF Study Group. Implanted intracerebroventricular. Glial cell line-derived neurotrophic factor ((2003) ) Randomized, double-blind trial of glial cell line-derived neurotrophic factor (GDNF) in PD. Neurology 60: , 69–73. |
[13] | Marks WJ Jr. , Ostrem JL , Verhagen L , Starr PA , Larson PS , Bakay RA , Taylor R , Cahn-Weiner DA , Stoessl AJ , Olanow CW , Bartus RT ((2008) ) Safety and tolerability of intraputaminal delivery of CERE-120 (adeno-associated virus serotype 2-neurturin) to patients with idiopathic Parkinson’s disease: An open-label, phase I trial. Lancet Neurol 7: , 400–408. |
[14] | Bartus RT , Baumann TL , Siffert J , Herzog CD , Alterman R , Boulis N , Turner DA , Stacy M , Lang AE , Lozano AM , Olanow CW ((2013) ) Safety/feasibility of targeting the substantia nigra with AAV2-neurturin in Parkinson patients. Neurology 80: , 1698–1701. |
[15] | Marks WJ Jr. , Baumann TL , Bartus RT ((2016) ) Long-term safety of patients with Parkinson’s disease receiving rAAV2-neurturin (CERE-120) gene transfer. Hum Gene Ther 27: , 522–527. |
[16] | Gowing G , Svendsen S , Svendsen CN ((2017) ) Ex vivo gene therapy for the treatment of neurological disorders. Prog Brain Res 230: , 99–132. |
[17] | Hitti FL , Yang AI , Gonzalez-Alegre P , Baltuch GH ((2019) ) Human gene therapy approaches for the treatment of Parkinson’s disease: An overview of current and completed clinical trials. Parkinsonism Relat Disord 66: , 16–24. |
[18] | Emborg ME , Ebert AD , Moirano J , Peng S , Suzuki M , Capowski E , Joers V , Roitberg BZ , Aebischer P , Svendsen CN ((2008) ) GDNF-secreting human neural progenitor cells increase tyrosine hydroxylase and VMAT2 expression in MPTP-treated cynomolgus monkeys. Cell Transplant 17: , 383–395. |
[19] | Behrstock S , Ebert A , McHugh J , Vosberg S , Moore J , Schneider B , Capowski E , Hei D , Kordower J , Aebischer P , Svendsen CN ((2006) ) Human neural progenitors deliver glial cell line-derived neurotrophic factor to parkinsonian rodents and aged primates. Gene Ther 13: , 379–388. |
[20] | Akhtar AA , Gowing G , Kobritz N , Savinoff SE , Garcia L , Saxon D , Cho N , Kim G , Tom CM , Park H , Lawless G , Shelley BC , Mattis VB , Breunig JJ , Svendsen CN ((2018) ) Inducible expression of GDNF in transplanted iPSC-derived neural progenitor cells. Stem Cell Rep 10: , 1696–1704. |
[21] | Corraliza I ((2014) ) Recruiting specialized macrophages across the borders to restore brain functions. Front Cell Neurosci 8: , 262. |
[22] | Chen C , Guderyon MJ , Li Y , Ge G , Bhattacharjee A , Ballard C , He Z , Masliah E , Clark RA , O’Connor JC , Li S ((2020) ) Non-toxic HSC transplantation-based macrophage/microglia-mediated GDNF delivery for Parkinson’s disease. Mol Ther Methods Clin Dev 17: , 83–98. |
[23] | Biju K , Zhou Q , Li G , Imam SZ , Roberts JL , Morgan WW , Clark RA , Li S ((2010) ) Macrophage-mediated GDNF delivery protects against dopaminergic neurodegeneration: A therapeutic strategy for Parkinson’s disease. Mol Ther 18: , 1536–1544. |
[24] | Biju KC , Santacruz RA , Chen C , Zhou Q , Yao J , Rohrabaugh SL , Clark RA , Roberts JL , Phillips KA , Imam SZ , Li S ((2013) ) Bone marrow-derived microglia-based neurturin delivery protects against dopaminergic neurodegeneration in a mouse model of Parkinson’s disease. Neurosci Lett 535: , 24–29. |
[25] | Zhao Y , Haney MJ , Gupta R , Bohnsack JP , He Z , Kabanov AV , Batrakova EV ((2014) ) GDNF-transfected macrophages produce potent neuroprotective effects in Parkinson’s disease mouse model. PLoS One 9: , e106867. |
[26] | Ge G , Chen C , Guderyon MJ , Liu J , He Z , Yu Y , Clark RA , Li S ((2018) ) Regulatable lentiviral hematopoietic stem cell gene therapy in a mouse model of Parkinson’s disease. Stem Cells Dev 27: , 995–1005. |
[27] | Chen C , Li X , Ge G , Liu J , Biju KC , Laing SD , Qian Y , Ballard C , He Z , Masliah E , Clark RA , O’Connor JC , Li S ((2018) ) GDNF-expressing macrophages mitigate loss of dopamine neurons and improve Parkinsonian symptoms in MitoPark mice. Sci Rep 8: , 5460. |
[28] | Zhao Y , Haney MJ , Jin YS , Uvarov O , Vinod N , Lee YZ , Langworthy B , Fine JP , Rodriguez M , El-Hage N , Kabanov AV , Batrakova EV ((2019) ) GDNF-expressing macrophages restore motor functions at a severe late-stage, and produce long-term neuroprotective effects at an early-stage of Parkinson’s disease in transgenic Parkin Q311X(A) mice. J Control Release 315: , 139–149. |
[29] | Newland B , Dunnett SB , Dowd E ((2016) ) Targeting delivery in Parkinson’s disease. Drug Discov Today 21: , 1313–1320. |
[30] | Newland B , Dowd E , Pandit A ((2013) ) Biomaterial approaches to gene therapies for neurodegenerative disorders of the CNS. Biomater Sci 1: , 556–576. |
[31] | Newland B , Newland H , Werner C , Rosser A , Wang W ((2015) ) Prospects for polymer therapeutics in Parkinson’s disease and other neurodegenerative disorders. Prog Polym Sci 44: , 79–112. |
[32] | Torres-Ortega PV , Saludas L , Hanafy AS , Garbayo E , Blanco-Prieto MJ ((2019) ) Micro- and nanotechnology approaches to improve Parkinson’s disease therapy. J Control Release 295: , 201–213. |
[33] | Garbayo E , Ansorena E , Lana H , Carmona-Abellan MD , Marcilla I , Lanciego JL , Luquin MR , Blanco-Prieto MJ ((2016) ) Brain delivery of microencapsulated GDNF induces functional and structural recovery in parkinsonian monkeys. Biomaterials 110: , 11–23. |
[34] | Requejo C , Ruiz-Ortega JA , Bengoetxea H , Garcia-Blanco A , Herran E , Aristieta A , Igartua M , Ugedo L , Pedraz JL , Hernandez RM , Lafuente JV ((2015) ) Topographical distribution of morphological changes in a partial model of Parkinson’s disease–Effects of nanoencapsulated neurotrophic factors administration. Mol Neurobiol 52: , 846–858. |
[35] | Herran E , Ruiz-Ortega JA , Aristieta A , Igartua M , Requejo C , Lafuente JV , Ugedo L , Pedraz JL , Hernandez RM ((2013) ) In vivo administration of VEGF- and GDNF-releasing biodegradable polymeric microspheres in a severe lesion model of Parkinson’s disease. Eur J Pharm Biopharm 85: , 1183–1190. |
[36] | Garbayo E , Ansorena E , Lanciego JL , Blanco-Prieto MJ , Aymerich MS ((2011) ) Long-term neuroprotection and neurorestoration by glial cell-derived neurotrophic factor microspheres for the treatment of Parkinson’s disease. Mov Disord 26: , 1943–1947. |
[37] | Garbayo E , Montero-Menei CN , Ansorena E , Lanciego JL , Aymerich MS , Blanco-Prieto MJ ((2009) ) Effective GDNF brain delivery using microspheres–a promising strategy for Parkinson’s disease. J Control Release 135: , 119–126. |
[38] | Jollivet C , Aubert-Pouessel A , Clavreul A , Venier-Julienne MC , Montero-Menei CN , Benoit JP , Menei P ((2004) ) Long-term effect of intra-striatal glial cell line-derived neurotrophic factor-releasing microspheres in a partial rat model of Parkinson’s disease. Neurosci Lett 356: , 207–210. |
[39] | Jollivet C , Aubert-Pouessel A , Clavreul A , Venier-Julienne MC , Remy S , Montero-Menei CN , Benoit JP , Menei P ((2004) ) Striatal implantation of GDNF releasing biodegradable microspheres promotes recovery of motor function in a partial model of Parkinson’s disease. Biomaterials 25: , 933–942. |
[40] | Meng XY , Huang AQ , Khan A , Zhang L , Sun XQ , Song H , Han J , Sun QR , Wang YD , Li XL ((2020) ) Vascular endothelial growth factor-loaded poly-lactic-co-glycolic acid nanoparticles with controlled release protect the dopaminergic neurons in Parkinson’s rats. Chem Biol Drug Des 95: , 631–639. |
[41] | Bensadoun JC , Pereira de Almeida L , Fine EG , Tseng JL , Deglon N , Aebischer P ((2003) ) Comparative study of GDNF delivery systems for the CNS: Polymer rods, encapsulated cells, and lentiviral vectors. J Control Release 87: , 107–115. |
[42] | Gouhier C , Chalon S , Aubert-Pouessel A , Venier-Julienne MC , Jollivet C , Benoit JP , Guilloteau D ((2002) ) Protection of dopaminergic nigrostriatal afferents by GDNF delivered by microspheres in a rodent model of Parkinson’s disease. Synapse 44: , 124–131. |
[43] | Schirmer L , Hoornaert C , Le Blon D , Eigel D , Neto C , Gumbleton M , Welzel PB , Rosser AE , Werner C , Ponsaerts P , Newland B ((2020) ) Heparin-based, injectable microcarriers for controlled delivery of interleukin-13 to the brain. Biomater Sci 8: , 4997–5004. |
[44] | Kulkarni AD , Vanjari YH , Sancheti KH , Belgamwar VS , Surana SJ , Pardeshi CV ((2015) ) Nanotechnology-mediated nose to brain drug delivery for Parkinson’s disease: A mini review. J Drug Target 23: , 775–788. |
[45] | Aly AE , Waszczak BL ((2015) ) Intranasal gene delivery for treating Parkinson’s disease: Overcoming the blood-brain barrier. Expert Opin Drug Deliv 12: , 1923–1941. |
[46] | Rehman S , Nabi B , Zafar A , Baboota S , Ali J ((2019) ) Intranasal delivery of mucoadhesive nanocarriers: A viable option for Parkinson’s disease treatment? Expert Opin Drug Deliv 16: , 1355–1366. |
[47] | Yue P , Gao L , Wang X , Ding X , Teng J ((2017) ) Intranasal administration of GDNF protects against neural apoptosis in a rat model of Parkinson’s disease through PI3K/Akt/GSK3beta pathway. Neurochem Res 42: , 1366–1374 . |
[48] | Migliore MM , Ortiz R , Dye S , Campbell RB , Amiji MM , Waszczak BL ((2014) ) Neurotrophic and neuroprotective efficacy of Intranasal GDNF in a rat model of Parkinson’s disease. Neuroscience 274: , 11–23. |
[49] | Gartziandia O , Herran E , Ruiz-Ortega JA , Miguelez C , Igartua M , Lafuente JV , Pedraz JL , Ugedo L , Hernandez RM ((2016) ) Intranasal administration of chitosan-coated nanostructured lipid carriers loaded with GDNF improves behavioral and histological recovery in a partial lesion model of Parkinson’s disease. J Biomed Nanotechnol 12: , 2220–2230. |
[50] | Hernando S , Herran E , Figueiro-Silva J , Pedraz JL , Igartua M , Carro E , Hernandez RM ((2018) ) Intranasal administration of TAT-conjugated lipid nanocarriers loading GDNF for Parkinson’s disease. Mol Neurobiol 55: , 145–155. |
[51] | Bender TS , Migliore MM , Campbell RB , John Gatley S , Waszczak BL ((2015) ) Intranasal administration of glial-derived neurotrophic factor (GDNF) rapidly and significantly increases whole-brain GDNF level in rats. Neuroscience 303: , 569–576. |
[52] | Jayant RD , Sosa D , Kaushik A , Atluri V , Vashist A , Tomitaka A , Nair M ((2016) ) Current status of non-viral gene therapy for CNS disorders. Expert Opin Drug Deliv 13: , 1433–1445. |
[53] | Boado RJ , Pardridge WM ((2011) ) The Trojan horse liposome technology for nonviral gene transfer across the blood-brain barrier. J Drug Deliv 2011: , 296151. |
[54] | Zhang Y , Pardridge WM ((2009) ) Near complete rescue of experimental Parkinson’s disease with intravenous, non-viral GDNF gene therapy. Pharm Res 26: , 1059–1063. |
[55] | Xia CF , Boado RJ , Zhang Y , Chu C , Pardridge WM ((2008) ) Intravenous glial-derived neurotrophic factor gene therapy of experimental Parkinson’s disease with Trojan horse liposomes and a tyrosine hydroxylase promoter. J Gene Med 10: , 306–315. |
[56] | Price RJ , Fisher DG , Suk JS , Hanes J , Ko HS , Kordower JH ((2019) ) Parkinson’s disease gene therapy: Will focused ultrasound and nanovectors be the next frontier? Mov Disord 34: , 1279–1282. |
[57] | Umlauf BJ , Shusta EV ((2019) ) Exploiting BBB disruption for the delivery of nanocarriers to the diseased CNS. Curr Opin Biotechnol 60: , 146–152. |
[58] | Nance E , Timbie K , Miller GW , Song J , Louttit C , Klibanov AL , Shih TY , Swaminathan G , Tamargo RJ , Woodworth GF , Hanes J , Price RJ ((2014) ) Non-invasive delivery of stealth, brain-penetrating nanoparticles across the blood-brain barrier using MRI-guided focused ultrasound. J Control Release 189: , 123–132. |
[59] | Lin CY , Hsieh HY , Chen CM , Wu SR , Tsai CH , Huang CY , Hua MY , Wei KC , Yeh CK , Liu HL ((2016) ) Non-invasive, neuron-specific gene therapy by focused ultrasound-induced blood-brain barrier opening in Parkinson’s disease mouse model. J Control Release 235: , 72–81. |
[60] | Yue P , Gao L , Wang X , Ding X , Teng J ((2018) ) Ultrasound-triggered effects of the microbubbles coupled to GDNF- and Nurr1-loaded PEGylated liposomes in a rat model of Parkinson’s disease. J Cell Biochem 119: , 4581–4591. |
[61] | Yue P , Miao W , Gao L , Zhao X , Teng J ((2018) ) Ultrasound-triggered effects of the microbubbles coupled to GDNF plasmid-loaded PEGylated liposomes in a rat model of Parkinson’s disease. Front Neurosci 12: , 222. |
[62] | Lin CY , Lin YC , Huang CY , Wu SR , Chen CM , Liu HL ((2020) ) Ultrasound-responsive neurotrophic factor-loaded microbubble- liposome complex: Preclinical investigation for Parkinson’s disease treatment. J Control Release 321: , 519–528. |
[63] | Niu J , Xie J , Guo K , Zhang X , Xia F , Zhao X , Song L , Zhuge D , Li X , Zhao Y , Huang Z ((2018) ) Efficient treatment of Parkinson’s disease using ultrasonography-guided rhFGF20 proteoliposomes. Drug Deliv 25: , 1560–1569. |
[64] | Mead BP , Kim N , Miller GW , Hodges D , Mastorakos P , Klibanov AL , Mandell JW , Hirsh J , Suk JS , Hanes J , Price RJ ((2017) ) Novel focused ultrasound gene therapy approach noninvasively restores dopaminergic neuron function in a rat Parkinson’s disease model. Nano Lett 17: , 3533–3542. |
[65] | Wang X , Cui G , Yang X , Zhang Z , Shi H , Zu J , Hua F , Shen X ((2014) ) Intracerebral administration of ultrasound-induced dissolution of lipid-coated GDNF microbubbles provides neuroprotection in a rat model of Parkinson’s disease. Brain Res Bull 103: , 60–65. |
[66] | Fan CH , Ting CY , Lin CY , Chan HL , Chang YC , Chen YY , Liu HL , Yeh CK ((2016) ) Noninvasive, targeted, and non-viral ultrasound-mediated GDNF-plasmid delivery for treatment of Parkinson’s disease. Sci Rep 6: , 19579. |